DOI:
10.1039/D4FB00135D
(Review Article)
Sustainable Food Technol., 2024,
2, 1652-1669
Gingerol: extraction methods, health implications, bioavailability and signaling pathways
Received
3rd May 2024
, Accepted 25th August 2024
First published on 9th September 2024
Abstract
Ginger (Zingiber officinale L. Z.o.) is a well-known spice that has been used for centuries as a food ingredient and in traditional medicine. One of the primary active components of ginger is gingerol, which has been studied extensively for its potential health benefits and has significant anti-inflammatory, antioxidant, antitumor, and antiulcer properties, confirming traditional use of ginger in ancient medicine as a home remedy for various ailments. Gingerol extraction techniques, health implications, bioavailability, and targeting signaling pathways in the gastrointestinal (GI) tract are areas of active research because it may be a promising therapeutic agent for various GI disorders including obesity, inflammation, diabetes, cancer and functional GI disorder. This review paper provides an overview of the current understanding of gingerol extraction techniques, the potential health benefits associated with gingerol consumption, and the mechanisms of action by which gingerol exerts its effects in the GI tract. In addition, this paper highlights the challenges associated with achieving optimal bioavailability of gingerol and potential strategies for improving its bioavailability. Finally, this paper explores the potential of targeting signaling pathways in the GI tract as a means of enhancing the therapeutic efficacy of gingerol. The research summarized in this abstract suggests that gingerol may be a promising therapeutic agent for various GI disorders. However, further research is needed to fully understand the mechanisms by which gingerol exerts its effects and to optimize its delivery and dosing for maximal therapeutic benefit.
Sustainability spotlight
Ginger (Zingiber officinale L. Z.o.) is a well-known spice that has been used for centuries as a food ingredient and in traditional medicine. One of the primary active components of ginger is gingerol. Gingerol has potential health benefits, including its impact on cancer, diabetes, obesity, inflammation, and functional GI problems. To maximise medicinal advantages, research has concentrated on creating efficient extraction methods for gingerol and enhancing its bioavailability. Gingerol's anti-inflammatory, antioxidant, anti-diabetic, and anti-obesity characteristics have a wide range of health consequences. Although it has poor bioavailability, there are a number of ways to increase its effectiveness and absorption. Ginger offers a traditional solution to achieve improved nutrition, health and wellbeing.
|
1. Introduction
Southeast Asian ginger rhizomes are grown worldwide. Old English, Greek, Mediaeval Latin, and Prakrit call Zingiber officinale ginger, gingiber, and Singapura. Ginger blooms perennially. Ginger (Zingiber officinale L.) grows 100 cm pseudostems with thin leaf blades. Ginger blooms have purple-edged yellow petals. Rhizomes produce single stems and bitter, red, and white ginger are the main gingers.1 Ginger contains over 100 compounds.2 Oleoresin (5–8%) and lipids or glycolipids (9%) give ginger its pungent taste. Ginger contains unpleasant compounds and 1–3% volatile oils. Fresh gingerols are the strongest. The most common gingerol chain length is 6.3 Ginger may cure constipation, ulcers, arthritis, rheumatism, atherosclerosis, hypertension, diabetes, vomiting, and cancer.4 Anti-inflammatory and anti-oxidative properties decrease ageing.5,6 Ginger is pungent due to gingerols, shogaols, paradols, and zingerone.7 Functionally, pharmacological characteristics including anti-inflammatory, antioxidant, and anti-tumor actions are shared by gingerol, shogaols, paradols, and zingerone. They may be helpful in the prevention and treatment of diabetes and obesity because of their comparable effects on glucose absorption and energy metabolism.1,2 Additionally, it has been shown that the bioavailability and metabolism of these substances are comparable, which may help to explain some of their common health effects. Overall, despite certain variations in these compound potencies and unique modes of action, their commonalities raise the possibility that they may combine to provide health advantages of ginger.
There are significant structural and functional commonalities among the bioactive substances found in ginger, including gingerol, shogaols, paradols, and zingerone. They are all members of the group of substances known as gingerols, which are extracted from the rhizome of the ginger plant. Gingerol, a bioactive compound of ginger occurs in different forms viz., 4-gingerol, 6-gingerol, 8-gingerol, 10-gingerol, and 12-gingerol. Among these 6-gingerol and 6-shogaol are profoundly present in fresh and dried ginger rhizomes, respectively.8 A dehydrated bioactive metabolite of 6-gingerol known as 6-shogaol is attained after dying and long-term storage of ginger.9 6-Gingerol has a molecular weight of 294.4 g mol−1 and a chemical formula of C17H26O4. It is poorly soluble in water but soluble in organic solvents. Known for its anti-inflammatory, antioxidant, and anticancer properties, 6-gingerol is metabolized in the gastrointestinal tract and liver, primarily as glucuronides, with a plasma half-life of 1 to 3 hours. Gingerol extraction has been the subject of research and there are two categories of gingerol extraction viz conventional and advanced methods. Conventional techniques such as soaking, maceration, and heat extraction are simple but have drawbacks such as the toxicity of solvent and a poor yield of gingerol.10,11 Advance methods overcome the above drawbacks and considered as more efficient methods for extraction of gingerol. Advance methods such as microwave assisted extraction (MAE), pressure liquid extraction (PLE), supercritical fluid extraction (SFE), ultrasound assisted extraction (UAE), pulsed electric field extraction (PEFE), and enzyme assisted extraction are efficient, distinct, and environment friendly.12,13
Gingerol is sensitive to heat and undergoes reduction when exposed to high temperatures. The health benefits associated with ginger are attributed to its abundant phytochemical content. The health-enhancing effects of ginger is assigned to its profuse “phytochemistry”. 6- gingerol is a major pharmacologically active compound known for its anti-inflammatory, antioxidant and anti-cancerous properties. 6-Gingerol is a water-soluble compound and has an optimal lipophilicity, with a partition coefficient between that of n-octanol and water (log
Po/w) of 3.13. Therefore, it possesses high gastrointestinal absorption and good blood–brain barrier permeation.14 One of gingerol’s most important advantages is its strong anti-inflammatory properties, which may help lower bodily inflammation and guard against chronic illnesses including cancer, cardiovascular disease, and arthritis.15–18 Additionally, gingerol has the ability to scavenge free radicals and lessen oxidative stress, which may help shield cells and tissues from harm and fend off illnesses linked to ageing. Since it improved glucose metabolism, increased insulin sensitivity, and decreased postprandial blood glucose levels, gingerol has also been demonstrated to have anti-diabetic properties.19,20 Gingerol is known to impede the enzymes nitric oxide synthase and cyclo-oxygenase21 and to restrain the spreading of tumors.22 The characteristic anti-inflammatory properties of gingerol are due to its ability to inhibit the secretion of prostaglandin E2 (PGE2) and tumor necrosis factor-α (TNF-α) in lipopolysaccharide (LPS)-activated RAW264.7.
Gastrointestinal (GI) diseases encompass a wide range of disorders affecting the digestive tract, including irritable bowel syndrome (IBS), inflammatory bowel disease (IBD), gastroesophageal reflux disease (GERD), peptic ulcers, and gastrointestinal cancers.23 GI diseases are prevalent worldwide, with varying incidence rates; IBD is more common in Western countries, while infectious GI diseases are more prevalent in developing regions. The World Gastroenterology Organisation notes that GI diseases significantly contribute to the global burden of disease, with colorectal cancer being the third most common cancer and a leading cause of cancer-related deaths.24 Discussing GI diseases in this review is essential due to their widespread impact on public health, quality of life, and healthcare costs. Chronic GI conditions cause pain, discomfort, and dietary restrictions, emphasizing the need for effective management strategies.
In conclusion, gingerol's anti-inflammatory, antioxidant, anti-diabetic, and anti-obesity characteristics have a wide range of health consequences. Although it has poor bioavailability, there are a number of ways to increase its effectiveness and absorption. The diverse processes involved in gingerol's signalling pathways emphasize the compound's potential as a treatment for a range of illnesses and medical disorders. The novelty of the article comes in its thorough examination of the state of knowledge about gingerol extraction methods, possible health advantages, and GI tract action mechanisms. The difficulties of getting the best bioavailability of gingerol are also discussed in the paper, along with possible solutions. This review paper also investigates the possibility of improving gingerol's medicinal effectiveness by focusing on signaling pathways in the GI tract. The review article offers an up-to-date and thorough assessment of the studies in this area, and its conclusions point to gingerol's potential as a treatment for a number of GI illnesses. To completely comprehend gingerol's mechanisms of action and to optimize its distribution and dosage for optimum therapeutic efficacy, more study is necessary. The urgency to expedite the development of this review arises from the critical necessity to elucidate the therapeutic efficacy of gingerol amidst the burgeoning prevalence of chronic ailments globally. Through a meticulous examination of its pharmacological attributes and underlying mechanisms, this review seeks to pave the path for the formulation of innovative therapeutic modalities harnessing the therapeutic potential of gingerol.
1.1 Materials and methods
A systematic approach was adopted to comprehensively gather and analyze the literature pertinent to gingerol. A systematic review was conducted utilizing established databases such as Google Scholar, PubMed, Academia, and additional reputable repositories. The search strategy encompassed keywords such as “gingerol extraction,” “gingerol health effects,” “gingerol bioavailability,” and “gingerol signaling pathways.” Inclusion criteria were meticulously defined to ensure relevance and quality of the gathered literature. Peer-reviewed articles, scholarly publications, and reputable academic sources were included, spanning a timeframe from inception to present, thus ensuring a robust and up-to-date publication knowledge of gingerol.
Each retrieved publication underwent a rigorous evaluation process, wherein relevance, methodological soundness, and contribution to the understanding of gingerol were scrutinized. Only studies meeting predefined quality criteria were included in the review, thereby upholding the integrity and validity of the summarized findings.
Data extraction encompassed pertinent information pertaining to gingerol extraction methodologies, health implications, bioavailability determinants, and elucidation of underlying signaling pathways. Key findings and insights from each included study were systematically collated and summarized to offer a comprehensive overview of the diverse facets of gingerol.
2. Extraction of gingerol
The extraction methods of gingerol can be widely categorized into two groups, i.e. first, conventional methods which include maceration, soaking, and heat extraction which are usually easy to carry out and don't require specific instrumentation. On the other hand, advanced methods such as microwave-assisted extraction (MAE), pressure liquid extraction (PLE), supercritical fluid extraction (SFE), ultrasound-assisted extraction (UAE), pulsed electric field extraction (PEFE), and enzyme-assisted extraction (EAE) require specific instrumentation as well as proper laboratory conditions. Conventional methods are easy to carry out but have certain limitations such as toxicity of the solvent, and a little mishandling leading to traces of solvent in the extract.
2.1. Conventional methods
Traditionally, gingerol can be extracted from dried or raw ginger powder by a simple maceration process. Conventional extraction methods, such as heat reflux, distillation, percolation, maceration, and Soxhlet extraction usually require high energy, time, and a huge quantity of organic solvent and produce a low yield of the active compounds,10,11 where dried ginger powder was extracted with 95 percent ethanol and then later, the solvent was evaporated and a thick paste was obtained. This paste was then suspended in water. The common organic solvents used for extraction include methanol, ethanol, ethyl acetate, distilled water, etc. The precipitate formed by the ginger resin was filtered and the leftovers were vacuum dried to obtain gingerol.25 Traditional methods for obtaining ginger extracts include Soxhlet or hot percolation extractions, which are regarded as industry standards.26,27 High temperatures also cause degradation of thermally sensible substances. The method's high organic solvent usage is yet another disadvantage. Cold percolation can be utilized as a reference approach for the extraction of thermolabile chemicals as it employs room temperature, but the application is not appealing due to the prolonged extraction periods, high cost, and low yields.28 Therefore, unconventional techniques may be able to overcome these problems and produce high-quality extracts with high yields, little time and energy requirements, and minimal environmental effect. Methanol and ethanol are more popular solvents for extracting ginger rhizomes.26,27,29 After 2 hours of percolation extraction, Ko et al.29 found that ethanol at 60 °C produced samples with higher concentrations of 6-gingerol (0.48 mg g−1) and antioxidant activity (5.11 mg TE per g), but water at 90 °C produced samples with lower concentrations of 6-gingerol (0.74 mg g−1) and no antioxidant capacity. In comparison to acetone or hexane, Shukla et al.26 found that ethanol reflux extraction produced greater global yields (9.8%) and 6-gingerol recovery (22.1 mg g−1). The selectivity of ethanol for ginger extraction over other solvents was underlined by the authors, which may be a result of its polarity.
