Sustainable nanofiber synthesis from corn protein meal for enhanced vitamin E and curcumin nutrient delivery in food systems†
Received
11th December 2023
, Accepted 17th May 2024
First published on 3rd June 2024
Abstract
Corn protein meal (corn gluten meal) is a byproduct of the cornstarch industry, and it has low solubility and low bioavailability. In the present study, nanofibers (NFs) were synthesized by electrospinning technique from the corn protein meal (CPM) with the necessary daily percentages of vitamin E and curcumin, to serve as a nutrient delivery vehicle for food systems. Thereafter, the physicochemical properties of developed nanofibers were characterized by SEM, FTIR, UV, and TGA, and their encapsulation efficiency, zeta potential, and size were studied. According to findings, with the incorporation of vitamin E, NFs are much thinner and more uniform in comparison to other combinations. It was also observed that corn protein meal NFs can encapsulate vitamin E and curcumin. This study validates the successful preparation of CPM NFs incorporating vitamin E and curcumin. These nanofibers have the potential to be used as a nutrient delivery vehicle for the food industry at a commercial scale.
Sustainability spotlight
The synthesis of sustainable nanofibers produced from corn protein meal, a byproduct of the cornstarch manufacturing process, shows a sustainable utilisation of cornstarch industrial byproducts. The transport of curcumin and vitamin E via nanofibers derived from corn protein meal enhances the bioavailability of nutrients within food systems. The successful production of these nanofibers not only validates their potential but also aligns with the U.N. Sustainable Development Goal 12, which advocates for the food industry to develop sustainable practices in manufacturing and consumption.
|
1. Introduction
It is well known that plant proteins, which are usually produced from plants or agricultural waste, are affordable, highly biocompatible and have low immunogenicity. Some of these proteins, such as soy protein, gluten (wheat) and zein (corn protein), are being used in the packaging industry for food items, and their potential for use in health applications is also being explored. Corn protein meal (CPM), also known as corn gluten meal, is a byproduct of the cornstarch manufacturing process. It has high zein concentration, which has been extensively investigated for biomedical applications due to its properties such as antibacterial resistance, adaptability, hydrophobicity, antioxidant activity and toughness1,2. Encapsulation carriers such as nanofibers are stable polymer-drug carriers with various features that meet the needs of a wide range of applications, including optimized porosity for controlled release and superior surface-to-volume ratios for loading and cell contact. The most researched method for the practical and scalable production of nanofibers is electrospinning (ES). This method allows for the creation of virgin and composite fibers in various designs, utilizing a large variety of polymers (synthetic and natural). The desirable properties of electrospun protein fibers (EPF) are solvolysis, low toxicity, biocompatibility, and degradability.3,4 Miyoshi et al.5 first used electrospinning to synthesise nanofibers (NF) and later examined the link between the electric potential energy and inverse ratio of polymer concentration, as well as their impacts on the characteristics of NFs. The amount of zein in solution was estimated to be between 18% and 60%. In comparison to an alkaline solution, which produces mold-fiber beaded formations, a previous study revealed that an acidic solution of zein can produce smoother NFs.6
Incorporating nanofibers with vitamins not only adds to their value but also has beneficial impacts on human health. Vitamins are bioactive molecules that serve as prosthetic groups on proteins and hormones. Thus, they are intimately linked to metabolism and advantageous for human health, including the treatment of several types of infection and reduction of oxidative stress and skin disorders.7 Various natural and manmade materials have been studied as NF-based systems for curing wounds, acne, and skin disease.8 NF-based systems can help in a number of ways, such as by improving cellular respiration conditions through their excellent permeability and by offering a perfect environment for wound healing. An appropriate barrier for ulcerative fluids, which are in charge of encasing proteins and safeguarding cytokines in the wound, can be provided by the moisture that is present beneath the wound dressing, which can aid in the healing process. Another important factor for selecting right materials is the pace of vapour transfer through the wound.8 Curcumin is also widely used for medicinal purposes in a number of Asian countries. It is used in traditional medicine to treat various conditions, including anorexia, cough, liver and sinus infections, and biliary problems. In addition, curcumin has been linked to anticancer, anti-HIV, anti-viral, hepatic, and wound-healing activities.1,9 Moreover, the FDA, FAO, and WHO have all regarded curcumin and turmeric as safe products. In clinical trials, there have been no reported adverse events associated with this compound when taken orally with a dose up to 12 g per day.10
Researchers have developed several wound dressing nanocomposites using curcumin nanoparticles to better understand how diabetic ulcers, burns, and other wounds are repaired.11 They have shown huge potential for various industries, but they are challenging to incorporate into various formulations due to their low solubility and susceptibility to physicochemical deterioration during storage and processing.12 Further efforts to prepare effective solutions to preserve their efficacy and modify their physicochemical features to increase their solubility and stability are required.13 The emulsion approach has received a lot of interest in this regard in recent decades because of its ease of use and great efficiency, as well as its low cost and use of environmentally benign components.14
In the present study, a nanotechnology approach has been used to solubilize compounds that are insoluble in water, such as corn proteins, certain vitamins, curcumin, and various other materials that can be used in future for medication delivery purposes, with some of these molecules being investigated at the scaffolds of nanofibers. Currently, the available information indicates that no studies have been conducted with corn protein meal nanofiber matrix and the curcumin nano-emulsion combined with vitamin E. The novelty of the present study arises from the use of the corn protein meal to create nanofibers incorporating vitamin E and curcumin, which demonstrate improved properties and encapsulation capabilities. Therefore, this work sets out to investigate the possibility to include vitamin E and curcumin nano-emulsion in corn protein meal nanofibers, along with the preparation, characterization, and effectiveness of the nanofibers produced from corn gluten meal. The physicochemical characterization supports the potential of these nanofibers as effective nutrient delivery vehicles for commercial applications in the food industry.
