Prospects of using non-thermal technologies for chia seed mucilage extraction
Received
6th March 2024
, Accepted 4th June 2024
First published on 5th June 2024
Abstract
Chia seed mucilage (CSM) possesses a range of functional and bio-functional properties, making it a valuable hydrocolloid. The CSM extraction process includes stages such as hydration, separation, recovery, and purification of polysaccharides. Traditional methods using strong bases or acids and high temperatures can damage CSM and demucilaged seeds, which are useful for oil extraction. To address this issue, non-thermal technologies have shown significant promise as an environmentally friendly extraction method. Based on the existing literature, this review focuses on non-thermal techniques such as ultrasonication, cold plasma, microwave, and pulsed electric field, for potential future use. It highlights the benefits of non-thermal technologies on the physical and functional properties of CSM. The review emphasises the potential of CSM as a novel food hydrocolloid across various industries, including its use in pharmaceuticals and cosmetics, emphasising sustainable extraction and application.
Sustainability spotlight
This is the first review discussing the prospects of non-thermal technologies for chia seed mucilage (CSM) extraction with a focus on the application of improved approaches of mucilage extraction over traditional methods. This will provide an understanding for researchers and industrialists to opt for a better technique to extract a novel food hydrocolloid which is having a positive impact on food attributes. This work emphasises the utilization of sustainable technologies without the use of chemicals and thermal treatments to extract CSM with minimal loss of nutritional qualities. This will provide opportunities to produce novel food ingredients, processing, and packaging-oriented use of chia seed.
|
1 Introduction
The increasing popularity of vegan and vegetarian diets is linked to changing dietary preferences and increased interest in plant-based alternatives to animal-derived products. These alternatives are preferred due to their health benefits, such as a rich content of essential amino acids and a lipid profile which is high in polyunsaturated fatty acids, and their greater environmental sustainability. Consequently, there is a growing demand for plant-based foods, emphasising the importance of identifying new plant-based ingredients for application in food and beverage products. This trend reflects a growing inclination towards plant-based food products, underscoring the necessity to explore and develop novel plant-derived ingredients suitable for the food and beverage industry.1
The need for plant-based alternatives to food hydrocolloids is growing. Food hydrocolloids, which enhance the functional properties of food, are traditionally sourced from seeds (locust bean gum, guar gum, and tara gum), plants (pectins, starch, and cellulose), microbial sources (gellan gum and xanthan gum), and seaweeds (carrageenan, agar, and alginate). Additionally, plant seed exudate, gums, or mucilage (gum karaya, gum arabic, and tragacanth) are used as hydrocolloids, often after purification with enzymes or chemicals.2 Recent studies have focused on mucilage from basil seeds,3,4 flaxseed,5 tamarind,6 quince seed,7 and chia seeds,8–10 analysing their effectiveness as stabilizers, fat replacers, or hydrocolloids in various food products, particularly suitable for people with dysphagia.
Plant seed mucilage (PSM), recognised as an eco-friendly, edible, and sustainable natural polysaccharide hydrocolloid, enhances food products by providing stabilization, texture, and structure.11–13 Research indicates that it not only improves the nutritional & functional properties of food formulation13,14 but is also cost-effective, biocompatible, biodegradable, and non-toxic making it a preferable alternative to synthetic thickeners in industrial applications.15,16 Additionally, seed mucilage serves as a carrier for various lipophilic and hydrophilic compounds such as essential oils, vitamins, probiotics, antimicrobial agents, and antioxidants. This delivery mechanism enhances these encapsulated molecules' bioavailability, bioactivity and stability, thereby improving the overall quality of food products.17
Numerous studies in the literature have detailed recent developments in the extraction, composition, functional and structural properties, and thermal properties of plant seed mucilage, highlighting its effectiveness as a hydrocolloid. For instance, Lira et al.1 reviewed the composition, extraction methods, and main uses of mucilage derived from flaxseed, chia seeds, and Barbados gooseberry. Similarly, Goksen et al.14 discussed polysaccharides in mucilage and their applications in the food industry, including in bakery and meat products, fermented dairy products, and biomedical and tissue engineering applications. Yang et al.18 focused on mucilage's potential as an emulsifier substitute. Cakmak et al.12 conducted a systematic review of various plant seed mucilage (PSM) extraction methods and their functional properties, supporting the shift toward plant-based food hydrocolloids. They also verified the use of PSM in edible films, coating materials, and encapsulating materials for protecting bioactive compounds and documented its biological properties as an antioxidant, antidiabetic, and prebiotic.12 The growing interest in natural ingredients is evident, with chia seeds being recognised as a mucilage source with yields of up to 15%19 depending on factors such as the extraction method, pH, the temperature of the extraction solvent, the seed-to-water ratio, seed origin and genotype, and mechanical stirring.11,20,21
Chia seeds (CSs) hold significant nutritional and therapeutic value in the human diet22 and are well-known for their soothing effects in skin care products derived from CSM.23 Chemically, chia seeds are a rich source of proteins (15–25%), oil (30–33%), carbohydrates (26–41%), minerals (4–5%), and dietary fibre (18–30%),24 surpassing the nutrient content of whole flours from quinoa, amaranth, and wheat.25 They contain mucilage, a heteropolysaccharide26 that expands around the seed upon contact with water.27 Numerous studies on CSM extraction have proved its effectiveness as a vegan thickener28 and highlighted its potential to revolutionise the hydrocolloid industry due to its scalable functional properties for use in food industries.
Non-thermal technologies offer significant advantages over thermal processing in conserving the flavours and nutrients of food products.29 These techniques are effective in altering the properties of macromolecules. Recent reviews from across the world have provided evidence supporting these technologies. Radhakrishnan et al.30 highlighted the benefits of emerging non-thermal technologies regarding food safety, sustainability, and quality maintenance, including their ability to operate without gas emissions and with lower water and energy use. On the other hand, López-Gámez et al.31 discussed how non-thermal technologies affect bioactive compounds and bio-accessibility in fruits and vegetables. According to Farahnaky et al.,32 the growing research in non-thermal technologies is driven by consumer demand for minimally processed foods with fresh flavour, delicate texture, and improved nutritional value. The adoption of these technologies in the food industry is expected to accelerate in the coming decade. Jadhav et al.33 described non-thermal technologies as safe, environment-friendly options for food processing, underlining their role in advancing the field. Jeevitha et al.34 noted that these technologies can preserve organic acids and bioactive compounds (anthocyanin, antioxidants, flavonoids and β-carotene), though they emphasised the need for industrial-scale trials, as most research is still at the laboratory scale.
This review is the first to discuss the use of non-thermal technologies for extracting CSM and their impact on its techno-functional properties. It also covers the mechanisms of CSM extraction and offers future perspectives on selecting appropriate technologies for large-scale CSM production. This review aims to update academics, students, and industry professionals on the latest trends in CSM modification and extraction methods.
2 Chia seed mucilage and its composition
Upon hydrating with water (Fig. 1), chia seeds develop a “mucilaginous gel (polysaccharide)” layer on their outer skin.15,35 This mucilage consists of water-soluble sugars and uronic acid linked by glycosidic bonds.36 The composition of chia seed mucilage includes a variety of sugars, including mannose, glucose, xylose, arabinose, galacturonic acid, glucuronic acid,37 and galactose.38 Mucilage is a natural “polysaccharide hydrocolloid” with a distinctive structural conformation and a wide range of physicochemical properties, endowed with diverse functional and health-related properties.11 Incorporating plant seed mucilage into food products has enhanced insulinemic and postprandial glycaemic responses, promoted satiety, mitigated hyperlipidaemia, and influenced gut microbe function.39
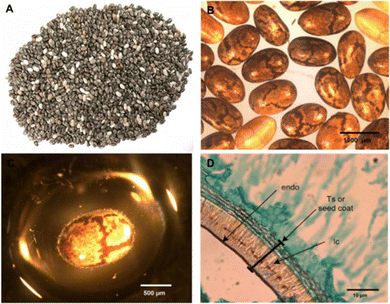 |
| Fig. 1 Chia seed. (A) Seeds, (B) whole dry seed, (C) chia seed with mucilage and (D) seed layers, endo: endocarp layer; lc: sclereid layer (adapted from Muñoz et al.27). | |
Chia seed mucilage (CSM) is a water-soluble fraction of dietary fibre derived from chia seeds. Its composition varies, typically containing 0.52–5.17 (%) fat, 48–83 (%) carbohydrates, and 4.3–25 (%) proteins.15,27,40,41 However, these values are not static and may vary depending on several factors. For instance, the extraction method, such as cold and hot processes, significantly influences CSM's composition. The composition is also affected by the key steps involved in its extraction such as hydration, extraction, and recovery.42 Additionally, the form of CSM, whether purified, semi-purified, or non-purified, plays a crucial role in its composition and techno-functional properties. Purified CSM lacks proteins, fat, and other components, whereas semi-purified CSM involves alcohol precipitation, and crude CSM is unrefined mucilage. The detailed composition of these forms of CSM (purified, semi-purified, and crude CSM) on a dry weight basis, the composition of CSM (semi-purified and non-purified) on a wet basis, along with their monosaccharide content as well as mineral profile, molecular weight, and colour characteristics, are presented in Tables 1–4 respectively. The names of CSM in these tables were taken from the reference article without making further changes.
Table 1 Composition of CSM (purified, semi-purified, and non-purified) on a dry basisa
Component (%) |
Purified gum |
Purified (free from proteins) |
Semi-purified |
Non-purified mucilage |
Chia seed gum43,44 |
CSM (heat and ultrasound)45 |
CSG46 |
Chia seed polysaccharide35 |
CSM47 |
CSM15 |
CSM48 |
Chia seed fatted gum49 |
Chia flour gel50 |
Chia seed gel50 |
CSM (HE)51 |
CSM(CE)51 |
Purified chia seed gum:43,44 data expressed on a dry basis except for moisture. CSM (heat and ultrasound):45 data expressed on a dry basis. CSM*: carbohydrate derived based on the difference. CSM35 #: presented in the reference articles as total NSP (non-starch polysaccharide). CSM:51 HE and CE represent hot extraction and mucilage cold extraction, respectively.
|
Moisture |
3.9 ± 0.3 |
7.03 ± 0.08 |
— |
— |
7.24 ± 0.55 |
— |
11.5 ± 0.3 |
9.32 |
— |
— |
12.3 |
3.7 |
Crude fat |
0.6 ± 0.1 |
1.22 ± 0.09 |
2.1 |
— |
1.68 ± 0.02 |
1.78–5.17 |
3.1 ± 0.2 |
26.24 |
— |
— |
4.96 |
3.35 |
Total carbohydrates |
93.8 ± 0.5 |
90.38 ± 0.13 |
78.3 |
95.0 ± 1.5# |
71.31 ± 2.3 |
66.83–78.90* |
63.7 ± 0.5* |
— |
— |
— |
47.68 |
73.48 |
Total protein |
2.6 ± 0.2 |
0.72 ± 0.08 |
6.5 |
3.8 ± 0.2 |
9.54 ± 1.48 |
16.15–25.20 |
11.2 ± 0.3 |
25.07 |
19.44 ± 0.19 |
3.54 ± 0.15 |
21.12 |
6.98 |
Total ash |
0.8 ± 0.1 |
7.68 ± 0.06 |
3.2 |
— |
8.62 ± 0.69 |
2.57–4.27 |
8.4 ± 0.1 |
5.48 |
8.61 ± 0.20 |
3.78 ± 0.29 |
17.30 |
8.71 |
Total neutral sugar |
— |
— |
— |
— |
— |
— |
— |
— |
— |
— |
— |
— |
Crude fibre |
— |
— |
5.6 |
— |
— |
— |
— |
28.96 |
21.86 ± 1.01 |
57.84 ± 1.59 |
9.02 |
6.44 |
Table 2 Composition of CSM (semi-purified and non-purified) on a wet basisa
Component (%) |
Semi-purified |
Non-purified |
Chia seed polysaccharide21 |
CSM52 |
CSM53 |
CSM21 |
Chia seed polysaccharide and CSM:21 protein as (N × 6.25).
