Exploring the nutritional, physicochemical and hypoglycemic properties of green banana flours from unexploited banana cultivars of southern India†
Received
29th February 2024
, Accepted 29th May 2024
First published on 30th May 2024
Abstract
The present study investigates the nutritional, phytochemical, and antioxidant potential of ten banana cultivars, in unripe form, popular in southern India, viz. Yangambi (YAN), Mysore Ethan (ME), Zanzibar (ZAN), Peyan (PEYA), Palayankodan (PALA), Malayannan (MA), Nendran (NEN), Robusta (ROB), Kappa and Monthan (MON). All cultivars in the unripe form were found to be good sources of resistant starch, with MA being the richest source (41.78 ± 1.29% d/w). The phenolic compounds, free vitamins, and amino acids were estimated and quantified using Liquid Chromatography Tandem Mass Spectrometry (LC-MS/MS). The antioxidant activity was assessed in terms of DPPH and ABTS free radical scavenging abilities. Among the varieties, MA showed higher phenolic content, whereas flavonoid content was highest for Kappa. LC-MS/MS characterisation revealed the presence of significant amounts of phenolic compounds such as shikimic acid, epicatechin, ferulic acid, and rutin; free amino acids like phenylalanine, histidine, glutamine, lysine, and arginine; and free vitamins such as pyridoxine, nicotinic acid, and ascorbic acid among the varieties. ROB and PALA demonstrated potential DPPH and ABTS radical scavenging activity, and α-amylase inhibition when compared to other cultivars. Molecular docking of shikimic and ferulic acid with α-amylase amino acid residues resulted in high binding energies of −5.45 and −5.48 kcal mol−1, respectively, confirming the hypoglycemic potential. The bioactive and nutritional potential of these cultivars, as detailed in the present study, positions them as excellent sources for food, functional food, and nutraceutical applications.
Sustainability spotlight
Research on the physicochemical and nutritional characteristics of banana flours made from underutilized southern Indian banana cultivars of stage II maturation opens up a new path for the development of sustainable food resources. By highlighting the significant amount of starch & resistant starch with appreciable presence of amino acids, vitamins, and polyphenols found in these flours, it presents their potential as a useful functional food component that can improve the nutritional value of food items, which supports United Nations Sustainable Development Goal (SDG) 2: zero hunger. This helps to ensure that everyone has access to adequate, nutritious, and secure food. Furthermore, the study aligns with SDG 3: good health and well-being, and the presence of a significant amount of resistant starch can potentially alleviate metabolic disorders such as diabetes, which would help in achieving overall health. Moreover, it also supports SDG 12 (responsible consumption and production) by promoting the efficient use of unexploited banana varieties by the food industry. In short, this study contributes to the broader mission of the UN's SDGs to achieve a more sustainable future.
|
1 Introduction
The banana, a member of the Musaceae family and belonging to the genus Musa, holds important global significance as one of the primary fruit crops. The banana ranks as the fourth major food crop globally, following wheat, rice, and maize.1 Bananas are widely harvested in tropical and semi-tropical regions.2,3 China, India, and Indonesia have consistently had the largest supply to their domestic markets since 2016.4,5 Among these, India is the largest producer of bananas, with a production of 32 million metric tonnes, which accounts for about 26.3% of the world's production in 2020. Bananas are cultivated in 150 nations in an estimated amount of 1000 different varieties. These banana varieties are further classified into desserts and plantains. Dessert bananas are consumed raw in fully ripe form, and plantains are usually cooked to be consumed at different maturity stages.6
The banana is highly valued worldwide due to its delightful flavor, nutritional value, and consistent availability throughout the year. The chemical compositions of raw bananas vary depending on the climate, soil type, variety, and degree of maturity. In raw bananas, the main component is starch, which undergoes vital changes during ripening. Starch accounts for about 70–80% of their dry weight (d/w), mostly resistant starch (RS), which is indigestible in the small intestine.7 Due to the presence of these functional ingredients, regular intake of raw banana flour is beneficial for human health.8 The higher content of RS in the diet reduces insulin responses and postprandial glucose levels in humans. Studies report a hypoglycemic effect in humans following the intake of unripe plantains.9 In unripe banana peel, the main lipophilic components present are stearyl esters and free sterols, while the pulp contains free sterols and fatty acids. Thus, the evaluation of bioactive compounds and antioxidant activity in raw bananas is of great interest. Other health benefits of bananas include aiding in improving digestion, as well as gut, heart, and kidney health, boosting immunity, helping in weight loss, and helping to fight against anemia.10,11
Considering the importance of diet in the management of chronic diseases, raw plantains are gaining attention among consumers as a healthy functional food ingredient.12,13 From this perspective, it is very important to explore the potential of underutilised and unexploited traditional varieties of local cultivars. However, there is no consolidated report on the nutritional composition of different plantain varieties. With this background, the objective of this study is the preliminary analysis of ten common but unexploited raw banana cultivars in terms of proximate composition, RS, free amino acids, vitamin content, phytochemical characterisation, antioxidant potential, and hypoglycemic effect.
2 Materials and methods
2.1 Chemicals and reagents
2,2-Diphenyl-1-picrylhydrazyl (DPPH), 2,2′-azino-bis(3-ethylbenzothiazoline-6-sulphonic acid) (ABTS), gallic acid, ascorbic acid, fetal bovine serum (FBS), Dulbecco's modified Eagle's medium (DMEM), 3-(4,5-dimethylthiazol-2-yl)-2,5-diphenyl tetrazolium bromide (MTT), dimethyl sulfoxide (DMSO), aluminum chloride (AlCl3), quercetin, α-amylase, amyloglucosidase, and protease were procured from Sigma-Aldrich Chemicals (St. Louis, MO, USA). Folin–Ciocalteu reagent, methanol, sodium carbonate (Na2CO3), sodium phosphate monobasic, sodium phosphate dibasic, and potassium acetate (CH3COOK) were purchased from MERCK, Mumbai, India. Trypsin–EDTA, antibiotic–antimycotic, starch, potassium iodide (KI), iodine (I2), hydrochloric acid (HCl), and sodium hydroxide (NaOH) were obtained from Himedia Laboratories, Mumbai, India. Formic acid, ammonium formate, and acetonitrile (MS grade) were obtained from Biosolve Ultra International, Bangalore, India. Glucose oxidase/peroxidase (GOD/POD) kits were purchased from Biosystems Diagnostics, Pvt, Ltd. Minigen Nylon syringe filters (0.20 μm diameter) were procured from Genetix Biotech Asia Pvt. Ltd, New Delhi, India. All the chemicals used were of high-quality analytical grade.