2. Material and methods
2.1. Materials
Prodigy Foods Pvt. Ltd (Zirakpur, Punjab, India) provided the CPM, which was kept at room temperature (RT) in the laboratory. Standards and reagents have been purchased at analytical grade from Merck, Germany. Zein, curcumin, Tween 80, black pepper oil, glacial acetic acid, dimethylthiazol-diphenyl tetrazolium bromide (MTT), dichloro-dihydro-fluorescein diacetate (DCFH-DA), dimethyl sulfoxide (DMSO) and other chemicals were procured from CDH, India, and Hi-Media. The L929 cell line was obtained from NABI, Mohali.
2.2. Synthesis of curcumin nanoparticles (CrN)
The desired CrN were synthesized using the previously described procedure by Moghaddasi et al.15 To summarize, three steps were used to create CrN using an oil-in-water (O/W) emulsion. First, 100 mg of curcumin was stirred into the oily phase, which was a blend of oil of black pepper and the surfactant Tween 80 in a 1
:
9 (w/w) ratio. The whole solution was agitated for 2 h at RT while being magnetically stirred at 500 rpm. Homogenization of the obtained mixture was done in an ultrasonic bath for an hour to eliminate remaining air bubbles and make a uniform yellow mixture. Finally, water was added to the oil phase in the ratio of 5
:
1 (v/v) and stirred at 500 rpm for an additional half-hour to obtain the final emulsion with a concentration of 1000 mg mL−1.
2.3. Preparation of corn protein nanoparticle (CpN) solution
To prepare and optimize the CpN (corn protein nanoparticle) solution with a modified protocol of Fereydouni et al.,8 the CPM (25% w/w) was dissolved in 70%, 80%, 90% and 100% ethanol, and the ratio of solid to liquid was optimized using 1
:
5, 1
:
10, 1
:
15, 1
:
20, and 1
:
30. Then, 2.5 g ascorbic acid was added followed by 4 h of stirring at 60 °C to homogenize the solution (CpN). Following that, 5 mg mL−1 vitamin E (VE) was added and stirred for another 15 minutes, thus preparing a solution containing corn protein nanoparticles with vitamin E (CpN-V). Additionally, to keep the final concentration at 25%, the produced CpN-V solution was mixed with a 10% (v/v) CrN solution. The final solution (CpCrNV) was mixture of the corn protein nanoparticles with vitamin E (CpN-V) and nano-curcumin (CrN), for preparation of nanofiber by electrospinning. Finally, a magnetic stirrer was used to stir this solution for 12 h, then produced solutions were left at ambient temperature for a day to remove any bubbles.
2.4. Electrospinning process
The nanoparticles of the corn protein meal were woven with curcumin and vitamin E using electrospinning to synthesize the nanofibers (NFs).16 The electrospinner was fitted with a 0–30 kV voltage power supply (Super-ES2, E-Spin Nanotech, India) at Oniosome Healthcare Pvt. Ltd, Mohali. Then, 5 mL polypropylene syringes were filled with CpN, CpN-V or CpN-V-CrN solution using a 16-gauge stainless steel (SS) needle. Next, using a syringe pump connected with the positive electrode and with the voltage of electrospinning set at 10 kV, the solutions were passed through the needle at the rate of 0.3 mL h−1. At the Taylor cone, the positively charged polymer was found to move from the needle's tip to the collecting surface, which was coated with aluminum foil. The distance between the needle and collector was 12 cm. The entire electrospinning procedure was carried out in ambient conditions at RT.
2.5. Characterization of the nanoparticles and nanofibers
2.5.1. Nanoparticle size and zeta potential.
Dynamic light scattering (DLS) was used to measure the zeta potential and size of the nanoparticles (Zetasizer Nano ZS, Malvern Instruments, UK). To eliminate numerous scattering effects, samples were diluted with deionized water prior to analysis of their size distribution and zeta potential. All analyses were conducted at RT.
2.5.2. UV-visible spectrophotometric analysis.
A UV-visible spectrophotometer (UV-2600, Shimadzu, Japan) was utilized to demonstrate the preliminary detection of manufactured nanoparticles, as well as their antioxidant capabilities, stability, and absorbance spectra.
2.5.3. Surface morphology (SEM).
Scanning electron microscopy (SEM; 6000 Benchtop, Joel, Japan) was utilized to study the morphology of the corn protein meal NF with curcumin and vitamin E, including NF surfaces. Following the gold coating procedure, pictures were collected at a 20 kV accelerating voltage. NF diameters were measured with inbuilt software of the SEM machine.
2.5.4. Fourier transform-infrared spectroscopy (FT-IR).
FTIR or Fourier transform-infrared spectroscopy, was utilized to analyze the NFs woven from the nano form of zein and curcumin along with vitamin E (Cary 600 series, Agilent Technologies, USA). The acquired spectra are presented in the 4000–400 cm−1 wavenumber range.
2.5.5. Thermogravimetric analysis (TGA).
The thermal characteristics of nanofiber samples were determined using a differential scanning calorimeter (STA-8000, PerkinElmer, USA). TGA analysis was used to evaluate the formation of nanofibers and their modification in comparison with starting materials and the different combinations. Thermal testing was carried out in N2 gas across the range of 25–300 °C (10 °C min−1), with a blank pan for reference. Each sample (2.0 mg) was carefully weighed in pans of tarred aluminum.
2.5.6. XRD.
The crystallinity of curcumin and vitamin E encapsulating nanofiber composite was determined by XRD (SmartLab, Rigaku, Japan), keeping the corn gluten meal as control, at 2θ = 5–70°, with a step size of 0.017° (2θ).