|
Moisture |
5.52 ± 0.14 |
9.60 ± 0.18 |
11.07 ± 0.13 |
6.49 ± 0.25 |
Crude fat |
Not detected |
1.01 ± 0.02 |
— |
7.32 ± 0.86 |
Total carbohydrates |
— |
73.59 ± 0.29 |
— |
— |
Total protein |
14.44 ± 0.14 |
9.45 ± 0.07 |
— |
14.45 ± 0.06 |
Total ash |
7.86 ± 0.38 |
6.26 ± 0.14 |
7.90 ± 0.11 |
9.40 ± 0.72 |
Total neutral sugar |
51.85 ± 1.26 |
— |
— |
36.63 ± 2.13 |
Uronic acid |
15.84 ± 1.12 |
— |
32.8 |
15.84 ± 1.12 |
Polyphenol |
0.58 ± 0.01 |
— |
— |
1.63 ± 0.07 |
Table 3 Monosaccharide composition of CSM (purified, semi-purified, and non-purified)
Component (%) |
Purified |
Semi-purified |
Non-purified |
Chia seed gum43,44 |
Chia polysaccharide21 |
CSM52 |
CSM35 |
CSM53 |
CSM47 |
CSM15 |
CSM21 |
Soluble fraction (35.0 ± 2.1%) |
Insoluble fraction (64.0 ± 3.2%) |
Xylose |
38.5 ± 2.4 |
11.0625 |
36.15 ± 0.15 |
11.0 ± 0.8 |
28.0 ± 2.2 |
27.8 |
63.07 |
43.1 |
9.1556 |
Fucose |
— |
— |
— |
— |
— |
— |
— |
0.5 |
— |
Glucose |
19.6 ± 3.2 |
0.8786 |
20.46 ± 0.16 |
82.0 ± 3.5 |
42.0 ± 2.4 |
26.6 |
5.88 |
6.3 |
4.394 |
Arabinose |
9.6 ± 1.8 |
4.9806 |
— |
1.0 ± 0.1 |
23.0 ± 1.1 |
11.5 |
9.80 |
41.8 |
3.3703 |
Galactose |
6.1 ± 1.7 |
1.2029 |
6.70 ± 0.07 |
4.0 ± 0.2 |
3.0 ± 0.1 |
1.4 |
3.16 |
7.8 |
3.7045 |
Rhamnose |
— |
— |
— |
— |
— |
— |
— |
0.5 |
— |
Mannose |
— |
0.0947 |
4.09 ± 0.01 |
3.0 ± 0.4 |
3.0 ± 0.1 |
— |
1.20 |
0 |
0.1211 |
Glucuronic acid |
18.7 ± 2.1 |
3.6196 |
— |
0 |
∼1 |
— |
13.72 |
— |
3.1257 |
Galacturonic acid |
5.3 ± 1.1 |
0.0858 |
— |
0 |
— |
— |
3.58 |
— |
0.14 |
Table 4 Minerals, molecular weight, and colour characteristics of CSM (purified, semi-purified, and non-purified)a
Component |
Chia polysaccharide (purified)43,44 |
CSM (heat and ultrasound)45 |
Semi-purified |
Non-purified |
Chia polysaccharide21 |
CSM35 |
CSM21 |
L*: lightness, a*: red/green coordinate, and b*: yellow/blue coordinate.
|
Mineral (mg/100 gm)
|
Potassium |
— |
— |
1635.00 ± 7.07 |
— |
3050.00 ± 0.00 |
Phosphorus |
— |
— |
255.00 ± 4.24 |
— |
601.50 ± 7.78 |
Magnesium |
— |
— |
530.50 ± 2.12 |
— |
481.50 ± 0.71 |
Calcium |
— |
— |
644.00 ± 4.24 |
— |
472.50 ± 2.12 |
Iron |
— |
— |
8.60 ± 0.13 |
— |
5.63 ± 0.22 |
Zinc |
— |
— |
0.84 ± 0.01 |
— |
1.24 ± 0.01 |
Copper |
— |
— |
0.52 ± 0.01 |
— |
1.07 ± 0.01 |
Molecular weight (Da) |
2.3 × 106 |
— |
— |
4.9 ± 0.2 × 105 |
— |
M
w (g mol−1) weight-averaged molecular weight |
— |
— |
1.718 × 105 |
— |
3.985 × 105 |
M
n (g mol−1) (number-averaged molecular weight) |
— |
— |
4.883 × 104 |
— |
3.463 × 105 |
M
w/Mn |
— |
— |
3.519 |
1.02 ± 0.05 |
1.151 |
L* |
85.7 ± 1.4 |
21.23 ± 0.24 to 38.42 ± 0.68 |
— |
— |
— |
a* |
0.67 ± 0.10 |
1.90 ± 0.03 to 4.26 ± 0.02 |
— |
— |
— |
b* |
8.5 ± 0.9 |
5.77 ± 0.06 to 10.85 ± 0.07 |
— |
— |
— |
2.1 Chemical makeup of chia seed mucilage
CSM is primarily made up of tetrasaccharide repeating units. These units are composed of (→4)-β-D-xylopyranose-(1 → 4)-α-D-glucopyranose-(1 → 4)-β-D-xylopyranose units with a substituent of 4-O-methyl-α-D-glucuronic acid at the O-2 position of alternate β-D-xylopyranose residues.54 CSM is also characterised by its diverse sugar compositions, including mannose, xylose, glucose, arabinose, galacturonic acid, glucuronic acid37 and galactose.43 Furthermore, it contains planteose, a member of the galactosyl-sucrose oligosaccharide family, noted for its health benefits.55 The detailed chemical makeup of CSM is illustrated in Fig. 2.
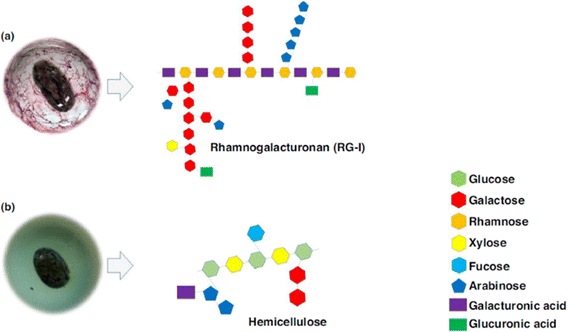 |
| Fig. 2 Illustration of the chemical structure of major polysaccharide fractions in crude plant seed mucilage. Part (a) displays the pectic polysaccharide fraction of CSM, highlighted using ruthenium red staining, whereas part (b) depicts the hemicellulose fraction of CSM, marked with methylene blue staining. This picture is adapted from Soukoulis et al.11 | |
2.2 CSM yield
The development of CSM around chia seeds with the contact of water was confirmed by various scientists with the help of microscopy and stains. According to Muñoz et al.,27 maximum thickness (414 ± 35 μm) of mucilage around the seed is visible after 2 hours of hydration. However, the calculation of yield in the literature varies depending on the method and formula used. When selecting the optimal extraction method, yield and the intended application of the mucilage are important considerations. Some studies have calculated yield by measuring the quantity of CSM obtained from the original quantity of chia seed used in the extraction. Other studies consider yield by comparing the weight between the mucilage-free seed, after drying post-mucilage separation and the seeds before separation. Additionally, yield calculations based on the dry matter content of the seeds are noted as well. Table 5 presents different ways of representing mucilage yield under various extraction conditions. It is obvious that extraction parameters significantly influence the yield, and in dry-fractionation processes, the sieve size impacts mucilage yield. A review by Chiang et al.42 has explained the impact of temperature, time, water/seed ratio, pH and the method of extraction on the yield of chia seed mucilage. Even some reports are available with the use of response surface methodology (RSM) to understand the impact of these factors on techno-functional properties.20,27,56,57 Interesting facts revealed from a review that hydration conditions have more impact on the yield of mucilage while the proximate composition is affected by the extraction and recovery method.42 However, a review by Chiang et al.42 has explained the impact of the literature available up to 2021. So, in this section, the explanation is focused on the impact of these factors (hydration ratio, temperature, pH, and time) on yield with the implication of non-thermal technologies only. Indeed, a few studies have used non-thermal technologies along with these parameters, which are further discussed in the following sections.
Table 5 Extraction conditions and yield of CSMa
Conditions for hydration |
Seed-to-water ratio |
Method of extraction |
CSM/CSG/CCSG |
Yield representation |
Reference |
CSM: chia seed mucilage, CCSG: crude chia seed gum, and CSG: chia seed gum.