2.2. Advanced methods
Advanced methods of extraction are cutting-edge techniques that can be used for their effectiveness, environmental friendliness, and distinctness. These newly developed sophisticated green extraction technologies support high efficiency, economic viability, reduced energy use, and environmental friendliness in line with sustainable development plans. Chemat et al.30 gave six principles of green extraction viz., use of minimal organic solvent; less energy consumption; less extraction time; well-reasoned sourcing; high value by-product; safe extraction. Advanced methods of exaction have overcome the limitations of conventional methods. Gingerols due to their thermal sensitive nature are prone to dehydration at 60 °C and above.31 Therefore, conventional extraction methods, such as Soxhlet extraction and percolation extraction, have low efficiency due to either high temperature or long extraction time.28
2.2.1. Microwave assisted extraction (MAE).
The microwave-assisted extraction method gained popularity probably due to its better yield, less time consumption, no residual solvents, eco-friendliness, etc. Recently, the usage of microwaves as a heating source affords fast in situ heating of volatiles.12,32,33 Microwave-assisted techniques have been described as time-saving, energy saving and highly efficient.34 This technique follows simple steps which involve the extraction of dehydrated ginger powder with ethanol in a 1
:
10 proportion at different powers (usually 400–600 W) and different temperatures (50–70 °C) for 20–30 min. Further extract dilution was carried out using rota vapor at 50 °C under 40 millibars reduced pressure. In an experiment, it was reported that the optimum conditions for extraction of gingerol were a microwave power of 528 W, a ratio of liquid to solid of 26 mL g−1, an extraction time of 31 s, and an ethanol proportion of 78%, whereas Guo et al.35 conducted MAE through ionic solvents and reported that [C10MIM]Br has the highest extraction yields of gingerols and shogaols, followed by [C8MIM]Br, [C6MIM]Br, [C10MIM]Cl, and [C10MIM]ToS. Ionic solvents (ILs), consist of a few organic salts in the liquid state that comprise organic cations coupled with organic or inorganic anions.35 In another case Teng et al.33 carried out the extraction process using five solvents i.e. ethanol, methanol, acetone, petroleum ether, and hexane. The high recovery of 6-gingerol was obtained with ethanol (9.24 mg g−1) while the highest shogaol recovery was observed with hexane. Also, the optimal conditions were 70% concentration of ethanol and an extraction time of 10 min with 180 W microwave power. MAE is an interesting method due to the fact that high temperature caused due to microwave heating reduces extraction time and the volume of solvent required for extraction. But it has a limitation that appropriate extraction conditions must be chosen carefully to avoid thermal degradation of the compound.
2.2.2. Pressure liquid extraction (PLE).
The pressure liquid extraction method involved the utilization of different pressures and temperatures for the extraction process. PLE works based on a principle similar to that of Soxhlet extraction, except that high temperatures and pressures are used in enclosed vessels, and requires a small amount of solvent for extraction and is completed in a very short amount of time.13,36 It was recognized that hot and pressurized solvents were more likely to dissolve the compounds effectively and penetrate the sample matrices.37,38
2.2.3. Supercritical fluid extraction (SFE).
Supercritical fluid carbon dioxide extraction was carried out under relatively mild operating conditions (Tc = 31 °C and Pc = 7.38 MPa) with a non-toxic solvent and completed with the separation of solvent by simply depressurizing.26,39–41 In a study conducted by Balachandran et al.42 supercritical fluid carbon dioxide extraction (SCFE-CO2) at 16 MPa and 40 °C was used to differentiate extraction yields from fresh, oven-dried and freeze-dried ginger. They found that higher yield and pungent gingerol content were obtained with fresh ginger samples as compared to the oven or air-dried ginger samples. Fitriady et al.43 suggested that at 40 °C and 4500 Psi in SFE of ginger with CO2 as the solvent for 4 hours produced the best yield of ginger extract. However, by curve fitting analysis, he suggested 42 °C as the temperature for 5 hours, 7 minutes, and 30 seconds to achieve the maximum yield. Souza et al.44 reported that the best conditions for SFE for ginger was 100 bar and 40 °C (0.0508 g oil per g plant) with 19.34% of α-zingiberene; for the SD, 3 bar (133 °C) (0.00616 g oil/g plant) with 28.9% of α-zingiberene; and HD, the volume of 750 mL (0.006988 g oil per g plant) with 15.70% of α-zingiberene, all measured on a dry weight basis.
2.2.4. Ultrasound-assisted extraction (UAE).
Ultrasonication or ultrasound-assisted extraction (UAE) is a novel and turbulent technique that was found to be effective in the extraction of polyphenols and other value-added compounds. In an aqueous solution, the ultrasound waves result in cavitations, a phenomenon in which the induction of bubbles within a liquid medium occurs.36,45,46 Cavitations arise due to the pressure waves formed by the mechanical vibrations in the ultrasonication device. Bubbles are formed within liquid mediums as a result of pressure wave compression and rarefaction regions. The bubbles created grow in size along the pressure waves and can rise to the surface and coalesce due to Bjerknes forces. On the other hand, these bubbles can expand and finally collapse during the compression of the wave. This collapse is an adiabatic process and leads to very high localized temperatures (in the range of 5000 K) and pressures of 1000 atm.12 Murphy et al.47 suggested that maximum polyphenols are extracted from 1200 mg of spice/20 mL sample in 86% (v/v) ethanol by subjecting it to ultrasound treatment for 11 min at 65 °C (1039.64 mg GAE per g of dw) using an ultrasonic frequency of 35 kHz.
2.2.5. Pulsed electric field (PEF) extraction.
Pulse electric field (PEF) extraction is a non-thermal, eco-friendly, and selective extraction method that does not need energy and was proven to be efficient in short extraction durations.48,49 The pore formation in the weak areas of the cell membrane due to electroporation results in an increase in membrane permeability. This ultimately results in the diffusion of more bioactive compounds from the plant tissue. Several factors influence the performance of PEF, including the intensity of the pulse-specific energy, electric field, pulse width, pulse shape, number of pulses, and frequency of the pulses.50,51 The idea behind pulsed electric field extraction is to apply a strong electric field in brief pulses that last between microseconds and milliseconds and have an intensity between 10 and 80 kV cm−1.52 By using PEFs at ambient temperatures, minimizing extraction time, and reducing solvent quantities, we reduce energy consumption and financial costs, as well as the degradation of temperature-sensitive compounds. PEF extraction is a promising green alternative extraction technique because of its above-mentioned properties and its selectivity, enhancing the recovery of bioactive compounds from plants and food industry waste, allowing for the production of bio-functional foods, food supplements, and drug precursors.48,53
2.2.6. Enzyme-assisted extraction (EAE).
Enzyme-assisted extraction (EAE) is known to facilitate the extraction of vital components of plant material in higher yield. Utilization of enzymes for fresh ginger oil extraction takes longer time and it is reported that the enzyme extraction method yields 50% higher volatile oil from fresh ginger.54 Manasa et al.55 reported that a higher yield of gingerol (12.2% ± 0.4) was obtained by treatment of ginger with viscozyme or amylase followed by acetone extraction as compared to the control (6.4% ± 0.4 gingerol). Varakumar et al.28 found that enzymatic pretreatment using accellerase increased the yield of 6, 8, 10-gingerols, and 6-shogaol by 64.10, 87.8, 62.78 and 32.0% within 4 h. Table 1 summarizes the different extraction methods used for the extraction of gingerols and the solvent use, conditions, and concentration of extracted compounds.
Table 1 The different extraction methods of gingerols from ginger and its concentration
S. no. |
Extraction method |
Solvent used, compound extracted, and optimal conditions |
Concentration |
References |
A
|
Conventional methods
|
1 |
Maceration extraction |
Methanol, gingerols and shogaol, time: 14 h |
7084 (μg bioactive per g dried ginger) |
56
|
2 |
Soxhlet extraction |
Ethanol, gingerols and shogaol |
8335 (μg bioactive per g dried ginger) |
56
|
3 |
Maceration extraction |
Acetone/ethanol/methanol |
208.6 mg L−1/258.4 mg L−1/183.7 mg L−1 |
57
|
6-Gingerol, 20 g of the sample soaked in 200 mL of each solvent separately, 200 rpm for 8 h in an orbital shaker, 10 min heating, evaporation 40 °C |
|
|
4 |
Cold percolation |
Methanol, oleoresin, 6-gingerol, 6-shogaol, 8-gingerol, and 10-gingerol, temp.: ∼32 °C, time: 8 h |
35 ± 8 g kg−1 |
28
|
2105.4 ± 124 μg g−1 |
1391.9 ± 132 μg g−1 |
451.5 ± 102 μg g−1 |
541.2 ± 83 μg g−1 |
5 |
Maceration extraction |
Ethanol, 100 g of roots were soaked in 500 mL absolute ethanol for 72 h and then filtered |
|
58
|
6 |
Maceration extraction |
Carbon dioxide, 6-Gingerol, 1-Pres:2 MPa; temp.: 40 °C for 3 h 2-Pres:1 MPa; temp.: 45 °C for 3 h, 3- Pres: 2 MPa; temp.: 45 °C for 5 h |
26.32 mg per g d.w. |
2
|
26.28 mg per g d.w. |
24.72 mg per g d.w. |
|
7 |
Soxhlet extraction |
Ethanol, gingerol, 20 g sample with 100 mL of absolute ethanol, temperature: 78.4 °C for 12 h, 5–8 cycles in a heating mantle for 1 h |
|
58
|
8 |
Soxhlet extraction |
Methanol, oleoresin, 6-gingerol, 6-shogaol, 8-gingerol, and 10-gingerol, temp.: above 65 °C, time: 8 h |
52 ± 5 g kg−1 |
28
|
4206.3 ± 156 μg g−1 |
2084.9 ± 112 μg g−1 |
597.5 ± 82 μg g−1 |
653.7 ± 94 μg g−1 |
![[thin space (1/6-em)]](https://www.rsc.org/images/entities/char_2009.gif) |
B
|
Advanced methods
|
9 |
Enzyme-assisted SWE |
Acetone/ethanol, gingerol, sprayed with enzyme separatecellulase, pectinase, protease, alpha amylase viscozyme, incubated at 50 ± 2 C for a period of 30–120 min |
Acetone 10.1 |
59
|
|
Ethano l6.2 |
|
|
|
|
10 |
Hydro distillation extraction |
Water, 6-gingerol, solid to solvent ratio: 1 : 20 (g mL−1); time: 90 min; drying temp: 50 °C |
35.3404 mg L−1 |
60
|
11 |
Sonication extraction |
Ethanol, gingerols and shogaol, 0.5 h + 0.5 h = 1h |
1393.5 (μg bioactive per g dried ginger) |
56
|
12 |
Enzyme-assisted SWE |
Ethanol, gingerols and shogaol, 1 h + 0.5 h = 1.5 h |
2990.5 (μg bioactive per g dried ginger) |
56
|
13 |
Sonication extraction |
75% methanol in water (v/v), 80 mL, zingerone, 6-gingerol, 8-gingerol, 6- shogaol, and 10-gingerol, time: 120 min |
3.80–8.11 ng mL−1 |
61
|
14 |
Microwave assisted extraction |
100 mM hyodeoxycholic acid sodium salt in water, 20 mL, zingerone, 6-gingerol, 8-gingerol, 6-shogaol, and 10-gingerol, time: 10 min, temp.: 60 °C |