2.6. Determination of encapsulation efficiency
Encapsulation efficiency (EE) is the proportion of molecules, which are effectively encapsulated during the preparation process. In this study, the proportion of curcumin and vitamin encapsulated in the corn protein meal NFs was determined by assessing the amount of encapsulating particles present in 1 cm2 of the supernatant, properly weighing it and centrifuging the NFs for 15 minutes at 15
000 g. Moreover, absorbance was assessed at 425 and 630 nm using a spectrophotometer (UV-2600, Shimadzu, Japan), and the amount of curcumin along with vitamin in the supernatant was determined using a reference graph of known concentration at prescribed absorptions. Residual curcumin and vitamin in nanofibers was used to compute the encapsulation efficiency (percentage) with the following calculation, eqn (1). We conducted all EE percent trials in triplicate, and data are presented as mean with SD. | EE (%) = (encapsulated particle/total added particles) × 100 | (1) |
2.7. Antioxidant assay
The antioxidant/anticancer potential of CGM and its nano derivatives sheets were evaluated by MTT assay using RAW-macrophage cell lines.17 The cell lines have been established at good confluence by culturing in DMEM (glucose cell culture medium) with 0.5% streptomycin/penicillin and 10% FBS, at 37 °C incubation with 90% humidity and 10% CO2. To attain confluence of 80%, cell seeding was done in a 96-well plate, which was incubated at ambient conditions to proliferate the cells for 24 h. Meanwhile, zein protein and its nano derivative sheets were UV sterilized and suspended in PBS buffer followed by sonication to make them compatible with cell culture media. After 24 h growth, cells were challenged with CGM and its derivatives and kept at 37 °C for 12–24 h. This study was carried out as a function of protein concentration, i.e., 20 and 100 μg (>0.5 and 2.0 μM). The negative control was wells without cells, and positive control was untreated cells. After successively adding 10 μL MTT (5 mg mL−1 in PBS) to each well, the culture medium was changed to 100 μL of DMSO so that formazan crystals dissolve after incubation at 37 °C for 4 h. The cells were then analyzed using a microplate spectrophotometer at wavelengths of 540 and 630 nm. All treatments were replicated 5–8 times.
2.8. Statistical analysis
The average value of replicates are reported as experimental results. ANOVA with statistical significance p < 0.05 was used for the variance analysis of data, which was then subjected to the least significant difference (LSD) test for the comparison. IBM-SPSS (Version-28, Armonk, New York) software was used to perform statistical analyses.
3. Results and discussion
The CPM is highly hydrophobic and contains a significant amount of protein; it is well suited for the direct synthesis of protein nanoparticles, making the process easy, whereas in previous studies, zein was extracted from the CPM and modified for use as a nanocarrier for a wide range of applications. This work contributes to identifying the best way to directly develop the nanoparticles from the CPM using a green solvent and incorporating other insoluble constituents such as vitamin E and curcumin to increase bioavailability and antioxidant effects to enhance its nutritional value and valorize a byproduct of the cornstarch industry.
3.1. Nanoparticles from the corn protein meal
To synthesize the nanoparticles from the corn protein meal by anti-solvent methods, the CPM was solubilised in varied ratios of ethanol. The maximum protein concentration was obtained when the corn protein meal was dissolved in 70% ethanol, providing a protein content of 2139 mg mL−1. Protein decreases as the ethanol ratio declines; however, 100% ethanol has the least amount of dissolved protein (ESI Fig. 1a†). Prior experiments have shown that solutions with concentrations less than 60% ethanol were ineffective.18
The UV-vis spectra of various ethanol concentrations were used to determine the solubility of corn protein meal (ESI Fig. 1b†). It was discovered that 70% ethanol had a maximum absorption wavelength of roughly 280 nm for the solution of the corn protein meal.19
The size of the protein nanoparticles can be influenced by the solvent/antisolvent ratio. Thus, from the various ratios of 1
:
5, 1
:
10, 1
:
15, 1
:
20, and 1
:
30, it was determined that a solvent/antisolvent ratio of 1
:
10 results in the smallest particle size of around 100 nm (ESI Fig. 1c†). The yellow colour of the corn protein meal was clearly and visibly transformed to opaque white during the synthesis of nanoparticles for the CPM (Cp) (ESI Fig. 1d†). These developed nanoparticles were generated utilising the antisolvent precipitation method, a straightforward and non-invasive process for the creation of the nanoparticles that is also environmentally safe.20 The CPM is first mixed in the solvent before being swiftly blended with the antisolvent. High supersaturation is caused by the drug solvent solution quickly diffusing into the antisolvent; this is what drives precipitation to occur.21 The imbalance of molecular interactions between the solute, solvent, and anti-solvent is the primary factor causing particle formation during anti-solvent precipitation. Precipitation occurs when solute–solute reactions at specific solvent/anti-solvent combinations are strong enough to outweigh other interactions and the entropy of mixing effects. To prevent the particles from clustering once they have formed, it is critical that there is an adequate amount of repulsion between them. The anti-solvent precipitation process has several distinct manufacturing steps, including supersaturation formation, nucleation, crystal development, and coagulation.22
The size of prepared CPM nanoparticles was 130 nm, and an apparent zeta potential of −50 mV was observed, as shown in Fig. 1a and b. To maximise the utility of these nanostructures, it is critical to understand their charge and size, as well as the effect on stability. It was observed (Fig. 1c) that at pH 7, the nanoparticles were created with a diameter of 134 nm and a negative charge of 47 mV, whereas, at pH 9, even though the particles were tiny and had a similar charge, particle settling was observed. Additionally, their further exploitation necessitates that the nanoparticles have a pH close to neutral.