|
Room temperature hydration (RT) |
1 : 20 (2 h), with continuous stirring |
Dry fractionation (200-micron sieve) |
Crude gum (non-purified) |
5.6% of dry seed mass |
43
|
RT |
1 : 20 (2 h), with continuous stirring |
Dry fractionation (200-micron sieve), followed by defatting, and purification |
Purified gum, yield calculated based on lyophilized gum with respect to dried seed mass |
3.8% |
43
|
4, 40 and 80 °C temperature, pH 4, 6, and 8 |
1 : 20, 1 : 30, and 1 : 40 ratios, and stirring with a magnetic stirrer |
Dry fractionation (40 mesh screen) |
CSM |
6.97% based on the initial seed taken |
27
|
80 ± 1.5 °C, pH of the mixture adjusted to 8 |
1 : 40 (2 h hydration using a magnetic stirrer) |
Oven drying followed by dry fractionation using a 40 mesh screen |
CSM |
6.42% |
51
|
pH value 8.0, 27 °C |
1 : 10, 1 : 20, 1 : 30 and 1 : 40 ratio; magnetically stirred and hydrated for 2 h |
Controlled pressing followed by freeze-drying |
CSM |
1 : 10–4.76%, 1 : 20–8.46%, 1 : 30–8.65% and 1 : 40–8.31% non-significant yield at different ratios (1 : 20–1 : 40) |
51
|
RT (25 °C) |
Defatted seed 1 : 20 ratio |
Mechanical agitation and sonication, followed by oven drying |
CSM |
15.1% |
19
|
Hydration at 85 °C, pH 6.0 |
1 : 10 (w/v) |
Continuous stirring using a magnetic stirrer |
Chia seed polysaccharide mucilage (purified by using 95% ethanol in water) (1 : 1 v/v) |
Not mentioned |
26
|
Deionised water, pre-boiling for 5 minutes |
1 : 20 (w/v) |
Stirring at 1300 rpm at room temperature for 2 h and further use of an ULTRA-TURRAX at 3000 rpm for 0, 0.5, 1, 1.5, 2.0, 2.5 and 3 minutes followed by centrifugation at 10 000 rpm for 10 min (20 °C) followed by oven drying at 50 °C |
CCSG (crude chia seed gum) and dry seed gum divided by chia seed mass |
1300 rpm-1.80 ± 0.41% 3000 rpm with 1.5, 2.0, 2.5, and 3 minutes-6.19%, 6.25%, 6.35% and 6.38% respectively showing a non-significant difference |
21
|
Deionised water, pre-boiling for 5 minutes |
1 : 20 (w/v) |
High shearing by using the ULTRA-TURRAX at 3000 rpm for 0, 0.5, 1, 1.5, 2.0, 2.5 and 3 minutes followed by centrifugation at 10 000 rpm for 10 min (20 °C), alcohol precipitation and freeze drying |
Chia seed gum (semi-purified) and dry seed gum divided by chia seed mass |
Yield not mentioned |
63
|
Warm distilled water (50 °C) |
1 : 20 (w/w) for 20 min at 1000 rpm of overhead stirring |
Use a Silverson mixer for 20 minutes at 2800 rpm, centrifugation at 12800g, at 20 °C, and freeze-drying |
CSG, extracted seed gum to the chia seed |
3.4 ± 0.2% (w/w) |
64
|
Warm Milli-Q water |
1 : 20 w/v |
Magnetic stirrer for 3 h at a constant temperature of 60 °C, followed by centrifugation at 3382g for 20 minutes and freeze-drying |
CSM, lyophilized mucilage per 100 g of seed |
7.24% |
65
|
Distilled water |
1 : 30 (weight to volume) |
Stirring at 80 °C for 30 min, filtration through nylon cloth, and ethanol precipitation and oven drying at 40 °C for 24 h |
Chia seed mucilage precipitated sample (MCHs), dried mucilage to the dried seed weight |
7.9 ± 1.5% |
53
|
Deionised water was maintained at 20 °C with constant stirring |
1 : 40 w/v |
Constant stirring at 20 °C, then oven drying at 50 °C for 10 h and dry fractionation using rubbing over a 40-mesh screen |
Crude mucilage calculated with respect to the seeds |
8.3% of the seed |
39
|
Ultrapure water |
1 : 20 (w/v) ratio for 12 h to extract the mucilage |
Vacuum filtration using a Buchner funnel followed by freeze-drying |
CSM-calculated as the amount of freeze-dried mucilage to the amount of seeds |
5% (w/w) of the dried seed mass |
66
|
With water for 30 minutes at 50 °C temperature |
1 : 20 (w/v) |
Milling the suspension and boiling at 50 °C under stirring for 15 min. Centrifugation at 9460g at 15 °C for 3 h, followed by freeze-drying |
Fatted (FCG) |
10.9% |
49
|
Whole chia seeds were immersed in distilled water, for 2 h, RT (25 °C) |
1 : 30 (w/v) |
An electric stirrer was used for the stirring, followed by 9000g for 30 min, vacuum filtration using cheesecloth, and oven drying at 60 °C for 18 h |
CSM |
Not determined |
16
|
Chia seeds (100 g) were washed with ethanol (200 mL) under magnetic stirring (2 min) to remove impurities and were subsequently recovered with a strainer |
Seed to water ratio (1 : 1000 p/v) |
Constant stirring at 4 h, hydrated seeds were freeze-dried for 5 days, and further CM was separated from seeds using sieves (mesh; 297 μm) |
CSM: yield was calculated based on the mass of chia seeds divided by mucilage |
5.31% total dry matter |
67
|
Distilled water, RT |
Seed-to-water ratio of 1 : 30 |
Mechanically stirred using an overhead stirrer (Caframo Ltd, model BDC2002, Ontario, Canada) for at least 2 h at 25 °C. Centrifugation at 11 600g for 30 min, followed by filtration with a vacuum pump and sieving. Furthermore, filtered through cheesecloth and freeze-drying |
Not calculated |
— |
68
|
Whole seeds using distilled water |
Water:seed ratio of 10 : 1–30 : 1 |
30–80 °C temperature during the extraction. The mixture was stirred for 2–4 h and dried overnight at 50 °C, followed by dry fractionation using a 30 mm screen |
CSM: yield determined based on the dry weight of the crude gum relative to the seed weight |
5.09 g/100 g, at 70 °C, 2.4 h with 14 : 1 (water : seed) ratio |
20
|
Deionized water, RT (25 °C) |
1 : 30 (w/w) |
Stirred using a mechanical stirrer for 3 h, 4.5 h, and 6 h, followed by vacuum filtration using cheesecloth and centrifugation at 4 °C, 18 000 rpm, for 40 min. Furthermore, freeze-drying of the insoluble fraction of mucilage |
CSM: dried weight of mucilage with respect to the initial weight of the seed |
3.94–4.75% |
15
|
Deionized water, RT (25 °C) |
1 : 30 (w/w) |
Stirred for 3 h at room temperature, and further sonicated at 500W, 20 kHz, amplitude of 40%, and pulse 02 s on and 03 s off for 3 min (U3), 10 min (U10), and 30 min (U30), respectively, followed by centrifugation and freeze-drying |
CSM: dried weight of mucilage with respect to the initial weight of the seed |
2.57–4.27% |
15
|
Distilled water, RT |
1 : 10 (w/v) |
Hydration in a tray at room temperature for 4 h. Freeze drying followed by dry fractionation (840 μm sieve) |
CSM: yield based on dry base weight |
3.8 ± 0.1(d.b.) |
69
|
Water, RT |
1 : 20 (w/v) |
Manual stirring followed by vacuum filtration (100 μm) at 220 mbar and further rotary evaporation at 55 °C under vacuum followed by freeze drying |
CSM: yield based on dry base weight |
3.7 ± 0.1% (d.b.) |
69
|
2.2.1 Hydration ratio (seed/water).
Recently Saporittis et al.57 have used various ratios such as 1
:
10, 1
:
15 and 1
:
20 for CSM extraction along with the extraction time and temperature as a factor. This study revealed that extraction yield was higher at a ratio from 1
:
20 to 1
:
15, along with extended extraction time and temperature. A previous report by Muñoz et al.27 defined the major impact of temperature and the seed/water ratio on the mucilage yield when the hot water & dry-fractionation method was employed. Orifici et al.56 revealed that considering 50 °C temperature for extraction and changing the ratio from 1
:
7.2 to 1
:
24 has raised the CSM yield from 80.3 ± 0.71 to 92.1 ± 0.56 (g kg −1 (d.b.)). In the same study, changing the ratio from 1
:
12 to 1
:
36 has increased CSM yield at 25 to 75 °C temperature.
2.2.2 Temperature.
A study by Campos et al.,20 who used the dry-fractionation method along with the ratio (water
:
seed ratio of 10
:
1–30
:
1), stirring time (2–4 h), and temperature (30–80 °C), confirmed that the impact of these independent variables on yield follows the order of extraction temperature > water
:
seed ratio > extraction time. This study confirmed that by fixing a 1
:
30 ratio, a higher yield can be derived at a higher extraction temperature (80 °C) and shorter extraction time. Regardless of the hydration ratio, the yield percentage increased from 20 to 80 °C during the temperature shift.27 Higher temperature gives more mucilage yield as the viscosity of the mucilage is reduced and affects the stickiness of the seeds.58 Elevated temperature leads to higher and faster mass transfer of water-soluble polysaccharides from the cell walls into the extract.59 This facilitates the release of mucilage and increases the yield of mucilage.58,59 Other researchers also studied the impact of temperature as a potential variable affecting the mucilage yield.56,57 The use of non-thermal technologies for mucilage extraction, such as ultrasound with different amplitudes and parameters at controlled temperatures, may exhibit different phenomena for mucilage extraction. This will give future research directions for a higher yield of mucilage with reduced temperature using non-thermal technologies.
2.2.3 pH.
A study by Muñoz et al.27 provided evidence for the impact of pH on the extraction yield. Studying the impact of pH ranging from 4 to 8 showed a reduction in mucilage yield when pH shifts from alkaline to acidic along with the other studied variables during hot water-based extraction. However, the study could not provide a direct explanation, but it was later determined that CSM has an anionic character. Under alkaline conditions, CSM attains maximum negative charge, which supports the solubility of CSM in the extraction medium due to the enhanced ionization of the carboxyl groups in uronic acids.43,45 This phenomenon is also considered while studying the solubility of CSM at various pH along with the iso-electric point of proteins in CSM.
2.2.4 Time.
According to Muñoz et al.,27 after 2 h of hydration, chia seeds attain maximum constant weight and water absorption is completed. In a later year, the report from Salgado-Cruz et al.60 suggested that, understanding the kinetic release of mucilage around the seeds, no more growth of mucilage was visible after 30 minutes of hydration. Some reports have mentioned the impact of solely hydration time and as a combined contributing factor with other variables. The effect of time on yield was more pronounced at higher temperatures observed by Campos et al.20 RSM-based analysis by Orifici et al.,56 who studied the impact of hydration time (1, 2, and 3 h) at 60 °C with 1
:
40 (seed
:
water ratio), confirmed an increase in yield along with the increase in hydration time and reduction at 3 h of hydration. Furthermore, other researchers also used 2 h of hydration for their work.21,27,28,40,43,51,61 It is interesting to study the mechanism of reduction of mucilage yield at higher hydration time with increased temperature in the future.56 According to the reports of Saporittis et al.,57 along with an increase in extraction time from 30–210 minutes, using a magnetic stirrer followed by 3 minutes of UAE has significantly affected the yield of mucilage. However, it was revealed that at lower extraction time, the yield was independent of the temperature, but along with an increase from temperature 25–65 °C, yield became more temperature dependent while extracting mucilage with ultrasound (3 minutes of treatment time).57 Longer stirring time, along with higher temperature, also affects the apparent viscosity of the mucilage, and modifying its molecular structure also impacts the apparent viscosity.15,58,62 While considering better methods for higher mucilage yield from the literature, it is important to consider the impact of yield calculation, the impact of pH, hydration ratio, temperature, hydration time, method of separation, and the variety of chia seeds.
2.3 Extraction of chia seed mucilage (CSM) through traditional techniques
The extraction process of CSM involves three main steps: (i) hydrating the seeds, (ii) extraction of the mucilage, and (iii) recovery of it.42 This process also includes defatting and deproteinization to purify chia seed polysaccharides. The yield of CSM varies between 1.2 and 15%, with hydration conditions being a key factor. These hydration conditions greatly influence mucilage yield, and subsequent extraction and recovery steps affect CSM's chemical composition.42 Hydration of chia seeds involves various parameters, such as the chia seed: water ratio (e.g., 1
:
10, 1
:
20, 1
:
30, and 1
:
40),20,27 temperature (ranging from 4 to 80 °C),27,70 hydrating or stirring time (from 30 minutes to 24 h),20 pH of the hydrating medium (4–8)27 and stirring speed. As per Muñoz et al.,27 2 hours of hydration is typically sufficient for water as evidenced by their constant seed weight. However, hydration times reported in the literature vary widely (2 to 24 hours)15,28,70,71 with no clear consensus. Studies found that hydrating chia seeds at a 1
:
40 ratio (seed
:
water) at 80 °C and pH 8.0 for 2.5, 3, and 3.5 h insignificantly affected the total exudative mass,72 aligning with the findings of Muñoz et al.,27 for a 2-hour hydration period.