2.2 Sample preparation
Stage II raw bananas harvested from the College of Agriculture in Kerala were used for this study. Ten banana cultivars were selected: Yangambi, Mysore Ethan, Zanzibar, Peyan, Palayankodan, Malayannan, Nendran, Robusta, Kappa, and Monthan (Fig. 1); their features are summarised in ESI Table 1.† The cultivars were washed, their peel was removed, and the pulp was cut into slices and dried in a Refrigerated Adsorption Dehumidified Drier (RADD) at 45 ± 2 °C. The dried slices were ground, passed through a 50 mm mesh sieve to obtain banana flour, and stored at room temperature in an air-tight container until further analysis.
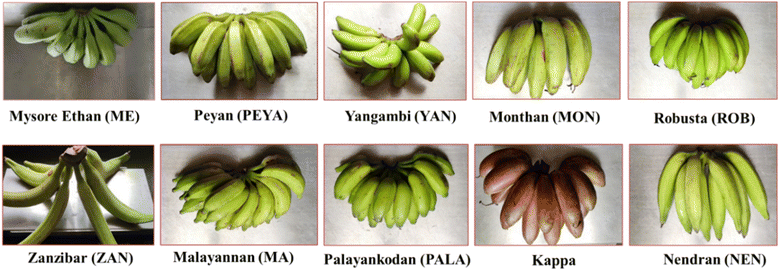 |
| Fig. 1 Ten banana cultivars. | |
2.3 Proximate composition
The moisture, ash, crude fat, and protein contents of cultivars have been determined using the standard AOAC methods (930.15, 923.03, 983.23, and 984.13).14–17 For determining the moisture content, triplicates of each sample were dried in an oven at 105 °C and then placed in a desiccator to cool to room temperature. The ash content was determined by weighing the sample both before and after heat treatment in a muffle furnace at 550 °C for 12 h. The Soxhlet extraction method was employed to measure the fat content, while protein levels were estimated using an automatic Kjeldahl distillation and titration system (KjelTRON™ Rapid Automatic Nitrogen Estimation System (Nitrogen Analyser), Tulin Equipments, model: KDIG 4M, Chennai, India). The nitrogen content was determined using the following equation, where 14 is the atomic weight of nitrogen |
 | (1) |
Then, the protein content was calculated using the equation,
|
Percentage protein = percentage nitrogen × 6.25
| (2) |
Carbohydrate content was determined using the difference method, as per the following formula:
|
Carbohydrate content = [100 − {moisture + ash + fat + protein}]
| (3) |
2.4 Isolation of starch
Starch was isolated from raw bananas using an alkaline extraction method18 with slight modifications. Initially, banana flour (5 g) was suspended in a 0.05 M NaOH solution (20 mL), and agitated continuously for 4 h. Subsequently, the mixture was centrifuged using a Kubota High-Speed Refrigerated Centrifuge (Model 7780, Kubota Corporation, Tokyo, Japan) at 956g for 15 min at 4 °C. The same procedure was repeated once again and the resulting pellet was dispersed in distilled water (50 mL), and filtered. The residue was neutralised using a 1 M HCl solution and centrifuged. The pellet was washed thrice with distilled water, centrifuged, and the final pellet was dried overnight at 45 °C. The dried product was then ground and sieved through a 50 mm mesh to obtain a fine starch powder, which was stored in an airtight container for further analysis.
2.5 Isolation of resistant starch
The method used by Zhang et al. and Escarpa et al.19,20 was slightly modified for the isolation and determination of RS from starch. Isolated starch (2 g) was mixed with 50 mL of 0.08 M phosphate buffer (pH 6) and α-amylase, incubated in a water bath shaker (Rivotek™ water bath shaker TC544, Mumbai, India) at 95 °C for 15 min, cooled and the pH was adjusted to 7.5 using 1 M NaOH. To this, phosphate buffer (2.0 mL, pH 7.5) containing protease enzyme (50 mg mL−1) was added, incubated at 60 °C for 30 min in a water bath shaker, and cooled to room temperature. The pH was adjusted to 4.5, followed by the addition of 2.5 mL of amyloglucosidase (AMG), and incubated in a water bath shaker at 60 °C for 30 min. It was allowed to cool after the incubation followed by the addition of ethanol (1
:
4 v/v) and allowed to stand overnight and centrifuged. The pellet was washed with distilled water, and freeze-dried using a lyophilizer (VirTis genesis, USA). The dried material was then ground to a fine powder. Total glucose was analysed using the GOD–POD (glucose oxidase/peroxidase) kit. The RS content was calculated using the equation RS = glucose × 0.9 (correction factor).
2.6 Phytochemical characterisation
Raw banana flour (20 g) was weighed and extracted with 100 mL of 70% ethanol by continuous stirring for 1 h; the slurry was centrifuged at 10
621g for 10 min, and the supernatant was collected. The resulting extract was filtered and evaporated using a rotary evaporator (Heidolph, Germany) at 45 °C under low pressure. The hydro-alcoholic extracts were subsequently dissolved in corresponding solvents, made up to a specific volume, and stored at 4 °C until further analysis for determining phenolic compounds, free amino acids, and vitamins.21
2.6.1 Determination of total phenolic content (TPC) and total flavonoid content (TFC). Folin–Ciocalteau and aluminum chloride colorimetric methods were used to obtain the TPC and TFC of banana flour extract, respectively.13 Briefly, different concentrations of the extracts and gallic acid (standard) were made up to 750 μL with methanol followed by the addition of Folin's reagent (80 μL) and 7.5% of sodium carbonate solution (200 μL) and kept at room temperature for 90 min in the dark. The absorbance was measured at 750 nm using a multimode plate reader (Synergy 4 BioTek, BioTek Instruments, Inc., Mumbai, India). The TPC was expressed as milligrams of gallic acid equivalent per gram of d/w (mg GAE per g).The aluminum chloride colorimetric method was used to estimate the TFC of banana flour extract. In short, various concentrations of the samples and quercetin (standard) were combined with 10 μL of 1 M CH3COOK, 10 μL of 10% AlCl3, and 280 μL of distilled water, and incubated at room temperature for 40 min. The absorbance was measured at 415 nm using the multi-plate reader. The TFC was represented as a milligram of quercetin equivalent per gram of d/w (mg QE per g).