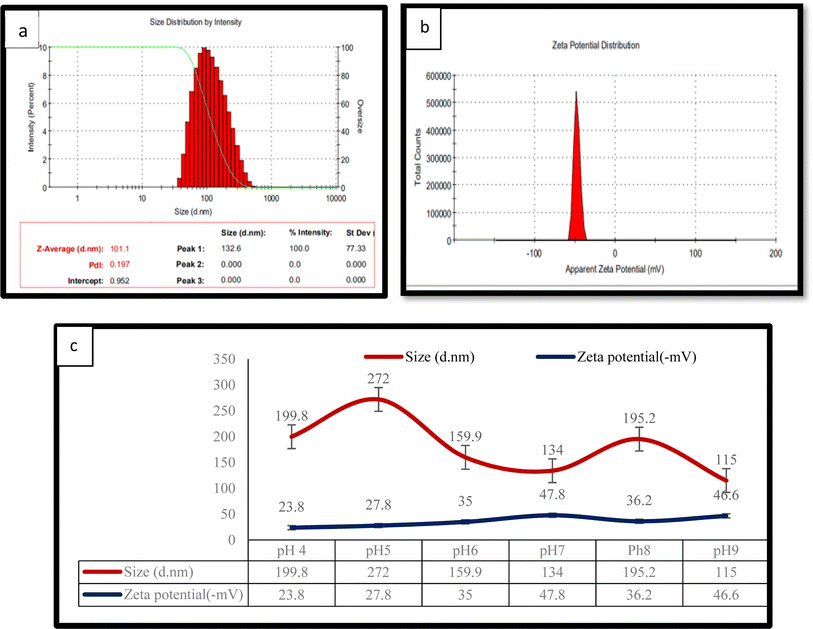 |
| Fig. 1 Nanoparticle prepared from the corn protein meal: (a) dynamic light scattering measurement, (b) zeta spectrum measurement and (c) effect of pH on the size of the nanoparticles and the zeta potential. | |
The preparation of the CPM nanoparticles was achieved successfully and can further be used for infusion of VE and CrNPs using electrospinning techniques for the development of nanofibers with additional health benefits.
3.2. Vitamin E (VE) and curcumin (CrNPs) infused nanofibers via electrospinning
Vitamin E and curcumin are proven to help the immune system perform better. However, because of their low bioavailability, these compounds must be delivered to the site of action in the body via a carrier. As a solution, the corn protein meal nanoparticles (CpN) were combined with 1% polyvinylpyrrolidone (PVP) to create a nanofiber composite with 5 mg mL−1 vitamin E and nano form of curcumin (7 mg mL−1).23,24 PVP is a large, non-ionic, non-toxic polymer containing functional groups for C
O, C–N, and CH2 and is frequently utilised in the synthesis of NPs. The pyrrolidone moiety, which is very hydrophilic, and the alkyl group, which is significantly hydrophobic, are present in the PVP molecule. Due to the extremely polar amide group inside the pyrrolidone ring and apolar methylene and methine groups inside the ring and along its backbone, water and several non-aqueous solvents are good solvents for PVP.25 In the electrospinning process, the formation of even-thickness nanofibers with the least droplet formation was achieved with 0.3 mL h−1 flow, 10 kV potential and 12 cm tip collector distance. The addition of 1% PVP was to achieve better shape of the nanofiber sheet.26 A previous study reported that as the quantity of nano curcumin was raised, the degree of porosity rises, which may be due to the induced decrease in nanofiber diameter along with better fibre homogeneity and enhanced nanofiber thickness. The sole parameters that were found to be capable of changing the diameter and expanding the thickness were a lower level of viscosity and extension of spinnability after setting up identical settings and assessing contributing components.8
The size and zeta potential of the corn protein meal nanofibers woven with vitamin E and CrNPs in sample 1 (CpN) indicated an average size of 355 nm along with a PDI of 0.3 (Fig. 2a). In sample 2 (CpN-V), the size of the nanofibers was around 400 nm with a PDI of 0.4 (Fig. 2b). Sample 3 (CpN-V-CrN) had an average of size 350 nm with PDI of 0.4. The lowest size was observed with sample 3 (Fig. 2c) due to the presence of nanoform curcumin paired with vitamin E. The zeta potential was negatively charged in all the samples. Sample 1 had a zeta potential of −10.4 mV with 9.60 mV deviation. Sample 2 had −6.64 mV zeta potential with 9.90 mV standard deviation. Sample 3 contained a zeta potential of −7 mV with 9.90 S.D., as shown in Fig. 2.
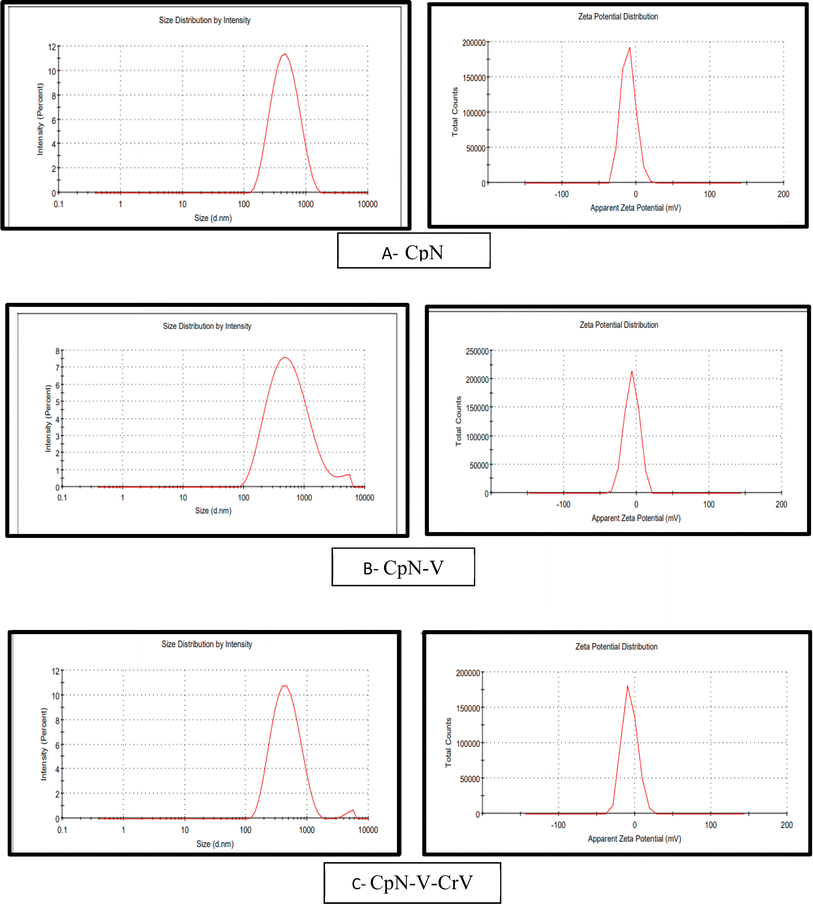 |
| Fig. 2 Dynamic light scattering measurement and zeta potential measurement after electrospinning: (A) CpN, (B) CpN-V, and (C) CpN-V-CrV. | |