The extraction method impacts the yield of chia seed mucilage.42 Various mechanical instruments, such as depulpers and mixers, are employed to shear the hydrated seeds and separate the tightly bound mucilage. This process results in a two-phase mixture of mucilage and seeds73 due to density differences. Other techniques to remove the mucilage include ultrasound, centrifugation, hand-pressed mesh/screens, and filtration. The purification process typically involves adding n-hexane and ethanol to remove non-polysaccharide compounds from the mucilage.41 Specific extraction methods are described in further detail below in Fig. 3.
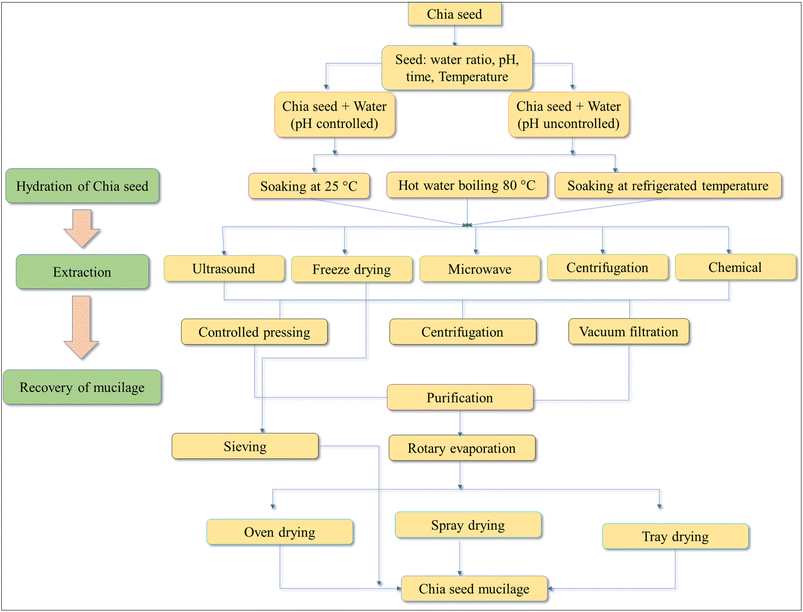 |
| Fig. 3 General process for extraction of CSM (modified from Chiang et al.42). | |
2.3.1 Hot water-based extraction.
Extracting mucilage from chia seeds often involves hot water-based extraction. This method has shown that using hot water at higher temperatures can reduce the viscosity of mucilage fibre and increase its solubility, thereby enhancing the mass transfer rate of these mucilage fibres. Consequently, mucilage fibres can be more effectively released from chia seeds, leading to higher yields.56
For instance, mucilage fibres were more dispersed on the surface of the chia seeds hydrated at 80 °C compared to those hydrated at 50 °C. This supports the effectiveness of hot water extraction.45
Muñoz et al.27 achieved a yield of 6.97% yield of CSM using a 1
:
40 seed-to-water ratio, a pH of 8, and hydration at 80 ± 1.5 °C for 2 h (temperature), followed by drying at 50 °C for 10 hours. They found the extraction yield (1.09%) of mucilage at 50 °C (60 minutes) to be significantly lower than the yield at 80 °C (1.86%).45 Other researchers have adapted these hydration conditions, applying additional methods to separate mucilage. For example, Felisberto et al.74 combined the hot water extraction method with mechanical separation and freeze drying to obtain 7.86 g of mucilage per 100 gm of chia seeds. The process of hot water-based extraction, followed by dry fractionation and centrifugation, is summarised in Table 5.
2.3.2 Chemical extraction.
Ethanol is another agent used in the purification of chia seed mucilage. By adding ethanol, the polarity of the solvent is reduced, aiding in the precipitation of mucilage.75 da Silveira Ramos et al.53 extracted 7.9% CSM by hydrating the seeds (seed
:
water 1
:
30 ratio) in hot water at 80 °C for 30 minutes, then filtering through nylon cloth and precipitating with ethanol (3
:
1 v/v), followed by oven drying at 40 °C for 24 h. Various methods for CSM extraction have been employed depending on the intended use of the mucilage. Timilsena et al.38 used sodium carbonate for extraction. They extracted oil from ground chia seeds and mixed the defatted chia seed flour (meal) and then mixed it with a 0.5 M NaHCO3 solution containing 0.02% w/v of sodium azide at a 1
:
10 ratio. After stirring for 18 h at room temperature, the mixture was filtered and washed with water (5 × 100 mL) to remove residual mucilage, resulting in flour ready for protein isolate extraction.
3 Potential of using non-thermal technologies for mucilage extraction
Several researchers have developed an alternate method to extract mucilage from chia seeds. This method involves a series of steps: defatting, hydrating, solubilizing, filtering, dehydrating, and grinding the seeds.76 Notably, supercritical fluid extraction technology (SFT) was used to remove oil from chia nutlets (both whole and crushed) under specific conditions (at 80 °C, 450 bars for 40 min of dynamic extraction with a CO2 flow rate of 1.8 g min−1). This method was compared to traditional Soxhlet extraction, examining the resultant functional properties. Results showed that whole nutlets treated with SFT yielded more chia seed gum than those processed by Soxhlet extraction, examining the resultant functional properties. Results showed that whole seeds treated with SFT yielded more chia seed gum than those processed by Soxhlet extraction.76 For crushed seeds, gum yields were similar with both methods. Nevertheless, the choice of oil extraction technique impacted the functional qualities of the gum. Gum from whole seeds extracted via SFT demonstrated superior water-holding capacity (WHC) and water adsorption, along with notable oil-holding capacity. These findings imply that such sustainable technologies can effectively produce essential ingredients for the food industry.
Various separation methods, such as dry fractionation, pressure-based separation, vacuum filtration, and centrifugation, are mentioned as prominent separation techniques. A higher number of reports are available with the use of centrifugation (low and high speed) as a separation technique to derive seeds or mucilage based on their objective of the study. Centrifugation is a technique for separating molecules with different densities by rapidly spinning them in solution around an axis (in a centrifuge rotor).77 It is a method to separate solid–liquid or liquid–liquid compounds that differ from each other in their density using centrifugal acceleration.78 In CSM extraction, centrifugation was used to protect the natural molecular structure of the biopolymer, which affects the functional properties of the mucilage.28 Centrifugation is a simplified extraction method, easy to apply in industry and less time-consuming as it combines the extraction and purification of CSM. Mainly, centrifugation was employed to extract both soluble and non-soluble gel fractions.28 In a study, Brütsch et al.28 hydrated chia seed in water (1
:
20 ratio) at 25 °C for 2 hours under continuous stirring using a magnetic stirrer for complete hydration. Centrifugation was carried out at 6600g for 50 minutes and produced three different layers in the tubes (top layer-seeds and excess water including some of the soluble polysaccharide fraction, the middle layer-mucilage, the bottom layer-chia seeds).28 This mucilage was further stabilized using a drying method, such as oven drying, rotary evaporation, or freeze-drying.28 Similar observations of three different layer formations during centrifugation were confirmed by the other researchers.15,21,35,57,64,79 A recent report, which used ultrasound and centrifugation, again explained the formation of two different phases (opaque and translucent phase) but correlated the ratios of translucent to opaque with the initial seed:water ratio during extraction and its impact on apparent viscosity.57 This also provided new insights about the direction for future optimization with non-thermal technologies. The yield of mucilage also differed upon mixing both phases and discarding the transparent phase after centrifugation. Apart from these, centrifugation was utilized by various researchers to separate the tightly bound mucilage from the seeds alone or in the company of vacuum filtration.8,10,15,16,26,28,41,57,72,80,81 Other techniques include vacuum filtration,70,80,82 brush depulper and then filtering through a 0.85 mm screen74 or 35/CM-876 finisher pulper with a stainless-steel wire mesh with 0.26 mm apertures,83 controlled pressing51etc.
Furthermore, non-thermal methods such as ultrasonication, cold plasma, microwave, and pulsed electric field are increasingly explored for extracting mucilage from chia seeds. These methods align with the concept of green technology.
3.1 Ultrasonication
Ultrasound is an eco-friendly extraction technology based on the cavitation phenomenon. This process involves the formation, growth and explosive collapse of air bubbles in a liquid.84 This collapse enhances the mass transfer of compounds, produces high shear forces in the extracting agent85 and increases solubility by stressing and deforming the cellular structure.84 The process allows the creation of microchannels as bubbles collapse, further aiding mass transfer. The accompanying increase in temperature, turbulence, and mixing due to cavitation enhances the extraction efficiency.84 These dynamics facilitate the breakdown of the matrix holding the desired product, promoting its transfer into the solvent.15 The impact of different times of ultrasonication is shown in Fig. 4.
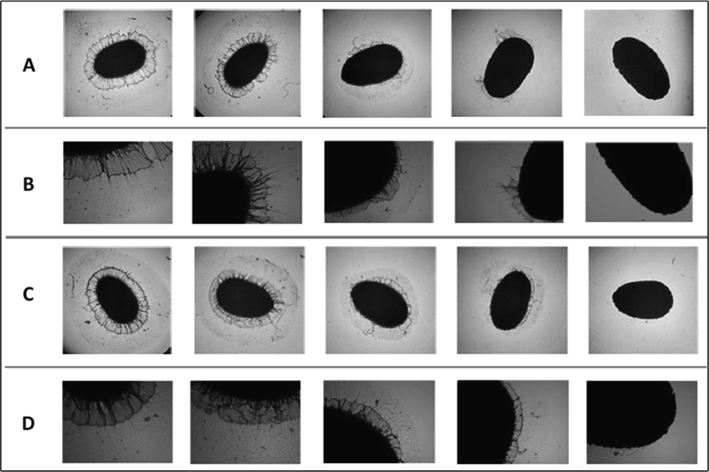 |
| Fig. 4 Optical microscopy images of chia seed mucilage extract using ultrasonication. Optical microscopy images of chia seed extract without sonication and with sonication for 10 s, 30 s, 1 min and 3 min at (A and B) a seed : water ratio of 1 : 20, temperature 65 °C and time 210 min, and (C and D) seed : water ratio of 1 : 10, temperature 45 °C and time 60 min. (A and C) Magnification 40×; (B and D) amplified sections of the original images. These images, magnified and zoomed in, are adapted from Saporittis et al.57 | |
In chia seed (CS) extraction, ultrasonication produces microjets that target specific seed structures, enabling efficient mucilage separation.70 Urbizo-Reyes et al.70 used this method, combined with vacuum-assisted filtration, to extract chia seed mucilage (CSM). The process involved initial hydration of the seeds (1
:
20 ratio by weight for 24 h under refrigerated conditions), followed by pre-heating to 55 ± 2 °C and then ultrasonication (5 min) at a 75% power input (amplitude 90 μm) using an ultrasonic cell disruptor. Using a double-walled beaker connected to an immersion circulator control water bath, the temperature was kept constant (60 ± 4 °C) during ultrasonication (Fig. 5).
