DOI:
10.1039/D4FB00119B
(Review Article)
Sustainable Food Technol., 2024,
2, 1166-1182
Road to valorisation of melon seeds (Cucumis melo L.): a comprehensive review of nutritional profiles, biological activities, and food applications
Received
18th April 2024
, Accepted 4th August 2024
First published on 11th August 2024
Abstract
Melon (Cucumis melo L.) is a commercial fruit planted worldwide in large quantities; meanwhile, substantial amounts of melon seeds as a by-product are generated within the food chain supply but are scarcely utilised. Currently, there is extensive attention on valorisation strategies of melon seeds, driven by circular economy strategies and the UN’s sustainable development goals agenda. This by-product has high potential value and could be re-utilised and re-introduced into the supply chain as a promising ingredient in food development. The aim of this review is to highlight melon seed nutritional composition, biological activities of individual components, and current advances in food product development. Besides that, this review also highlights some promising green extraction technologies for maximising recovery value from melon seeds by reducing environmental burden. Ultimately, this review intends to promote a better understanding of melon seed properties that could enable the efficient utilisation of melon seeds and promote viable valorisation routes.
Sustainability spotlight
Melon seeds (Cucumis melo L.), a major by-product from the melon supply chain, are scarcely utilised and end up as food waste in landfills. However, this waste disposal strategy represents inefficient utilisation of natural resources, leading to economic losses and negative impacts on sustainable food system development. To this end, recycling and adding value to melon seeds as a novel ingredient and re-introducing them into the food chain align well with the sustainable food development and help to enhance the resilience of the food system. This review is expected to contribute to developing valorisation strategies of melon seeds, reducing food waste, and promoting their further development as a sustainable ingredient in food application, which is in line with SDG 2 and SDG 12.
|
1. Introduction
The Food and Agriculture Organisation (FAO) of the United Nations reported in 2015 that around 1.3 billion tons per year of food produced globally are lost or wasted across the food supply chain.1 Food waste is currently a significant issue worldwide and has resulted in negative environmental, economic, and social impacts.1 For this reason, most countries and organisations have been focusing on the development of circular food systems that are based on capturing value and minimizing waste, aiming to reduce food waste by 50% by 2030.1–3 To this end, a variety of food waste management practices have been proposed: (i) recovery and reuse can contribute to food waste reduction and promote an efficient use of natural resources; (ii) development and design of green technologies and circular economy systems towards more sustainable patterns of production, to minimize the adverse effect of chemicals and waste on human health and the environment; (iii) raise public awareness for sustainable development and promote lifestyles in harmony with nature.2–5 Based on this background, the food biorefinery concept has been put forward to advance circular economy across the food supply chain.6–8 The food biorefinery concept considers the recovery of components from food supply chains, including waste or by-products and develops novel valorisation strategies as an extension or addition to established strategies (e.g. animal feed and composting), leading to the production of medium/high value products, such as functional ingredients, chemicals and biomaterials.9
Melon (Cucumis melon L.) is an important commercial horticultural crop in the world. It belongs to the Cucurbitaceae family and is cultivated in several warm parts of the world, including Europe, Asia, America, and Africa.10 Due to its sweet and juicy flesh as well as attractive aroma, melon appeals to a large consumer base and is also processed by the food industry into a variety of food products (e.g. juices, fruit salads, desserts, canned fruit, and ice cream). Data from FAOSTAT (Food and Agriculture Organization of the United Nations Statistical Analysis) in 2021 showed that the annual worldwide production of melon was approximately 29 million tons, with the largest production in Asia (77%), followed by America (11.5%) and Europe (6.9%). As a seasonal fruit, roughly 70% of melon is directly consumed as fresh fruit by households and the rest is processed into food products; in addition, melon seeds, about 5–10% of the total melon weight (about 1.5 to 2.9 million tons), are generally considered as a non-edible part, and therefore classified as a by-product and usually end up in landfills.11–15 This disposal method reflects the inefficient use of natural resources, leading to economic value loss, and has a negative impact on the environment [generating greenhouse gas (GHG) emissions when decomposed in landfills, and soil/water contamination].16,17 In line with the content of the United Nations Sustainable Development Goals (SDG), such as SDG 2 (zero hunger) and SDG 12 (sustainable consumption and production), melon seeds have recently attracted attention as a potential food ingredient, aiming to improve the sustainability of the food system and stimulate the circular economy.
In this context, this review aims to comprehensively summarise the nutritional value of melon seeds and biological activities of individual components, and provide state-of-the-art applications as valuable ingredients in food product development. Besides, this review also highlights the promising green extraction methods to maximise the potential value of melon seeds with a sustainable and eco-friendly approach.
2. Botany, origin and cultivation of Cucumis melo
Melon (Cucumis melo) is one of the most ancient and important crops in the world and has been cultivated for several thousand years. The origin of melon is under debate, but several areas have been proposed such as Asia, Africa and Australia.18 According to the geographical distribution and the most comprehensive phylogenetic analysis, it seems that the initially domesticated melon (Cucumis melo) originated in Asia.19,20 In addition, the highly diversified melon varieties in India and China further support this view.21
Melon (Cucumis melo L.) belongs to the Cucurbitaceae family which includes watermelon, squash, cucumber and pumpkin.22,23 As a result of variety, and morphological and physiological diversity, two subspecies were put forward to distinguish melon, C. melo subsp. melo and C. melo subsp. agrestis.24 In addition, melon is divided further into 16 botanical species, with 11 botanical group classifications in C. melo subsp. melo and 5 botanical group classifications in C. melo subsp. agresits.25,26 Some of these groups are major commercial melon varieties due to their great taste and high productivity; the most commercially important groups and their representing varieties are listed in Table 1 and Fig. 1. In the UK, the most consumed melon varieties are Galia, Honeydew, Cantaloupe, Piel de Sapo, and Matice, mainly from Spain, Brazil, Honduras, and Costa Rica.11
Table 1 The most commercially important cultivar groups of melon and their characteristicsa
Number |
Group name |
Representing variety |
Description |
Compiled from ref. 25 and 27–29.
|
(a) |
Reticulatus (subsp. melo) |
Netted muskmelon |
Round, netted, having a green or orange flesh |
(b) |
Cantalupensis (subsp. melo) |
Cantaloupe melon |
Round, smooth or warted, flesh green or orange, non-netted |
(c) |
Inodorus (subsp. melo) |
Honeydew melon |
Smooth-skinned, having a sweet, juicy, and light green to white flesh |
(d) |
Flexuosus (subsp. melo) |
Serpent melon |
Long to very long (cucumber-like), wrinkled or smooth, flesh white and not sweet or slightly acidic |
(e) |
Conomon (subsp. agrestis) |
Oriental pickling melon |
Smooth, green or white peel, with white flesh, sweet or bland not aromatic |
(f) |
Chito (subsp. melo) |
Garden melon |
Round, smooth, small lemon or orange-size, with bland white flesh |
(g) |
Dudaim (subsp. melo) |
Queen Anne's pocket melon |
Small, striped orange or brown, white and bland flesh, highly aromatic |
(h) |
Chinensis (subsp. agrestis) |
Songwhan Charmi melon |
Pyriform, green with spots, medium sweet with green or orange flesh, not aromatic |
(i) |
Makuwa (subsp. agrestis) |
Oriental melon |
Oblate, smooth, light or yellow peel, sweet white flesh and quite aromatic |
 |
| Fig. 1 Images of representative melon cultivar varieties.27,28 | |
3. Chemical composition of melon seeds
Melon seeds have an oval shape, a smooth hard surface with yellow colour, and a white-yellow inner kernel (Fig. 2). It has been reported that melon seeds are a rich source of oil, protein, and minerals.10,30 Their composition is summarised in Table 2 and is affected by multiple factors, including variety, region, climate, and growing conditions among others.12,31–34
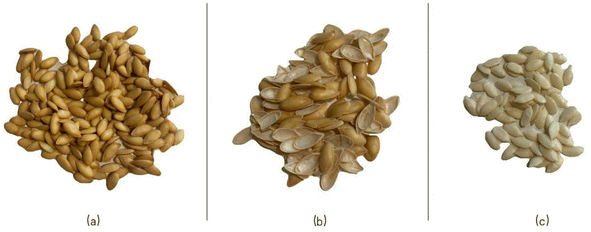 |
| Fig. 2 The morphology of the different parts of melon seeds (Cucumis melo L.): (a) the whole melon seeds; (b) the shell of melon seeds; (c) the kernel of melon seeds. | |
Table 2 Proximate composition of melon seeds
Component (%, w/w) |
Melon seed (Cucumis melo) |
“Maazoun” variety |
“Ananas” variety |
C. melo var. reticulates Naud hybrid “Chunli” |
Three varieties |
Cucumis melo var. inodorus |
Cucumis melo hybrid AF-522 |
Protein |
— |
27.41 |
26.15 |
29.90 |
34.6–39.8 |
25.0 |
14.91 |
Ash |
4.08 |
4.83 |
2.78 |
4.05 |
4.6–5.1 |
2.4 |
4.20 |
Oil |
30.7 |
30.65 |
28.44 |
35.36 |
41.6–44.5 |
25.0 |
30.83 |
Fibre |
— |
25.32 |
34.08 |
19.52 |
4.5–8.5 |
23.3 |
19.00 |
![[thin space (1/6-em)]](https://www.rsc.org/images/entities/char_2009.gif) |
Minerals (mg per 100 g)
|
Potassium |
509.804 |
1148.75 |
994.04 |
— |
— |
— |
— |
Magnesium |
101.715 |
1062.25 |
745.94 |
— |
— |
— |
— |
Calcium |
55.392 |
506.13 |
691.9 |
— |
— |
— |
— |
Sodium |
41.176 |
336.5 |
400.00 |
— |
— |
— |
— |
Iron |
4.901 |
2.69 |
23.13 |
— |
— |
— |
— |
Zinc |
4.656 |
2.34 |
9.40 |
— |
— |
— |
— |
Copper |
0.833 |
0.53 |
64.86 |
— |
— |
— |
— |
References |
61
|
30
|
60
|
34
|
33
|
31
|
62
|
3.1. Protein
The protein content of melon seeds ranges from 14.91% to 39.8% (w/w) (Table 2) and is comparable to other high oil seeds in protein content, including flaxseeds (20.3%, w/w), rapeseed (19%, w/w), sesame seeds (15.7%, w/w), and sunflower seeds (24.2%, w/w);35 in addition, compared with other by-products in protein content, such as Japanese quince (Chaenomeles japonica) seeds (24.6–33.2%, w/w),36 chayote (Sechium edule (Jacq.) Swartz) seeds (5.5% w/w),37 mango seed kernels (6.7% w/w);38 the above results indicate that melon seeds could be a potential source of protein. Glutelin (38.7%, w/w of protein), albumin (34.5%, w/w of protein), and globulin (24.1%, w/w of protein) are the predominant protein fractions, whereas prolamin is usually present in low levels (2.8%, w/w of protein). In terms of protein solubility, it is a typical U curve and the minimum solubility is at pH 4.39,40 With regard to the amino acid profile, the major amino acids of melon seed protein are glutamic acid (17.5–20% of protein), arginine (13.4–17% of protein), leucine (7.4–7.5% of protein), and aspartic (9.0–10% of protein), whereas lysine (2.8–3.5% of protein) and methionine (0.8–2.2% of protein) are present in low levels.32,34,41 Several studies have shown that melon seed protein contains all 10 essential amino acids but has only a small amount of cysteine (non-essential amino acid).32,34,42
3.2. Fibre
Dietary fibre is important to human gut health and can increase satiety to reduce food intake.43 Many studies in the literature have demonstrated the biological activity of dietary fibre through in vitro (e.g. antioxidant capacity assays and α-amylase activity inhibition) and in vivo (e.g. animal models and human trials), including maintenance of a health gut microbiome, hypoglycaemic properties, and antioxidant activity.44–47 The dietary fibre in melon seeds ranges from 19.52% to 34.08% w/w (Table 2). In addition, dietary fibre can be divided into soluble dietary fibre (SDF) (e.g. pectin, oligosaccharides, and soluble hemicelluloses) and insoluble dietary fibre (IDF) (e.g. cellulose, insoluble hemicelluloses, and lignin).43 Studies conducted by Mallek-Ayadi et al.30 reported that SDF and IDF in melon seeds of Maazoun variety were 3.14% and 22.18% w/w, respectively, indicating that IDF is the major dietary fibre in melon seeds. In addition, Zhang et al.48 investigated the carbohydrate composition of melon seeds and the results showed that melon seeds contained 4.8–5.4% (w/w) of cellulose, 3.3–3.7% (w/w) of hemicellulose, and 6.0–6.8% (w/w) of lignin. However, further detailed information on the carbohydrate composition of melon seeds is still scarce; further research is needed to provide more comprehensive understanding of melon seed carbohydrates, their nutritional profile and any potential benefits for human health.