3.3. Characterization of the corn protein meal nanofibers infused with VE and curcumin
3.3.1. Scanning electron microscope (SEM).
The control (CPM) appears to be a plane with an uneven surface in the SEM image. While electrospinning with the CPM nanoparticles did not result in the development of the nanofibers, as depicted in Fig. 3, there was formation of nanobeads of various sizes. It was also established that when the CPM nanoparticles mix with vitamin E, it facilitates the synthesis of the nanofibers with varying lengths and thicknesses, whereas the CPM nanoparticles with VE and CrN result in fibres with increased thickness and length. The addition of vitamin E has increased viscosity and binding forces in the formation of the nanofibers, while the nano form of curcumin has contributed to reducing the nanofibers' thickness.8
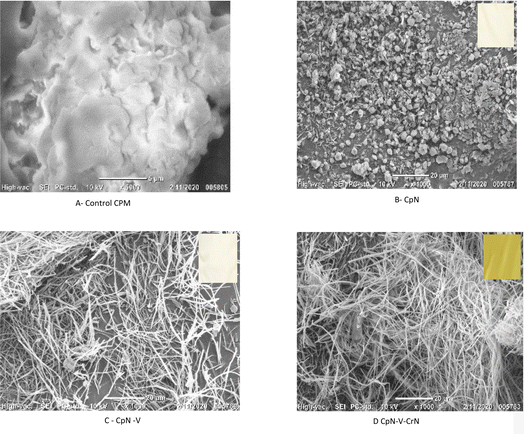 |
| Fig. 3 SEM image of the nanofibers after electrospinning the corn protein meal nanoparticles. (A) Control CPM, (B) CpN, (C) CpN woven with vitamin E and (D) CpN woven with vitamin E and CrN. | |
3.3.2. FTIR (Fourier transform-infrared spectroscopy).
To verify the availability of functional groups along with their changes within the samples, FTIR analysis was done. FTIR spectra make it very evident that the recorded absorption peaks match distinctive chemical linkages existing in corn protein meal (CPM). In sample 1 (CpN), the vibrations of the hydroxyl group were found at around 3288 cm−1 with a broad peak, and the peak of C–H vibrations was observed at 2926 cm−1. The 1537 cm−1 peak resembles C–C vibrations (amide I), and the C
O amide bond stretching peak was seen at 1648 cm−1 (amide II).27 The amide C–N stretching peak at 1243 cm−1 was observed (Fig. 4).
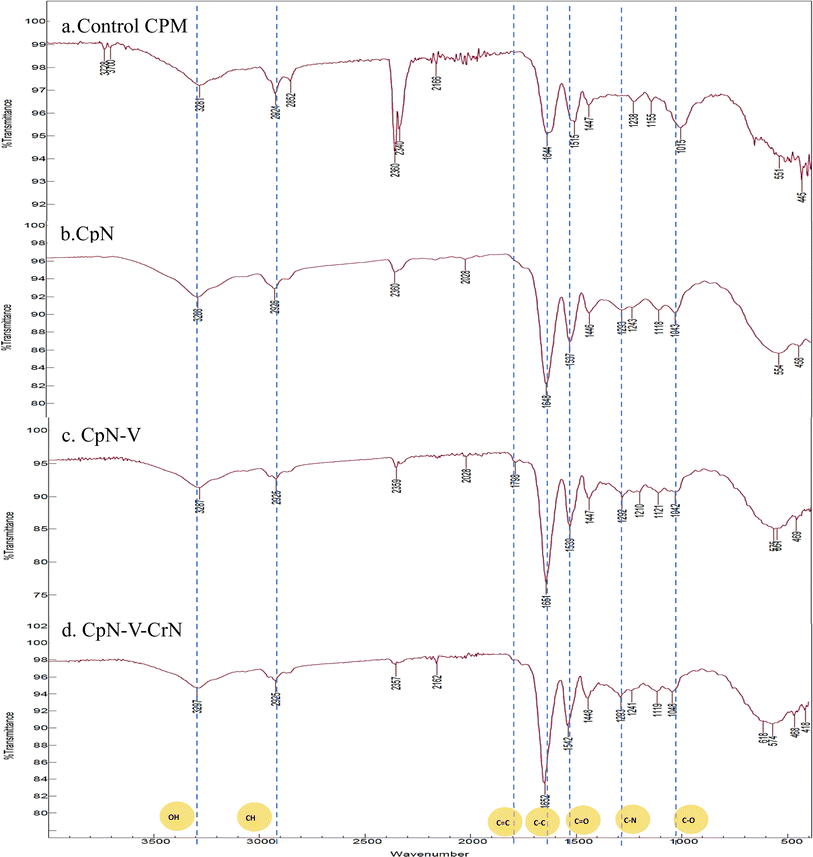 |
| Fig. 4 FTIR of (a) control CPM, (b) CpN, (c) CpN-V, and (d) CpN-V-CrV. | |
For sample 2 (CpN-V), the spectrum revealed that C
C at 1798 cm−1, C–O at 1042 cm−1, C
O at 1651 cm−1, and the C–H alkane group at 2925 cm−1 were present. However, the terminal hydroxyl group in vitamin E is responsible for the absorption band at 3287 cm−1, along with the stretching vibration of C–O at 1042–1292 cm−1. However, in sample 3 (CpN-V-CrN), the phenol hydroxyl group's stretching vibration is observed at 3297 cm−1. The inter-ring chain and keto reveals the existence of curcumin and represents the aromatic ring's stretching vibration at 1448 cm−1, 1293 cm−1, and 1119 cm−1.28