2.6.2 DPPH and ABTS radical scavenging activity. 2,2-Diphenyl-1-picrylhydrazyl (DPPH) and 2,2′-azino-bis(3-ethylbenzothiazoline-6-sulphonic acid) (ABTS) assays were used to determine banana flour extract's free radical scavenging activity.13,22 In brief, 500 μL of 0.2 mM DPPH solution was added to 500 μL of sample and gallic acid (standard) at different concentrations and incubated at room temperature for 30 min in the dark, and its absorbance was measured at 517 nm using a multimode reader. The percentage inhibition of DPPH was determined using the following equation. The IC50 values were calculated using linear regression analysis. |
 | (4) |
For ABTS radical scavenging activity, ABTS radicals were prepared by combining 50 mL of 2 mM ABTS solution with 200 μL of 70 mM potassium persulfate. This mixture was allowed to stand in a darkroom for 12–18 h. Different concentrations of standard (ascorbic acid) and samples were prepared and made up to 250 μL using distilled water followed by the addition of ABTS solution and 850 μL of phosphate buffer (pH 7.4) and incubated at room temperature for 7 min in the dark. The absorbance was read at 734 nm using a multimode reader. The percentage inhibition of ABTS was measured using the following equation.
|
 | (5) |
The IC50 values were calculated using linear regression analysis.
2.7 Chemical profiling and quantification of raw banana flour extract using LC-MS/MS
Quantitative analysis of phenolic compounds, free amino acids, and vitamins present in the hydroalcoholic extracts (prepared as per Section 2.6) was carried out by LC-MS/MS. The extracts were filtered using a 0.20 μm syringe filter before the analysis.
2.7.1 Quantification of polyphenols. Quantitative analysis was conducted on a total of 28 compounds, including catechol, quinine, tocopherol, gallic acid, naringenin, catechin, chlorogenic acid, epicatechin, syringic acid, vanillic acid, genistein, epigallocatechin, p-coumaric acid, ferulic acid, myricetin, luteolin, apigenin, kaempferol, rutin, daidzein, quercetin, hesperetin, shikimic acid, morin, caffeic acid, cinnamic acid, and chrysin.23 Initial standardisation of the method was carried out using corresponding standard compounds. In brief, the analysis was done with an LC-MS/MS (Nexera LC-30AD) with an autosampler (SIL30AC), temperature-controlled column oven (CTO-20AC), and Prominence diode array detector (SPD-M20A), coupled to a triple quadrupole mass spectrometer. A Shimadzu Shim-pack GISS C18 column (150 × 2.1 mm i.d., 1.9 μm) was used with a mobile phase consisting of solvent A (water/formic acid, 100/0.1%) and solvent B (100% methanol). The following linear gradient system was used to elute the polyphenols: for 0.5 to 1.9 min 5% of solvent B, for 2.0 to 10.0 min and 10.1–15 min 98% of solvent B, and for 15.1–17 min 5% of solvent B, with a 0.3 mL min−1 flow rate, 40 °C oven temperature and 10 μL injection volume. During LC-MS/MS with electrospray ionisation (ESI), both positive and negative modes of the multiple reaction-monitoring (MRM) modes were operated. The Lab Solutions software (Shimadzu, Kyoto, Japan) was used to gather and process the LC-MS/MS data. Ionisation required an interface temperature of 400 °C, a desolvation line temperature of 300 °C, a heat block temperature of 400 °C, a drying gas flow rate of 10 L min−1 for nitrogen, and a nebulizing gas flow rate of 3 L min−1 for nitrogen. The average value of the data was taken as the representative for each point after every calibration solution was analysed in triplicate. The resulting data are expressed as polyphenols in μg mL−1 with SD (n = 3).
2.7.2 Quantification of amino acids and vitamins. Free amino acids (tryptophan, serine, leucine, histidine, phenylalanine, asparagine, methionine, cystine, threonine, valine, tyrosine, lysine, glutamic acid, glycine, proline, aspartic acid, glutamine, isoleucine, arginine, hydroxyproline, cysteine, and alanine) and vitamins (thiamine, pyridoxine, nicotinic acid, folic acid, riboflavin, and ascorbic acid) present in the extracts were analysed by LC-MS/MS.24 In brief, an LC-MS/MS (Nexera LC-30AD) equipped with an autosampler (SIL30AC), temperature-controlled column oven (CTO-20AC), and Prominence diode array detector (SPD-M20A), coupled to a triple quadrupole mass spectrometer (Nexera with LCMS-8045, Shimadzu Corporation, Kyoto, Japan) was used. The quantification was carried out with a Shimadzu Shim-pack GISS C18 column (150 × 2.1 mm i.d., 1.9 μm). The mobile phase used for amino acids consists of solvent A (water/formic acid, 100/0.1%) and solvent B (100% methanol). The following is the method of eluting amino acids using a linear gradient system: for 0.5–4.9 min 5% of solvent B, for 5.0–13 min and 13.1–15 min 85% and 5% of solvent B respectively, with a flow rate of 0.3 mL min−1, and 40 °C oven temperature. Both positive and negative MRM modes were used during LC-MS/MS with ESI. The ion spray voltage was 4 kV, the injection volume was 10 μL, and the collision-induced dissociation (CID) gas pressure was 230 kPa. The average value of the results was taken as the representative for each point after each calibration solution was examined thrice. The concentration of amino acids are represented as μg per mL with SD (n = 3).The mobile phase used for vitamins was 10 mM ammonium formate in water and 0.1% formic acid for solvent A and 10 mM ammonium formate in methanol and 0.1% formic acid for solvent B. A linear gradient system was employed as follows: for 0.01–2.0 min 5% of solvent B, for 2.0–5.0 min 30% of solvent B, for 5.0–8.0 min 45% of solvent B, for 8.0–12.0 min 90% of solvent B, for 12.0–16.0 min 5% of solvent B with a flow rate of 0.3 mL min−1 and 33 °C oven temperature. In both positive and negative MRM modes, ESI was performed. For ascorbic acid only, the negative mode was employed. There was a 10 μL injection volume, 4 kV ion spray voltage, and the drying and heating gas flow rate was 10 L min−1, whereas the nebulizing gas flow was 3.0 L min−1. Each calibration solution was examined in triplicate, and the average of the findings was taken as the representative for each analysis. The vitamin content is expressed as μg per mL with SD (n = 3). The analytical method was initially standardized using standard amino acids and vitamins. This standardisation ensured accurate quantification of the components in the extracts.