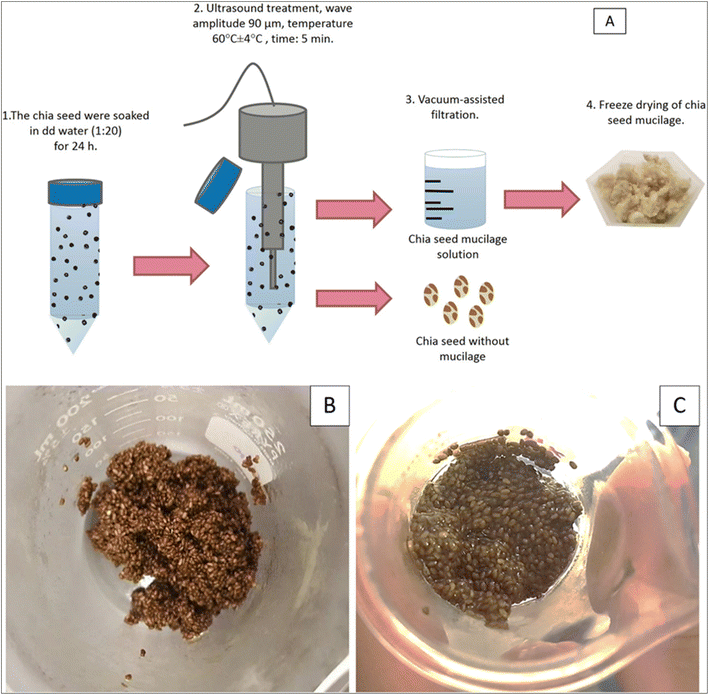 |
| Fig. 5 (A) Ultrasonication and vacuum-assisted separation of CSM, (B) ultrasound and vacuum-filtration separated clean seeds, and (C) ultrasound and centrifugation separated seeds (adapted from Urbizo-Reyes et al.70). | |
Further separation was carried out using vacuum filtration.70 This method yielded significantly more CSM (7.65%) compared to oven drying (3.65%) and freeze-drying (4.21%). Wang et al.45 combined ultrasound and heat to extract CSM, achieving higher yields (6.92–10.52%) than with heat alone (1.03–1.86%). Similarly, Castejón et al.86 used ultrasonication (30% of maximum ultrasonic power) on hydrated (1
:
40 seed-to-water ratio for 2 h with constant stirring) and heated (50 °C) chia seeds for 3 min followed by centrifugation (4000 rpm for 20 min), noting the impact of high temperature during sonication on seed and mucilage interaction. They reported a 6.52% yield of CSM and recommend controlled temperature-based extraction between 37 and 40 °C for optimal results.
Conversely, Silva et al.15 observed lower yields using room temperature ultrasonication (for 3, 10, and 30 minutes) on hydrated chia seeds [1
:
30 (seed
:
water) for 3 hours], followed by separation centrifugation and freeze-drying. The reduced yield was 3.35% (3 min), 2.34% (10 min), and 3.68% (30 min) attributed to the loss of soluble components during the process. This method resulted in different protein contents compared to other room-temperature extraction methods.
In another study, ultrasound was utilized,86 presenting an alternative to basic and acidic media for mucilage extraction, which could negatively affect the demucilaged seeds and the quality of the subsequently extracted oil, particularly omega-3 PUFA. The study achieved a 6.52% ± 0.08 yield of mucilage after 2 h of hydration at 50 °C, followed by 3 minutes of ultrasonication, indicating that ultrasonication can enhance mucilage yield without harsh effects on the demucilaged seeds. Furthermore, pressurized liquid extraction of ultrasound-treated seeds yielded effective results in oil extraction from chia seeds.86
3.2 Cold plasma
Cold plasma, recognised as the fourth state of matter, is a partially ionized gas formed when sufficient energy is applied to a neutral gas or combination of gases. This process generates various reactive species such as ions, electrons, and radicals.87 Recent advancements in plasma physics engineering have shown that generating cold plasma at atmospheric pressure is both cost-effective and straightforward, making it applicable for treating solid and liquid food ingredients. Popular methods include plasma jets and dielectric barrier discharge configurations, chosen for their simple design, scalability, and ease of implementation.88 Cold plasma is increasingly utilised in food processing for diverse purposes, such as microbial disinfection, enzyme deactivation, breakdown of allergens or pesticides, and enhancing nutritional values.89 The effectiveness of cold plasma treatment depends on various factors: treatment duration, gas type, frequency, voltage, and electrode spacing, which influence the reactive substances produced.90 One specific application involved using a cold atmospheric pressure plasma jet on CS, with argon as the working gas. Treatments lasted 30, 60, and 120 seconds.91,92 Mucilage extraction from CS was performed through dry fractionation of freeze-dried and soaked seeds,92 as well as centrifugation followed by oven drying.91 While the yield of mucilage was not specified, notable changes were observed in its functional properties. FTIR revealed the crosslinking of polysaccharides upon the use of argon gas in cold plasma. Crosslinking in the polysaccharide chains of CSM enhanced resistance to deformation and improved elasticity and viscosity of the gel due to a stronger polymer network. These alterations suggest potential new uses for this gum in food applications.
3.3 Microwave-assisted extraction (MAE)
Microwave-assisted extraction is an increasingly popular method that offers more efficient extraction than conventional techniques. This method involves transmitting microwaves, which can permeate biomaterials and heat them by interacting with polar molecules such as water. This interaction allows microwaves to heat the material up to a certain depth uniformly.
Essentially, the process operates on the principle of ionic conduction and dipole rotation induced by microwaves, leading to heat generation. This heat creates pressure on the cell wall, resulting in the breakdown of cell constituents into the extraction solvent, thereby enhancing yield.93 A notable example is the successful use of MAE in extracting mucilage from Opuntia ficus-indica cladodes94 and chia seeds.95
Specifically, Sameera and coworkers95 applied MAE for CSM extraction, achieving an 8% yield. The process involved hydrating the seeds at a 1
:
40 seed
:
water ratio, heating in a microwave at 1.3 W gm−1 until reaching 80 °C (∼15 minutes) and then stirring at room temperature for 30 minutes. The microwave-treated sample underwent filtration and was dried in a hot air oven at 50 °C for 12 to 14 h. However, this is the only study that has reported on microwave-assisted extraction of CSM. Additionally, MAE has been successfully applied in extracting mucilage from flaxseed.94,96
3.4 Pulsed electric field (PEF)
Pulsed electric field (PEF) processing utilizes brief, high-intensity electric pulses ranging from nanoseconds to microseconds. A PEF system involves two electrodes connected to a high-voltage electric field source, typically ranging from 5–50 kV cm−1, and a food matrix is positioned between these electrodes. The food matrix is exposed to several (1–1000) short pulses from microseconds (μs) to milliseconds (ms) at specified frequencies (Hz). The amount of specific energy inputs (kJ kg−1) delivered varies based on factors such as electrode dimensions, electric field strength, pulse width, frequency, treatment period and many other factors.97
A PEF leads to “electroporation” that increases the permeability of cell membranes either in animal or plant cells. This effect benefits diverse fields, including biotechnology and medicine for cell substance delivery, cell fusion, water treatment, and food processing for pasteurisation and structure modification that enhances pressing, extraction, drying, and diffusion.98 Research also indicates its effects on the starch digestibility of oat flour99 and modifications in the technological and structural properties of vegetable protein concentrates (pea, rice, and gluten);100 whey protein isolates101 and soy protein isolates.102 The PEF has also been effective in protein extraction and the generation of peptides.103 It offers non-thermal methods of food preservation104 and is reported to alter the thermal stability of pectins105 and the physical and biochemical properties of chitosan106 and tapioca starch.107 While its effects on chia seeds remain unexplored, it is hypothesised that electroporation may aid in mucilage separation from chia seed when various energy inputs are applied, with subsequent separation techniques such as centrifugation and lyophilization for mucilage recovery while preserving its fibrous structure.
4 Effects of non-thermal processing on the physical and functional properties of CSM
4.1 Ultrasonication
The extraction and recovery of CSM significantly affect its physical and functional properties. While commonly described as milky white, CSM's colour varies with different extraction methods. Wang et al.45 found that CSM extracted using ultrasound and heat displayed a darker colour than that by other methods. This method resulted in lower lightness compared to heat extraction alone. However, ultrasound extraction from Salvia macrosiphon seeds suggests that parameter optimisation is crucial. High ultrasound intensity alters the molecular and rheological properties,108 increases non-polysaccharide components, reduces lightness, and affects the consistency of hydrocolloid solutions from S. macrosiphon seeds, as compared to milder conditions.108
Saporittis et al.57 examined the effects of chia seed
:
water ratios (w/v) (1
:
10, 1
:
15, and 1
:
20), extraction temperatures (25–65 °C), and magnetic stirrer durations (30–210 minutes) followed by 3 minutes of ultrasonication on yield and apparent viscosity. They found that optimal yield and viscosity were achieved with a 1
:
10 seed: water ratio, 25.3 °C temperature, and 53.7 minutes of extraction. Conversely, for minimal viscosity and maximal yield, the parameters differed: 1
:
20 ratio, 48.8 °C, and 208.4 minutes. This demonstrated that the rheological properties of CSM vary with extraction methods.