3.80–8.11 ng mL−1 |
61
|
15 |
Three phase partitioning (TTP) |
10% (w/v) ammonium sulphate, oleoresin, 6-gingerol, 6-shogaol, 8-gingerol, and 10-gingerol, slurry: t-butanol ratio (1 : 0.5), 5% (w/v) solid load, pH 5, temp.: ∼32 °C |
61.6 ± 3.4 g kg−1 |
28
|
6741.0 ± 182 μg g−1 |
1758.6 ± 124 μg g−1 |
1120.2 ± 98 μg g−1 |
1021.0 ± 87 μg g−1 |
16 |
Enzyme assisted three phase partitioning (EATPP) |
10% (w/v) ammonium sulphate, oleoresin, 6-gingerol, 6-shogaol, 8-gingerol, and 10-gingerol, enzyme:stargen or accellerase 0.5% (v/v), temp: 50 °C, slurry: t-butanol ratio (1 : 0.5), 5% (w/v) solid load, pH 5, extraction temp.: ∼32 °C, time: 4 h |
69 ± 9.0 g kg−1 |
28
|
6902.7 ± 113 μg g−1 |
2752.3 ± 102 μg g−1 |
1122.1 ± 112 μg g−1 |
1064.1 ± 56.5 μg g−1 |
17 |
Ultrasound assisted three phase partitioning (UATPP) |
10% (w/v) ammonium sulphate, oleoresin, 6-gingerol, 6-shogaol, 8-gingerol, and 10-gingerol duty cycle at 40% and a power output of 36W + 10% (w/v), ammonium sulphate, slurry: t-butanol ratio (1 : 0.5), 5% (w/v) solid load, pH 5, extraction temp: ∼32 °C |
64 ± 5.6 g kg−1 |
28
|
6982.0 ± 95 μg g−1 |
6982.0 ± 95 μg g−1 |
1182.5 ± 32.5 μg g−1 |
1023.5 ± 142 μg g−1 |
18 |
Subcritical water extraction (SWE) |
Deionized water with 2% ethanol and 2% acetone, total gingerol & shogaol, tempe.: 130 °C, pressure: 20 bars, time: 30 min |
2990.5 5 μg bioactive per g dried ginger |
62
|
19 |
Ionic liquid-based ultrasonic-assisted extraction (ILUAE) |
Aqueous ionic liquid, total gingerol, temp.: 25 °C, solid/liquid ratio: 1 : 20 (g mL−1). Time: 10 min, ultrasonic power: 200 W |
12.21 mg g−1 |
63
|
|
|
20 |
Reflux cooling |
Ethanol, gingerol andshogaol, 70% ethanol, time: 70 min, temp: 70 °C, 70% ethanol, time: 51.9 min, temp: 62.29 °C |
2.8 mg g−1 |
64
|
1.3 mg g−1 |
|
|
|
21 |
Microwave assisted extraction |
Ethanol, gingerol, shogaol, paradol, zingerone, 95% ethanol, power: 400W, time: 1 min |
71.5 ± 3.6 mg g−1 |
32
|
12.5 ± 1.0 mg g−1 |
23.1 ± 1.1 mg g−1 |
5.0 ± 0.3 mg g−1 |
22 |
Ultrasonic-assisted subcritical water extraction (UASWE) |
Deionized water with 2% ethanol and 2% acetone total gingerols and shogaol, ultrasonic frequency: 50/60Hz; ultrasonic power: 280 W; ultrasonic temperature: 40 °C; ultrasonic time: 30 min; ethanol: 2%; particle size: 1 mm; extraction temperature: 130 °C; pressure: 20 bars; extraction time: 30 min |
2990.5/5 μg bioactive per g dried ginger |
62
|
2.3. Advantages and disadvantages of extraction methods
The advantages and disadvantages of each approach are contingent on a number of variables, including cost, level of efficiency, and influence on the environment. Both Soxhlet extraction and maceration are generally easy and affordable processes, although they may be time-consuming and need a significant amount of solvent. Both microwave-assisted extraction and ultrasound-assisted extraction are quicker and more effective than traditional methods, but they also have the potential to degrade the chemicals. The extraction of supercritical fluids is both very effective and benign to the surrounding ecosystem; yet, it calls for specialized equipment and may be rather expensive. Ultrasound-assisted extraction (UAE) extracts gingerol quickly and efficiently with minimum solvent and energy use. High equipment cost and temperature may induce thermal deterioration. PEFE is fast, efficient, energy-efficient, and leaves no solvent residue. Scale-up is limited by high equipment cost, voltage, and cell membrane damage. Gingerol extraction is selective, gentle, and high-yielding using enzyme-assisted extraction (EAE). However, enzyme cost, extraction time, and high temperature or pH inactivation are drawbacks.
3. Health implications of gingerol
6-Gingerol has a wide range of health benefits due to its array of biological activities. Based on currently available published records, [6]-gingerol's biological activities are summarized below (Fig. 1).
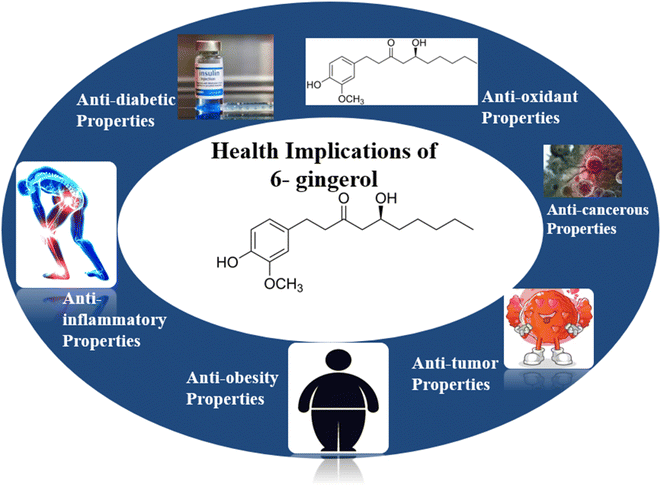 |
| Fig. 1 Health implications of gingerol. | |
3.1. Antioxidant properties
6-Gingerol, a natural antioxidant is recommended for the prevention of many diseases.65 The antioxidant properties of 6-gingerol might be related to its ability to act as an electron donor and to act as a free radical scavenger by the formation of a stable phenoxyl radical.15 Oxidative stress plays a major role in age-related and chronic diseases, such as cancer, arthritis, diabetic nephropathy, and osteoporosis.66 The transition state theory within the context of the density functional theory was used to examine the mechanisms of anti-oxidant activity of 6-gingerol as a hydroxyl radical scavenger.67 Research was conducted to examine the processes of hydrogen abstraction by a hydroxyl radical from various sites on 6-gingerol and addition of hydrogen from the former to various sites on the latter. In addition to this, the transport of electrons from 6-gingerol to a hydroxyl radical was investigated. 6-Gingerol exhibited considerable scavenging activities with half-maximal inhibitory concentration i.e. IC50 values of 4.0, 26.3, and 4.6 μM against superoxide, DPPH, and hydroxyl radicals, respectively, and prevented the production of ROS in human polymorphonuclear neutrophils at 6.0 μM.68 It also demonstrated protective effects against ischemia/reperfusion-induced intestinal mucosa injury by preventing the formation of ROS and p38 MAPK activation.69 In chlorpyrifos (CPF)-induced oxidative damage rats, 6-gingerol extract reportedly suppressed oxidative stress by lowering the levels of H2O2, GST, MPO, NO, TNF-α, MDA, and caspase-3 while increasing the levels of antioxidant enzymes like catalase, SOD, GPx, and GSH.70 Similar results have been obtained by Ajayi et al.71 and Ezzat et al.72 in rats with oxidative damage brought on by chlorpyrifos (CPF), where 6-gingerol extract reportedly reduced levels of H2O2, MDA, MPO, NO, TNF-α, and caspase-3 while increasing levels of antioxidant enzymes like catalase, SOD, GPx, GST, and GSH. 6-Gingerol significantly reduced the DNA strand breaks and micronucleus formation caused by patulin (PAT). Moreover, 6- gingerol effectively suppressed PAT-induced intracellular ROS formation and the 8-OHdG level. Fig. 2 shows the different biological activities and mechanisms of gingerol.
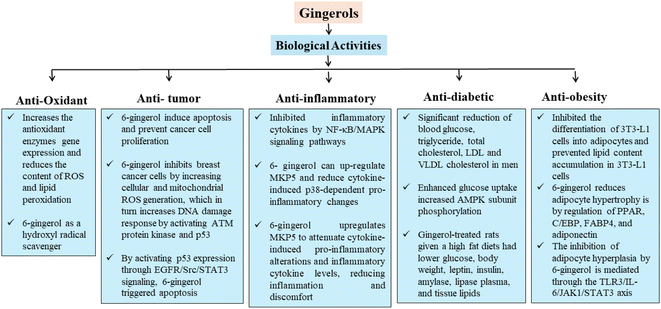 |
| Fig. 2 The different biological activities and effects of gingerol. | |
3.2. Anti-tumor properties
Ginger is being studied for its various health-beneficial properties and the anti-tumor property is one of them. It was recently reported that ginger extract inhibited cell proliferation and enhanced apoptosis in rats induced with diethylnitrosamine.73 Apart from inhibiting oxidative stress and inflammation, the extract down regulated NF-κB.16 In another study, extracts from ginger were found to exhibit cytotoxicity on HepG2 cells which show that ginger possesses anti-tumor and anti-cancer properties.58 As part of the control of the cell cycle, ginger components including 6-gingerol and 6-shogaol induce apoptosis and prevent cancer cell proliferation.9,74 According to a recent study, 6-gingerol reduced colorectal cancer through anti-proliferative, apoptotic, and anti-inflammatory pathways in mice. 6-Gingerol lowered tumor growth factor and -catenin expression, although it increased adenomatous polyposis coli (APC) expression. TNF-α, IL-1, INOS, COX-2, and cyclin D1 expression were then all reduced as a result. Inhibiting angiogenesis by lowering VEGF levels in the colon of mice was another effect of 6-gingerol.75 In another study, it was demonstrated that 6-gingerol inhibits breast cancer cells, specifically MDA-MB-231 and MCF-7, by increasing cellular and mitochondrial ROS generation, which in turn increases DNA damage response by activating ATM protein kinase and p53. By activating p53 expression through EGFR/Src/STAT3 signaling, 6-gingerol triggered apoptosis. Additionally, it caused the reduction of BAX/BCL-2 ratio and cytochrome-C release, which resulted in G0/G1 cell arrest and mitochondrial death.76 Ginger extract and its constituents, such as 6-gingerol and 6-shogaol, have been shown to possess anti-tumor and anti-cancer activities. These effects are achieved in several kinds of cancer cells by inducing apoptosis, blocking cancer cell growth, and inhibiting angiogenesis via a variety of distinct mechanisms.
3.3. Anti-inflammatory properties
The active compounds present in ginger are long known for their anti-inflammatory properties. Additionally, numerous recent randomized controlled trials demonstrated ginger's potential anti-inflammatory and pain-relieving effects.77,78 Many studies have recently been published elucidating ginger's unique biological properties and the process of preparing ginger extracts for increased anti-inflammatory activity. The two factors TNF-α (cytokine tumor necrosis factor) and (IL)-1β (interleukin) are the major cytokine factors to initiate the inflammatory effect in cells by reviving the expression of pro-inflammatory genes. Also, nitrogen-activated protein kinase phosphatase-5 (MKP5) plays a major role in mediating anti-inflammatory activities.79 6-Gingerol can up-regulate MKP5 and reduce cytokine-induced p38-dependent pro-inflammatory changes. Significant depletion of inflammatory cytokine levels, i.e., IL-6, IL-1β, TNF-α, INFr, MCP-1, normal T-cell expressed and secreted (RANTES), and myeloperoxidase activity, as well as an oxidative stress marker NO were observed.72,80 Gingerol dose-dependently decreased blood glucose, creatinine, BUN, pro-inflammatory cytokines, NF-κB activation, renal p38 MAPK, and TGF-β. These results suggest that gingerol improves renal tissue via altering p38 MAPK and NF-κB activity and controlling inflammation and oxidative stress.81 6-gingerol upregulates MKP5 to attenuate cytokine-induced pro-inflammatory alterations and inflammatory cytokine levels, reducing inflammation and discomfort. Gingerol reduced renal tissue inflammation and oxidative stress through changing p38MAPK and NF-κB activity.
3.4. Anti-diabetic properties
Son et al.20 suggested that 6- gingerol has anti-diabetic properties. 6-Gingerol restrains advanced glycation end product-induced increase in ROS levels in RIN-5F pancreatic β-cells. 6-Gingerol feeding restrains the rise in fasting blood glucose levels and enhances glucose intolerance in db/db mice. Gingerol was given orally to HFD-induced obese rats for 30 days in comparison to Lorcaserin (10 mg kg−1).82 HFD rats had higher body weight, glucose, lipid profile, insulin, insulin resistance, leptin, amylase, and lipase (P < 0.05). Gingerol-treated rats given an HFD had lower glucose, body weight, leptin, insulin, amylase, lipase plasma, and tissue lipids than controls (P < 0.05). Gingerol had the greatest impact at 75 mg kg−1. Gingerol has been shown to have anti-obesity benefits in rats, with the largest impact occurring at a dosage of 75 mg kg−1, while ginger extract and 6-gingerol have been shown to have anti-hyperglycaemic and anti-diabetic qualities.