3.3.3. TGA (thermogravimetric analysis).
TGA analysis of the corn protein meal fibers prepared with corn protein meal nanoparticles woven with vitamin E and curcumin was conducted (Fig. 5), and it was observed that in the range of 200–400 °C, the value of delta Y in CpN is 40.47%; in CpN-V, 62.88%; and in CpN-V-CrN, 57.00%. However, above 700 °C, the value of delta Y in CpN is 27.51%; in CpN-V, 16.90%; and in CpN-V-CrN, 21.11%. The maximum decomposition in both the samples was observed above 200 °C.6
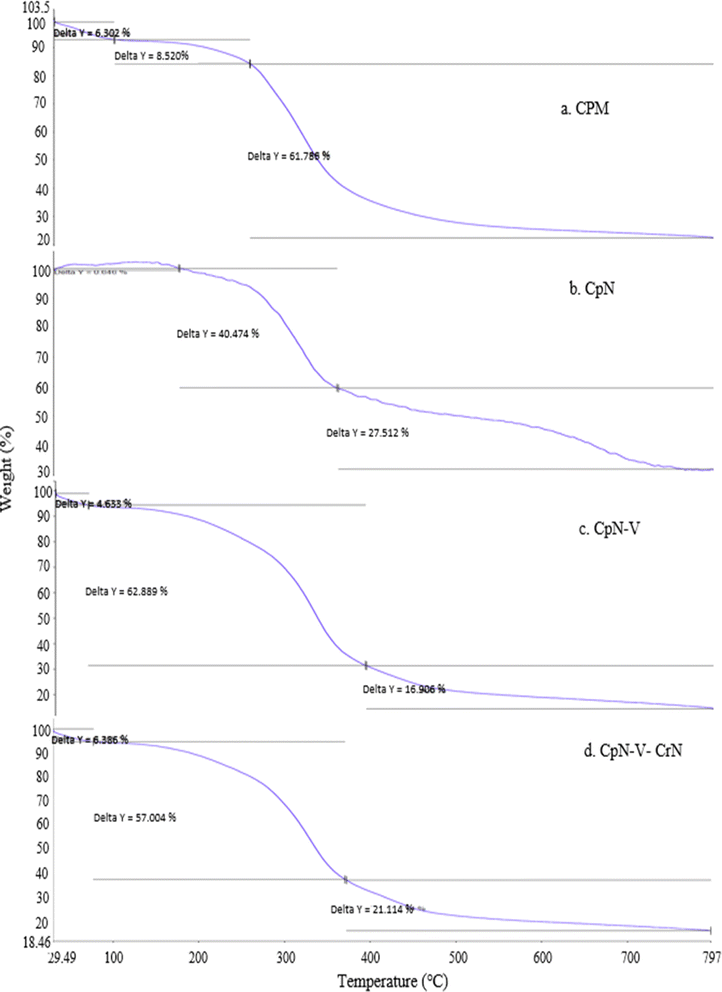 |
| Fig. 5 TGA of (a) control CPM, (b) CpN, (c) CpN-V, and (d) CpN-V-CrV. | |
3.3.4. XRD.
A change in the crystallinity of the corn gluten meal synthesized nanofibre was observed upon encapsulation of vitamin E and curcumin as compared to the control (CGM), which indicates structural changes due to binding of bioactive agents, as shown in ESI Fig. 2.† This is because superior solvents such as ethanol tend to dissolve unstructured proteins by breaking up the interactions of proteins inside and among structures.4
3.3.5. Encapsulation efficiency.
Sample 2 (CpN-V) contained 3.01 mg mL−1 vitamin E, while sample 3 (CpN-V-CrN) contained 3.04 mg mL−1 added vitamin E (which was added at 5 mg mL−1). The amount of curcumin present in the nanofiber of sample 3 was 5.16 mg mL−1 (from 7 mg mL−1 addition). This means that around 60% vitamin E and 73% curcumin are retained in the nanofibers.24 A previous study showed that carboxylic curdlans with three different chain configurations coated on zein protein nanoparticles had 67% of encapsulation efficiency of curcumin. Hydrogen bonding, electrostatic and hydrophobic interactions played a significant role between the carboxylic coated zein and curcumin nanoparticles.29
3.3.6. Antioxidant and anticancer potential.
Vitamin E and curcumin are well reported to exhibit antioxidant and thus cytotoxic potential on various cancer cell lines.30–32 Herein, we evaluated the antioxidant potential of the nanofibers in sheet form prepared by electrospinning of infused corn protein meal nanoparticles (a) CpN, (b) CpN-V, and (c) CpN-V-CrV as a function of two different concentrations (Fig. 6). At a higher dose (100 μg), all the corn protein meal-derived sheets and corn protein meal nanoparticles themselves caused significant cell death on the RAW macrophage cell line. At a lower dose (20 μg), corn protein and its derived curcumin sheet showed a favorable safety profile for cell growth, whereas corn protein-derived vitamin E, and curcumin along with vitamin E sheets, showed a much higher anticancer effect. It is interesting to note that vitamin E itself was more effective in comparison to curcumin, while curcumin had a slightly suppressed effect when given in combination with vitamin E at a higher as well as lower dose. Corn protein exhibited the lowest cell death. This study depicted that the corn protein meal and derived nanosheets demonstrate dose-dependent inhibition on cancer cell growth30,32 because of their antioxidant properties, which still remain intact when converted into nanosheets.