Silva et al.15 compared ultrasound-treated CSM (3, 10, and 30 minutes) with 3 h of mechanical stirring to untreated samples (3, 4.5, and 6 h of mechanical stirring at room temperature). They observed that prolonged stirring and ultrasonication affected the apparent viscosity (η100 s−1) and consistency index (k) of CSM dispersions. Thirty minutes of sonication reduced viscosity by one-third compared to other samples. Longer stirring times have reduced the fibrillar aggregates, while prolonged ultrasonication affected the carbohydrate content. Wang et al.45 also noted a decrease in carbohydrate content when heat and ultrasound were applied at high temperatures and for extended periods for CSM extraction, which further affected mucilage solubility and other functional properties. Extreme conditions also lowered the water-holding capacity (WHC) of CSM, though the oil-holding capacity (OHC) remained unchanged. Additionally, the emulsion stability index (ESI) decreased due to carbohydrate degradation from high heat ultrasonication. Such treatments produced gels with more Newtonian-like behaviour and reduced viscosity.45
The viscoelastic behaviour of CSM was also affected by stirring time and ultrasonication duration. Extended ultrasonication resulted in CSM with lower G′ values, especially at lower frequency values. More fragile structures emerged under intense ultrasonication, whereas longer stirring times yielded more elastic and resistant structures, likely due to the more heterogeneous structures formed through ultrasonication, separating fibrils from aggregates compared to untreated samples.15
4.2 Cold plasma
Limited research, specifically two studies, explored the effects of cold plasma on chia seeds and the functional properties of CSM recovered via freeze-drying92 and oven-drying91 methods. These studies demonstrated that treating chia seeds with cold plasma for 30, 60, and 120 seconds and subsequently extracting mucilage using the dry fractionation technique led to a decrease in the CSM's lightness and an increase with prolonged treatment durations. This effect is likely due to the interaction of reactive plasma species (e.g., H+, H3O+, O+, and OH−) with the chia seeds, triggering various chemical reactions. Notably, the Maillard reaction, encompassing both enzymatic and non-enzymatic browning, became more susceptible during cold plasma treatment, as the temperature reached 60–70 °C, even for brief processing periods (<120 s).92 Another study confirmed similar findings by treating chia seeds with cold plasma, followed by hot water extraction and oven drying, and then dry fractionation. The research noted a decrease in lightness and increases in a* (red-greenness) and b* (blue-yellowness) values, indicating the high energy plasma's impact on protein surfaces, causing unfolding. This unfolding led to the activation of the protein surface, increasing free amino acids, which then reacted with sugars, facilitating Maillard reactions by providing the necessary energy.91,92
It was also noted that the duration of cold plasma treatment adversely affected the solubility, WHC, and OHC of the CSM. The reduction in solubility was attributed to a decrease in free OH groups, essential for hydrogen bonding with water, enhancing solubility. Furthermore, a decrease in uronic acids, which contribute to CSM's solubility, and the formation of insoluble compounds at elevated temperatures during extraction were also contributing factors.91 The WHC reduction was similarly linked to the reduced presence of free OH groups. Interestingly, the emulsion capacity and stability remained constant in cold plasma-treated samples compared to controls. Structural changes in cold plasma-treated chia seeds were confirmed through scanning electron microscopy (SEM), revealing laminar plate aggregates in the hot water-extracted CSM pre-treated with cold plasma. These structural changes, attributed to condensation and prolonged treatment,91 resulted in a more compact structure. Conversely, cold extraction maintained a smoother surface, influencing the rheological properties of CSM.
Fourier-transform infrared spectroscopy (FTIR) analyses confirmed cross-linking in CSM caused by cold plasma, particularly when using argon gas. This process correlated with reduced OH group absorbance due to cross-linking.91 This trend was not seen in CSM extracted cold from cold plasma-treated seeds,92 where cold extraction facilitated more OH group incorporation, stabilising structures. Cold extraction enhanced the OH absorbance peak compared to hot extraction.92 Moreover, the choice of working gas in cold plasma, such as argon, plays a crucial role in the oxidation reaction or cross-linking.91 Excitation of argon atoms via high energy electrons can lead to dehydration or cleavage between polymeric chains (C–OH), primarily at the C-2 site, forming new C–O–C linkages.109
The rheological properties of cold plasma treated CSM indicated increased viscosity with longer treatment times compared to control samples, likely due to cold plasma generated reactive species.91,92 The increase in viscosity aligned with reduced solubility, as cold plasma could enhance hydrophobic over hydrogen and electrostatic interactions. The pseudoplastic behaviour of CSM post cold plasma treatment suggested increased intermolecular interactions, improving food texture92 and mouthfeel during mastication. Cold plasma treatment for 60 seconds was found to reduce yield stress, possibly due to polysaccharide chain depolymerization and intermolecular cross-linking. These findings provide potential applications for cold plasma as an eco-friendly technology for depolymerizing food waste and by-products.91 Nonetheless, this trend was not observed in CSM derived via cold extraction from cold plasma-treated seeds, indicating that cold extraction promoted more intermolecular linkage,92 leading to stronger gel structures induced by cross-linking. However, depolymerization was observed to create weaker gel structures, hindering cross-linking. CSM from seeds treated for 120 seconds exhibited higher modulus values, contributing to gel elasticity,91 a trait also observed in cold-extracted CSM.92
4.3 Microwave-assisted extraction (MAE)
Research on microwave-assisted extraction of CSM is limited, presenting a chance to investigate its potential in hydrocolloid extraction from plants. Sameera et al.95 employed microwaves for extracting CSM, applying it in biscuits as a fat replacer. CSM's without using alkaline water. This method yielded results similar to those of traditional hot and cold extraction processes,44,51,69 although CSM's functional properties were not examined. The viscosity of the microwave extracted CSM matched that reported in earlier studies.
CSM exhibits properties akin to those of basil seed mucilage. Recent research has focused on using microwaves for extracting basil seed mucilage (Ocimum basilicum var. album (L.)), comparing its yield and functional properties with those by conventional techniques.110,111 Such studies have demonstrated the effectiveness of MAE in enhancing basil seed mucilage's functional qualities96 such as improved emulsion stability, water absorption capacity, foaming stability, and comparable foaming capacity to conventionally extracted basil seed mucilage. MAE-extracted mucilage displayed a more porous structure under SEM, leading to increased water absorption and quicker gelation compared to conventional methods.110
Keisandokht et al.111 also explored the extraction of basil seed polysaccharide using MAE, finding it more efficient than the conventional heat stirring method in terms of yield and extraction time. The pH levels where MAE was most effective were 4 and 11, surpassing the conventional method. MAE-extracted mucilage's higher viscosity and the degradation of functional groups in the heat stirring method suggest that MAE-extracted mucilage was superior to conventionally extracted mucilage. The viscosity and viscoelastic behaviour of MAE-extracted mucilage were superior to those of conventionally extracted mucilage. This positions MAE as a promising and efficient technique for hydrocolloid production, particularly from basil seeds.111 The research gap in this field presents further opportunities for exploration.
5 Applications of CSM in food products
CSM has been widely applied in the food industry, serving various functions such as a fat replacer, stabilizer, and emulsifier in diverse food products. The use of CSM in the food sector was comprehensively reviewed by Chiang et al.,42 which includes application in cake, bread, cookies, dairy products (ice cream and yoghurt), mayonnaise, sausage, pasta, etc. Soukoulis et al.11 discussed the combined usage of plant seed mucilage and CSM in products such as bread, cake, pita bread, ice cream, emulsions, edible filmm, and CS oil-enriched nanoparticles. Senna et al.112 recently highlighted the feasibility of chia seed ingredients, including CSM in meat analogues. Lira et al.1 reviewed the incorporation of CSM in soy-based desserts, wheat bread, pita bread, and cookies. Fernandes et al.113 focused on CSM as a carrier in emulsions, encapsulations, coatings, and films, noting its role in retaining hydrophilic compounds when mixed with alginate.114
The development of edible coatings from CSM, chitosan, and levan (a naturally occurring fructan present in many plants and microorganism) based composites has been effective in preserving the postharvest freshness of sweet cherries, extending their shelf life,115 and maintaining the physical and chemical integrity of tomatoes during storage.116 CSM's role in packaging films117 has been noted for preserving the flavour, aroma, sweet taste, homogeneity, and shine of passion fruit nectar118 and extending the shelf life of perishable mushrooms when used with essential oils.119 Its potential in dysphagia management was explored through soup preparation, aiming to tailor products for dysphagia patients modifying their textural, rheological, viscoelastic, and sensory attributes, as well as their characteristics during oral processing characteristics.8 The mixture of CSM and levan has also been effective in preserving the antibacterial properties of nanocomposite carbohydrate polymer films, with applications in industrial and medical fields.120 Barazandegan et al.121 briefly discussed CSM's role as a prebiotic and fat replacer in the baking industry, dairy industry, and meat industry. Additionally, CSM has been utilised in fast-dissolving tablets122 and characterized for its versatile roles in the food, cosmetic and pharmaceutical industries.53
6 Future perspectives in the application of non-thermal technologies
Non-thermal technologies are emerging as pivotal in ensuring food safety and extending shelf life, significantly transforming the food processing sector.123 These technologies are effective in terms of nutritional value and freshness and utilise by-products from various sources. They offer a substantial, chemical-free alternative to traditional heat treatments. Research can be directed towards the effects of these non-thermal technologies on deoiled chia seed flour, particularly focusing on chia seed mucilage. It is essential to validate the presence of bioactive, phenolic, and flavonoid compounds in CSM obtained through these methods. Exploring the functional properties of CSM, extracted via non-thermal and conventional methods, and its potential uses in the food industry is another area of interest. This research may also reveal how non-thermal technologies alter the digestion patterns of CSM, providing new directions for future studies. Adopting suitable non-thermal and drying technologies can mitigate the negative effects of food processing and ingredient development on the environment, energy, and economy.124 Overall, the full potential of these technologies remains largely unexplored and could be further revealed by optimizing extraction parameters in future studies.
7 Conclusion
This review has detailed the impact of non-thermal technologies employed in the extraction process of CSM. When combined with centrifugation, these technologies offer a means to avoid oxidative damage to seeds without relying on dry-fractionation methods. It has been established that non-thermal technologies are preferable to thermal treatments due to their ability to maintain the integrity of the hydrocolloid, thereby broadening its potential applications. This is because the quality of CSM is influenced by many factors, ranging from its hydration to the chosen recovery method. Additionally, the rheological properties show variation across different non-thermal technologies. Hence, before scaling up these technologies for commercial use, it is crucial to prioritize process optimization, particularly to achieve more efficient utilization of water and energy during the extraction process.
Data availability
All data generated or analysed during this study are included in this published article.
Author contributions
Conceptualization; writing the draft: Divyang Solanki and Indrawati Oey; writing, editing, and reviewing: Indrawati Oey, Sangeeta Prakash, Bhesh Bhandari, and Jatindra K. Sahu.
Conflicts of interest
All the authors declare that there is no conflict of interest.
Acknowledgements
The first author acknowledges the Department of Food Science, Otago University, Dunedin, New Zealand, for providing an opportunity to work on non-thermal technology.
References
- M. M. Lira, J. G. d. Oliveira Filho, T. L. d. Sousa, N. M. d. Costa, A. C. Lemes, S. S. Fernandes and M. B. Egea, Food Res. Int., 2023, 169, 112822 CrossRef CAS PubMed.
- E. Dickinson, Food Hydrocolloids, 2003, 17, 25–39 CrossRef CAS.
- S. Y. Kim, O. Hyeonbin, P. Lee and Y.-S. Kim, J. Dairy Sci., 2020, 103, 1324–1336 CrossRef CAS PubMed.
- P. Methacanon, A. Kongjaroen and C. Gamonpilas, Food Hydrocolloids, 2023, 143, 108903 CrossRef CAS.
- K. Yang, R. Xu, X. Xu and Q. Guo, Foods, 2022, 11, 282 CrossRef CAS PubMed.
- A. H. Al-Jobouri, J. Agric. Sci., 2020, 12, 14–23 Search PubMed.
- S. Yousuf and S. S. Maktedar, Sustainable Food Technol., 2023, 1, 107–115 RSC.
- S. Ribes, R. Grau and P. Talens, Food Hydrocolloids, 2022, 123, 107171 CrossRef CAS.
- S. Ribes, M. Gallego, J. M. Barat, R. Grau and P. Talens, J. Funct. Foods, 2022, 89, 104943 CrossRef CAS.
- S. Ribes, N. Peña, A. Fuentes, P. Talens and J. M. Barat, J. Dairy Sci., 2021, 104, 2822–2833 CrossRef CAS PubMed.