3.5. Anti-obesity properties
Ginger contains several active physiological nutrients, phenolic compounds such as gingerols and shogaols and active components such as flavonoids and terpenoids. 6-Gingerol is found to be responsible for the distinctive taste of ginger and has been found to exhibit antiseptic, anti-inflammatory, and antioxidant activities. Saravanan et al.82 reported decreased glucose levels, insulin, body weight, amylase, lipase plasma, leptin, and tissue lipids with a dose of 75 mg kg−1 of gingerol compared to normal control. Seo et al.83 found that ginger augmentation significantly enhances HF-diet-induced BW gain, hypercholesterolemia, hyperglycemia, and hepatic steatosis without altering food uptake in mice. Table 2 summarizes the different health implications of gingerols. The researchers19 were able to obtain information about the potential mechanism by which 6-gingerol may be used to treat obese mice by using bioinformatics. The method by which 6-gingerol reduces adipocyte hypertrophy is by regulation of PPAR, C/EBP, FABP4, and adiponectin. The inhibition of adipocyte hyperplasia by 6-gingerol is mediated through the TLR3/IL-6/JAK1/STAT3 axis. Because it inhibits excessive hypertrophy and hyperplasia of adipocytes in the WAT, 6-gingerol may cure metabolic disorders. 6-Gingerol, which gives ginger its taste, has antiseptic, anti-inflammatory, and antioxidant properties and may help treat metabolic disorders by inhibiting adipocyte hypertrophy and hyperplasia through the TLR3/IL-6/JAK1/STAT3 axis.
Table 2 The health implication of gingerols
Health implications |
Findings |
Doses |
References |
Anti-diabetic properties |
Reduced fasting blood glucose, glycosylated hemoglobin, fasting insulin, and homeostasis model assessment-insulin resistance index |
200 mg kg−1 of 6-gingerol |
84 and 85 |
Significant reduction of blood glucose, triglyceride, total cholesterol, LDL and VLDL cholesterol in men |
|
86
|
Enhanced glucose uptake and increased AMPK subunit phosphorylation |
|
87
|
Anti-inflammatory properties |
Inhibited inflammatory cytokines by NF-κB/MAPK signaling pathways |
25 mg kg−1 |
81
|
Inhibiting the production of NO and PGE2 in RAW 264.7 mouse |
|
88
|
Anti-oxidant |
Increases antioxidant enzyme gene expression; reduces the amount of ROS and lipid peroxidation in C28I2 human chondrocyte cells |
100–600 μM |
89 and 90 |
Anti-obesity |
Inhibited the differentiation of 3T3-L1 cells into adipocytes and prevented lipid content accumulation in 3T3-L1 cells |
2–4 g per day |
91
|
4. Targeting and modulating signaling pathways in the GI tract
In order to overcome the problems related to delivery, potential targets, toxicity, and availability, innovative therapeutic methods to target the signaling pathways have been explored such as a self-micro emulsifying drug delivery system that improved the 6-gingerol concentration in the plasma and ultimately enhanced its solubility and bioavailability (about 6.58-fold) as compared with that of the free drug when supplied orally, as it reduces the partial dissolution and enables micro-emulsion formation in the intestine.92 To discover an active strategy or signaling for enhancing the solubility, bioavailability, and stability of gingerols, the process or mechanism of binding between bovine serum albumin (BSA) and gingerols was evaluated. The hydrogen bonds and hydrophobic interactions are primarily responsible for the bonding and can form a stable complex. Among the gingerols, 10-gingerol demonstrates the most pronounced affinity for binding with Bovine Serum Albumin (BSA), exhibiting a Ka value of 4.016 × 104 L mol−1.93,94 Gingerols by binding at the IIA subdomain or site I of BSA can shrink the BSA structure due to conformational changes and lead to decreased surface hydrophobicity. The formation of BSA and gingerol binding complexes increases the antioxidant ability and solubilities of gingerols multiple times, for example, the solubility of 6-gingerol, 8-gingerol, and 10-gingerol is enhanced by approximately 1.5, 6.0, and 23.5 times, respectively. A nanoparticle drug delivery approach of coupling gingerols and soya lecithin-based phytosome with chitosan amended the bioavailability of gingerols with improved and prolonged oral absorption.95–97 The antibacterial, antioxidant, and anti-inflammatory activities of gingerols improved through this method and can be used to treat respiratory disorders. The drug loading and efficacy of entrapment through this method were about 8% and 86%, respectively. In the market, few liposomal products of ginger formed by combination with many other bioactive compounds are available such as Micelle Liposomal Curcumin Gold and Synchro Gold Lemon Ginger which possess better bioavailability and oral absorption. The explicitly designed food matrices can augment the bioavailability of gingerols by controlling their release, solubility, absorption, transference, and metabolism in the GI tract. Gingerols inhibit the proliferation of cancer cells and encouraged apoptosis by stimulating 3-, 7-, 8-, and 9-caspases and cleaving poly-ADP ribose polymerase.
The main mechanism for the anti-cancerous activity of 6-gingerols is the inhibition of the pathway of the ERK1-JNK-AP-1 complex.98 It has been interpreted through functional protein–protein interaction analysis by targeting the stomach and small intestine that 6-gingerols regulate a fat-enriched diet metabolically by reducing biosynthesis and accumulation of fats, and augment the activity of transporters for metal ions present in the transmembrane.99 In this way, more consumed ATP may burn fatty acids to warm the internal parts of the stomach and/or small intestine. Additionally, it lessens the contraction of smooth muscles for improving the volume of blood flow and ultimately retains cells in a good state in the long run by regulating the cell cycle in the stomach and small intestine. It has been reported in the literature that dose-dependent treatment with 6-gingerol (approximately 800 mg kg−1) in NMRI mice suffering from non-alcoholic fatty liver disease improved various parameters including biochemical and histological, and altered the indices of fatty liver by efficiently dropping cholesterol, triglycerides, low-density lipoproteins, very low-density lipoproteins, glucose, and leptin and enhancing various antioxidant enzymes, therefore improving fatty liver and could be a good remedy.100 6-gingerol-based physiochemically stable pro-liposomes with narrow size dispersal prepared by the improved thin-film dispersion technique showed 5-times enhanced bioavailability in comparison to free 6-gingerol and a better antitumor effect when studied in vivo.101 Several studies have investigated the molecular mechanisms underlying the anti-inflammatory and anti-cancer effects of gingerol in the GI tract. One study found that gingerol could suppress the NF-κB signaling pathway, which is involved in inflammation and cancer development, in gastric cancer cells.102 Another study suggested that gingerol could inhibit the Wnt/β-catenin signaling pathway, which is involved in cell proliferation and tumorigenesis, in colon cancer cells.103
The effects of gingerol on oxidative stress and inflammation in the GI tract were studied by Farzaei et al.104 The study found that gingerol inhibited oxidative stress and inflammation in the GI tract by activating the Nrf2 signaling pathway, which leads to the upregulation of antioxidant enzymes such as superoxide dismutase (SOD), catalase (CAT), and glutathione peroxidase (GPx). Sampath et al.105 investigated the anti-obesity effects of gingerol in both cultured 3T3-L1 adipocytes and high-fat diet-induced obese mice. They found that gingerol increased AMP-activated protein kinase (AMPK) phosphorylation and subsequent activation, leading to increased fatty acid oxidation and decreased lipid accumulation. The study also showed that gingerol treatment decreased body weight gain, fat mass, and liver weight in obese mice. Hattori et al.106 investigated the effects of gingerol on obesity-induced inflammation and gut microbial composition in high-fat diet-fed mice. They found that gingerol treatment decreased adipose tissue inflammation and improved gut microbial diversity and composition. The study also showed that gingerol treatment improved glucose tolerance and insulin sensitivity in obese mice. The authors suggested that the anti-obesity effects of gingerol may be partly due to its modulation of the gut microbiota and associated signaling pathways. Dried ginger (DG) and Coptis chinensis (CC) have a potent anti-tumor effect on gastric cancer.107 The combination of CC and DG suppresses tumor growth by inhibiting tumor cell glucose metabolism and promoting apoptosis. The signaling pathway of CC-DG involves the downregulation of SLC2A1 and LDHA expression, resulting in the inhibition of glycolysis. The inhibition of glycolysis further affects cellular biological processes, inducing cell apoptosis in gastric cancer cells. The downregulation of SLC2A1 and LDHA results in the activation of related genes, including LDHD, ME2, PKM, PCX, and Got1. The study also revealed that the extraction mixture of CC-DG contains secondary metabolites, including magnoflorine, columbamine, epi berberine, jatrorrhizine, coptisine, palmatine, berberine, and 6-gingerol, which work together to cause the anti-gastric cancer effect. Ginseng polysaccharide injection can be used in combination with chemotherapy to treat advanced malignancies such as lung, stomach, and bowel cancer. It can reduce the toxic side effects of chemotherapy on patients and improve their quality of life, thereby enhancing their compliance with chemotherapy. Additionally, ginseng polysaccharide injection can improve the cellular immune function of patients and enhance the anti-cancer effect.108 The main mechanism of plant polysaccharides for achieving anticancer effects is inducing apoptosis of cancer cells, along with other mechanisms such as preventing cancer cell metastasis and enhancing immune activity. Therefore, ginseng may play a role in inducing apoptosis of cancer cells when used in combination with chemotherapy to treat various types of cancer. The signaling pathway for inducing apoptosis of cancer cells by plant polysaccharides involves a variety of mechanisms, including activation of caspases, release of cytochrome c, and regulation of the Bcl-2 family of proteins. Plant polysaccharides can also activate the PI3K/Akt signaling pathway, which is involved in regulating cell survival and proliferation, and can also induce apoptosis in cancer cells. Additionally, some studies have explored the role of gingerol in regulating gut microbiota and improving gut health. One study demonstrated that gingerol could modulate the gut microbiota composition and reduce gut inflammation in a mouse model of colitis.109 Another study reported that gingerol could enhance the production of short-chain fatty acids (SCFAs), which are important for gut health and immune function, in human fecal fermentation.110 Overall, these studies suggest that gingerol may have therapeutic potential for GI diseases by modulating various signaling pathways and promoting gut health. However, more research is needed to elucidate the molecular mechanisms underlying the effects of gingerol on the GI tract and to evaluate its safety and efficacy in humans. Gingerols first phosphorylates the p38 factor with the help of p50 and IkBa phosphorylates the nuclear target p65 at the serine residue at the 536 position. This p65 binds at the upstream site of many transcription factors and regulates the activation or repression of genes (Fig. 3). In intestinal ischemia reperfusion (I/R) damaged intestinal tissues, 6-gingerol dramatically reduced the production of proinflammatory cytokines like TNF-α, IL-1, and IL-6 and prevented the expression of inflammatory mediators iNOS/NO. In I/R wounded rats and in Caco-2 and IEC-6 cells that are characterized by suppressing p38 MAPK phosphorylation, nuclear translocation of NF-B, and expression of myosin light chain kinase (MLCK) protein, the damaged intestinal barrier function was restored by 6-gingerol. In Caco-2 and IEC-6 cells, 6-gingerol also decreased the production of reactive oxygen species (ROS). The impacts of 6 G on NF-B and MLCK expression were lessened by the in vitro transfection of p38 MAPK siRNA, and the outcomes further supported the protective effects of 6 G on intestinal I/R injury by inhibiting p38 MAPK signaling9,69 (Fig. 3).