2.8 Cytotoxicity assay
The cytotoxicity of extracts was quantified by MTT assay using L6 myoblast cells.25 L6-rat skeletal myoblast cells (NCCS, Pune, India) were cultured in DMEM with 10% FBS and a 0.5% antibiotic–antimycotic solution and kept in an incubator at 37 °C for 24–48 h provided with 5% carbon dioxide. Cells were trypsinized and seeded into a 96-well plate. After the cell attachment, it was treated with different concentrations of extracts and incubated for 24 h at 37 °C. The cytotoxicity of cells was quantified by the MTT assay, and cell viability was calculated using a subsequent equation. |
 | (6) |
2.9 α-Amylase inhibition assay
Extracts were tested for α-amylase inhibition with modifications to Xiao et al.'s method.26 The test mixture containing 40 μL of 0.02 M sodium phosphate buffer (pH 6.9), 0.02 units of porcine pancreatic amylase (PPA) solution, and varied concentrations of extracts, was incubated at 37 °C for 10 min. After adding 1% soluble starch, it was incubated for an additional 15 min. To halt the enzyme process, 20 μL of 1 M HCl was added, followed by 100 μL of iodine reagent (5 mM I2 and 5 mM KI). Absorbance at 620 nm was measured, using acarbose as a positive control. The inhibition percentage was calculated based on the defined formula. |
 | (7) |
2.10 Molecular docking studies of compounds with α-amylase
The human pancreatic α-amylase structure (PDB ID 4W93) from the Protein Data Bank was utilized for molecular docking, with hydrogens and Kollman charges being added post-structure refinement. The structures of the LCMS/MS-identified compound were obtained from PubChem and prepared as ligands in 3D .sdf format. Energy minimization and conversion to .pdbqt format were performed using OpenBabelGUI. Autodock 4.2.6 facilitated molecular docking with 100 runs and a population size of 150, assessing ligand interactions against α-amylase. PyMol was employed for 3D visualisation of ligand interactions with α-amylase's amino acid residues. Binding energies (kcal mol−1) indicated the strength of affinity, with more negative values suggesting stronger interactions.
2.11 Statistical analysis
Statistical analysis was performed using GraphPad Prism 9.3.0, presenting results as mean ± standard deviation (SD). A one-way ANOVA and Dunnett's multiple comparison test were employed, with significance considered at p < 0.05.
3 Results and discussion
3.1 Proximate composition
Proximate analysis provides essential data on the major constituents of food products, including moisture, ash, fat, protein, and carbohydrates. This data is crucial for understanding the nutritional profile and potential applications of different food items. In the study, the proximate composition of ten banana cultivars is reported, showcasing the variance in composition that can impact both nutritional value and usability.
The proximate composition of ten varieties of banana cultivars is presented in Fig. 2a. The ash content, representing inorganic minerals, varied significantly across the cultivars, from 1.61 ± 0.35% in NEN to 3.52 ± 0.19% in Kappa. This variation suggests a considerable influence of soil mineral content and agricultural conditions on the mineral uptake by the bananas, as seen in other studies.27–29 Elsewhere, Kumar et al.30 reported 2.5% ash content for Nendran, which was higher than that in the present study. This difference could be due to the variation in geographical and climatic changes. These cultivars varied in fat content, with PALA having 1.07 ± 0.77% and Kappa having 2.89 ± 0.45%. This low fat content, as reported by Khoza et al.31 and Amini Khoozani et al.,32 is advantageous for reducing oxidative spoilage, thus extending the shelf life of banana flour. The sequence of fat content from lowest to highest (PALA, MON, MA, PEYA, ROB, YAN, ME, NEN, ZAN, Kappa) indicates that certain cultivars like Kappa might be more prone to oxidation than others like PALA, influencing storage and processing decisions.
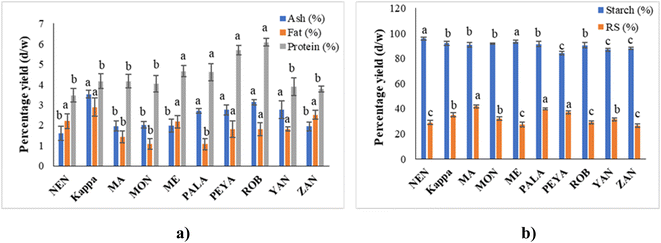 |
| Fig. 2 Proximate composition (a), and starch and RS yield (b) of banana cultivars (% d/w). Data represented are mean value ± SD, while data bars with different alphabets show significant differences (p < 0.05). | |
Protein content also showed variability, with NEN at the lowest (3.47 ± 0.63%) and ROB at the highest (6.08 ± 0.19%). These figures align closely with findings from Kumar et al.30 who reported protein contents for Monthan and Nendran that are similar to those observed in our study. Also, Khoza et al.31 reported the raw banana flour protein content within the range of the current study. Given that protein content can affect the nutritional quality of food products, cultivars like ROB could be more suitable for protein-enrichment purposes, while NEN might be preferred in situations where lower protein content is beneficial.
Proximate analysis of these banana cultivars reveals crucial differences in their chemical makeup that could inform both agricultural practices and food processing industries. For instance, selecting specific cultivars for cultivation based on soil mineral content could optimise mineral uptake (ash content), while the choice of cultivar for flour production could be influenced by desired characteristics such as longer shelf life (low fat) or specific nutritional needs (higher or lower protein content). This analysis aids in both understanding the inherent properties of these banana cultivars and utilising them for specialised food production and nutritional approaches.
3.2 Isolation of starch and resistant starch
The percentage yield of starch ranged between 84.30 ± 1.25 and 95.8 ± 1.32% dry weight (d/w) (Fig. 2b). It can be seen that the NEN variety had the highest and PEYA had the lowest content of starch. A previous study by Babu et al.33 reported 92.62% starch content in the Monthan variety, which is similar to that in the present study (91.60%). Furthermore, the plantain banana harvested from Mexico is reported to have 82.3% starch content.34 Many studies have also reported that raw banana pulp contains up to 60–80% starch.35–37 The variation in the starch content of the reported values from those in the current study may be associated with the differences in the isolation process, variety of the cultivars, maturity, and geographical origin.