3.3. Minerals
The mineral content of melon seeds is detailed in Table 2, with the most prominent ones being potassium, magnesium, calcium, and sodium. It is important to note that the potassium content can reach 1148.75 mg per 100 g in melon seeds (Table 2). According to the new guidance on dietary potassium from the WHO, potassium-rich foods include bean and peas (∼1300 mg/100 g), nuts (∼600 mg/100 g), spinach (∼550 mg/100 g), and banana (∼350 mg/100 g).49 Compared with these potassium-rich foods, it can be concluded that melon seeds have a high content of potassium and could be considered as a new source of potassium. The high sodium dietary intake is a major public health issue in both developed and developing countries and is linked with increased risk of hypertension and cardiovascular diseases. The WHO suggests increased potassium intake and decreased sodium intake to reduce the risk of heart disease.50,51 In potassium fortified food applications, Srivastava52 used virgin coconut meal (1700 mg/100 g of potassium) as wheat flour substitution in biscuit production and found that biscuits containing 25% virgin coconut meal (442.06 mg/100 g) had a seven-fold increase in potassium content compared to control biscuit (100% wheat flour, 59.24 mg/100 g). Additionally, Sanz-Penella et al.53 added whole amaranth flour (470 mg/100 g of potassium) in bread production and 40% addition level increased potassium content of bread from 188 mg/100 g to 321 mg/100 g. Based on the above studies, the potential of melon seeds as a promising ingredient to fortify potassium content in food production is revealed. Besides, melon seeds can also be regarded as a source of calcium (506 mg/100 g) when compared with plain low-fat yogurt (189 mg/100 g), tofu (183 mg/100 g), and whole milk (116 mg/100 g).54
3.4. Oil
The oil content of melon seeds ranges from 25.0% to 44.5% w/w (Table 2). Compared with other conventional oilseeds such as sunflower seeds (approximately 40% w/w),55 peanut (approximately 50% w/w),56 and soybean (approximately 15% w/w),57 the oil content in melon seeds is generally similar to that in sunflower seeds and peanut and higher than that of soybean. Due to the expansion of markets and the increased requirement for food and non-food applications of vegetable oils (e.g. biodiesel), the development of new oil sources has been important for many oilseed-importing countries.58 Compared with other novel sources, melon seeds have higher quantities of oil than mango seeds (7.0% w/w), strawberry seeds (7.6% w/w), grape seeds (9.7% w/w) and kumquat (33.5% w/w),59 indicating that melon seeds could be used as a novel source of oil.
3.4.1. Fatty acid composition of melon seed oil.
The fatty acid composition of melon seed oil is shown in Table 3. It is mainly constituted of linoleic acid (C18:2, 55.90–68.98%), followed by oleic acid (C18:1, 12.101–33.96%), palmitic acid (C16:1, 7.19–23.878%), and stearic acid (C18:0, 4.57–7.80%).30,31,33,34,60–62 The linoleic acid content of melon seed oil is similar to that of apple seed oil (58.9%), sunflower oil (62.2%), soybean oil (53.2%), and grape seed oil (74.7%).42,59,63 According to Marangoni et al.64 linoleic acid is inversely correlated with cardiovascular disease risk. Additionally, Wang et al.14 detected conjugated linolenic acid (CLnA) in melon seeds (1.97–2.16 mg CLnA per g seed), which was lower than that in cherry seeds (12.79 CLnA per g seed), but higher than that in apple seeds (0.38 mg CLnA per g seed) and pear seeds (0.05 mg CLnA per g seed). CLnA is a positional and geometric isomer of linolenic acid (C18:3), and has been suggested to have potentially positive effects on cardiovascular health.65,66
Table 3 Fatty acid composition of melon seed oila
Fatty acid compositions (%) |
Nine varieties of melon seed oil |
“Maazoun” variety |
Cantaloupe melon seed oil (Cucumis melo L. var. reticulatus) |
Three varieties of melon seed oil |
Honeydew melon seed oil (C. melo var. inodorus) |
C. melo var. reticulates Naud hybrid ‘ChunLi’ |
Culinary melon seed oil (Cucumis melo var.acidulus) |
Cucumis melo hybrid AF-522 |
SFA – saturated fatty acid; MUFA – monounsaturated fatty acid; PUFA – polyunsaturated fatty acid.
|
C12:0 |
— |
— |
— |
— |
0.2 |
0.023 |
— |
— |
C13:0 |
— |
— |
— |
— |
— |
0.006 |
— |
0.36 |
C14:0 |
0.04–0.06 |
0.04 |
— |
— |
0.2 |
0.251 |
0.056 |
0.04 |
C15:0 |
0.02–0.03 |
0.03 |
— |
— |
— |
0.132 |
— |
0.03 |
C16:0 |
7.19–9.74 |
8.71 |
9.1 |
9.88–15.65 |
8.4 |
23.878 |
11.65 |
9.52 |
C17:0 |
0.06–0.08 |
0.07 |
0.1 |
— |
— |
0.191 |
— |
0.07 |
C18:0 |
4.57–5.86 |
5.54 |
4.6 |
5.81–7.80 |
4.6 |
5.671 |
7.40 |
4.89 |
C20:0 |
0.17–0.28 |
0.16 |
0.1 |
0.24–0.56 |
0.5 |
0.272 |
0.248 |
0.18 |
C21:0 |
0.00–0.01 |
— |
— |
— |
— |
0.035 |
— |
— |
C22:0 |
0.03–0.07 |
— |
— |
— |
— |
0.425 |
0.077 |
— |
C23:0 |
— |
— |
— |
— |
— |
0.221 |
— |
— |
C24:0 |
0.04–0.05 |
0.06 |
0.1 |
— |
— |
1.456 |
— |
0.12 |
SFA |
12.12–16.18 |
14.61 |
14.0 |
15.93–24.01 |
13.9 |
32.561 |
19.431 |
15.21 |
C15:1 |
— |
— |
— |
— |
— |
0.309 |
— |
— |
C16:1 |
0.08–0.12 |
0.08 |
0.1 |
— |
0.3 |
0.096 |
0.076 |
0.17 |
C17:1 |
0.01–0.03 |
0.03 |
— |
— |
— |
0.019 |
|
0.03 |
C18:1 |
15.23–33.96 |
15.84 |
15.9 |
14.25–29.30 |
16.8 |
12.101 |
13.70 |
19.42 |
C20:1 |
0.11–0.16 |
0.13 |
0.1 |
— |
— |
0.078 |
— |
0.11 |
C22:1 |
— |
0.02 |
— |
— |
— |
— |
— |
0.40 |
C24:1 |
— |
— |
— |
— |
— |
0.071 |
— |
— |
MUFA |
15.43–34.27 |
16.1 |
16.1 |
15.25–29.30 |
17.1 |
12.674 |
13.776 |
20.1 |
C18:2 |
50.69–69.22 |
68.98 |
63.5 |
45.90–68.07 |
69.0 |
53.872 |
66.55 |
64.13 |
C18:3 |
0.15–0.26 |
0.2 |
0.2 |
0–0.22 |
— |
0.871 |
0.22 |
0.20 |
C20:2 |
0.01–0.01 |
— |
— |
— |
— |
0.028 |
— |
— |
PUFA |
50.85–69.49 |
70.08 |
63.70 |
45.90–68.27 |
69.0 |
54.771 |
66.77 |
64.33 |
References |
12
|
30
|
115
|
60
|
31
|
34
|
73
|
62
|
3.4.2. Physicochemical characteristics of melon seed oil.
The physicochemical characteristics of the oil indicate its quality. Table 4 lists the various chemical and physical parameters of melon seed oil which highlight its potential as edible oil. The acid value is normally positively correlated with the free fatty acid content, whereas the peroxide value is related to the degree of lipid oxidation.67,68 These two parameters indicate oil stability during storage because high acid and peroxide values characterise oil that are more susceptible to oxidation.69 Melon seed oil has a relatively good oxidative stability, as shown by its low peroxide and acid values (Table 4).12,70–72 The iodine value reflects the level of unsaturated content in oil.69 The high iodine value of melon seed oil indicates a high level of unsaturated fatty acid composition as also confirmed by its high content of oleic acid (18
:
1) and linoleic acid (18
:
2).30,60,72,73 The saponification value indicates the content of high molecular weight triacylglycerols.71 The saponification value of melon seed oil is slightly higher than that of other crop oils (e.g. sunflower oil, pumpkin oil, and kenaf seed oil) and this indicates a high content of high molecular weight triacylglycerols.69,71,74 Generally, the iodine and saponification values can be used as indicators of the potential nutritional value of the oil.68,75 Therefore, and considering the above, it can be suggested that melon seed oil could be considered a novel edible oil with commercial potential.