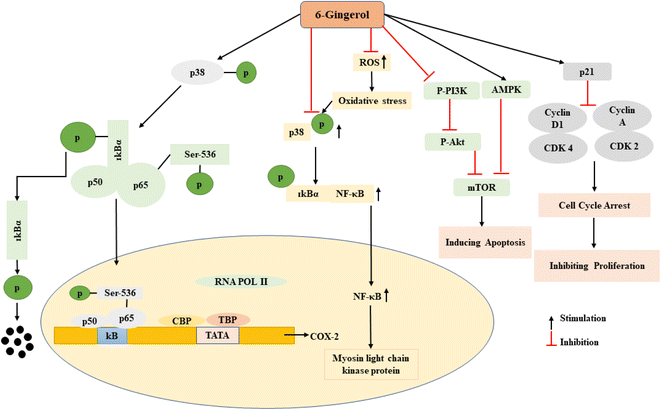 |
| Fig. 3 Signaling pathway of gingerols. Gingerols activate the nuclear cascades by the phosphorylation of nuclear target factors p38 and p65 at the serine amino acid. It regulates many downstream transcription factors by binding upstream of the gene site. Gingerol suppresses p38 MAPK phosphorylation, nuclear translocation of NF-B, and expression of myosin light chain kinase (MLCK) protein. Gingerols are also involved in the anticancer mechanism by controlling several signaling pathways and inducing apoptosis in cells (see abbreviations in the Abbreviations section). | |
5. Bioavailability of gingerols in the GI tract
Gingerols possess various bioactivities such as anti-cancerous, anti-inflammatory, analgesic, antiulcer, antithrombotic, and antioxidant activities.111 The chemo-preventive potential of gingerols presents a promising future alternative to expensive and toxic therapeutic agents. Due to this potential of gingerols, ginger is widely used as an herbal medicine for several disorders related to digestion such as gastritis, nausea, diarrhea, and dyspepsia. The occurrence of ginger in dietary food is believed to be beneficial in reducing the chances of gastrointestinal, breast, colon, and other cancers. Gingerols act strongly on the GI mucous membrane and control the function of the intestine to simplify absorption.102
After the oral consumption of ginger, the quantity of its active compounds including gingerols in the blood is comparatively less because of its loss through gastroenteric absorption. Like other phenolics, gingerols conjugate through glucuronide or sulphate bonding in the epithelium of the intestine, partially in the liver or any another tissue.63 Gingerols are characteristically found in conjugated structures. Likewise, the oral consumption of 6-gingerol in rats resulted in its immediate conjugation in the epithelium of the intestine and liver to form S-6-gingerol-4′-O-β-glucuronide, which is then excreted through bile. Pharmacokinetic analysis showed that 6-gingerol, 8-gingerol, 10-gingerol, and 6-shogaol have half-lives of 1 to 3 h in the plasma of humans. In humans, after 1 h of oral administration of 2 g ginger extracts, free 10-gingerol, and 6-shogaol were distinguished in the plasma at 9.5 ± 2.2 and 13.6 ± 6.9 ng per mL concentrations, respectively. Moreover, dissimilar kinetics of absorption has been studied for various gingerols of the ginger extract. The peak of 6-gingerol, 10-gingerol, 8-gingerol and 6-shogaol is reported at 27.18 μg L−1, 55.95 μg L−1, and 4.04 ± 5.37 μg L−1 although with high errors especially for 6-gingerol in the plasma of rats after 2 h, 30 min and 10 min of consumption, respectively. Furthermore, 6-gingerol has weak oral absorption due to poor solubility. Because of this, soft capsules of gingerols are most effective and valuable.112 When 6-gingerol is supplied orally, the maximum concentration can be found in the gastrointestinal tract. It has been studied that 6-gingerol was absorbed speedily in the plasma after dosing of 250 mg per kg ginger extract (having approximately 52% 6-gingerol) in experimental rats and post 10 min of dose, the concentration reached a maximum i.e., 4.23 μg mL−1, reduced biexponentially by time, and has wide-ranging dispersal and high partitioning in tissues. According to the findings of studies conducted in vivo, gingerols have a very low bioavailability.113 According to Hitomi et al.,114 just 1.3% of gingerols that were orally administered to rats was absorbed and made it into circulation. After consumption of a standardised ginger extract, just 5% of gingerols was found to be bioavailable, according to the studies of Santos,115 that were conducted on healthy human volunteers. In vitro research has also revealed that the bioavailability of gingerols is affected by factors such as pH, solubility, and intestinal permeability.116 These factors all have a role in the absorption of gingerols. Gingerol absorption was shown to increase when pH declined in a study that used human intestinal cell line Caco-2 cells. This finding suggests that gingerols are more readily absorbed in the acidic environment of the stomach. Another piece of research suggests that gingerol bioavailability might be increased by the use of lipid-based delivery systems, which would also improve the solubility of gingerols in water. Gingerols, in and of themselves, have a low bioavailability; however, their bioavailability may be enhanced by mixing them with other drugs or using delivery techniques that boost their solubility and absorption. In vivo and in vitro studies gave valuable insights into the factors that influence the bioavailability of gingerols.117 These insights might be helpful in the development of effective delivery strategies for gingerols and other substances.
The reported half-time was 1.77 h and the seeming clearance was 40.8 L h−1. In another pharmacokinetics study, oral administration of 5% gingerols was rapidly absorbed with a maximum time of 55–66 min, they possess half-lives of elimination of 75–120 min, and no free forms were detected in the plasma of experimental mice. It has been shown that gingerols are metabolized extensively following conjugation with the help of gastrointestinal glucuronosyltransferase specifically the uridine diphosphate enzyme family, and about half the amount of consumed 6-gingerol is excreted in the form of glucuronides in bile and approximately 3% is excreted in the free form in urine.115 Some other metabolites detected in urine were octanoic acid, ferulic acid, 4-(4-OH-3-methoxyphenyl) butanoic acid, vanillic acid, and 9-OH-6-gingerol. However, a complete detailed analysis of the metabolism and excretion of gingerols is been available in the literature yet. An intestinal UDP-glucuronosyltransferase enzyme named UGT1A8 and two other UGT1A9 and UGT2B7 showed the greatest activity for gingerols. UGT1A9 is primarily involved in the catalysis of formed 5-O-glucuronide whereas UGT2B7 catalyses glucuronides at both sites 4 or 5-OH but more preferably at position 4. The glucuronosyltransferases which are mainly involved in the catalysis or mechanism of gingerols in the liver are UGT2B7 and UGT1A9 for the 4th and 5th hydroxyl sites, respectively. It has been reported through kinetic study analysis of enzymes that shagols possess higher metabolic activity in the liver than the intestine which is CLint −1.37–2.87 and 0.67–0.85 mL min−1 mg−1, respectively and UGT2B7 showed the greatest ability of catalysis which is CLint – 0.47–1.17 mL min−1 mg−1.118 Moreover, about 41%, 34%, and 36% glucuronidation of 6-shagols, 8-shagols and 10-shagols occurred in human liver microsomes, respectively. In summary, gingerols including shagols were proficiently metabolized by pathway of glucuronidation, and UGT2B7 is a vital component for glucuronidation. The diagrammatic representation of the bioavailability route of gingerols after oral consumption is illustrated in Fig. 4.
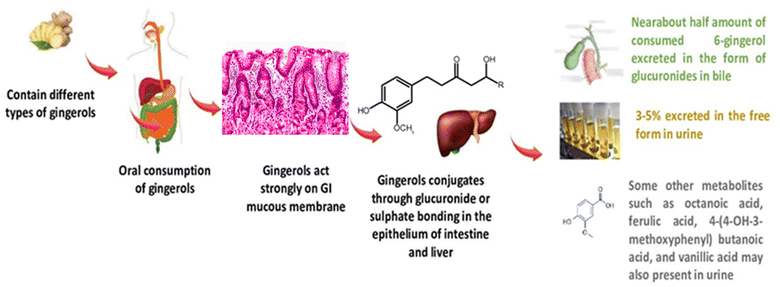 |
| Fig. 4 Diagrammatic representation of the bioavailability route of gingerols after oral consumption. | |
6. Conclusion
In conclusion, ginger contains bioactive compounds, particularly gingerols, which have anti-inflammatory, anti-cancerous, analgesic, antioxidant, antiulcer, and antithrombotic properties. Including ginger in the diet may reduce the risk of gastrointestinal, breast, colon, and other cancers. Gingerol extraction can be performed through conventional methods, which are simpler but less efficient, or advanced methods like Microwave-Assisted Extraction (MAE), which offer higher yields and are more eco-friendly. After oral consumption, gingerols are metabolized and conjugated in the intestine and liver, with half-lives of 1 to 3 hours in human plasma. Soft capsules are most effective due to the poor solubility of 6-gingerol. Approximately half of the consumed 6-gingerol is excreted as glucuronides in bile, and about 3% is excreted in urine. However, a detailed analysis of gingerol metabolism and excretion is still needed.
7. Future perspectives
Future research perspectives in the study of ginger could focus on exploring the optimal extraction method for gingerols and their conjugated forms, as well as the comprehensive analysis of their metabolism and excretion pathways in humans. Additionally, further investigation is needed to determine the potential health benefits of ginger extract in the prevention and treatment of specific types of cancer, and to develop new formulations and delivery methods to enhance their therapeutic effects. The research needs to be focused on the targeted and modulated signalling pathways of gingerol in the gastrointestinal tract. This research can lead to a better understanding of the molecular mechanisms of gingerol's anti-inflammatory, anti-cancerous, and antiulcer properties. By identifying the specific signaling pathways and targets of gingerol, novel therapeutic strategies and drugs can be developed that can help in the prevention and treatment of various GI disorders. Additionally, more research is needed to determine the optimal dosage and duration of gingerol treatment in humans for maximal therapeutic benefit. When analysing the favourable health benefits of ginger against chronic illnesses, several confounding variables must be taken into account. There is little doubt that the kind of ginger affects upcoming clinical studies. In a small number of in vitro and animal investigations, the bioactivities of gingerols and shogaols in certain illness models were directly compared. More disease models should be used to examine the effectiveness of gingerols and shogaols in order to create customised ginger formulations for various disorders. The study of the additive and synergistic interactions between gingerols and shogaols as well as between gingerols, shogaols, and other minor components in ginger will be necessary to determine the ideal oral ginger composition. This study will also aid researchers in developing a trustworthy standardisation method to monitor the composition and quality of a ginger formulation for a future trial. The optimal dosage range and dose frequency should be determined after an optimised ginger formulation for a specific condition has been created. Both shogaols and gingerols undergo significant in vivo metabolization. The research of the bioactivities of ginger metabolites, which is still in its infancy since the majority of the metabolites are not readily accessible commercially, is of utmost importance. Future research should take into account the interindividual variations in ginger chemical metabolism and absorption caused by genetic variants and/or changes in gut flora. It is crucial to determine the metabotype of shogaols and gingerols based on an individual's genome and/or gut microbiome, as well as to further investigate the pharmacokinetics of ginger compounds and their metabolites in various metabotypes. Doing so will aid in selecting the appropriate study populations for upcoming clinical trials.
Data availability
The data that support the findings of this study are available from the corresponding author upon reasonable request.
Author contributions
MKS contributed to the organization, writing, and composition of the manuscript. MR, MK and DG wrote the sections of the manuscript. All authors contributed to the article and approved the submitted version.
Conflicts of interest
The authors declare that the research was conducted in the absence of any commercial or financial relationships that could be construed as a potential conflict of interest.
Abbreviations
Akt | Protein kinase B |
AMPK | 5′adenosine monophosphate-activated protein kinase |
APC | Adenomatous polyposis coli |
Bax | Bcl-2-associated X protein |
Bcl-2 | B-cell lymphoma 2 |
CDK | Cyclin-dependent kinase |
CLint: | Intrinsic clearance |
(IL)-1β | Interleukin |
°C | Degree celsius |
μM | Micrometer |
BSA | Bovine serum albumin |
EAE | Enzyme-assisted extraction and high-voltage electrical discharge (HVED) |
EATPP | Enzyme assisted three phase partitioning |
gm | Gram |
Hr | Hour |
IC50 | Inhibitory concentration |
ILUAE | Ionic liquid-based ultrasonic-assisted extraction |
K | Kelvin |
kHz | Kilo hertz |
LPS | Lipopolysaccharide |
MAE | Microwave-assisted extraction |
MAPK | Mitogen-activated protein kinase |
mg g−1 | Milligram per gram |
min | Minute |
mL | Mililiter |
MPa | MegaPascal |
mTOR | Mammalian target of rapamycin |
NFκB | Nuclear factor kappa B |
PEFE | Pulsed electric field extraction |
PGE2: | Prostaglandin E2 |
PI3K | Phosphoinositide 3-kinase |
PLE | Pressure liquid extraction |
RIN-5F |
Rattus norvegicus
|
ROS | Reactive oxygen species |
rpm | Revolutions per minute |
SCFE-CO2 | Supercritical fluid carbon dioxide extraction |
SFE | Supercritical fluid extraction |
SWE | Subcritical water extraction |
temp. | Temperature |
TNF-α | Tumor necrosis factor-alpha |
TTP | Three phase partitioning |
UAE | Ultrasound-assisted extraction |
UASWE | Ultrasonic-assisted subcritical water extraction |
UATPP | Ultrasound assisted three phase partitioning |
UGT1A8 | UDP-glucuronosyltransferase enzyme |
w/v | Weight to volume ratio |
W | Watt |
Acknowledgements
Sincere thanks to Director, ICAR-Central Institute of Post-harvest Engineering and technology for providing facilities.