The inclusion of banana starch can impact the physicochemical and pasting properties of the substance through many mechanisms, including structural interactions, interactions with proteins and lipids, water retention, and flavor.38,39 In addition to this, the electrostatic interactions within banana flour have several effects on its physicochemical properties, like viscosity, gelation, water absorption, texture, and elasticity. These interactions play a crucial role in the development of food products, such as bread, pasta, or gluten-free foods.32,40 The unripe banana contains a significant amount of starch that breaks down into sugars during ripening with the aid of ethylene-mediated regulation of starch-degrading enzymes.41
Unripe bananas are reported to have health benefits due to the high amount of indigestible polysaccharides, mainly RS. Even though many studies have discussed RS from bananas, there are no previous reports on the RS of some of the varieties (YAN, PEYA, ZAN, and ME) that have been discussed in the present study (Fig. 2b). The RS yield varied between 41.78 ± 1.29% and 26.43 ± 1.27% d/w, with MA having the highest and ZAN the lowest yields. Unripe Taiwanese bananas reported a similar yield of RS.35 Prior studies also compared the RS content of peeled and unpeeled stage II cavendish bananas, and the results showed that the RS content was higher for peeled ones (40.16%) than unpeeled ones (33.86%);42 this result supports the current study.
A recent investigation by Nguyen Minh et al.43 compared the RS content of different starchy food sources like sweet potato, black beans, red kidney beans, mung beans, corn starch, potato starch, and green banana starch and found 48.29% RS in green banana. RS resists the action of digestive enzymes in the small intestine and serves as a substrate for beneficial gut microbiota. Furthermore, RS aids in the lowering of blood cholesterol, postprandial glucose due to the low glycemic index, and in the prevention of colon cancer.44 Foods with high RS exhibit functional as well as physiological health benefits. Thus, raw banana flour could be exploited as an ingredient for the formulation of various functional foods.
3.3 Quantification of amino acids (aa) and vitamins using LC-MS/MS
Literature surveys reveal limited research on the amino acid profile of bananas, possibly due to the low protein content in green bananas. According to the US Department of Agriculture, bananas are rich in non-essential amino acids like aspartic acid and glutamic acid.45 Unripe bananas, serving as a natural source of phenylalanine, and other essential and non-essential aa contribute to overall dietary intake.46 The study confirms bananas' richness in histidine compared to other fruits.42 The study examined 22 aa in the investigated banana cultivars (Table 1), revealing phenylalanine as the predominant amino acid in all the variants studied, except for Kappa and YAN.
Table 1 Amino acid quantification in ethanolic extracts of cultivarsa
Amino acids |
Concentration in the samples (μg mL−1) |
NEN |
Kappa |
MA |
MON |
ME |
PALA |
PEYA |
ROB |
YAN |
ZAN |
Values are mean ± SD; they are followed by different alphabets in each row which indicate significantly different values (p < 0.05). The letter ‘a’ represents the highest value. |
Tryptophan |
3.10 ± 0.002h |
5.29 ± 0.001f |
6.98 ± 0.072e |
9.64 ± 0.005d |
3.31 ± 0.007g |
12.26 ± 0.003c |
12.97 ± 0.002b |
20.20 ± 0.001a |
1.40 ± 0.003i |
3.04 ± 0.006h |
Serine |
6.89 ± 0.006d |
5.11 ± 0.007f |
7.10 ± 0.002d |
5.11 ± 0.003f |
4.77 ± 0.001g |
7.55 ± 0.008c |
15.02 ± 0.043a |
10.31 ± 0.002b |
5.75 ± 0.002e |
7.43 ± 0.004c |
Leucine |
1.03 ± 0.001f |
2.19 ± 0.004c |
1.44 ± 0.009e |
1.08 ± 0.003f |
1.03 ± 0.006f |
2.74 ± 0.012b |
1.84 ± 0.007d |
6.37 ± 0.032a |
1.16 ± 0.056f |
0.98 ± 0.009f |
Histidine |
36.46 ± 0.004i |
98.26 ± 0.006c |
37.89 ± 0.002h |
47.92 ± 0.011g |
79.72 ± 0.008d |
108.06 ± 0.044b |
52.88 ± 0.014f |
142.57 ± 0.003a |
73.42 ± 0.021e |
37.88 ± 0.023h |
Asparagine |
5.44 ± 0.002f |
5.95 ± 0.005e |
6.29 ± 0.016d |
3.58 ± 0.003i |
4.61 ± 0.006g |
8.45 ± 0.018b |
6.91 ± 0.009c |
31.52 ± 0.001a |
2.32 ± 0.092j |
3.85 ± 0.007h |
Cystine |
0.38 ± 0.011e |
0.41 ± 0.014d |
0.68 ± 0.004b |
0.24 ± 0.023h |
0.31 ± 0.006g |
0.45 ± 0.001c |
0.98 ± 0.002a |
0.20 ± 0.006i |
0.16 ± 0.004j |
0.36 ± 0.009f |
Threonine |
2.25 ± 0.005g |
3.05 ± 0.003d |
2.45 ± 0.039f |
2.00 ± 0.036h |
1.96 ± 0.002h |
4.39 ± 0.017c |
5.19 ± 0.008b |
8.22 ± 0.023a |
1.64 ± 0.003i |
2.99 ± 0.002e |
Valine |
1.93 ± 0.006f |
2.03 ± 0.053e |
2.54 ± 0.002d |
1.64 ± 0.007h |
1.73 ± 0.016g |
2.90 ± 0.004c |
4.30 ± 0.008b |
5.003 ± 0.033a |
1.63 ± 0.014h |
1.75 ± 0.005g |
Phenylalanine |
204.17 ± 0.025e |
66.06 ± 0.014j |
366.17 ± 0.008b |
162.42 ± 0.004g |
96.45 ± 0.055h |
241.16 ± 0.020d |
495.67 ± 0.011a |
352.49 ± 0.006c |
72.65 ± 0.005i |
177.00 ± 0.003f |
Glutamic acid |
1.002 ± 0.008f |