Table 4 Physicochemical parameters of melon seed oil
Parameters |
“Ananas” variety |
C. melo var. reticulates Naud hybrid “Chunli” |
Three varieties |
Cucumis melo hybrid AF-522 |
Three varieties |
Acid value (mg KOH per g) |
9.60 |
1.51 |
1.5–2.1 |
2.06 |
07–1.8 |
Peroxide value (meq O2 per kg) |
1.04 |
3.95 |
1.1–3.4 |
4.96 |
6.7–8.9 |
Iodine value (g I2 per 100 g) |
128.44 |
89.5 |
115–128 |
112 |
117.0–147.2 |
Saponification value (mg KOH per g) |
178.30 |
226.73 |
— |
210.62 |
108.9–205.4 |
References |
60
|
34
|
33
|
62
|
105
|
3.4.3. Sterols and tocopherols in melon seed oil.
Sterols have similar chemical structures and biological properties to cholesterol and are associated with LDL-cholesterol reduction in humans.76,77 The sterol content in melon seed oil is shown in Table 5. Total sterol content is reported to be higher than that of some conventional oils, such as sunflower (192 mg/100 g of oil), pumpkin (91.3 mg/100 g of oil), and soybean (164.9 mg/100 g of oil), but lower than that of grape seed (300.5 mg/100 g of oil), tomato seed (169.8 mg/100 g of oil) and passion fruit seed oil (244.4 mg/100 g of oil).30,78,79 Petkova & Antova33 analysed the sterol composition from three melon seed varieties and reported that β-sitosterol was the main component (50–64%), followed by Δ5-avenasterol (19.7–42.7%) and stigmasterol (2.7–4.8%).
Table 5 Sterols and tocopherol content in melon seed oil
Sterols (mg per 100 g of oil) |
Yellow melon seed oil (Cucumis melo var. inodorus Naudin) |
Canary melon seed oil (Cucumis melo L.) |
“Maazoun” variety |
“Ananas” variety |
Nine varieties |
Campesterol |
— |
— |
0.92 |
1.13 |
— |
Campestanol |
— |
|
0.59 |
0.20 |
— |
Stigmasterol |
— |
— |
1.29 |
2.11 |
— |
β-Sitosterol |
21.02 |
— |
206.42 |
324.84 |
— |
Sitostanol |
— |
— |
4.84 |
10.78 |
|
Δ5-Avenasterol |
— |
— |
2.18 |
153.31 |
— |
Cholesterol |
— |
— |
0.88 |
0.36 |
— |
Stimastanol |
11.71 |
— |
— |
— |
— |
Brassicasterol |
|
— |
— |
3.20 |
— |
Total phytosterol |
32.73 |
— |
217.12 |
495.93 |
— |
![[thin space (1/6-em)]](https://www.rsc.org/images/entities/char_2009.gif) |
Tocopherols (mg per 100 g of oil)
|
α-Tocopherol |
2.05 |
6.88 |
2.85 |
— |
3.74–7.47 |
β-Tocopherol |
— |
— |
— |
— |
— |
γ-Tocopherol |
24.96 |
63.08 |
18.13 |
— |
9.98–45.67 |
δ-Tocopherol |
ND |
0.77 |
6.09 |
— |
0.93–2.72 |
Total tocopherols |
27.01 |
70.73 |
27.07 |
— |
14.65–55.86 |
References |
78
|
80
|
30
|
60
|
12
|
Tocopherols, major forms of vitamin E, are natural lipid-soluble antioxidants, which include homologues of α, β, γ, and δ (Fig. 3). γ-Tocopherol is predominant in melon seed oil80 and is considered a highly effective antioxidant that can inhibit oil oxidation and increase the oxidative stability by scavenging the peroxy radicals81 Hashemi et al.82 investigated the oxidative stability of several seed oils under microwave heating conditions; melon seed oil was more stable than watermelon oil, due to the tocopherol content of the former (125 mg kg−1) compared to watermelon oil (111 mg kg−1).
 |
| Fig. 3 Chemical structure of tocopherol (Fine et al.79). | |
4. Extraction technology
Based on their composition, melon seeds hold potential as a source of value-added compounds, such as lipids, protein, fibre, minerals, and polyphenols, and can have a variety of applications in many sectors, such as food, pharmaceutical, and cosmetics. Recovery of these value-added compounds can maximise the value of melon seeds. Therefore, appropriate extraction technologies are key for scale-up studies and commercial applications83 In the following sections, extraction technologies are discussed in detail, including conventional and novel green technologies, targeting the recovery of value-added compounds from melon seeds. Additionally, the advantages and disadvantages of conventional and novel green technologies (supercritical fluid extraction, enzyme assisted extraction, and ultrasonic assisted extraction) are listed in Table 6.
Table 6 Summary of advantages and disadvantages of conventional and novel green technologiesa
Method |
Advantages |
Disadvantages |
Data compiled from ref. 84–90.
|
Conventional solvent extraction (CSE) |
Simplicity of process |
Solvent residue in final products |
Cost effectiveness |
Use of large amount of toxic solvents |
Modulation of selectivity by solvent choice |
Energy intensive |
|
Flammable risks |
Supercritical fluid extraction (SFE) |
Low temperature |
Expensive and complex equipment |
Less or no solvent usage |
High operation cost |
Fast extraction rate |
Low polarity of supercritical CO2 |
Easy solvent recovery avoiding expensive post-processing |
|
Enzyme assisted extraction (EAE) |
Enzyme reusability |
Long incubation time |
Mild reaction conditions |
Complicated drying process after enzymatic treatment |
Low energy consumption and operational costs |
Lack of long-term stability of enzymes |
High selectivity |
Enzyme cost |
Ease of operation |
|
Ultrasonic assisted extraction (UAE) |
Simplicity of process |
Non-uniform distribution of ultrasound energy |
High yield |
Degradation of active compounds from plant matrices due to oxidative pyrolysis caused by hydroxyl (OH–) radicals during cavitation |
Fast extraction rate |
|
Less solvent usage |
|
4.1. Conventional solvent extraction (CSE)
Solvent extraction is a conventional method for the recovery of high value-added compounds, such as protein, oil, polysaccharides, and polyphenols. Solvent extraction usually includes solid–liquid and liquid–liquid extraction. The process involves mixing the material with a suitable non-polar solvent (e.g. hexane and petroleum ether for oil extraction) or polar solvent (e.g. ethanol and methanol for polyphenol extraction) to make the compound move into the extraction solvent where it can be separated or concentrated.83,91,92 However, some of the organic solvents used, such as n-hexane and tetrahydrofuran, could be potentially harmful and toxic for humans, animals and/or the environment.93 Besides, conventional solvent extraction has many drawbacks, including long operation times, poor extraction efficiency, solvent residue in final products and flammable risks.84,85 Therefore, novel, environmentally friendly extraction methods are needed to overcome these drawbacks.
4.2. Supercritical fluid extraction (SFE)
Supercritical fluid extraction (SFE) is an environmentally friendly extraction method that mainly uses carbon dioxide (CO2; typical non-polar solvent; non-toxic and green) as a supercritical fluid. CO2 at its supercritical fluid state has high solvation power and can diffuse like a gas in solid matrices.94–96 In addition, ethanol or methanol, as a polar co-solvent, can be added into supercritical CO2 to target the extraction of polar compounds (e.g. phenolics) (Fig. 4).93 Supercritical fluid extraction has many advantages compared with conventional solvent extraction including a faster, cleaner, and easier separation of the solute from the solvent. SFE has demonstrated it potential for extraction of many compounds, such as oils, phenolics, and pigments. For example, Lima et al.98 used the SFE technique to extract phenolics from potato (Solanum tuberosum) peels and found that 37% of the total phenolic acid content and 82% of the caffeic acid in this matrix were successfully extracted under the optimized process conditions (80 °C, 350 bar, MeOH 20%, and CO2 flow rate of 18.0 g min−1). Additionally, Maran et al.99 used SFE to extract oil from melon seeds. They reported that the fatty acid composition of melon seed oil was not different between SFE and hexane Soxhlet extraction, but the total yield of oil (48.11%) using SFE (extraction at 44 MPa, 49 °C, 0.64 g min−1 of flow rate, and 81 min of extraction time) was slightly higher than that of hexane Soxhlet extraction (46.83%). Ekinci & Gürü100 reported that the highest yields of extracted oil, β-sitosterol, and stigmasterol by SFE were 36.8 g/100 g, 304 mg kg−1, and 121 mg kg−1, respectively, whereas the optimal conditions were 33 °C, 200 bar, and 11 g CO2/min, extraction time of 3 h, and the mean particle size of 0.4 mm.