References
-
A. Nag, Phytochemistry and Antigenotoxic Properties of Six Ethnobotanically Important Members From the Family Zingiberaceae, In Research Anthology on Recent Advancements in Ethnopharmacology and Nutraceuticals, IGI Global, 2022, pp. 378–400 Search PubMed.
- N. H. Anh, S. J. Kim, N. P. Long, J. E. Min, Y. C. Yoon, E. G. Lee, M. Kim, T. J. Kim, Y. Y. Yang, E. Y. Son and S. J. Yoon, Ginger on human health: a comprehensive systematic review of 109 randomized controlled trials, Nutrients, 2020, 12(1), 157 CrossRef CAS PubMed.
- F. Zhang, K. Thakur, F. Hu, J. G. Zhang and Z. J. Wei, Cross-talk between 10-gingerol and its anti-cancerous potential: a recent update, Food Funct., 2017, 8(8), 2635–2649 RSC.
- T. Kausar, S. Anwar, E. Hanan, M. Yaseen, S. M. Aboelnaga and Z. R. Azad, Therapeutic role of ginger (Zingiber officinale)-A review, J. Pharm. Res. Int., 2021, 33(29B), 9–16 CrossRef.
- M. K. Samota, M. Sharma, K. Kaur, Y. D. K. Sarita, A. K. Pandey, Y. Tak, M. Rawat, J. Thakur and H. Rani, Onion anthocyanins: Extraction, stability, bioavailability, dietary effect, and health implications, Front. Nutr., 2022, 9, 917617 CrossRef PubMed.
- Y. Tak, M. Kaur, M. C. Jain, M. K. Samota, N. K. Meena, G. Kaur, R. Kumar, D. Sharma, J. M. Lorenzo and R. Amarowicz, Jamun seed: A review on bioactive constituents, nutritional value and health benefits, Pol. J. Food Nutr. Sci., 2022, 72(3), 211–228 CrossRef CAS.
- R. Verma and P. S. Bisen, Ginger-A Potential Source of Therapeutic and Pharmaceutical Compounds, J. Food Bioact., 2022, 18(18), 67–76 Search PubMed.
- S. H. Ahmed, T. Gonda and A. Hunyadi, Medicinal chemistry inspired by ginger: Exploring the chemical space around 6-gingerol, RSC Adv., 2021, 11(43), 26687–26699 RSC.
- Q. Q. Mao, X. Y. Xu, S. Y. Cao, R. Y. Gan, H. Corke, T. Beta and H. B. Li, Bioactive compounds and bioactivities of ginger (Zingiber officinale Roscoe), Foods, 2019, 8(6), 185 CrossRef CAS PubMed.
- S. Armenta, S. Garrigues, F. A. Esteve-Turrillas and M. de la Guardia, Green extraction techniques in green analytical chemistry, Trac. Trends Anal. Chem., 2019, 116, 248–253 CrossRef CAS.
- F. Chemat, M. Abert-Vian, A. S. Fabiano-Tixier, J. Strube, L. Uhlenbrock, V. Gunjevic and G. Cravotto, Green extraction of natural products. Origins, current status, and future challenges, Trac. Trends Anal. Chem., 2019, 118, 248–263 CrossRef CAS.
- R. R. Dalsasso, G. A. Valencia and A. R. Monteiro, Impact of drying and extractions processes on the recovery of gingerols and shogaols, the main bioactive compounds of ginger, Food Res. Int., 2022, 154, 111043 CrossRef CAS PubMed.
- M. S. Kamaruddin, G. H. Chong, N. M. Daud, N. R. Putra, L. M. Salleh and N. Suleiman, Bioactivities and green advanced extraction technologies of ginger oleoresin extracts: A review, Food Res. Int., 2023, 164, 112283 CrossRef CAS PubMed.
- O. A. Durojaye, B. C. Nwanguma, P. E. Joshua, J. O. Ogidigo, S. Cosmas, R. O. Asomadu and J. N. Obeta, Evaluation of 6-Gingerol and its modified analogues as therapeutic candidates against Schistosoma mansoni phosphofructokinase, VacciMonitor, 2019, 28(1), 38–47 CAS.
- M. M. Rahaman, R. Hossain, J. Herrera-Bravo, M. T. Islam, O. Atolani, O. S. Adeyemi, O. A. Owolodun, L. Kambizi, S. D. Daştan, D. Calina and J. Sharifi-Rad, Natural antioxidants from some fruits, seeds, foods, natural products, and associated health benefits: An update, Food Sci. Nutr., 2023, 11(4), 1657–1670 CrossRef PubMed.
- A. A. Hamza, G. H. Heeba, S. Hamza, A. Abdalla and A. Amin, Standardized extract of ginger ameliorates liver cancer by reducing proliferation and inducing apoptosis through inhibition oxidative stress/inflammation pathway, Biomed. Pharmacother., 2021, 134, 111102 CrossRef CAS PubMed.
-
P. Nath, N. Pandey, M. Samota, K. Sharma, S. Kale, P. Kannaujia, S. Sethi and O. P. Chauhan, Browning reactions in foods, in Advances in Food Chemistry: Food Components, Processing and Preservation, Springer Nature Singapore, Singapore, 2022, pp. 117–159 Search PubMed.
- S. Singh, A. Sharma, R. Reddy and M. K. Samota, Eco-friendly processing of momordica Charantia L. based chemical free functionally enriched nectar and evaluation of its nutritional profile, Bangladesh J. Bot., 2022, 51(3), 445–453 CrossRef.
- Z. Cheng, X. Xiong, Y. Zhou, F. Wu, Q. Shao, R. Dong, Q. Liu, L. Li and G. Chen, 6-gingerol ameliorates metabolic disorders by inhibiting hypertrophy and hyperplasia of adipocytes in high-fat-diet induced obese mice, Biomed. Pharmacother., 2022, 146, 112491 CrossRef CAS PubMed.
- M. J. Son, Y. Miura and K. Yagasaki, Mechanisms for antidiabetic effect of gingerol in cultured cells and obese diabetic model mice, Cytotechnology, 2015, 67, 641–652 CrossRef CAS PubMed.
-
K. Pundarikakshudu, Antiinflammatory and antiarthritic activities of some foods and spices, Bioactive food as dietary interventions for arthritis and related inflammatory diseases, 2019, vol. 1, pp. 51–68 Search PubMed.
-
E. Köngül Şafak and G. Şeker Karatoprak, Ginger (Gingerols and 6-Shogaol) Against Cancer, Nutraceuticals and Cancer Signaling: Clinical Aspects and Mode of Action, 2021, pp. 291–321 Search PubMed.
- C. Suri, B. Pande, T. Sahu, L. S. Sahithi and H. K. Verma, Revolutionizing Gastrointestinal Disorder Management: Cutting-Edge Advances and Future Prospects, J. Clin. Med., 2024, 13(13), 3977 CrossRef CAS PubMed.
- S. R. Jardim, L. M. P. de Souza and H. S. P. de Souza, The rise of gastrointestinal cancers as a global phenomenon: unhealthy behavior or progress?, Int. J. Environ. Res. Publ. Health, 2023, 20(4), 3640 CrossRef CAS PubMed.
- D. D. Gaikwad, S. K. Shinde, A. V. Kawade, S. J. Jadhav and M. V. Gadhave, Isolation and standardization of gingerol from ginger rhizome by using TLC, HPLC, and identification tests, Pharma Innov., 2017, 6(2, Part C), 179 CAS.
- A. Shukla, S. N. Naik, V. V. Goud and C. Das, Supercritical CO2 extraction and online fractionation of dry ginger for production of high-quality volatile oil and gingerols enriched oleoresin, Ind. Crops Prod., 2019, 130, 352–362 CrossRef CAS.
- S. Tanweer, T. Mehmood, S. Zainab, Z. Ahmad and A. Shehzad, Comparison and HPLC quantification of antioxidant profiling of ginger rhizome, leaves and flower extracts, Clin. Phytosci., 2020, 6, 1–2 CrossRef.
- S. Varakumar, K. V. Umesh and R. S. Singhal, Enhanced extraction of oleoresin from ginger (Zingiber officinale) rhizome powder using enzyme-assisted three phase partitioning, Food Chem., 2017, 216, 27–36 CrossRef CAS PubMed.
- M. J. Ko, H. H. Nam and M. S. Chung, Conversion of 6-gingerol to 6-shogaol in ginger (Zingiber officinale) pulp and peel during subcritical water extraction, Food Chem., 2019, 270, 149–155 CrossRef CAS PubMed.
- F. Chemat, N. Rombaut, A. G. Sicaire, A. Meullemiestre, A. S. Fabiano-Tixier and M. Abert-Vian, Ultrasound assisted extraction of food and natural products. Mechanisms, techniques, combinations, protocols and applications. A review, Ultrason. Sonochem., 2017, 34, 540–560 CrossRef CAS PubMed.
- A. Tzani, S. Kalafateli, G. Tatsis, M. Bairaktari, I. Kostopoulou, A. R. Pontillo and A. Detsi, Natural deep eutectic solvents (NaDESs) as alternative green extraction media for ginger (Zingiber officinale Roscoe), Sustainable Chem., 2021, 2(4), 576–598 CrossRef CAS.
- N. Utama-Ang, S. Sida, P. Wanachantararak and A. Kawee-Ai, Development of edible Thai rice film fortified with ginger extract using microwave-assisted extraction for oral antimicrobial properties, Sci. Rep., 2021, 11(1), 14870 CrossRef CAS PubMed.
- H. Teng, K. T. Seuseu, W. Y. Lee and L. Chen, Comparing the effects of microwave radiation on 6-gingerol and 6-shogaol from ginger rhizomes (Zingiber officinale Rosc), PLoS One, 2019, 14(6), e0214893 CrossRef CAS PubMed.
- S. A. Baghban, M. Ebrahimi, S. Bagheri-Khoulenjani and M. Khorasani, A highly efficient microwave-assisted synthesis of an LED-curable methacrylated gelatin for bio applications, RSC Adv., 2021, 11(25), 14996–15009 RSC.
- J. B. Guo, Y. Fan, W. J. Zhang, H. Wu, L. M. Du and Y. X. Chang, Extraction of gingerols and shogaols from ginger (Zingiber officinale Roscoe) through microwave technique using ionic liquids, J. Food Compos. Anal., 2017, 62, 35–42 CrossRef CAS.
- C. Garza-Cadena, D. M. Ortega-Rivera, G. Machorro-García, E. M. Gonzalez-Zermeño, D. Homma-Dueñas, M. Plata-Gryl and R. Castro-Muñoz, A comprehensive review on Ginger (Zingiber officinale) as a potential source of nutraceuticals for food formulations: Towards the polishing of gingerol and other present biomolecules, Food Chem., 2023, 413, 135629 CrossRef CAS PubMed.
- A. L. Dias, A. C. de Aguiar and M. A. Rostagno, Extraction of natural products using supercritical fluids and pressurized liquids assisted by ultrasound: Current status and trends, Ultrason. Sonochem., 2021, 74, 105584 CrossRef CAS PubMed.
-
A. P. Sánchez-Camargo, L. Montero, J. A. Mendiola, M. Herrero and E. Ibáñez, Novel extraction techniques for bioactive compounds from herbs and spices, Herbs, Spices and Medicinal Plants: Processing, Health Benefits and Safety, 2020, vol. 12, pp. 95–128 Search PubMed.
- E. Reverchon and I. De Marco, Supercritical fluid extraction and fractionation of natural matter, J. Supercrit. Fluids, 2006, 38(2), 146–166 CrossRef CAS.
- G. Sodeifian, S. A. Sajadian and N. S. Ardestani, Extraction of Dracocephalum kotschyi Boiss using supercritical carbon dioxide: Experimental and optimization, J. Supercrit. Fluids, 2016, 107, 137–144 CrossRef CAS.
- J. T. Paula, A. C. Aguiar, I. M. Sousa, P. M. Magalhaes, M. A. Foglio and F. A. Cabral, Scale-up study of supercritical fluid extraction process for Baccharis dracunculifolia, J. Supercrit. Fluids, 2016, 107, 219–225 CrossRef CAS.