1.15 ± 0.001e |
2.06 ± 0.036c |
2.17 ± 0.006b |
1.12 ± 0.007e |
2.06 ± 0.023c |
7.24 ± 0.008a |
2.06 ± 0.014c |
1.04 ± 0.025f |
1.62 ± 0.006d |
Glycine |
0.68 ± 0.002e |
0.69 ± 0.006e |
1.03 ± 0.016d |
0.704 ± 0.001e |
0.67 ± 0.088e |
1.32 ± 0.002c |
1.83 ± 0.004b |
2.41 ± 0.045a |
0.45 ± 0.019f |
0.93 ± 0.076d |
Proline |
1.52 ± 0.036g |
1.11 ± 0.016j |
2.35 ± 0.005d |
2.15 ± 0.001e |
1.48 ± 0.005h |
3.59 ± 0.022c |
5.97 ± 0.025a |
5.46 ± 0.006b |
1.20 ± 0.004i |
1.64 ± 0.002f |
Aspartic acid |
5.39 ± 0.008h |
4.95 ± 0.004i |
4.48 ± 0.003j |
13.52 ± 0.009c |
5.88 ± 0.016g |
8.53 ± 0.003e |
36.85 ± 0.064a |
15.70 ± 0.002b |
10.19 ± 0.013d |
7.39 ± 0.001f |
Glutamine |
38.11 ± 0.005h |
41.61 ± 0.016g |
88.99 ± 0.032e |
99.01 ± 0.046c |
35.93 ± 0.025i |
95.510 ± 0.009d |
345.94 ± 0.004a |
118.83 ± 0.012b |
29.13 ± 0.003j |
54.45 ± 0.008f |
Lysine |
27.74 ± 0.012h |
30.99 ± 0.002g |
64.04 ± 0.005e |
71.77 ± 0.017c |
25.96 ± 0.004i |
69.75 ± 0.077d |
246.72 ± 0.064a |
87.10 ± 0.002b |
21.22 ± 0.005j |
39.47 ± 0.003f |
Tyrosine |
1.53 ± 0.021g |
2.07 ± 0.005d |
1.77 ± 0.001f |
1.97 ± 0.006e |
0.87 ± 0.071j |
3.00 ± 0.002a |
2.37 ± 0.007b |
2.31 ± 0.008c |
1.29 ± 0.009i |
1.37 ± 0.001h |
Isoleucine |
1.12 ± 0.032h |
2.45 ± 0.004c |
1.59 ± 0.009e |
1.26 ± 0.006f |
1.17 ± 0.033g |
3.19 ± 0.002b |
2.28 ± 0.009d |
7.38 ± 0.003a |
1.25 ± 0.005f |
1.11 ± 0.029h |
Arginine |
64.65 ± 0.005g |
33.48 ± 0.002i |
23.29 ± 0.032j |
70.68 ± 0.004d |
44.71 ± 0.015h |
75.64 ± 0.004c |
64.97 ± 0.007f |
144.18 ± 0.041a |
66.10 ± 0.037e |
116.91 ± 0.001b |
Hydroxyproline |
1.00 ± 0.014f |
2.19 ± 0.064c |
1.44 ± 0.003e |
1.10 ± 0.052f |
1.07 ± 0.099f |
2.74 ± 0.001b |
1.95 ± 0.004d |
6.37 ± 0.009a |
1.12 ± 0.003f |
0.99 ± 0.002f |
Cysteine |
1.200 ± 0.003e |
1.570 ± 0.024d |
1.630 ± 0.007c |
0.803 ± 0.008g |
1.180 ± 0.062e |
1.850 ± 0.017a |
1.650 ± 0.003c |
1.790 ± 0.002b |
1.20 ± 0.005e |
1.130 ± 0.001f |
Alanine |
0.51 ± 0.005e |
0.14 ± 0.023g |
0.58 ± 0.006e |
0.46 ± 0.024e |
0.26 ± 0.006f |
0.86 ± 0.002d |
1.74 ± 0.081b |
2.05 ± 0.004a |
0.28 ± 0.008f |
1.07 ± 0.074c |
Phenylalanine dominated in NEN (204.17 ± 0.025 μg mL−1), followed by arginine (64.65 ± 0.005 μg mL−1). In Kappa, histidine was the prominent aa (98.26 ± 0.006 μg mL−1), followed by phenylalanine (66.06 ± 0.014 μg mL−1). MA featured the highest phenylalanine (366.17 ± 0.008 μg mL−1) and lysine content (64.04 ± 0.005 μg mL−1). MON displayed amino acids in the order phenylalanine > glutamine > lysine > arginine > histidine, with 162.42 ± 0.004 μg mL−1 phenylalanine. ME showed the highest phenylalanine (96.45 ± 0.055 μg mL−1) and histidine content (79.72 ± 0.008 μg mL−1). PALA had phenylalanine at 241.16 ± 0.020 μg mL−1 and histidine at 108.06 ± 0.044 μg mL−1. PEYA topped with phenylalanine (495.67 ± 0.011 μg mL−1), glutamine (345.94 ± 0.004 μg mL−1), and lysine (246.72 ± 0.004 μg mL−1). In ROB, histidine content (142.57 ± 0.003 μg mL−1) exceeded others, while YAN had the highest histidine content at 73.42 ± 0.021 μg mL−1. In ZAN, amino acids were ranked as phenylalanine > arginine > glutamine > lysine > histidine. Representative LC-MS/MS chromatograms of the standard and sample are shown in ESI Fig. 1a and b,† and ion chromatograms in ESI Fig. 2A and B.† Amino acids play vital roles as biocatalysts, nutrients, cell constituents, and neurotransmitters.47 Prior studies on vegetables like carrots, sweet potatoes, peas, cabbage, spinach, and komatsuna found higher levels of free amino acids, particularly asparagine and glutamine.48 Examining wild fruits in Assam revealed a high content of aspartic acid, arginine, glutamic acid, histidine, valine, and leucine, aligning with our findings.49 Studies indicate that phenylalanine, tryptophan, and tyrosine are three essential components in plant metabolism that act as precursors of production of aromatic secondary metabolites such as lignin, anthocyanins, flavonoids, and alkaloids. The amount of aromatic amino acids has significant influences on the production of phenolic compounds.50,51
Unripe bananas are reported to be a good source of vitamins B6, C, and provitamin A.52 Raw bananas selected under the present study were analysed for water-soluble vitamins (thiamine, pyridoxine, nicotinic acid, folic acid, riboflavin, and ascorbic acid) using LC-MS/MS (Table 2). Folic acid (0.860 ± 0.051 μg mL−1) and pyridoxine (0.790 ± 0.065 μg mL−1) were found to be abandoned in Kappa, while MA had higher ascorbic acid content (0.770 ± 0.067 μg mL−1) and nicotinic acid (0.730 ± 0.005 μg mL−1). All cultivars showed significant amounts of Vit B6 and Vit C. Prior studies noted vitamin C and B complex vitamins (B1, B2, B3, B9, and B6) in raw bananas.53–56 It is observed from the available literature that the vitamin C content varies significantly throughout cultivars, with Cavendish and Dwarf Brazilian levels varying between 4.5 and 12.7 mg per 100 g.52 The varieties investigated in the current study displayed lower vitamin C content than that of the reported ones. This difference may be attributed to factors like variety, growing conditions, maturity, and drying conditions. A recent study showed that increased drying temperatures in ripe Nendran bananas resulted in decreased vitamin content.57 The present study highlights the nutritional richness of raw bananas, despite variations, offering essential vitamins in a well-balanced diet.58 Representative LC-MS/MS chromatograms of the standard and sample are presented in ESI Fig. 3a and b,† and ion chromatograms in ESI Fig. 4A and B.†