 |
| Fig. 4 Schematic representation of the supercritical CO2 system coupled with co-solvent (Koubaa et al.97). | |
4.3. Enzyme assisted extraction (EAE)
Enzyme assisted extraction (EAE) is an environmentally friendly extraction method because enzymes can be combined with green solvents (water) or aqueous-alcoholic solvents (e.g. aqueous methanol solvent and aqueous ethanol solvent) to eliminate or reduce the amount of organic solvent usage.101 The principle of enzyme assisted extraction is that specific enzymes (such as cellulases, pectinases, and hemicellulases) are selected to disrupt or hydrolyse the cell wall structure, enhance cell permeability and thus increase the extraction yield of target components (e.g. oil and polyphenols) (Fig. 5).91,102,103 It is important to note that many parameters should be considered to achieve optimal extraction conditions in the enzyme assisted processing, including pH, temperature, time, particle size of samples, nature of the extraction solvent, enzyme concentration, agitation rate and the ratio between the enzyme and substrate.84,91,101 EAE has demonstrated its potential for oil extraction. For example, Ribeiro et al.104 compared oil extraction from sesame oil by EAE (using pectinase and alcalase) and conventional solvent extraction; it showed that the quantity of the oil extracted by EAE (36.65%) was lower than that from solvent extraction (59.97%), while the quality of the EAE extracted sesame oil was better than that conventionally extracted in terms of antioxidant capacity, total phytosterol content, and total polyunsaturated fatty acid content. In addition, Zhang et al.105 extracted melon seed oil in three varieties and compared the oil quality between EAE (using protease and cellulase) and conventional extraction methods (cold-pressed and Soxhlet extraction); the results showed that melon seed oil obtained by EAE (15.0–67.3 mg/100 g of oil) had higher tocopherol content than that of oil extracted by conventional extraction methods (6.8–52.4 mg/100 g of oil). In addition, during the storage test, the EAE derived oil exhibited better oxidative stability compared to the oil extracted by other two conventional methods. However, in practical oil extraction, EAE could lead to the formation of an emulsion, which in turn needs de-emulsification processing to further improve the oil extraction yield.
 |
| Fig. 5 Schematic representation of extraction assisted by enzymes. | |
4.4. Ultrasonic assisted extraction (UAE)
The principle of ultrasonic assisted extraction (UAE) is based on the energy change of the expansion of sound waves in a liquid (Fig. 6).92 In UAE processing, the transmission of ultrasound through the medium generates cavitation bubbles which grow further and collapse, resulting in vibration, mixing, and pulverization; these in turn, enhance the molecular vibration frequency, disrupt the cell wall, and accelerate the rate of heat or mass transfer.91,93,107 Therefore, the extraction yield is increased and extraction time is decreased.107 Process parameters such as type of solvent, extraction time and temperature, as well as frequency of ultrasound waves, have been mentioned as key variables for the effectiveness and efficiency of the UAE process.108 UAE has been successfully applied in oil, protein, phenolics, and other bioactivity compounds. Rivas et al.109 and Piasecka et al.110 successfully used the UAE method to extract dietary fibre and oil from broccoli by-products (leaves, stems and inflorescence remains) and cranberry seeds, respectively. Besides, UAE can be combined with other extraction methods to facilitate cell wall disruption and overcome some limitations of other extraction methods (e.g. considerable long extraction time and low extraction efficiency).101 For example, it can be combined with the enzymes, leading to an ultrasonic-assisted enzymatic extraction (UAEE) method.91 Tang et al.103 and Kumar T. et al.111 used the UAEE method to extract dietary fibre and oil in bamboo shoot by-products (the head and foot of Chimonobambusa quadrangularis shoots) and sea buckthorn berry, respectively. The potential advantage that UAEE could offer is the acceleration of extraction and enzymatic hydrolysis processes through cavitation, thereby improving the enzymatic extraction efficiency.112
 |
| Fig. 6 Schematic representation of ultrasonic-assisted extraction (More et al.106). | |
5. Utilisation of melon seeds in food product development
The conversion of food by-products as ingredients in food formulations is one of the most attractive strategies to reduce food loss and capture value. Recently, the utilisation of melon seeds and their subsequent components in food formulation has been explored and has demonstrated their potential as ingredients. The following section presents current advances in the application of melon seeds and their components in various food matrices.
Karakaya et al.113 developed a melon seed beverage that was made of melon seeds, sugar, and water through blending, and demonstrated that the beverage was a good source of minerals (iron and magnesium) and protein, and gave very good results in the hedonic test as ‘liked very much’ (4.9 on a 5-point hedonic scale). In addition, Zungur Bastıoğlu et al.114 produced a plant-based milk from melon seed spray dried powder as alternatives to vegans, vegetarians, and lactose-intolerant. Melon seed spray-dried powder showed good solubility (92.0–99.2%) and storage stability (moisture content and water activity are 2.1–2.4% and 0.260–0.310, respectively) but exhibited poor flowability (50.44–53.23 Carr index value) and tended to form lumps. In terms of sensory evaluation, melon seed milk showed very good visual attributes, particularly a creamy, white colour appearance.
da Cunha et al.115 used melon seeds in baker's confectionary products. Specifically, melon seed flour was used as raw material to partly replace wheat flour in three inclusions: 10% melon seed flour, 30% melon seed flour, and 50% melon seed flour; the control was 100% wheat flour. The results demonstrated that all cakes containing melon seed flour were well accepted by the panel, as did the control group (100% wheat flour); in terms of their flavour (sweetness) attribute, the score was slightly reduced with increasing the ratio of melon seed flour. In the analysis of global acceptance (nine-point scale), the 10% melon seed flour cake had the highest acceptance (7.08) compared to the control (6.56).
Tarjuelo et al.116 reformulated dried sausage (fuet) using melon seed oil (extracted using a hydraulic press) as a pork fat substitute, at 50%, 75%, and 100% (w/w). Reformulated fuet sausages had higher linoleic acid content as compared to control fuet sausages (100% pork fat). However, in the sensory evaluation, the sensory score for fuet sausages made with melon seed oil was lower than that of the control in terms of appearance, texture, odour, and taste. In terms of texture, fuet sausage made with melon seed oil had a significantly lower (p < 0.05) hardness value than the control. Overall, from a nutritional point of view, the evidence in this study suggests the possibility of melon seed oil in sausage application, which could lead to nutritionally more balanced meat products.
Zhang et al.117 used defatted melon seed as a wheat flour substitute in bread production at 5% and 10% replacing levels. The results showed that adding defatted melon seeds can improve the bread nutritional value, especially fibre content; bread made with 10% defatted melon seeds (3.41 g/100 g) showed more than five-fold fibre content compared to 100% wheat flour bread (0.56 g/100 g). However, in terms of bread physical characteristics, defatted melon seed addition decreased the bread volume and cell number and increased the bread hardness value; in addition, as addition of defatted melon seed increased, bread crust became more darker. Subsequently, Zhang & Li118 used different particle sizes of melon seed oil cake (MSOC, obtained from cold-pressed) as wheat flour substitution in bread production at 3% and 6% replacing levels. The results demonstrated that bread containing 3% MSOC exhibited satisfactory quality compared to control bread (100% wheat flour) in terms of bread volume and texture; in addition, reduction of the MSOC particle size could result in a lower bread volume and harder texture but with an improved crumb structure.
The above studies highlight some promising progress with regard to melon seed utilisation in food formulations. However, challenges in product quality and organoleptic aspects still exist and further work is required to optimise processing parameters and develop products acceptable by consumers. Additionally, the food matrix can influence the bioactivity and bioavailability of nutrients during the digestion process, and currently, there is still no sufficient information regarding the potentially nutritional properties of incorporated melon seed in food. Based on this point, further research is needed to identify and evaluate the bioavailability, absorption, and bioactivity of melon seed nutrients in vivo. Moreover, considering the safety and quality of by-products is also an important part of valorisation strategies to ensure food safety for customer consumption. In general, allergens, microbiological safety, and contamination assessment (e.g. pesticide residues and toxins) are the most common considerations in food safety evaluation.17,119 Although there is no available information about melon seed safety and quality at present, it merits an important aspect of research in the future.
6. Biological activity of melon seed compounds
Melon seeds could be a potential source of natural bioactive compounds. Research studies have indicated the biological activity of melon seeds including their antioxidant and anti-proliferative activities, as well as their health benefits in preventing or reducing the risk of chronic diseases, such as diabetes, obesity, and cardiovascular conditions.120–124 Studies by Chen & Kang120 demonstrated in vitro that hexane extracts of the melon (Cucumis melo L. var. makuwa Makino) seed have potential ability as an anti-diabetic by inhibiting the activity of α-glucosidase and α-amylase, by 35% and 62% respectively; this is because the inhibition of these enzymes can delay carbohydrate digestion and glucose absorption thereby controlling the increase in post-prandial plasma glucose, which has potential benefits for type 2 diabetics. According to the study, this could be attributed to presence of unsaturated fatty acids in the hexane extracts of melon seeds. Additionally, Chen et al.121 further demonstrated that the three key fatty acids in the melon extracts, palmitic, linoleic and oleic acid, had high enzyme inhibitory effects in vitro, especially linoleic acid which demonstrated the strongest inhibition towards α-glucosidase and α-amylase.
Rolim et al.122 studied the anti-proliferative effects of melon seed extracts on cancer cells (SiHa, HeLa, HT-29, and 786-0) and attributed this to their phenolic content. The results showed that melon seed extracts, obtained using distilled water, aqueous ethanol (30
:
70, v/v), and aqueous methanol (30
:
70, v/v) as solvents, exhibited inhibition activity for all four types of cancer cells. Zhang et al.125 demonstrated that melon seed extracts obtained using methanol, distilled water, and chloroform showed highly effective anticancer activity in HeLa cell lines and were more cytotoxic to HCT116 cell lines.
Rasouli et al.124 purified protein from melon seeds as a trypsin inhibitor and explored its potential anti-angiogenesis and anti-proliferation activities. The results showed that the molecular mass of purified protein was 3 kDa and the trypsin inhibition activity was 765 CIU mg−1 (chymotrypsin inhibitory unit per mg of protein). When the concentration of purified protein reached 40 μg mL−1, the angiogenesis in the Human Umbilical Vein Endothelial Cells (HUVEC) capillary tube formation model was completely inhibited. Moreover, even at very high concentrations, i.e. 120 μg mL−1, there was no cytotoxic effect as assessed by LDH cytotoxicity assays. Thus, this study indicated that purified melon seed protein could be used as a botanical derivative promoting anti-angiogenesis and anti-proliferation effects.
Although melon seed extracts seem to exhibit promising bioactive properties in in vitro studies, there is lack of information derived from in vivo studies that could further support these findings. Therefore, there is a need for in vivo studies to produce more comparable and reliable data, to validate the bioactive effects of melon seed extracts on health and assist in the design of melon seed valorisation strategies.
7. Environmental and economic impact
Food and agriculture process waste is becoming an acute issue in the world. According to the estimates, global food waste is around 30% (around 1.3 billion tons), which increases greenhouse gas (GHG) emissions by 6% indirectly and causes economic value losses about 1 trillion US dollar value.126,127 As mentioned earlier, melon seed waste is around 1.5–2.9 million tons. To this end, developing comprehensive valorisation strategies for melon seed waste is of great significance to reduce food waste, which can reduce economic losses and is beneficial for the environment.