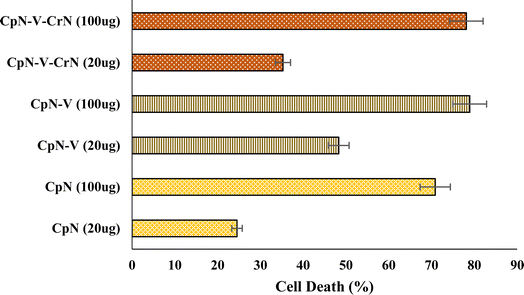 |
| Fig. 6 Cell death in RAW macrophage cells challenged with two different concentrations, i.e. 20 μg and 100 μg of CpN and its sheets (data bars are the mean of 5–8 replicates ± standard deviation, expressed as a percentage of dead cells over total counted. They are significantly different from each other and with respect to untreated cells). | |
4. Conclusion
This study demonstrated the successful fabrication of the corn protein meal nanofibers embedded with vitamin E and curcumin, showcasing their potential as a promising platform for drug delivery applications. According to the present study's data, there is feasibility of loading curcumin and vitamins into the corn protein meal nanofibers, which also shows binding efficiency with vitamin and curcumin. The observed binding efficiency, cost-effectiveness, and environmentally friendly nature of the methodology mark its significance for future biomedical advancements.
Conflicts of interest
The authors declare that there is no conflict of interest.
Acknowledgements
The authors gratefully acknowledge the support received from the Department of Biotechnology – Center of Innovative and Applied Bioprocessing (DBT-CIAB), Mohali. The authors thank the entire CIAB and NABI (National Agri Food Biotechnology Institute) for their help and cooperation during the research study.
References
- S. Tortorella, M. Maturi, V. Vetri Buratti, G. Vozzolo, E. Locatelli, L. Sambri and M. Comes Franchini, Zein as a Versatile Biopolymer: Different Shapes for Different Biomedical Applications, RSC Adv., 2021, 11(62), 39004–39026, 10.1039/D1RA07424E.
- H. Chen and Q. Zhong, A Novel Method of Preparing Stable Zein Nanoparticle Dispersions for Encapsulation of Peppermint Oil, Food Hydrocolloids, 2015, 43, 593–602, DOI:10.1016/j.foodhyd.2014.07.018.
- M. Rostami, H. Kolahi Azar, M. Salehi, S. Abedin Dargoush, H. Rostamani, G. Jahed-Khaniki and N. Rezaei, The food and biomedical applications of curcumin-loaded electrospun nanofibers: a comprehensive review, Crit. Rev. Food Sci. Nutr., 2023, 1–28 CrossRef PubMed.
- M. Rostami, N. Beheshtizadeh, F. E. Ranjbar, N. Najafi, A. Ahmadi, P. Ahmadi and S. M. Jafari, Recent advances in electrospun protein fibers/nanofibers for the food and biomedical applications, Adv. Colloid Interface Sci., 2023, 311, 102827 CrossRef CAS PubMed.
- T. Miyoshi, K. Toyohara and H. Minematsu, Preparation of Ultrafine Fibrous Zein Membranes via Electrospinning, Polym. Int., 2005, 54(8), 1187–1190, DOI:10.1002/pi.1829.
- S. Torres-Giner, E. Gimenez and J. M. Lagaron, Characterization of the Morphology and Thermal Properties of Zein Prolamine Nanostructures Obtained by Electrospinning, Food Hydrocolloids, 2008, 22(4), 601–614, DOI:10.1016/j.foodhyd.2007.02.005.
- R. Semba and M. Bloem, The Anemia of Vitamin A Deficiency: Epidemiology and Pathogenesis, Eur. J. Clin. Nutr., 2002, 56(4), 271–281, DOI:10.1038/sj.ejcn.1601320.
- N. Fereydouni, J. Movaffagh, N. Amiri, S. Darroudi, A. Gholoobi, A. Goodarzi, A. Hashemzadeh and M. Darroudi, Synthesis of Nano-Fibers Containing Nano-Curcumin in Zein Corn Protein and Its Physicochemical and Biological Characteristics, Sci. Rep., 2021, 11(1), 1902, DOI:10.1038/s41598-020-73678-w.
- I. Chattopadhyay, K. Biswas, U. Bandyopadhyay and R. K. Banerjee, Turmeric and curcumin: biological actions and medicinal applications, Curr. Sci., 2004, 87, 44–53 CAS.
- P. Basnet and N. Skalko-Basnet, Curcumin: An Anti-Inflammatory Molecule from a Curry Spice on the Path to Cancer Treatment, Molecules, 2011, 16(6), 4567–4598, DOI:10.3390/molecules16064567.
- N. K. Rajendran, S. S. D. Kumar, N. N. Houreld and H. Abrahamse, A Review on Nanoparticle Based Treatment for Wound Healing, J. Drug Delivery Sci. Technol., 2018, 44, 421–430, DOI:10.1016/j.jddst.2018.01.009.
- M. A. Augustin and L. Sanguansri, Challenges and Solutions to Incorporation of Nutraceuticals in Foods, Annu. Rev. Food Sci. Technol., 2015, 6(1), 463–477, DOI:10.1146/annurev-food-022814-015507.
- M. Martínez-Ballesta, Á. Gil-Izquierdo, C. García-Viguera and R. Domínguez-Perles, Nanoparticles and Controlled Delivery for Bioactive Compounds: Outlining Challenges for New “Smart-Foods” for Health, Foods, 2018, 7(5), 72, DOI:10.3390/foods7050072.
- D. M. dos Santos, D. S. Correa, E. S. Medeiros, J. E. Oliveira and L. H. C. Mattoso, Advances in Functional Polymer Nanofibers: From Spinning Fabrication Techniques to Recent Biomedical Applications, ACS Appl. Mater. Interfaces, 2020, 12(41), 45673–45701, DOI:10.1021/acsami.0c12410.
- F. Moghaddasi, M. R. Housaindokht, M. Darroudi, M. R. Bozorgmehr and A. Sadeghi, Synthesis of Nano Curcumin Using Black Pepper Oil by O/W Nanoemulsion Technique and Investigation of Their Biological Activities, LWT--Food Sci. Technol., 2018, 92, 92–100, DOI:10.1016/j.lwt.2018.02.023.