Table 2 Vitamin quantification in ethanolic extracts of cultivarsa
Vitamins |
Concentration in the samples (μg mL−1) |
NEN |
Kappa |
MA |
MON |
ME |
PALA |
PEYA |
ROB |
YAN |
ZAN |
Values are mean ± SD; they are followed by different alphabets in each row which indicate significantly different values (p < 0.05). The letter ‘a’ represents the highest value. |
Thiamine |
0.007 ± 0.013a |
— |
— |
— |
0.005 ± 0.007a |
— |
— |
— |
— |
0.002 ± 0.076a |
Pyridoxine |
0.060 ± 0.001e |
0.790 ± 0.065a |
0.220 ± 0.002c |
0.0610 ± 0.013e |
0.190 ± 0.009d |
0.180 ± 0.006d |
0.078 ± 0.024e |
0.307 ± 0.006b |
0.240 ± 0.005c |
0.164 ± 0.002d |
Folic acid |
0.206 ± 0.011c |
0.860 ± 0.051a |
0.018 ± 0.007h |
0.140 ± 0.004d |
— |
0.090 ± 0.081e |
0.480 ± 0.003b |
0.068 ± 0.007f |
— |
0.054 ± 0.004g |
Nicotinic acid |
0.440 ± 0.090e |
0.640 ± 0.007c |
0.730 ± 0.005a |
0.280 ± 0.009h |
0.340 ± 0.008g |
0.640 ± 0.016c |
0.430 ± 0.025e |
0.700 ± 0.005b |
0.601 ± 0.015d |
0.370 ± 0.017f |
Riboflavin |
0.150 ± 0.006a |
— |
— |
— |
— |
— |
— |
— |
— |
0.130 ± 0.008b |
Ascorbic acid |
0.280 ± 0.032d |
0.210 ± 0.009f |
0.770 ± 0.067a |
0.307 ± 0.005c |
0.240 ± 0.045e |
0.240 ± 0.076e |
0.360 ± 0.007b |
0.082 ± 0.009h |
0.360 ± 0.008b |
0.180 ± 0.014g |
3.4 Phytochemical characterisation
3.4.1 Total phenolic and flavonoid content. Phenolic compounds, owing to their free radical-scavenging properties, act as excellent antioxidants.59 Hydro-alcoholic extracts of banana flour were analysed for total phenolic content and total flavonoid content. The phenolic content of raw banana flour followed the order MA > PALA > ZAN > Kappa > ME > ROB > PEYA > YAN > NEN > MON, ranging from 50.78 ± 0.15 to 96.14 ± 0.64 mg GAE per 100 g (Fig. 3a). Comparable TPC was observed in Musa acuminata by Menezes et al.,60 while Kumar et al.30 reported 44.50 mg GAE per 100 g TPC in Monthan. Higher TPC in Nendran was recorded earlier; variations in TPC are attributed to genomic content, planting area, or geographical conditions affecting banana varieties.10,61
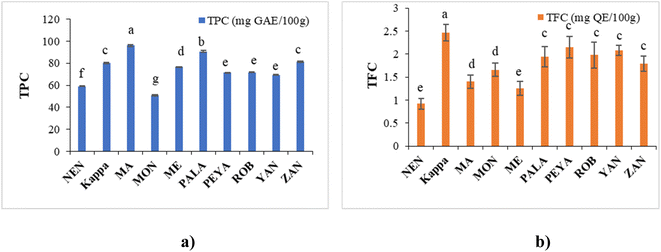 |
| Fig. 3 Phytochemical assays: (a) TPC and (b) TFC of different banana cultivars. Data represented are mean value ± SD, while data bars with different alphabets show significant differences (p < 0.05). | |
The TFC in this study ranged from 0.92 ± 1.32 to 2.46 ± 1.88 mg QE per 100 g, in the order Kappa > PEYA > YAN > ROB > PALA > ZAN > MON > MA > ME > NEN (Fig. 3b). Siji and Nandini55 reported TFC of 3.58 mg QE per 100 g for Kappa, aligning closely with our study. Suniati and Purnomo62 reported comparable TFC for raw Goroho (Musa acuminata sp.). A study on Dwarf Cavendish also documented similar findings (1.69 ± 0.34 mg RE per g) in terms of TFC.63 Flavonoid synthesis, stimulated by sunlight, concentrates them in the outer portions of fruits.64 The most prevalent polyphenols in our regular diets are flavonoids, which exhibit antioxidant, antiviral, antibacterial, antiallergenic, antithrombotic, anti-inflammatory, and hepatoprotective actions.65,66 In banana fruit, soluble flavonoids were shown to have a higher concentration and stronger antioxidant activity than bound flavonoids.67 Moreover, flavonoids have a complex structure, which makes them quite poor in bioavailability. Since only small amounts of them can be absorbed by the small intestine, a large amount reaches the large intestine and interacts with intestinal microbes to promote the growth of beneficial microbiota.68 Gut microbiota, like Bifidobacteriaceae, Lactobacillaceae, Lachnospiraceae, and Enterococcaceae, contribute to flavonoid transformation (O-deglycosylation of flavonoids), whereas other species are involved in the degradation of aglycones or cleaving of flavonoid-C-glucosides.69,70 Dietary flavonoid intake can improve gut microbiota regulation, impacting host immunity, inflammation, and diversity of microbiota.71 The regulation of gut microbes could be associated with regulating short-chain fatty acid metabolism and bile acid synthesis and uptake, potentially promoting bile acid homeostasis.72,73 Thus, the phenolic and flavonoid composition established from the present study further unveils the potential application of raw banana flour in functional foods and health food applications.