From an environmental impact point of view, GHG emissions per ton of food waste/by-products could be up to 706–896 kg CO2 eq.128 Therefore, reducing and re-utilisation of melon seed waste could reduce GHG emissions to a large extent, which can reduce the environmental burden. In addition, high efficiency utilisation of food waste for food production can reduce food losses, and help to reduce food waste in landfills, contributing to a win–win situation across the dimensions of food security and environmental protection.
Apart from the environmental protection, evidence supports the economic viability of upcycled food by-products. For example, Wang et al.14 reported that in the USA, melon seed waste can generate 37.5 tons of CLnA (conjugated linolenic acids), which can create over 6 million US dollar value. As mentioned earlier, melon seeds also contain other high potential phytochemicals available, such as fibre, protein, minerals, and linoleic acid, which can be extracted and then re-utilised in various sectors (e.g. food, chemical, personal care, and pharmaceutical). Taking into account the above potential value of melon seed waste, upcycling of melon seed waste could provide financial benefits.
8. Future perspectives
Given the current advances in melon seed valorisation and applications, various perspectives are needed to be focused on future studies. As discussed in above sections, future studies could focus on the following aspects:
(i) Quality, microbiological, and contaminants assessment of melon seeds. Although current study has demonstrated the potential value of melon seeds, the quality and safety of melon seeds are still gaps. Therefore, to guarantee melon seeds' edibility and consumers' safety, more studies on the quality and safety of melon seeds are necessary, such as allergens, content of anti-nutritional compounds (e.g. phytic acid, tannins, and oxalate), microbiological and contaminant assessment. Apart from that, the application of processing technologies to improve the quality and safety of melon seeds and its related products is also must in this area of research in future.
(ii) Technological improvement; the validation and assessment of bioactive effects of extracted compounds. The comparable data between green extraction technologies and conventional extraction technologies on recovering value-added compounds of melon seeds are still lacking. Additionally, the data on bioactive effects of melon seed extracts by an in vivo study are still lacking. An appropriate extraction method is important, since it can determine the quality, safety, and yield of final products;101,103,105 in addition, novel green technologies can overcome the limitation of conventional technologies (e.g. extraction efficiency, sustainability, and safety) and are in line with sustainable development and green economy.83,106 Therefore, enormous studies are needed to identify the effect of these technologies on the biological properties (in vitro and in vivo) and quality of the target compounds, aiming to produce high-quality end products that can be used in various sectors.
(iii) Consumer acceptance and affordability; improvement of melon seed food quality. Consumer acceptance is an important determinant of the successful commercialization of either newly developed or reformulated products.129 Melon seeds are an uncommon ingredient in most of the countries, thus, melon seed food products could cause food neophobia for consumers.130 To this end, how to increase consumers' acceptance and affordability for melon seed food products is necessary in future studies, including designing and marketing new products, and improvement of melon seed food quality (e.g. flavour, texture, appearance, nutritional value, and nutrient bioavailability).
9. Conclusions
Melon seeds hold potential as a source of oil as well as other functional food ingredients (protein/peptides, polyphenols, minerals, and dietary fibre). Research on the valorisation of melon seeds should aim at maximising their utilisation, converting into value-added products, applicable in food or other sectors (e.g. pharmaceuticals and cosmetics), to reduce waste and promote sustainability. Moreover, one of the important concepts for the circular economy is valorisation of food waste to achieve economic growth by lowering the environmental burden approach. From this point of view, in order to better transform towards a circular economy, extraction methods should be further developed and evaluated to create a more eco-friendly and sustainable recycling process to produce diverse value-added products. Regarding melon seed applications in food, the main limitation is related to their quality and organoleptic aspects. Therefore, optimising intrinsic quality parameters influencing the incorporation of melon seeds or their components in foods is an important area of research which should be further pursued.
Data availability
No primary research results, software or code have been included and no new data were generated or analysed as part of this review.
Conflicts of interest
The authors report there are no competing interests to declare.
Acknowledgements
The authors reported there is no funding associated with the work featured in this article.
References
-
FAO, Global Initiative on Food Loss and Food Waste Reduction, United Nations, 2015 Search PubMed.
-
Ellen MacArthur Foundation, Cities and Circular Economy for Food, 2019 Search PubMed.
-
European Commission, A Farm to Fork Strategy for a fair, healthy and environmentally-friendly food system, 2020, https://eur-lex.europa.eu/legal-content/EN/TXT/?qid=1590404602495%26uri=CELEX:52020DC0381, accessed December 2, 2022.
-
European Commission, Closing the Loop – an EU Action Plan for the Circular Economy - (ANNEX 1), Communication from the Commission to the European Parliament, the Council, the Europena Economic and Social Committee and the Committee of the Regions, 2015 Search PubMed.
-
R. Ritchie and O.-O. Mispy, Measuring progress towards the Sustainable Development Goals, SDG-Tracker, 2018, https://sdg-tracker.org/sustainable-consumption-production, accessed August 11, 2024.
- D. A. Teigiserova, L. Hamelin and M. Thomsen, Review of high-value food waste and food residues biorefineries with focus on unavoidable wastes from processing, Resour., Conserv. Recycl., 2019, 149, 413–426 CrossRef.
- Q. Jin, L. Yang, N. Poe and H. Huang, Integrated processing of plant-derived waste to produce value-added products based on the biorefinery concept, Trends Food Sci. Technol., 2018, 74, 119–131 CrossRef CAS.
- A. Ekman, M. Campos, S. Lindahl, M. Co, P. Börjesson, E. N. Karlsson and C. Turner, Bioresource utilisation by sustainable technologies in new value-added biorefinery concepts - Two case studies from food and forest industry, J. Cleaner Prod., 2013, 57, 46–58 CrossRef CAS.
- C. S. K. Lin, L. A. Pfaltzgraff, L. Herrero-Davila, E. B. Mubofu, S. Abderrahim, J. H. Clark, A. A. Koutinas, N. Kopsahelis, K. Stamatelatou, F. Dickson, S. Thankappan, Z. Mohamed, R. Brocklesby and R. Luque, Food waste as a valuable resource for the production of chemicals, materials and fuels. Current situation and global perspective, Energy Environ. Sci., 2013, 6(2), 426–464 RSC.
- M. A. Silva, T. G. Albuquerque, R. C. Alves, M. B. P. P. Oliveira and H. S. Costa, Melon (Cucumis melo L.) by-products: Potential food ingredients for novel functional foods?, Trends Food Sci. Technol., 2020, 98, 181–189 CrossRef CAS.
- A. Frankowska, H. K. Jeswani and A. Azapagic, Life cycle environmental impacts of fruits consumption in the UK, J. Environ. Manage., 2019, 248, 109111 CrossRef PubMed.
- A. Rabadán, M. Antónia Nunes, S. M. F. Bessada, J. E. Pardo, M. P. P. Beatriz Oliveira and M. Álvarez-Ortí, From by-product to the food chain: Melon (Cucumis melo l.) seeds as potential source for oils, Foods, 2020, 9(10), 1341 CrossRef PubMed.
- J. F. Fundo, F. A. Miller, E. Garcia, J. R. Santos, C. L. M. Silva and T. R. S. Brandão, Physicochemical characteristics, bioactive compounds and antioxidant activity in juice, pulp, peel and seeds of Cantaloupe melon, J. Food Meas. Char., 2018, 12, 292–300 CrossRef.
- D. H. Wang, Z. Wang, K. P. Le, J. R. Cortright, H. G. Park, H. J. Tobias and J. T. Brenna, Potentially High Value Conjugated Linolenic Acids (CLnA) in Melon Seed Waste, J. Agric. Food Chem., 2019, 67(37), 10306–10312 CrossRef CAS PubMed.
- F. A. Miller, J. F. Fundo, E. Garcia, J. R. Santos, C. L. M. Silva and T. R. S. Brandão, Physicochemical and Bioactive Characterisation of Edible and Waste Parts of “Piel de Sapo” Melon, Horticulturae, 2020, 6(4), 60 CrossRef.
- L. L. D. R. Osorio, E. Flórez-López and C. D. Grande-Tovar, The Potential of Selected Agri-Food Loss and Waste to Contribute to a Circular Economy: Applications in the Food, Cosmetic and Pharmaceutical Industries, Molecules, 2021, 26(2), 515 CrossRef PubMed.
- B. Socas-Rodríguez, G. Álvarez-Rivera, A. Valdés, E. Ibáñez and A. Cifuentes, Food by-products and food wastes: are they safe enough for their valorization?, Trends Food Sci. Technol., 2021, 144, 133–147 CrossRef.
- J. Endl, E. G. Achigan-Dako, A. K. Pandey, A. J. Monforte, B. Pico and H. Schaefer, Repeated domestication of melon (Cucumis melo) in Africa and Asia and a new close relative from India, Am. J. Bot., 2018, 105(10), 1662–1671 CrossRef CAS PubMed.
- G. Laghetti, R. Accogli and K. Hammer, Different cucumber melon (Cucumis melo L.) races cultivated in Salento (Italy), Genet. Resour. Crop Evol., 2008, 55, 619–623 CrossRef.
- H. S. Paris, Z. Amar and E. Lev, Medieval emergence of sweet melons, Cucumis melo (Cucurbitaceae), Ann. Bot., 2012, 110(1), 23–33 CrossRef PubMed.
- H. Thakur, S. Sharma and M. Thakur, Recent trends in muskmelon (Cucumis melo L.) research: an overview, J. Hortic. Sci. Biotechnol., 2019, 94(4), 533–547 CrossRef CAS.
- J. Garcia-Mas, A. Benjak, W. Sanseverino, M. Bourgeois, G. Mir, V. M. Gonźalez, E. Heńaff, F. Câmara, L. Cozzuto, E. Lowy, T. Alioto, S. Capella-Gutieŕrez, J. Blancae, J. Cañizares, P. Ziarsolo, D. Gonzalez-Ibeas, L. Rodriǵuez-Moreno, M. Droege, L. Du, M. Alvarez-Tejado, B. Lorente-Galdos, M. Meleć, L. Yang, Y. Weng, A. Navarro, T. Marques-Bonet, M. A. Aranda, F. Nuez, B. Picó, T. Gabaldoń, G. Roma, R. Guigoć, J. M. Casacuberta, P. Aruś and P. Puigdomènech, The genome of melon (Cucumis melo L.), Proc. Natl. Acad. Sci. U. S. A., 2012, 109(29), 11872–11877 CrossRef CAS PubMed.