- S. Balachandran, S. E. Kentish and R. Mawson, The effects of both preparation method and season on the supercritical extraction of ginger, Sep. Purif. Technol., 2006, 48, 94–105 CrossRef CAS.
-
M. A. Fitriady, A. Sulaswatty, E. Agustian, S. Salahuddin and D. P. Aditama. Supercritical fluid extraction of ginger (Zingiber Officinale Var. Amarum): Global yield and composition study, In AIP Conference Proceedings, AIP Publishing, 2017, Nov 27, vol. 1904, No. 1 Search PubMed.
- J. E. T. Souza, L. M. Siqueira, R. N. Almeida, A. M. Lucas, C. G. Silva, E. Cassel and R. M. Vargas, Comparison of different extraction techniques of Zingiber officinale essential oil, Braz. Arch. Biol. Technol., 2020, 63, e20190213 CrossRef.
- A. Dukare, B. Bibwe, M. K. Samota, S. Dawange, M. Kumar and J. M. Lorenzo, Assessment of bioactive compounds, physicochemical properties, and microbial attributes of hot air–dried mango seed kernel powder: an approach for quality and safety evaluation of hot air–dried mango seed kernel powder, Food Anal. Methods, 2022, 15(10), 2675–2690 CrossRef.
- A. Dukare, M. K. Samota, B. Bibwe and S. Dawange, Using convective hot air drying to stabilize mango peel (Cv-Chausa): evaluating effect on bioactive compounds, physicochemical attributes, mineral profile, recovery of fermentable sugar, and microbial safety, J. Food Meas. Char., 2022, 16(5), 3897–3909 CrossRef.
- A. Murphy, E. Norton, F. Montgomery, A. Jaiswal and S. Jaiswal, Ultrasound-assisted extraction of polyphenols
from ginger (Zingiber officinale) and evaluation of its antioxidant and antimicrobial properties, J. Food Chem. Nanotechnol., 2020, 6(2), 88–96 Search PubMed.
- E. Bozinou, I. Karageorgou, G. Batra, V. Dourtoglou and S. Lalas, Pulsed electric field extraction and antioxidant activity determination of Moringa oleifera dry leaves: A comparative study with other extraction techniques, Beverages, 2019, 5(1), 8 CrossRef CAS.
- M. A. Maza, C. Pereira, J. M. Martínez, A. Camargo, I. Álvarez and J. Raso, PEF treatments of high specific energy permit the reduction of maceration time during vinification of Caladoc and Grenache grapes, Innovat. Food Sci. Emerg. Technol., 2020, 63, 102375 CrossRef CAS.
- D. Gabrić, F. Barba, S. Roohinejad, S. M. Gharibzahedi, M. Radojčin, P. Putnik and D. Bursać Kovačević, Pulsed electric fields as an alternative to thermal processing for preservation of nutritive and physicochemical properties of beverages: A review, J. Food Process. Eng., 2018, 41(1), e12638 CrossRef.
- B. Martín-García, U. Tylewicz, V. Verardo, F. Pasini, A. M. Gómez-Caravaca, M. F. Caboni and M. Dalla Rosa, Pulsed electric field (PEF) as pre-treatment to improve the phenolic compounds recovery from brewers' spent grains, Innovat. Food Sci. Emerg. Technol., 2020, 64, 102402 CrossRef.
- F. J. Barba, O. Parniakov, S. A. Pereira, A. Wiktor, N. Grimi, N. Boussetta, J. A. Saraiva, J. Raso, O. Martin-Belloso, D. Witrowa-Rajchert and N. Lebovka, Current applications and new opportunities for the use of pulsed electric fields in food science and industry, Food Res. Int., 2015, 77, 773–798 CrossRef.
-
M. Kandušer and D. Miklavčič, Electrotechnologies for Extraction from Food Plants and Biomaterial: An overview, In Electrotechnologies for Extraction from Food Plants and Biomaterials, ed. E. Vorobiev and N. Lebovka, Springer, New York, NY, USA, 1st edn, 2019, pp. 1–37 Search PubMed.
-
T. R. Shamala, H. Manonmani, G. Venkateswaran, H. B. Sowbhagya, S. R. Sampathu and R. Joseph, An enzymatic process for the preparation of spice oil, Sri Lankan Patent No. 14126, April 2009.
- D. Manasa, P. Srinivas and H. B. Sowbhagya, Enzyme-assisted extraction of bioactive compounds from ginger (Zingiber officinale Roscoe), Food Chem., 2013, 139(1–4), 509–514 Search PubMed.
- W. Liu, C. L. Zhou, J. Zhao, D. Chen and Q. H. Li, Optimized microwave-assisted extraction of 6-gingerol from Zingiber officinale roscoeand evaluation of antioxidant activity in vitro, Acta Sci. Pol., Technol. Aliment., 2014, 13(2), 155–168 CrossRef CAS PubMed.
- M. F. Sharif and M. T. Bennett, The effect of different methods and solvents on the extraction of polyphenols in ginger Zingiber officinale, J. Teknol., 2016, 78(11–2), 49–54 Search PubMed.
- S. T. Nguyen, P. H. Vo, T. D. Nguyen, N. M. Do, B. H. Le, D. T. Dinh, K. D. Truong and P. Van Pham, Ethanol extract of Ginger Zingiber officinale Roscoe by Soxhlet method induces apoptosis in human hepatocellular carcinoma cell line, Biomed Res Ther., 2019, 6(11), 3433–3442 CrossRef.
- K. L. Nagendra chari, D. Manasa, P. Srinivas and H. B. Sowbhagya, Enzyme-assisted extraction of bioactive compounds from ginger (Zingiber officinale Roscoe), Food Chem., 2013, 139(1–4), 509–514 CrossRef CAS PubMed.
- M. A. Awang, N. A. Azelan, N. A. A. W. Mustaffa, A. Aziz and R. Hasham, Influence of processing parameters on the yield and 6-gingerol content of Zingiber officinale extract, J. Chem. Pharmaceut. Res., 2014, 6(11), 358–363 Search PubMed.
- L. Q. Peng, J. Cao, L. J. Du, Q. D. Zhang, J. J. Xu, Y. B. Chen, Y. T. Shi and R. R. Li, Rapid ultrasonic and microwave-assisted micellar extraction of zingiberone, shogaol and gingerols from gingers using biosurfactants, J. Chromatogr. A, 2017, 1515, 37–44 CrossRef CAS PubMed.
- Z. Nourbakhsh Amiri, G. D. Najafpour, M. Mohammadi and A. A. Moghadamnia, Subcritical water extraction of bioactive compounds from ginger (Zingiber officinale Roscoe), Int. J. Eng., 2018, 31(12), 1991–2000 Search PubMed.
- X. Kou, Y. Ke, X. Wang, M. R. Rahman, Y. Xie, S. Chen and H. Wang, Simultaneous extraction of hydrophobic and hydrophilic bioactive compounds from ginger (Zingiber officinale Roscoe), Food Chem., 2018, 257, 223–229 CrossRef CAS PubMed.
- J. Cha, C. T. Kim and Y. J. Cho, Optimizing extraction conditions for functional compounds from ginger (Zingiber officinale Roscoe) using response surface methodology, Food Sci. Biotechnol., 2020, 29, 379–385 CrossRef CAS PubMed.
- K. S. Alharbi, M. S. Nadeem, O. Afzal, S. I. Alzarea, A. S. Altamimi, W. H. Almalki, B. Mubeen, S. Iftikhar, L. Shah and I. Kazmi, Gingerol, a natural antioxidant, attenuates hyperglycemia and downstream complications, Metabolites, 2022, 12(12), 1274 CrossRef CAS PubMed.
- N. Promdam and P. Panichayupakaranant, [6]-Gingerol: a narrative review of its beneficial effect on human health, Food Chem. Adv., 2022, 1, 100043 CrossRef.
- M. K. Tiwari and P. C. Mishra, Anti-oxidant activity of 6-gingerol as a hydroxyl radical scavenger by hydrogen atom transfer, radical addition and electron transfer mechanisms, J. Chem. Sci., 2016, 128, 1199–1210 CrossRef CAS.
- S. Dugasani, M. R. Pichika, V. D. Nadarajah, M. K. Balijepalli, S. Tandra and J. N. Korlakunta, Comparative antioxidant and anti-inflammatory effects of [6]-gingerol,[8]-gingerol,[10]-gingerol and [6]-shogaol, J. Ethnopharmacol., 2010, 127(2), 515–520 CrossRef CAS PubMed.
- Y. Li, B. Xu, M. Xu, D. Chen, Y. Xiong, M. Lian, Y. Sun, Z. Tang, L. Wang, C. Jiang and Y. Lin, 6-Gingerol protects intestinal barrier from ischemia/reperfusion-induced damage via inhibition of p38 MAPK to NF-κB signalling, Pharmacol. Res., 2017, 119, 137–148 CrossRef CAS PubMed.
- A. O. Abolaji, M. Ojo, T. T. Afolabi, M. D. Arowoogun, D. Nwawolor and E. O. Farombi, Protective properties of 6-gingerol-rich fraction from Zingiber officinale (Ginger) on chlorpyrifos-induced oxidative damage and inflammation in the brain, ovary and uterus of rats, Chem. Biol. Interact., 2017, 270, 15–23 CrossRef CAS PubMed.
- B. O. Ajayi, I. A. Adedara and E. O. Farombi, 6-Gingerol abates benzo [a] pyrene-induced colonic injury via suppression of oxido-inflammatory stress responses in BALB/c mice, Chem. Biol. Interact., 2019, 307, 1–7 CrossRef CAS PubMed.
- S. M. Ezzat, M. I. Ezzat, M. M. Okba, E. T. Menze and A. B. Abdel-Naim, The hidden mechanism beyond ginger (Zingiber officinale Rosc.) potent in vivo and in vitro anti-inflammatory activity, J. Ethnopharmacol., 2018, 214, 113–123 CrossRef PubMed.
- M. A. Alsahli, S. A. Almatroodi, A. Almatroudi, A. A. Khan, S. Anwar, A. G. Almutary, F. Alrumaihi and A. H. Rahmani, 6-Gingerol, a major ingredient of ginger attenuates diethylnitrosamine-induced liver injury in rats through the modulation of oxidative stress and anti-inflammatory activity, Mediat. Inflamm., 2021, 2021(1), 6661937 Search PubMed.
- R. Kiyama, Nutritional implications of ginger: Chemistry, biological activities and signaling pathways, J. Nutr. Biochem., 2020, 86, 108486 CrossRef CAS PubMed.
- E. O. Farombi, B. O. Ajayi and I. A. Adedara, 6-Gingerol delays tumorigenesis in benzo [a] pyrene and dextran sulphate sodium-induced colorectal cancer in mice, Food Chem. Toxicol., 2020, 142, 111483 CrossRef CAS PubMed.
- N. Sp, D. Y. Kang, J. M. Lee, S. W. Bae and K. J. Jang, Potential antitumor effects of 6-gingerol in p53-dependent mitochondrial apoptosis and inhibition of tumor sphere formation in breast cancer cells, Int. J. Mol. Sci., 2021, 22(9), 4660 CrossRef CAS PubMed.
- N. Aryaeian, F. Shahram, M. Mahmoudi, H. Tavakoli, B. Yousefi, T. Arablou and S. J. Karegar, The effect of ginger supplementation on some immunity and inflammation intermediate genes expression in patients with active Rheumatoid Arthritis, Gene, 2019, 698, 179–185 CrossRef CAS PubMed.
- M. Rondanelli, F. Fossari, V. Vecchio, C. Gasparri, G. Peroni, D. Spadaccini, A. Riva, G. Petrangolini, G. Iannello, M. Nichetti and V. Infantino, Clinical trials on pain lowering effect of ginger: A narrative review, Phytother Res., 2020, 34(11), 2843–2856 CrossRef PubMed.
- C. Zhong, K. Min, Z. Zhao, C. Zhang, E. Gao, Y. Huang, X. Zhang, M. Baldini, R. Roy, X. Yang and W. J. Koch, MAP kinase phosphatase-5 deficiency protects against pressure overload-induced cardiac fibrosis, Front. Immunol., 2021, 12, 790511 CrossRef CAS PubMed.
- X. Han, P. Liu, M. Liu, Z. Wei, S. Fan, X. Wang, S. Sun and L. Chu, [6]-Gingerol Ameliorates ISO-Induced Myocardial Fibrosis by Reducing Oxidative Stress, Inflammation, and Apoptosis through Inhibition of TLR4/MAPKs/NF-κB Pathway, Mol. Nutr. Food Res., 2020, 64(13), 2000003 CrossRef CAS PubMed.