- M. Khatri, Z. Khatri, S. El-Ghazali, N. Hussain, U. A. Qureshi, S. Kobayashi, F. Ahmed and I. S. Kim, Zein Nanofibers via Deep Eutectic Solvent Electrospinning:
Tunable Morphology with Super Hydrophilic Properties, Sci. Rep., 2020, 10(1), 15307, DOI:10.1038/s41598-020-72337-4.
- R. Nunes, A. Baião, D. Monteiro, J. das Neves and B. Sarmento, Zein Nanoparticles as Low-Cost, Safe, and Effective Carriers to Improve the Oral Bioavailability of Resveratrol, Drug Delivery Transl. Res., 2020, 10(3), 826–837, DOI:10.1007/s13346-020-00738-z.
- Y. Li, L.-T. Lim and Y. Kakuda, Electrospun Zein Fibers as Carriers to Stabilize (−)-Epigallocatechin Gallate, J. Food Sci., 2009, 74(3), C233–C240, DOI:10.1111/j.1750-3841.2009.01093.x.
- E. E. Moros, D. Darnoko, M. Cheryan, E. G. Perkins and J. Jerrell, Analysis of Xanthophylls in Corn by HPLC, J. Agric. Food Chem., 2002, 50(21), 5787–5790, DOI:10.1021/jf020109l.
- J. Meewan, S. Somani, J. Almowalad, P. Laskar, M. Mullin, G. MacKenzie, S. Khadke, Y. Perrie and C. Dufès, Preparation of Zein-Based Nanoparticles: Nanoprecipitation versus Microfluidic-Assisted Manufacture, Effects of PEGylation on Nanoparticle Characteristics and Cellular Uptake by Melanoma Cells, Int. J. Nanomed., 2022, 17, 2809–2822 CrossRef PubMed.
- C. Y. Wu and W. Wang, Application of antisolvent precipitation method for formulating excipient-Free nanoparticles of Psychotropic drugs, Pharmaceutics, 2022, 14(4), 819 CrossRef CAS PubMed; I. J. Joye and D. J. McClements, Production of nanoparticles by anti-solvent precipitation for use in food systems, Trends Food Sci. Technol., 2013, 34(2), 109–123 CrossRef.
- M. Fathi, M. N. Nasrabadi and J. Varshosaz, Characteristics of Vitamin E-Loaded Nanofibres from Dextran, Int. J. Food Prop., 2017, 20(11), 2665–2674, DOI:10.1080/10942912.2016.1247365.
- A. Celebioglu and T. Uyar, Fast-Dissolving Antioxidant Curcumin/Cyclodextrin Inclusion Complex Electrospun Nanofibrous Webs, Food Chem., 2020, 317, 126397, DOI:10.1016/j.foodchem.2020.126397.
- K. M. Koczkur, S. Mourdikoudis, L. Polavarapu and S. E. Skrabalak, Polyvinylpyrrolidone (PVP) in nanoparticle synthesis, Dalton Trans., 2015, 44(41), 17883–17905 RSC.
- Y.-N. Jiang, H.-Y. Mo and D.-G. Yu, Electrospun Drug-Loaded Core–Sheath PVP/Zein Nanofibers for Biphasic Drug Release, Int. J. Pharm., 2012, 438(1–2), 232–239, DOI:10.1016/j.ijpharm.2012.08.053.
- D. Brahatheeswaran, A. Mathew, R. G. Aswathy, Y. Nagaoka, K. Venugopal, Y. Yoshida, T. Maekawa and D. Sakthikumar, Hybrid Fluorescent Curcumin Loaded Zein Electrospun Nanofibrous Scaffold for Biomedical Applications, Biomed. Mater., 2012, 7(4), 045001, DOI:10.1088/1748-6041/7/4/045001.
- Y. He, H. Liu, W. Bian, Y. Liu, X. Liu, S. Ma, X. Zheng, Z. Du, K. Zhang and D. Ouyang, Molecular Interactions for the Curcumin-Polymer Complex with Enhanced Anti-Inflammatory Effects, Pharmaceutics, 2019, 11(9), 442, DOI:10.3390/pharmaceutics11090442.
- N. Reddy and M. Rapisarda, Properties and applications of nanoparticles from plant proteins, Materials, 2021, 14(13), 3607 CrossRef CAS PubMed.
- B. B. Aggarwal, W. Yuan, S. Li and S. C. Gupta, Curcumin-Free Turmeric Exhibits Anti-Inflammatory and Anticancer Activities: Identification of Novel Components of Turmeric, Mol. Nutr. Food Res., 2013, 57(9), 1529–1542, DOI:10.1002/mnfr.201200838.
- D. Chin, P. Huebbe, K. Pallauf and G. Rimbach, Neuroprotective Properties of Curcumin in Alzheimer's Disease – Merits and Limitations, Curr. Med. Chem., 2013, 20(32), 3955–3985, DOI:10.2174/09298673113209990210.
- R. Zulkapli, F. Abdul Razak and R. B. Zain, Vitamin E (α-Tocopherol) Exhibits Antitumour Activity on Oral Squamous Carcinoma Cells ORL-48, Integr. Cancer Ther., 2017, 16(3), 414–425, DOI:10.1177/1534735416675950.
- A. Gagliardi, S. Voci, S. Bonacci, G. Iriti, A. Procopio, M. Fresta and D. Cosco, SCLAREIN (SCLAREol Contained in ZeIN) Nanoparticles: Development and Characterization of an Innovative Natural Nanoformulation, Int. J. Biol. Macromol., 2021, 193, 713–720, DOI:10.1016/j.ijbiomac.2021.10.184.
|
This journal is © The Royal Society of Chemistry 2024 |
Click here to see how this site uses Cookies. View our privacy policy here.