- G. Zhang, Z. Li and M. Fu, Comparison of quality and oxidative stability of pumpkin seed (Cucurbita maxima ) oil between conventional and enzymatic extraction methods, Sustainable Food Technol., 2024, 2, 1033–1040 RSC.
- M. Nee and J. H. Kirkbride, Biosystematic Monograph of the Genus Cucumis (Cucurbitaceae)-Botanical Identification of Cucumbers and Melons, Bull. Torrey Bot. Club, 1993, 121, 300–301 CrossRef.
-
Y. Burger, H. S. Paris, R. Cohen, N. Katzir, Y. Tadmor, E. Lewinsohn and A. A. Schaffer, Genetic Diversity of Cucumis Melo, in Hortic Rev, Am Soc Hortic Sci, 2010, vol. 36, pp. 165–198 Search PubMed.
-
M. Pitrat, Melon (Cucumis melo L.), in Hand-Book of Crop Breeding Vegetables, 2008, vol 1 Search PubMed.
- A. J. Monforte, A. Diaz, A. Caño-Delgado and E. Van Der Knaap, The genetic basis of fruit morphology in horticultural crops: Lessons from tomato and melon, J. Exp. Bot., 2014, 65(16), 4625–4637 CrossRef CAS PubMed.
- K. R. M. Swamy, Origin, distribution and systematics of culinary cucumber (Cucumis melo subsp. agrestis var. conomon), J. Hortic. Sci., 2017, 12(1), 1–22 CrossRef.
- A. Moing, J. William Allwood, A. Aharoni, J. Baker, M. H. Beale, S. Ben-Dor, B. Biais, F. Brigante, Y. Burger, C. Deborde, A. Erban, A. Faigenboim, A. Gur, R. Goodacre, T. H. Hansen, D. Jacob, N. Katzir, J. Kopka, E. Lewinsohn, M. Maucourt, S. Meir, S. Miller, R. Mumm, E. Oren, H. S. Paris, I. Rogachev, D. Rolin, U. Saar, J. K. Schjoerring, Y. Tadmor, G. Tzuri, R. C. H. de Vos, J. L. Ward, E. Yeselson, R. D. Hall and A. A. Schaffer, Comparative metabolomics and molecular phylogenetics of melon (Cucumis melo, Cucurbitaceae) biodiversity, Metabolites, 2020, 10(3), 121 CrossRef CAS PubMed.
- S. Mallek-Ayadi, N. Bahloul and N. Kechaou, Chemical composition and bioactive compounds of Cucumis melo L. seeds: Potential source for new trends of plant oils, Process Saf. Environ. Prot., 2018, 113, 68–77 CrossRef CAS.
- N. A. M. Yanty, O. M. Lai, A. Osman, K. Long and H. M. Ghazali, Physicochemical properties of cucumis melo var. inodorus (honeydew melon) seed and seed oil, J. Food Lipids, 2008, 15(1), 42–55 CrossRef CAS.
- S. Mallek-Ayadi, N. Bahloul and N. Kechaou, Phytochemical profile, nutraceutical potential and functional properties of Cucumis melo L. seeds, J. Sci. Food Agric., 2019, 99(3), 1294–1301 CrossRef CAS PubMed.
- Z. Petkova and G. Antova, Proximate composition of seeds and seed oils from melon (Cucumis melo L.) cultivated in Bulgaria, Cogent Food Agric., 2015, 1(1), 1018779 CrossRef.
- H. Mian-Hao and A. Yansong, Characteristics of some nutritional composition of melon (Cucumis melo hybrid ’ChunLi’) seeds, Int. J. Food Sci. Technol., 2007, 42(12), 1397–1401 CrossRef.
- K. Kotecka-Majchrzak, A. Sumara, E. Fornal and M. Montowska, Oilseed proteins – Properties and application as a food ingredient, Trends Food Sci. Technol., 2020, 106, 160–170 CrossRef CAS.
- J. Czubinski, J. Ruško and P. Górnaś, Japanese Quince Seeds as a Promising Rich Source of Proteins and Essential Amino Acids, Plant Foods Hum. Nutr., 2021, 76(4), 533–535 CrossRef CAS PubMed.
- E. F. Vieira, A. Q. Fontoura and C. Delerue-Matos, Chayote (Sechium edule (Jacq.) Swartz) Seed as an Unexploited Protein Source: Bio-Functional and Nutritional Quality of Protein Isolates, Foods, 2023, 12(15), 2949 CrossRef CAS PubMed.
- A. E. M. Abdalla, S. M. Darwish, E. H. E. Ayad and R. M. El-Hamahmy, Egyptian mango by-product 1. Compositional quality of mango seed kernel, Food Chem., 2007, 103(4), 1134–1140 CrossRef CAS.
- A. Siddeeg, Y. Xu, Q. Jiang and W. Xia,
In vitro antioxidant activity of protein fractions extracted from seinat (Cucumis melo var. tibish) seeds, CyTA–J. Food, 2015, 13(3), 472–481 CrossRef CAS.
- D. Pasrija and D. S. Sogi, Extraction optimization and functional properties of muskmelon seed protein concentrate, J. Food Meas. Char., 2022, 16(5), 4137–4150 CrossRef.
- M. L. S. De Mello, P. S. Bora and N. Narain, Fatty and Amino Acids Composition of Melon (Cucumis melo Var. saccharinus) Seeds, J. Food Compos. Anal., 2001, 14(1), 69–74 CrossRef CAS.
- T. Zhang, E. Guan, Y. Yang, F. Liu, L. Zhang, J. Pang and K. Bian, Fatty acid profiles of vegetable oils from four different plant sources and their effects on dough rheology and Chinese steamed bread quality, Int. J. Food Sci. Technol., 2021, 56(5), 2407–2414 CrossRef CAS.
- M. Hua, J. Lu, D. Qu, C. Liu, L. Zhang, S. Li, J. Chen and Y. Sun, Structure, physicochemical properties and adsorption function of insoluble dietary fiber from ginseng residue: A potential functional ingredient, Food Chem., 2019, 286, 522–529 CrossRef CAS PubMed.
- J. Cao, K. Wang, N. Li, L. Zhang, L. Qin, Y. He, J. Wang, C. Qu and J. Miao, Soluble dietary fiber and cellulose from Saccharina japonica by-product ameliorate Loperamide-induced constipation via modulating enteric neurotransmitters, short-chain fatty acids and gut microbiota, Int. J. Biol. Macromol., 2023, 226, 1319–1331 CrossRef CAS PubMed.
- H. Liu, X. Zeng, J. Huang, X. Yuan, Q. Wang and L. Ma, Dietary fiber extracted from pomelo fruitlets promotes intestinal functions, both in vitro and in vivo, Carbohydr. Polym., 2021, 252, 117186 CrossRef CAS PubMed.
- C. B. Breneman and L. Tucker, Dietary fibre consumption and insulin resistance – the role of body fat and physical activity, Br. J. Nutr., 2013, 110(2), 375–383 CrossRef CAS PubMed.
- X. Tang, Z. Wang, J. Zheng, J. Kan, G. Chen and M. Du, Physicochemical, structure properties and in vitro hypoglycemic activity of soluble dietary fiber from adlay (Coix lachryma-jobi L. var. ma-yuen Stapf) bran treated by steam explosion, Front. Nutr., 2023, 10, 1124012 CrossRef PubMed.
- G. Zhang, Z. Li, A. Chatzifragkou and D. Charalampopoulos, Effect of processing methods on the phytochemical content of melon seeds (Cucumis melo L.), Future Foods, 2024, 100399 CrossRef CAS.
- WHO, WHO issues new guidance on dietary salt and potassium, Cent. Eur. J. Public Health, 2013, 21, 16 Search PubMed.
- K. Cepanec, S. Vugrinec, T. Cvetković and J. Ranilović, Potassium Chloride-Based Salt Substitutes: A Critical Review with a Focus on the Patent Literature, Compr. Rev. Food Sci. Food Saf., 2017, 16(5), 881–894 CrossRef CAS PubMed.
- S. Du, H. Wang, B. Zhang and B. M. Popkin, Dietary potassium intake remains low and sodium intake remains high, and most sodium is derived from home food preparation for chinese adults, 1991–2015 trends, J. Nutr., 2020, 150(5), 1230–1239 CrossRef PubMed.
- Y. Srivastava, Effect of Virgin Coconut Meal (VCM) on the Textural, Thermal and Physico Chemical Properties of Biscuits, Food Nutr. Sci., 2010, 1(2), 38–44 CAS.
- J. M. Sanz-Penella, M. Wronkowska, M. Soral-Smietana and M. Haros, Effect of whole amaranth flour on bread properties and nutritive value, LWT–Food Sci. Technol., 2013, 50(2), 679–685 CrossRef CAS.
- C. A. Titchenal and J. Dobbs, A system to assess the quality of food sources of calcium, J. Food Compos. Anal., 2007, 20(8), 717–724 CrossRef CAS.
- B. Pérez-Vich, L. Velasco and J. M. Fernández-Martínez, Determination of seed oil content and fatty acid composition in sunflower through the analysis of intact seeds, husked seeds, meal and oil by near-infrared reflectance spectroscopy, J. Am. Oil Chem. Soc., 1998, 75(5), 547–555 CrossRef.
- M. L. Wang, P. Raymer, M. Chinnan and R. N. Pittman, Screening of the USDA peanut germplasm for oil content and fatty acid composition, Biomass Bioenergy, 2012, 39, 336–343 CrossRef CAS.
- J. Medic, C. Atkinson and C. R. Hurburgh, Current knowledge in soybean composition, J. Am. Oil Chem. Soc., 2014, 91, 363–384 CrossRef CAS.
- F. Zanetti, A. Monti and M. T. Berti, Challenges and opportunities for new industrial oilseed crops in EU-27: A review, Ind. Crops Prod., 2013, 50, 580–595 CrossRef CAS.
- A. C. da Silva and N. Jorge, Bioactive compounds of oils extracted from fruits seeds obtained from agroindustrial waste, Eur. J. Lipid Sci. Technol., 2017, 119(4), 1600024 CrossRef.
- L. Rezig, M. Chouaibi, W. Meddeb, K. Msaada and S. Hamdi, Chemical composition and bioactive compounds of Cucurbitaceae seeds: Potential sources for new trends of plant oils, Process Saf. Environ. Prot., 2019, 127, 73–81 CrossRef CAS.