- S. Song, M. Dang and M. Kumar, Anti-inflammatory and renal protective effect of gingerol in high-fat diet/streptozotocin-induced diabetic rats via inflammatory mechanism, Inflammopharmacology, 2019, 27(6), 1243–1254 CrossRef CAS PubMed.
- G. Saravanan, P. Ponmurugan, M. A. Deepa and B. Senthilkumar, Anti-obesity action of gingerol: effect on lipid profile, insulin, leptin, amylase and lipase in male obese rats induced by a high-fat diet, J. Sci. Food Agric., 2014, 94(14), 2972–2977 CrossRef CAS PubMed.
- S. H. Seo, F. Fang and I. Kang, Ginger (Zingiber officinale) attenuates obesity and adipose tissue remodeling in high-fat diet-fed C57BL/6 mice, Int. J. Environ. Res. Publ. Health, 2021, 18(2), 631 CrossRef CAS PubMed.
- F. Shidfar, A. Rajab, T. Rahideh, N. Khandouzi, S. Hosseini and S. Shidfar, The effect of ginger (Zingiber officinale) on glycemic markers in patients with type 2 diabetes, J. Compl. Integr. Med., 2015, 12(2), 165–170 CAS.
- A. Alipour, V. Baradaran Rahimi and V. R. Askari, Promising influences of gingerols against metabolic syndrome: A mechanistic review, BioFactors, 2022, 48(5), 993–1004 CrossRef CAS PubMed.
- B. Andallu, B. Radhika and V. Suryakantham, Effect of aswagandha, ginger and mulberry on hyperglycemia and hyperlipidemia, Plant Foods Hum. Nutr., 2003, 58, 1–7 CrossRef.
- Y. Li, V. H. Tran, N. Koolaji, C. Duke and B. D. Roufogalis, (S)-[6]-Gingerol enhances glucose uptake in L6 myotubes by activation of AMPK in response to [Ca 2+] i, J. Pharm. Pharmaceut. Sci., 2013, 16(2), 304–312 CAS.
- G. Zhang, V. Nitteranon, L. Y. Chan and K. L. Parkin, Glutathione conjugation attenuates biological activities of 6-dehydroshogaol from ginger, Food Chem., 2013, 140(1–2), 1–8 CrossRef CAS PubMed.
- A. Hosseinzadeh, K. Bahrampour Juybari, M. J. Fatemi, T. Kamarul, A. Bagheri, N. Tekiyehmaroof and A. M. Sharifi, Protective effect of ginger (Zingiber officinale roscoe) extract against oxidative stress and mitochondrial apoptosis induced by interleukin-1β in cultured chondrocytes, Cells Tissues Organs, 2017, 204(5–6), 241–250 CrossRef CAS PubMed.
- X. Han, P. Liu, B. Zheng, M. Zhang, Y. Zhang, Y. Xue, C. Liu, X. Chu, X. Wang, S. Sun and L. Chu, 6-Gingerol exerts a protective effect against hypoxic injury through the p38/Nrf2/HO-1 and p38/NF-κB pathway in H9c2 cells, J. Nutr. Biochem., 2022, 104, 108975 CrossRef CAS PubMed.
- E. Gumbarewicz, A. Jarząb, A. Stepulak and W. Kukula-Koch, Zingiber officinale Rosc. in the treatment of metabolic syndrome disorders—A Review of In Vivo Studies, Int. J. Mol.
Sci., 2022, 23(24), 15545 CrossRef CAS PubMed.
- Y. Xu, Q. Wang, Y. Feng, C. K. Firempong, Y. Zhu, E. Omari-Siaw, Y. Zheng, Z. Pu, X. Xu and J. Yu, Enhanced oral bioavailability of [6]-Gingerol-SMEDDS: Preparation, in vitro and in vivo evaluation, J. Funct.Foods, 2016, 27, 703–710 CrossRef.
- L. Zhong, R. Wang, Q. H. Wen, J. Li, J. W. Lin and X. A. Zeng, The interaction between bovine serum albumin and [6]-,[8]-and [10]-gingerol: An effective strategy to improve the solubility and stability of gingerol, Food Chem., 2022, 372, 131280 CrossRef CAS PubMed.
- M. K. Samota, M. Kaur, M. Sharma, V. Krishnan, J. Thakur, M. Rawat, B. Phogat and P. N. Guru, Hesperidin from citrus peel waste: extraction and its health implications, Qual. Assur. Saf. Crop Foods, 2023, 15(2), 71–99 CrossRef CAS.
- R. P. Singh, H. V. Gangadharappa and K. Mruthunjaya, Phytosome complexed with chitosan for gingerol delivery in the treatment of respiratory infection: In vitro and in vivo evaluation, Eur. J. Pharm. Sci., 2018, 122, 214–229 CrossRef CAS PubMed.
- S. Kaur, S. K. Thukral, P. Kaur and M. K. Samota, Perturbations associated with hungry gut microbiome and postbiotic perspectives to strengthen the microbiome health, Future Foods, 2021, 4, 100043 CrossRef CAS.
- S. R. Falsafi, S. P. Bangar, V. Chaudhary, E. Hosseini, Z. Mokhtari, A. C. Karaca, M. K. Samota, D. Goswami, V. Krishnan, G. Askari and H. Rostamabadi, Recent advances in oral delivery of bioactive molecules: Focus on prebiotic carbohydrates as vehicle matrices, Carbohydr. Polym., 2022, 298, 120074 CrossRef CAS PubMed.
- J. F. Lechner and G. D. Stoner, Gingers and their purified components as cancer chemopreventative agents, Molecules, 2019, 24(16), 2859 CrossRef CAS PubMed.
-
G. Zheng, Y. Yang, C. Li and H. Guo, Functional protein-protein interaction networks regulated by 6-gingerol targeting stomach and small intestine, In 2017 IEEE International Conference on Bioinformatics and Biomedicine (BIBM), IEEE, 2017 Nov 13, pp. 1413–1419 Search PubMed.
- A. Sarrafan, M. Ghobeh and P. Yaghmaei, The effect of 6-gingerol on biochemical and histological parameters in cholesterol-induced nonalcoholic fatty liver disease in NMRI mice, Braz. J. Pharm. Sci., 2021, 57, e18020 CrossRef CAS.
- Q. Wang, Q. Wei, Q. Yang, X. Cao, Q. Li, F. Shi, S. S. Tong, C. Feng, Q. Yu, J. Yu and X. Xu, A novel formulation of [6]-gingerol: Proliposomes with enhanced oral bioavailability and antitumor effect, Int. J. Pharm., 2018, 535(1–2), 308–315 CrossRef CAS PubMed.
- S. Mathur, S. Pareek, R. Verma, D. Shrivastava and P. S. Bisen, Therapeutic potential of ginger bio-active compounds in gastrointestinal cancer therapy: the molecular mechanism, Nutrire, 2022, 47(2), 15 CrossRef CAS.
- J. F. Chen, S. W. Wu, Z. M. Shi and B. Hu, Traditional Chinese medicine for colorectal cancer treatment: potential targets and mechanisms of action, Chin. Med., 2023, 18(1), 14 CrossRef PubMed.
- H. M. Farzaei, R. Bahramsoltani and R. Rahimi, Phytochemicals as adjunctive with conventional anticancer therapies, Curr. Pharmaceut. Des., 2016, 22(27), 4201–4218 CrossRef PubMed.
- S. J. Sampath, S. Birineni, S. Perugu, N. Kotikalapudi and V. Venkatesan, Therapeutic efficacy of 6-gingerol and 6-shogaol in promoting browning of white adipocytes vis-à-vis enhanced thermogenesis portrayed in high fat milieu, Food Biosci., 2021, 42, 101211 CrossRef CAS.
- H. Hattori, A. Moriyama, T. Ohno, T. Shibata, H. Iwahashi and T. Mitsunaga, Molecular networking-based lipid profiling and multi-omics approaches reveal new contributions of functional vanilloids to gut microbiota and lipometabolism changes, Food Chem.: Mol. Sci., 2022, 5, 100123 CAS.
- M. Fu, Y. Liu, H. Cheng, K. Xu and G. Wang,
Coptis chinensis and dried ginger herb combination inhibits gastric tumor growth by interfering with glucose metabolism via LDHA and SLC2A1, J. Ethnopharmacol., 2022, 284, 114771 CrossRef CAS PubMed.
- Q. X. Gan, J. Wang, J. Hu, G. H. Lou, H. J. Xiong, C. Y. Peng and Q. W. Huang, Modulation of apoptosis by plant polysaccharides for exerting anti-cancer effects: a review, Front. Pharmacol, 2020, 11, 792 CrossRef CAS PubMed.
- H. Abdul Khaliq, M. Alhouayek, J. Quetin-Leclercq and G. G. Muccioli, 5’AMP-activated protein kinase: An emerging target of phytochemicals to treat chronic inflammatory diseases, Crit. Rev. Food Sci. Nutr., 2024, 64(14), 4763–4788 CrossRef CAS PubMed.
- D. Domínguez-Balmaseda, C. Bressa, A. Fernández-Romero, B. de Lucas, M. Pérez-Ruiz, A. F. San Juan, M. Roller, N. Issaly and M. Larrosa, Evaluation of a Zingiber officinale and Bixa orellana Supplement on the Gut Microbiota of Male Athletes: A Randomized Placebo-Controlled Trial, Planta Med., 2022, 88(13), 1245–1255 CrossRef PubMed.
-
A. Patel, S. Tiwari, N. Pandey, D. Gupta and S. M. Prasad, Role of spices beyond a flavouring agent: The antioxidant and medicinal properties, In Research Anthology on Recent Advancements in Ethnopharmacology and Nutraceuticals, IGI Global, 2022, pp. 616–648 Search PubMed.
- M. Amouee, S. Montazeri, R. A. Zadeh and M. Ghorbani, The effect of ginger capsule on nausea and vomiting during and after caesarean section under spinal anesthesia, Int. J. Clin. Med., 2016, 7(01), 106 CrossRef CAS.
- Z. Salari, A. Khosravi, E. Pourkhandani, E. Molaakbari, E. Salarkia, A. Keyhani, I. Sharifi, H. Tavakkoli, S. Sohbati, S. Dabiri and G. Ren, The inhibitory effect of 6-gingerol and cisplatin on ovarian cancer and antitumor activity: In silico, in vitro, and in vivo, Front. Oncol., 2023, 13, 1098429 CrossRef CAS PubMed.
- S. Hitomi, K. Ono, K. Terawaki, C. Matsumoto, K. Mizuno, K. Yamaguchi, R. Imai, Y. Omiya, T. Hattori, Y. Kase and K. Inenaga, [6]-gingerol and [6]-shogaol, active ingredients of the traditional Japanese medicine hangeshashinto, relief oral ulcerative mucositis-induced pain via action on Na+ channels, Pharmacol. Res., 2017, 117, 288–302 CrossRef CAS PubMed.
- S. Santos Braga, Ginger: Panacea or consumer's hype?, Appl. Sci., 2019, 9(8), 1570 CrossRef.
- L. Zhen, Q. Wei, Q. Wang, H. Zhang, M. Adu-Frimpong, C. Kesse Firempong, X. Xu and J. Yu, Preparation and in vitro/in vivo evaluation of 6-Gingerol TPGS/PEG-PCL polymeric micelles, Pharmaceut. Dev. Technol., 2020, 25(1), 1–8 CrossRef CAS PubMed.
- A. Amalraj, K. Varma, S. Jude, R. V. Nair and S. Kuttappan, Preparation and characterization of a novel bioavailable gingerol formulation using polar–nonpolar sandwich technology, its antioxidant potential, and an in vitro release study, ACS Food Sci. Technol., 2021, 1(6), 1059–1067 CrossRef CAS.
- L. He, J. Xu, Q. Wang, Y. Zhang, Z. Qin, Y. Yu, Z. Qian, Z. Yao and X. Yao, Glucuronidation of [6]-shogaol,[8]-shogaol and [10]-shogaol by human tissues and expressed UGT enzymes: identification of UGT2B7 as the major contributor, RSC Adv., 2018, 8(72), 41368–41375 RSC.
|
This journal is © The Royal Society of Chemistry 2024 |
Click here to see how this site uses Cookies. View our privacy policy here.