- C. Soukoulis, C. Gaiani and L. Hoffmann, Curr. Opin. Food Sci., 2018, 22, 28–42 CrossRef.
- H. Cakmak, H. Ilyasoglu-Buyukkestelli, E. Sogut, V. H. Ozyurt, C. E. Gumus-Bonacina and S. Simsek, Food Hydrocolloids Health, 2023, 3, 100131 CrossRef CAS.
- I. A. A. Kassem, T. Joshua Ashaolu, R. Kamel, N. A. Elkasabgy, S. M. Afifi and M. A. Farag, Food Funct., 2021, 12, 4738–4748 RSC.
- G. Goksen, D. Demir, K. Dhama, M. Kumar, P. Shao, F. Xie, N. Echegaray and J. M. Lorenzo, Int. J. Biol. Macromol., 2023, 230, 123146 CrossRef CAS PubMed.
- L. A. Silva, P. Sinnecker, A. A. Cavalari, A. C. K. Sato and F. A. Perrechil, Food Chem., 2022, 1, 100024 CrossRef.
- C. de Campo, P. P. dos Santos, T. M. H. Costa, K. Paese, S. S. Guterres, A. d. O. Rios and S. H. Flôres, Food Chem., 2017, 234, 1–9 CrossRef CAS PubMed.
- M. R. I. Shishir, H. Suo, F. S. Taip, M. Ahmed, J. Xiao, M. Wang, F. Chen and K.-W. Cheng, Crit. Rev. Food Sci. Nutr., 2023, 1–23 CrossRef PubMed.
- Y. Yang, V. K. Gupta, Y. Du, M. Aghbashlo, P. L. Show, J. Pan, M. Tabatabaei and A. Rajaei, Int. J. Biol. Macromol., 2023, 124800 CrossRef CAS PubMed.
-
F. M. Marin Flores, M. J. Acevedo, R. M. Tamez, M. J. Nevero and A. L. Garay, Method for obtaining mucilage from Salvia hispánica, WO/2008/0044908, Word Internacional Property Organization, 2008.
- B. E. Campos, T. Dias Ruivo, M. R. da Silva Scapim, G. S. Madrona and R. d C. Bergamasco, LWT–Food Sci. Technol., 2016, 65, 874–883 CrossRef CAS.
- L. Li, J.-N. Yan, B. Lai, C. Wang, J.-J. Sun and H.-T. Wu, Food Hydrocolloids, 2023, 144, 108936 CrossRef CAS.
- Z.-u. Din, M. Alam, H. Ullah, D. Shi, B. Xu, H. Li and C. Xiao, Food Hydrocolloids Health, 2021, 1, 100010 CrossRef CAS.
-
V. Srivastava, US Patent Application No. 13/966756, 2014, pp. 1–7.
- M. R. Segura-Campos, N. Ciau-Solís, G. Rosado-Rubio, L. Chel-Guerrero and D. Betancur-Ancona, Agric. Sci., 2014, 5(3), 220–226 Search PubMed.
- K. C. Miranda-Ramos and C. M. Haros, Foods, 2020, 9, 1859 CrossRef CAS PubMed.
- S. Punia and S. B. Dhull, Int. J. Biol. Macromol., 2019, 140, 1084–1090 CrossRef CAS PubMed.
- L. A. Muñoz, A. Cobos, O. Diaz and J. M. Aguilera, J. Food Eng., 2012, 108, 216–224 CrossRef.
- L. Brütsch, F. J. Stringer, S. Kuster, E. J. Windhab and P. Fischer, Food Funct., 2019, 10, 4854–4860 RSC.
- H. Rostamabadi, M. Nowacka, R. Colussi, S. F. Frasson, I. Demirkesen, B. Mert, P. Singha, S. K. Singh and S. R. Falsafi, Trends Food Sci. Technol., 2023, 104208 CrossRef CAS.
- M. Radhakrishnan, S. Maqsood and K. Siliveru, Front. Sustain. Food Syst., 2023, 7, 1190320 CrossRef.
- G. López-Gámez, P. Elez-Martínez, O. Martín-Belloso and R. Soliva-Fortuny, Foods, 2021, 10, 1538 CrossRef PubMed.
- A. Farahnaky, M. Majzoobi and M. Gavahian, Foods, 2022, 11, 1003 CrossRef PubMed.
- H. B. Jadhav, U. S. Annapure and R. R. Deshmukh, Front. Nutr., 2021, 8, 657090 CrossRef PubMed.
- G. Jeevitha, R. Saravanan, A. Mittal and S. V. Kumar, Discov. Food, 2023, 3, 26 CrossRef.
- K. K. T. Goh, L. Matia-Merino, J. H. Chiang, R. Quek, S. J. B. Soh and R. G. Lentle, Carbohydr. Polym., 2016, 149, 297–307 CrossRef CAS PubMed.
- K. K. T. Goh, R. Kumar and S.-S. Wong, Funct. Foods Diet. Suppl., 2014, 187–225, DOI:10.1002/9781118227800.ch8.
-
L. M. Hernandez, Mucilage from chia seeds (Salvia hispanica): Microestructure, physico-chemical characterization and applications in food industry, Doctoral thesis, Pontificia Universidad Catolica de Chile, Santiago, Chile, 2012, pp. 1–146.
- Y. P. Timilsena, R. Adhikari, C. J. Barrow and B. Adhikari, Food Chem., 2016, 212, 648–656 CrossRef CAS PubMed.
- A. Tamargo, C. Cueva, L. Laguna, M. V. Moreno-Arribas and L. A. Muñoz, J. Funct. Foods, 2018, 50, 104–111 CrossRef CAS.
- L. L. Garcia e Silva, R. Alves Bastos, G. V. Souza Lima, L. de Souza Soares, J. Selia dos Reis Coimbra, M. Arêdes Martins and R. de Castro Santana, Food Biophys., 2022, 17, 568–574 CrossRef.
- C. Soukoulis, S. Cambier, T. Serchi, M. Tsevdou, C. Gaiani, P. Ferrer, P. S. Taoukis and L. Hoffmann, Food Hydrocolloids, 2019, 89, 542–553 CrossRef CAS.
- J. H. Chiang, D. S. M. Ong, F. S. K. Ng, X. Y. Hua, W. L. W. Tay and C. J. Henry, Trends Food Sci. Technol., 2021, 115, 105–116 CrossRef CAS.
- Y. P. Timilsena, R. Adhikari, S. Kasapis and B. Adhikari, Carbohydrate Polym., 2016, 136, 128–136 CrossRef CAS PubMed.
- Y. P. Timilsena, R. Adhikari, S. Kasapis and B. Adhikari, Int. J. Biol. Macromol., 2015, 81, 991–999 CrossRef CAS PubMed.
- W.-H. Wang, C.-P. Lu and M.-I. Kuo, Processes, 2022, 10, 519 CrossRef CAS.
- Y. P. Timilsena, B. Wang, R. Adhikari and B. Adhikari, Food Hydrocolloids, 2016, 52, 554–563 CrossRef CAS.
- L. A. Muñoz, N. Vera C, M. C. Zúñiga-López, M. Moncada and C. M. Haros, J. Food Compos. Anal., 2021, 104, 104138 CrossRef.
- M. I. Capitani, V. Y. Ixtaina, S. M. Nolasco and M. C. Tomás, J. Sci. Food Agric., 2013, 93, 3856–3862 CrossRef CAS PubMed.
- M. R. Segura-Campos, N. Ciau-Solís, G. Rosado-Rubio, L. Chel-Guerrero and D. Betancur-Ancona, Int. J. Food Sci., 2014, 2014, 241053 Search PubMed.
- R. Coorey, A. Tjoe and V. Jayasena, J. Food Sci., 2014, 79, E859–E866 CrossRef CAS PubMed.
- L. S. Tavares, L. A. Junqueira, Í. C. De Oliveira Guimarães and J. V. De Resende, J. Food Sci. Technol., 2018, 55, 457–466 CrossRef CAS PubMed.
- T. Hijazi, S. Karasu, Z. H. Tekin-Çakmak and F. Bozkurt, Foods, 2022, 11, 363 CrossRef CAS PubMed.
- I. F. da Silveira Ramos, L. M. Magalhães, C. do O Pessoa, P. M. Pinheiro Ferreira, M. dos Santos Rizzo, J. A. Osajima, E. C. Silva-Filho, C. Nunes, F. Raposo, M. A. Coimbra, A. B. Ribeiro and M. P. Costa, Ind. Crops Prod., 2021, 171, 113981 CrossRef CAS.
- K.-Y. Lin, J. R. Daniel and R. L. Whistler, Carbohydr. Polym., 1994, 23, 13–18 CrossRef CAS.
- X. Xing, Y. S. Y. Hsieh, K. Yap, M. E. Ang, J. Lahnstein, M. R. Tucker, R. A. Burton and V. Bulone, Carbohydr. Polym., 2017, 175, 231–240 CrossRef CAS PubMed.
- S. C. Orifici, M. I. Capitani, M. C. Tomás and S. M. Nolasco, J. Sci. Food Agric., 2018, 98, 4495–4500 CrossRef CAS PubMed.
- K. Saporittis, J. Marasco, R. Morales, M. J. Martinez and A. M. Pilosof, J. Sci. Food Agric., 2023 Search PubMed.
- A. Koocheki, S. A. Mortazavi, F. Shahidi, S. M. A. Razavi, R. Kadkhodaee and J. M. Milani, J. Food Process. Eng., 2010, 33, 861–882 CrossRef.
- B. T. Amid and H. Mirhosseini, Food Chem., 2012, 132, 1258–1268 CrossRef CAS PubMed.
- M. d. l. P. Salgado-Cruz, G. Calderón-Domínguez, J. Chanona-Pérez, R. R. Farrera-Rebollo, J. V. Méndez-Méndez and M. Díaz-Ramírez, Ind. Crops Prod., 2013, 51, 453–462 CrossRef CAS.
- G. Avila-de la Rosa, J. Alvarez-Ramirez, E. J. Vernon-Carter, H. Carrillo-Navas and C. Pérez-Alonso, Food Hydrocolloids, 2015, 49, 200–207 CrossRef CAS.
- A. Koocheki, A. R. Taherian, S. M. Razavi and A. Bostan, Food Hydrocolloids, 2009, 23, 2369–2379 CrossRef CAS.
- L. Li, B. Lai, J.-N. Yan, M. H. Yambazi, C. Wang and H.-T. Wu, Food Hydrocolloids, 2024, 148, 109445 CrossRef CAS.
- R. Feizi, K. K. T. Goh and A. N. Mutukumira, Int. Dairy J., 2021, 120, 105087 CrossRef CAS.
- A. M. G. Darwish, R. E. Khalifa and S. A. El Sohaimy, Alex. Sci. Exch. J., 2018, 39, 450–459 Search PubMed.
- F. Cuomo, S. Iacovino, M. C. Messia, P. Sacco and F. Lopez, Food Hydrocolloids, 2020, 105, 105860 CrossRef.