- N. Bouazzaoui and J. K. Mulengi, Fatty acids and mineral composition of melon (Cucumis Melo) and pumpkin (Cucurbita moschata) seeds, J. Herbs, Spices Med. Plants, 2018, 24(4), 315–322 CrossRef.
- M. L. S. De Melo, N. Narain and P. S. Bora, Characterisation of some nutritional constituents of melon (Cucumis melo hybrid AF-522) seeds, Food Chem., 2000, 68(4), 411–414 CrossRef CAS.
- J. Orsavova, L. Misurcova, J. Ambrozova, R. Vicha and J. Mlcek, Fatty Acids Composition of Vegetable Oils and Its Contribution to Dietary Energy Intake and Dependence of Cardiovascular Mortality on Dietary Intake of Fatty Acids, Int. J. Mol. Sci., 2015, 16(6), 12871–12890 CrossRef CAS PubMed.
- F. Marangoni, C. Agostoni, C. Borghi, A. L. Catapano, H. Cena, A. Ghiselli, C. La Vecchia, G. Lercker, E. Manzato, A. Pirillo, G. Riccardi, P. Risé, F. Visioli and A. Poli, Dietary linoleic acid and human health: Focus
on cardiovascular and cardiometabolic effects, Atherosclerosis, 2020, 292, 90–98 CrossRef CAS PubMed.
- A. L. Fontes, L. L. Pimentel, C. D. Simões, A. M. P. Gomes and L. M. Rodríguez-Alcalá, Evidences and perspectives in the utilization of CLNA isomers as bioactive compounds in foods, Crit. Rev. Food Sci. Nutr., 2017, 57(12), 2611–2622 CrossRef CAS PubMed.
- K. K. Dhar Dubey, G. Sharma and A. Kumar, Conjugated Linolenic Acids: Implication in Cancer, J. Agric. Food Chem., 2019, 67(22), 6091–6101 CrossRef CAS PubMed.
- Z. He, H. Zhu, W. Li, M. Zeng, S. Wu, S. Chen, F. Qin and J. Chen, Chemical components of cold pressed kernel oils from different Torreya grandis cultivars, Food Chem., 2016, 209, 196–202 CrossRef CAS PubMed.
- Y. Huang, C. Liu, Z. Ge, F. Huang, H. Tang, Q. Zhou, R. Liu, J. Huang and C. Zheng, Influence of different thermal treatment methods on the processing qualities of sesame seeds and cold-pressed oil, Food Chem., 2023, 404, 134683 CrossRef CAS PubMed.
- I. A. Nehdi, H. Sbihi, C. P. Tan and S. I. Al-Resayes, Evaluation and characterisation of Citrullus colocynthis (L.) Schrad seed oil: Comparison with Helianthus annuus (sunflower) seed oil, Food Chem., 2013, 136(2), 348–353 CrossRef CAS PubMed.
- P. S. Bora, N. Narain and M. L. S. de MeIIo, Characterization of the seed oils of some commercial cultivars of melon, Eur. J. Lipid Sci. Technol., 2000, 102(4), 266–269 CrossRef CAS.
- K. L. Nyam, C. P. Tan, O. M. Lai, K. Long and Y. B. Che Man, Physicochemical properties and bioactive compounds of selected seed oils, LWT–Food Sci. Technol., 2009, 42(8), 1396–1403 CrossRef CAS.
- H. Zhang, Y. Yuan, X. Zhu, R. Xu, H. Shen, Q. Zhang and X. Ge, The Effect of Different Extraction Methods on Extraction Yield, Physicochemical Properties, and Volatile Compounds from Field Muskmelon Seed Oil, Foods, 2022, 11(5), 721 CrossRef CAS PubMed.
- S. H. Manohar and H. N. Murthy, Fatty acid profile of Cucumis melo var. acidulus (culinary melon) seed oil, J. Am. Oil Chem. Soc., 2014, 91(5), 815–816 CrossRef CAS.
- L. Rezig, M. Chouaibi, K. Msaada and S. Hamdi, Chemical composition and profile characterisation of pumpkin (Cucurbita maxima) seed oil, Ind. Crops Prod., 2012, 37(1), 82–87 CrossRef CAS.
- G. Kaur, D. Kaur, S. K. Kansal, M. Garg and M. Krishania, Potential cocoa butter substitute derived from mango seed kernel, Food Chem., 2022, 372, 131244 CrossRef CAS PubMed.
- N. Shahzad, W. Khan, S. MD, A. Ali, S. S. Saluja, S. Sharma, F. A. Al-Allaf, Z. Abduljaleel, I. A. A. Ibrahim, A. F. Abdel-Wahab, M. A. Afify and S. S. Al-Ghamdi, Phytosterols as a natural anticancer agent: Current status and future perspective, Biomed. Pharmacother., 2017, 88, 786–794 CrossRef CAS PubMed.
- S. Jew, J. M. Antoine, P. Bourlioux, J. Milner, L. C. Tapsell, Y. Yang and P. J. H. Jones, Nutrient essentiality revisited, J. Funct. Foods, 2015, 14, 203–209 CrossRef CAS.
- A. C. da Silva and N. Jorge, Bioactive compounds of the lipid fractions of agro-industrial waste, Food Res. Int., 2014, 66, 493–500 CrossRef CAS.
- F. Fine, C. Brochet, M. Gaud, P. Carre, N. Simon, F. Ramli and F. Joffre, Micronutrients in vegetable oils: The impact of crushing and refining processes on vitamins and antioxidants in sunflower, rapeseed, and soybean oils, Eur. J. Lipid Sci. Technol., 2016, 118(5), 680–697 CrossRef CAS.
- P. Górnaś, A. Soliven and D. Segliņa, Seed oils recovered from industrial fruit by-products are a rich source of tocopherols and tocotrienols: Rapid separation of α/β/γ/δ homologues by RP-HPLC/FLD, Eur. J. Lipid Sci. Technol., 2015, 117(6), 773–777 CrossRef.
- E. Choe and D. B. Min, Mechanisms and factors for edible oil oxidation, Compr. Rev. Food Sci. Food Saf., 2006, 5(4), 169–186 CrossRef CAS.
- S. M. B. Hashemi, A. Mousavi Khaneghah, M. Koubaa, J. Lopez-Cervantes, S. H. A. Yousefabad, S. F. Hosseini, M. Karimi, A. Motazedian and S. Asadifard, Novel edible oil sources: Microwave heating and chemical properties, Food Res. Int., 2017, 92, 147–153 CrossRef CAS PubMed.
- P. R. More, A. R. Jambrak and S. S. Arya, Green, environment-friendly and sustainable techniques for extraction of food bioactive compounds and waste valorization, Trends Food Sci. Technol., 2022, 128, 296–315 CrossRef CAS.
- F. Garavand, S. Rahaee, N. Vahedikia and S. M. Jafari, Different techniques for extraction and micro/nanoencapsulation of saffron bioactive ingredients, Trends Food Sci. Technol., 2019, 89, 26–44 CrossRef CAS.
- K. Wu, T. Ju, Y. Deng and J. Xi, Mechanochemical assisted extraction: A novel, efficient, eco-friendly technology, Trends Food Sci. Technol., 2017, 66, 166–175 CrossRef CAS.
- J. AlYammahi, K. Rambabu, A. Thanigaivelan, G. Bharath, S. W. Hasan, P. L. Show and F. Banat, Advances of non-conventional green technologies for phyto-saccharides extraction: current status and future perspectives, Phytochem. Rev., 2023, 22(4), 1067–1088 CrossRef CAS.
- K. Ameer, H. M. Shahbaz and J. Kwon, Green Extraction Methods for Polyphenols from Plant Matrices and Their Byproducts: A Review, Compr. Rev. Food Sci. Food Saf., 2017, 16(2), 295–315 CrossRef PubMed.
- C. H. Geow, M. C. Tan, S. P. Yeap and N. L. Chin, A Review on Extraction Techniques and Its Future Applications in Industry, Eur. J. Lipid Sci. Technol., 2021, 123(4), 2000302 CrossRef CAS.
- O. Gligor, A. Mocan, C. Moldovan, M. Locatelli, G. Crişan and I. C. F. R. Ferreira, Enzyme-assisted extractions of polyphenols – A comprehensive review, Trends Food Sci. Technol., 2019, 88, 302–315 CrossRef CAS.
- C. M. G. C. Renard, Extraction of bioactives from fruit and vegetables: State of the art and perspectives, LWT, 2018, 93, 390–395 CrossRef CAS.
- E. M. C. Alexandre, S. A. Moreira, L. M. G. Castro, M. Pintado and J. A. Saraiva, Emerging technologies to extract high added value compounds from fruit residues: Sub/supercritical, ultrasound-, and enzyme-assisted extractions, Food Rev. Int., 2018, 34(6), 581–612 CrossRef CAS.
- A. K. Jha and N. Sit, Extraction of bioactive compounds from plant materials using combination of various novel methods: A review, Trends Food Sci. Technol., 2022, 119, 579–591 CrossRef CAS.
- F. Chemat, M. Abert Vian, A. S. Fabiano-Tixier, M. Nutrizio, A. Režek Jambrak, P. E. S. Munekata, J. M. Lorenzo, F. J. Barba, A. Binello and G. Cravotto, A review of sustainable and intensified techniques for extraction of food and natural products, Green Chem., 2020, 22(8), 2325–2353 RSC.
- A. Rai, B. Mohanty and R. Bhargava, Fitting of broken and intact cell model to supercritical fluid extraction (SFE) of sunflower oil, Innovative Food Sci. Emerging Technol., 2016, 38, 32–40 CrossRef CAS.
- K. M. Sharif, M. M. Rahman, J. Azmir, A. Mohamed, M. H. A. Jahurul, F. Sahena and I. S. M. Zaidul, Experimental design of supercritical fluid extraction - A review, J. Food Eng., 2014, 124, 105–116 CrossRef CAS.
- N. A. Sagar, S. Pareek, S. Sharma, E. M. Yahia and M. G. Lobo, Fruit and Vegetable Waste: Bioactive Compounds, Their Extraction, and Possible Utilization, Compr. Rev. Food Sci. Food Saf., 2018, 17(3), 512–531 CrossRef CAS PubMed.
- M. Koubaa, E. Roselló-Soto, J. Šic Žlabur, A. Režek Jambrak, M. Brnčić, N. Grimi, N. Boussetta and F. J. Barba, Current and New Insights in the Sustainable and Green Recovery of Nutritionally Valuable Compounds from Stevia rebaudiana Bertoni, J. Agric. Food Chem., 2015, 63(31), 6835–6846 CrossRef CAS PubMed.
- M. d. A. Lima, R. Andreou, D. Charalampopoulos and A. Chatzifragkou, Supercritical carbon dioxide extraction of phenolic compounds from potato (Solanum tuberosum) peels, Appl. Sci., 2021, 11(8), 3410 CrossRef CAS.
- J. P. Maran and B. Priya, Supercritical fluid extraction of oil from muskmelon (Cucumis melo) seeds, J. Taiwan Inst. Chem. Eng., 2015, 47, 71–78 CrossRef CAS.
- M. S. Ekinci and M. Gürü, Extraction of phytosterols from melon (Cucumis melo) seeds by supercritical CO2 as a clean technology, Green Process. Synth., 2019, 8(1), 677–682 CAS.
- L. Wen, Z. Zhang, D. W. Sun, S. P. Sivagnanam and B. K. Tiwari, Combination of emerging technologies for the extraction of bioactive compounds, Crit. Rev. Food Sci. Nutr., 2020, 60(11), 1826–1841 CrossRef CAS PubMed.
- M. Marić, A. N. Grassino, Z. Zhu, F. J. Barba, M. Brnčić and S. Rimac Brnčić, An overview of the traditional and innovative approaches for pectin extraction from plant food wastes and by-products: Ultrasound-, microwaves-, and enzyme-assisted extraction, Trends Food Sci. Technol., 2018, 76, 28–37 CrossRef.
- C. Tang, L. Wu, F. Zhang, J. Kan and J. Zheng, Comparison of different extraction methods on the physicochemical, structural properties, and in vitro hypoglycemic activity of bamboo shoot dietary fibers, Food Chem., 2022, 386, 132642 CrossRef CAS PubMed.
- S. A. O. Ribeiro, A. E. Nicacio, A. B. Zanqui, P. B. F. Biondo, B. A. de Abreu-Filho, J. V. Visentainer, S. T. M. Gomes and M. Matsushita, Improvements in the quality of sesame oil obtained by a green extraction method using enzymes, LWT, 2016, 65, 464–470 CrossRef CAS.
- G. Zhang, Z. Li, Z. Guo and D. Charalampopoulos, Comparative extraction of melon seed (Cucumis melo L.) oil by conventional and enzymatic methods: Physicochemical properties and oxidative stability, J. Agric. Food Res., 2024, 16, 101182 CAS.
- P. R. More, A. R. Jambrak and S. S. Arya, Green, environment-friendly and sustainable techniques for extraction of food bioactive compounds and waste valorization, Trends Food Sci. Technol., 2022, 128, 296–315 CrossRef CAS.
- P. W. Mwaurah, S. Kumar, N. Kumar, A. K. Attkan, A. Panghal, V. K. Singh and M. K. Garg, Novel oil extraction technologies: Process conditions, quality parameters, and optimization, Compr. Rev. Food Sci. Food Saf., 2020, 19(1), 3–20 CrossRef CAS PubMed.
- F. Bruno Siewe, T. G. Kudre and B. Narayan, Optimisation of ultrasound-assisted enzymatic extraction conditions of umami compounds from fish by-products using the combination of fractional factorial design and central composite design, Food Chem., 2021, 334, 127498 CrossRef CAS PubMed.
- M. Á. Rivas, M. J. Benito, A. Martín, M. d. G. Córdoba, S. Ruíz-Moyano and R. Casquete, Improve the functional properties of dietary fibre isolated from broccoli by-products by using different technologies, Innovative Food Sci. Emerging Technol., 2022, 80, 103075 CrossRef CAS.
- I. Piasecka, R. Brzezińska, E. Ostrowska-Ligęza, A. Wiktor and A. Górska, Ultrasound-assisted extraction of cranberry seed oil: food waste valorization approach, Eur. Food Res. Technol., 2023, 249(11), 2763–2775 CrossRef CAS.
- A. Kumar T., S. Pareek, R. Kaur, N. A. Sagar, L. Singh, R. Sami, N. I. Aljuraide, A. Elhakem, Z. D. Alsharari, R. S. Alruwais, M. D. Aljabri and M. M. Rahman, Optimization of Ultrasonic-Assisted Enzymatic Extraction of Freeze-Dried Sea Buckthorn
(Hippophae rhamnoides L.) Berry Oil Using Response Surface Methodology, Sustainability, 2022, 14(17), 10849 CrossRef CAS.
- Y. Gao, Q. Dong, S. Zhao, Y. Zhao, Y. Zhang, H. Wang, Y. Wang, W. Wang, L. Wang and H. Wang, Efficient ultrasound-assisted enzymatic method for extraction of immunostimulant QS-21 from Quillaja saponaria Molina, Ind. Crops Prod., 2022, 189, 115807 CrossRef CAS.
- S. Karakaya, A. Kavas, S. N. El, N. Gündüç and L. Akdoǧan, Nutritive value of a melon seed beverage, Food Chem., 1995, 52(2), 139–141 CrossRef CAS.
- A. Zungur Bastıoğlu, D. Tomruk, M. Koç and F. K. Ertekin, Spray dried melon seed milk powder: physical, rheological and sensory properties, J. Food Sci. Technol., 2016, 53, 2396–2404 CrossRef PubMed.
- J. A. da Cunha, P. M. Rolim, K. S. F. da Silva Chaves Damasceno, F. C. de Sousa, R. C. Nabas and L. M. A. J. Seabra, From seed to flour: Sowing sustainability in the use of cantaloupe melon residue (Cucumis melo L. Var. Reticulatus), PLoS One, 2020, 15(1), e0219229 CrossRef PubMed.
- L. Tarjuelo, J. E. Pardo, M. Álvarez-Ortí, A. Pardo-Giménez, C. Millán and A. Rabadán, Development of Seed-Oil Based Dried Sausages, Considering Physicochemical and Nutritional Quality and the Role of Food Neophobia, Nutrients, 2022, 14(15), 3106, DOI:10.3390/nu14153106.
- G. Zhang, A. Chatzifragkou, D. Charalampopoulos and J. Rodriguez-Garcia, Effect of defatted melon seed residue on dough development and bread quality, LWT, 2023, 183, 114892 CrossRef CAS.
- G. Zhang and Z. Li, Impact of melon seed oil cake with different particle sizes on bread quality, Food Prod., Process. Nutr., 2024, 6(1), 44 CrossRef CAS.
- M. Ayuso, M. Carpena, O. Taofiq, T. G. Albuquerque, J. Simal-Gandara, M. B. P. P. Oliveira, M. A. Prieto, I. C. F. R. Ferreira and L. Barros, Fig “Ficus carica L.” and its by-products: A decade evidence of their health-promoting benefits towards the development of novel food formulations, Trends Food Sci. Technol., 2022, 127, 1–13 CrossRef CAS.
- L. Chen and Y.-H. Kang, In vitro inhibitory effect of oriental melon (Cucumis melo L. var. makuwa Makino) seed on key enzyme linked to type 2 diabetes, J. Funct. Foods, 2013, 5(2), 981–986 CrossRef CAS.
- L. Chen, Y. H. Kang and J. K. Suh, Roasting processed oriental melon (Cucumis melo L. var. makuwa Makino) seed influenced the triglyceride profile and the inhibitory potential against key enzymes relevant for hyperglycemia, Food Res. Int., 2014, 56, 236–242 CrossRef CAS.
- P. M. Rolim, G. P. Fidelis, C. E. A. Padilha, E. S. Santos, H. A. O. Rocha and G. R. Macedo, Phenolic profile and antioxidant activity from peels and seeds of melon (Cucumis melo L. var. reticulatus) and their antiproliferative effect in cancer cells, Braz. J. Med. Biol. Res., 2018, 51, e6069 CrossRef CAS PubMed.
- W. Hao, H. Zhu, J. Chen, E. Kwek, Z. He, J. Liu, N. Ma, K. Y. Ma and Z. Y. Chen, Wild Melon Seed Oil Reduces Plasma Cholesterol and Modulates Gut Microbiota in Hypercholesterolemic Hamsters, J. Agric. Food Chem., 2020, 68(7), 2071–2081 CrossRef CAS PubMed.
- H. Rasouli, S. Parvaneh, A. Mahnam, M. Rastegari-Pouyani, Z. Hoseinkhani and K. Mansouri, Anti-angiogenic potential of trypsin inhibitor purified from Cucumis melo seeds: Homology modeling and molecular docking perspective, Int. J. Biol. Macromol., 2017, 96, 118–128 CrossRef CAS PubMed.
- X. Zhang, Y. Bai, Y. Wang, C. Wang, J. Fu, L. Gao, Y. Liu, J. Feng, M. K. Swamy, M. Yogi, G. R. Rudramurthy, B. Purushotham and Y. Deng, Anticancer Properties of Different Solvent Extracts of Cucumis melo L. Seeds and Whole
Fruit and Their Metabolite Profiling Using HPLC and GC-MS, BioMed Res. Int., 2020, 2020(1), 5282949 Search PubMed.
- B. Rakesh and R. Mahendran, Upcycling of food waste and food loss – A sustainable approach in the food sector, Trends Food Sci. Technol., 2024, 143, 104274 CrossRef CAS.
- S. Punia Bangar, V. Chaudhary, P. Kajla, G. Balakrishnan and Y. Phimolsiripol, Strategies for upcycling food waste in the food production and supply chain, Trends Food Sci. Technol., 2024, 143, 104314 CrossRef CAS.
- M. Al-Obadi, H. Ayad, S. Pokharel and M. A. Ayari, Perspectives on food waste management: Prevention and social innovations, Sustain. Prod. Consum., 2022, 31, 190–208 CrossRef.
- Q. Yang, Y. Shen, T. Foster and J. Hort, Measuring consumer emotional response and acceptance to sustainable food products, Food Res. Int., 2020, 131, 108992 CrossRef PubMed.
- A. A. Faria and J. Kang, It's not just about the food: Motivators of food patterns and their link with sustainable food neophobia, Appetite, 2022, 174, 106008 CrossRef PubMed.
|
This journal is © The Royal Society of Chemistry 2024 |
Click here to see how this site uses Cookies. View our privacy policy here.