- D. A. Guzmán-Díaz, M. Z. Treviño-Garza, B. A. Rodríguez-Romero, C. T. Gallardo-Rivera, C. A. Amaya-Guerra and J. G. Báez-González, Foods, 2019, 8, 677 CrossRef PubMed.
- M. Dick, T. M. H. Costa, A. Gomaa, M. Subirade, A. d. O. Rios and S. H. Flôres, Carbohydr. Polym., 2015, 130, 198–205 CrossRef CAS PubMed.
- M. I. Capitani, L. J. Corzo-Rios, L. A. Chel-Guerrero, D. A. Betancur-Ancona, S. M. Nolasco and M. C. Tomás, J. Food Eng., 2015, 149, 70–77 CrossRef.
- U. Urbizo-Reyes, M. F. San Martin-González, J. Garcia-Bravo, A. López Malo Vigil and A. M. Liceaga, Food Hydrocolloids, 2019, 97, 105187 CrossRef CAS.
- P. Sacco, S. Lipari, M. Cok, M. Colella, E. Marsich, F. Lopez and I. Donati, Gels, 2021, 7 CrossRef CAS PubMed.
- M. A. Chaves, J. Piati, L. T. Malacarne, R. E. Gall, E. Colla, P. R. S. Bittencourt, A. H. P. de Souza, S. T. M. Gomes and M. Matsushita, J. Food Sci. Technol., 2018, 55, 4148–4158 CrossRef CAS PubMed.
-
A. Farahnaky, M. Majzoobi and S. Bakhshizadeh-Shirazi, Emerging Natural Hydrocolloids, 2019, pp. 451–472, DOI:10.1002/9781119418511.ch18.
- M. H. F. Felisberto, A. L. Wahanik, C. R. Gomes-Ruffi, M. T. P. S. Clerici, Y. K. Chang and C. J. Steel, LWT–Food Sci. Technol., 2015, 63, 1049–1055 CrossRef CAS.
- S. Zha, Q. Zhao, J. Chen, L. Wang, G. Zhang, H. Zhang and B. Zhao, Carbohydrate Polym., 2014, 111, 584–587 CrossRef CAS PubMed.
- M. Segura-Campos, Z. Acosta-Chi, G. Rosado-Rubio, L. Chel-Guerrero and D. Betancur-Ancona, Food Sci. Technol., 2014, 34, 701–709 CrossRef.
-
F. H. Stephenson, Calculations for Molecular Biology and Biotechnology, ed. F. H. Stephenson, Academic Press, Boston, 3rd edn, 2016, pp. 431–438, DOI:10.1016/B978-0-12-802211-5.00012-6.
-
Z. Berk, Food Process Engineering and Technology, ed. Z. Berk, Academic Press, San Diego, 2nd edn, 2013, pp. 241–257, DOI:10.1016/B978-0-12-415923-5.00009-5.
- Z. Xiao, C. Yan, C. Jia, Y. Li, Y. Li, J. Li, X. Yang, X. Zhan and C. Ma, Food Chem.: X, 2023, 20, 101011 CAS.
- M. I. Capitani, S. M. Nolasco and M. C. Tomás, Food Hydrocolloids, 2016, 61, 537–546 CrossRef CAS.
- D. Solanki, S. Prakash, N. Hans, T. Nagpal, S. Satheeshkanth, J. K. Sahu and B. Bhandari, Food Hydrocolloids, 2023, 108883 CrossRef CAS.
- G. Ferraro, E. Fratini, P. Sacco, F. Asaro, F. Cuomo, I. Donati and F. Lopez, Food Hydrocolloids, 2022, 129, 107614 CrossRef CAS.
- A. K. F. I. Câmara, P. K. Okuro, R. L. d. Cunha, A. M. Herrero, C. Ruiz-Capillas and M. A. R. Pollonio, Lebensm. Wiss. Technol., 2020, 125, 109193 CrossRef.
- E. Gençdağ, A. Görgüç and F. M. Yılmaz, Food Rev. Int., 2021, 37, 447–468 CrossRef.
- A. Görgüç, C. Bircan and F. M. Yılmaz, Food Chem., 2019, 283, 637–645 CrossRef PubMed.
- N. Castejón, P. Luna and F. J. Señoráns, J. Agric. Food Chem., 2017, 65, 2572–2579 CrossRef PubMed.
- S. A. Mir, M. A. Shah and M. M. Mir, Food Bioprocess Technol., 2016, 9, 734–750 CrossRef CAS.
- R. Thirumdas, D. Kadam and U. S. Annapure, Food Biophys., 2017, 12, 129–139 CrossRef.
- B. Kopuk, R. Gunes and I. Palabiyik, Food Chem., 2022, 382, 132356 CrossRef CAS PubMed.
- A. Segat, N. N. Misra, P. J. Cullen and N. Innocente, Innovat. Food Sci. Emerg. Technol., 2015, 29, 247–254 CrossRef CAS.
- S. Mutlu, I. Palabiyik, B. Kopuk, R. Gunes, E. Boluk, U. Bagcı, D. Özmen, O. S. Toker and N. Konar, Food Biosci., 2023, 103178, DOI:10.1016/j.fbio.2023.103178.
- S. Mutlu, B. Kopuk and I. Palabiyik, Foods, 2023, 12, 1563 CrossRef CAS PubMed.
- L. Wang and C. L. Weller, Trends Food Sci. Technol., 2006, 17, 300–312 CrossRef CAS.
- L. Felkai-Haddache, H. Remini, V. Dulong, K. Mamou-Belhabib, L. Picton, K. Madani and C. Rihouey, Food Bioprocess Technol., 2016, 9, 481–492 CrossRef CAS.
- N. Sameera and D. Subba Rao, Int. J. Math. Eng. Res., 2020, 9(7), 922–927 Search PubMed.
- J.-F. Fabre, E. Lacroux, R. Valentin and Z. Mouloungui, Ind. Crops Prod., 2015, 65, 354–360 CrossRef CAS.
- I. Tomasevic, V. Heinz, I. Djekic and N. Terjung, Ital. J. Anim. Sci., 2023, 22, 857–866 Search PubMed.
- F. Donsì, G. Ferrari and G. Pataro, Food Eng. Rev., 2010, 2, 109–130 Search PubMed.
- S. M. M. Duque, S. Y. Leong, D. Agyei, J. Singh, N. Larsen, K. Sutton and I. Oey, Appl. Sci., 2022, 12, 10293 CrossRef CAS.
- S. Melchior, S. Calligaris, G. Bisson and L. Manzocco, Food Bioprocess Technol., 2020, 13, 2145–2155 CrossRef CAS.
- B. Y. Xiang, M. O. Ngadi, L. A. Ochoa-Martinez and M. V. Simpson, Food Bioprocess Technol., 2011, 4, 1341–1348 CrossRef CAS.
- B. Xiang, M. Ngadi, B. Simpson and M. Simpson, J. Food Process. Preserv., 2011, 35, 563–570 CrossRef CAS.
- S. Zhang, L. Sun, H. Ju, Z. Bao, X.-a. Zeng and S. Lin, Food Res. Int., 2021, 139, 109914 Search PubMed.
-
H. Vega-Mercado, M. M. Gongora-Nieto, G. V. Barbosa-Canovas and B. G. Swanson, Handbook of Food Preservation, CRC Press, 2007, pp. 801–832 Search PubMed.
- S. Ma and Z.-h. Wang, Carbohydr. Polym., 2013, 92, 1700–1704 CrossRef CAS PubMed.
- W.-b. Luo, Z. Han, X.-a. Zeng, S.-j. Yu and J. F. Kennedy, Innovat. Food Sci. Emerg. Technol., 2010, 11, 587–591 CrossRef CAS.
- Z. Han, X. A. Zeng, N. Fu, S. J. Yu, X. D. Chen and J. F. Kennedy, Carbohydr. Polym., 2012, 89, 1012–1017 CrossRef CAS PubMed.
- A. Farahnaky, S. Bakhshizadeh-Shirazi, G. Mesbahi, M. Majzoobi, E. Rezvani and G. Schleining, Innovat. Food Sci. Emerg. Technol., 2013, 20, 182–190 CrossRef CAS.
- J.-J. Zou, C.-J. Liu and B. Eliasson, Carbohydrate Polym., 2004, 55, 23–26 CrossRef CAS.
- M. Shiehnezhad, S. Zarringhalami and N. Malekjani, J. Food Process. Preserv., 2023, 2023 Search PubMed.
- S. Keisandokht, N. Haddad, Y. Gariepy and V. Orsat, Food Hydrocolloids, 2018, 74, 11–22 Search PubMed.
- C. Senna, L. Soares, M. B. Egea and S. S. Fernandes, Molecules, 2024, 29, 440 CrossRef CAS PubMed.
- S. S. Fernandes, P. da Silva Cardoso, M. B. Egea, J. P. Quintal Martínez, M. R. Segura Campos and D. M. Otero, Food Res. Int., 2023, 172, 113125 CrossRef CAS PubMed.
- M. d. J. Perea-Flores, H. F. Aguilar-Morán, G. Calderón-Domínguez, A. B. García-Hernández, M. Díaz-Ramírez, H. E. Romero-Campos, A. D. J. Cortés-Sánchez and M. d. l. P. Salgado-Cruz, Appl. Sci., 2023, 13, 1213 Search PubMed.
- M. Mujtaba, Q. Ali, B. A. Yilmaz, M. Seckin Kurubas, H. Ustun, M. Erkan, M. Kaya, M. Cicek and E. T. Oner, Food Chem., 2023, 416, 135816 CrossRef CAS PubMed.
- Q. Ali, M. S. Kurubas, M. Mujtaba, A. W. Nazari, A. Dogan, M. Kaya, E. T. Oner, B. A. Yilmaz and M. Erkan, Sci. Hortic., 2024, 323, 112500 CrossRef CAS.
- L. Jiang and K. Zheng, Food Hydrocolloids, 2023, 144, 109050 CrossRef CAS.
- A. d. C. C. Soares, M. B. F. B. Tavares, E. d. P. M. Ortega, M. C. T. R. Vidigal and R. d. C. Santana, Int. J. Gastron. Food Sci., 2024, 35, 100849 Search PubMed.
- A. M. Faraj and M. Nouri, Food Packag. Shelf Life, 2024, 41, 101232 CrossRef CAS.
- G. Koşarsoy Ağçeli, Int. J. Biol. Macromol., 2022, 218, 751–759 CrossRef PubMed.
-
Y. Barazandegan, Extraction, Optimization, and Characterization of Chia Seed Mucilage as an Emerging Biopolymer, Doctoral dissertation, University of Missouri-Columbia, 2023, pp. 1–124.
- R. Madaan, R. Bala, S. K. Zandu and I. Singh, Acta Pharm. Sci., 2020, 58 CAS.
- F. M. Allai, Z. R. A. A. Azad, N. A. Mir and K. Gul, Appl. Food Res., 2023, 3, 100258 CrossRef CAS.
- A. Martynenko and G. N. A. Vieira, Sustainable Food Technol., 2023, 1, 629–640 RSC.
|
This journal is © The Royal Society of Chemistry 2024 |
Click here to see how this site uses Cookies. View our privacy policy here.