3.4.2 Antioxidant activity. The antioxidant activity of raw banana flour was studied by DPPH and ABTS free radical scavenging assays and is summarised in Fig. 4a and b. These assays are used to assess the free radical scavenging properties based on spectrophotometric measurements of the DPPH and ABTS concentration changes resulting from the reaction with an antioxidant. The results of the DPPH assay showed that the free radical scavenging potential was higher in ROB and PALA as compared to other varieties. The IC50 values for ROB and PALA were 35.27 ± 0.0062 and 35.39 ± 0.0063 μg mL−1, respectively. The minimum DPPH activity shown by Kappa was 48.105 ± 0.0053 μg mL−1. A lower IC50 value indicates strong free radical inhibitory activity. The DPPH activity of ethanolic extracts in this study was in agreement with that reported by Siji and Nandini, 2017.74 Consistent with the DPPH activity, the ABTS free radical scavenging capacity of ROB (520.74 ± 0.0068 μg mL−1) and PALA (832.53 ± 0.0021 μg mL−1) was considerably higher than that of others; ME and Kappa had the lowest ABTS activity, 1680.68 ± 0.0024 μg mL−1 and 1504.78 ± 0.0084 μg mL−1, respectively. The ABTS activity of six Australian banana cultivars (Cavendish, Ladyfinger, Plantain, Ducasse, Monkey, and Red Dacca) was reported in the range of 0.98–2.35 mg AAE per g, and these were close to those of the NEN, MON, YAN, ZAN and PEYA cultivars of our study.75 Similar results are reported for crude ethanolic extracts of other plant species wherein the radical scavenging activity increased in a dose dependent manner.76–78 It is also reported that TPC and antioxidant activity are positively correlated, and the variation in TPC and TFC is influenced by changes in climate, environmental conditions, and the cultivar.79–81
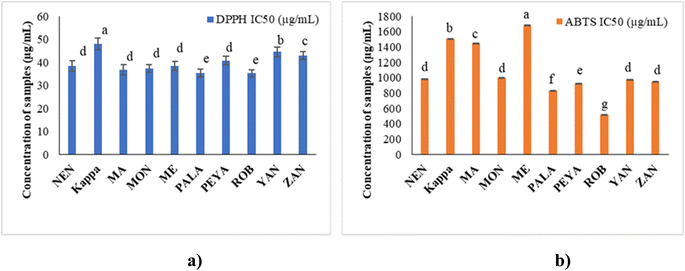 |
| Fig. 4 Antioxidant assays: (a) DPPH and (b) ABTS activity of different banana cultivars. Data presented are mean value ± SD, while data bars with different alphabets show significant differences (p < 0.05). | |
3.5 Quantification of polyphenols
TPC analysis highlighted raw bananas as excellent sources of phenolic compounds. LC-MS/MS is generally used for the quantitative estimation of phytochemicals in plant-based extracts.82–84 In the present study, we have employed the technique to analyse 28 polyphenolic compounds in raw banana flour extracts.
The LC-MS/MS analysis unveiled the polyphenol profile in various banana cultivars, with shikimic acid, epicatechin, ferulic acid, and rutin as predominant ones (Table 3). NEN demonstrated significant content of polyphenols, with shikimic acid (78.820 ± 0.0021 μg mL−1) and ferulic acid (3.490 ± 0.0076 μg mL−1) as the major ones. Kappa was rich in shikimic acid (118.940 ± 0.0042 μg mL−1) and ferulic acid (4.120 ± 0.0032 μg mL−1). MA contained shikimic acid (63.490 ± 0.0078 μg mL−1) and gallic acid (3.630 ± 0.0031 μg mL−1). MON had shikimic acid (49.100 ± 0.0015 μg mL−1). Shikimic acid (81.23 ± 0.0019 μg mL−1) and ferulic acid (1.720 ± 0.0071 μg mL−1) were the major phytochemicals in ME. PALA excelled with abundant shikimic acid (133.380 ± 0.0013 μg mL−1) and notable amounts of ferulic acid (3.820 ± 0.008 μg mL−1). Shikimic acid (65.130 ± 0.0042 μg mL−1) and epicatechin (5.830 ± 0.0016) topped in PEYA. ROB featured the highest ferulic acid (19.430 ± 0.0023 μg mL−1) among the cultivars and the highest shikimic acid (109.180 ± 0.0065 μg mL−1). The phytochemical profile in YAN followed the order shikimic acid > ferulic acid > epicatechin, with a shikimic acid content of 107.730 ± 0.0012 μg mL−1. Shikimic acid (80.50 ± 0.0017 μg mL−1) and ferulic acid (2.8360 ± 0.0033 μg mL−1) were the major compounds in ZAN. Representative LC-MS/MS chromatograms of the standard and sample are shown in ESI Fig. 5a and b,† and ion chromatograms in ESI Fig. 6A and B.†
Table 3 Polyphenol quantification in ethanolic extracts of cultivarsa
Compounds |
Concentration in the samples (μg mL−1) |
NEN |
Kappa |
MA |
MON |
ME |
PALA |
PEYA |
ROB |
YAN |
ZAN |
Values are mean ± SD; they are followed by different alphabets in each row which indicate significantly different values (p < 0.05). The letter ‘a’ represents the highest value. |
Catechin |
1.220 ± 0.0011e |
2.280 ± 0.0022b |
1.040 ± 0.0065f |
1.040 ± 0.0054f |
0.510 ± 0.0033g |
1.520 ± 0.0012c |
3.470 ± 0.0021a |
1.380 ± 0.0064d |
0.260 ± 0.0070h |
1.530 ± 0.0052c |
Quinine |
0.022 ± 0.0032b |
0.021 ± 0.0063b |
0.011 ± 0.0032d |
0.007 ± 0.0076e |
0.020 ± 0.0023b |
0.023 ± 0.0054a |
0.013 ± 0.0033d |
0.016 ± 0.0018c |
0.005 ± 0.0042e |
0.006 ± 0.0015e |
Naringenin |
1.360 ± 0.0055d |
2.140 ± 0.0085a |
1.260 ± 0.0023e |
1.010 ± 0.0011g |
1.110 ± 0.0051f |
0.890 ± 0.0040h |
1.530 ± 0.0013c |
0.980 ± 0.0063g |
0.840 ± 0.0056i |
1.650 ± 0.0012b |
Tocopherol |
0.088 ± 0.0057c |
0.130 ± 0.0044b |
0.160 ± 0.0034a |
0.097 ± 0.0066c |
0.095 ± 0.0076c |
0.120 ± 0.0011b |
0.120 ± 0.0025b |
0.100 ± 0.0098c |
0.110 ± 0.0084c |
0.091 ± 0.0067c |
Gallic acid |
0.570 ± 0.0121g |
0.950 ± 0.0052d |
3.630 ± 0.0031a |
0.730 ± 0.0011e |
0.610 ± 0.0060f |
1.610 ± 0.0054c |
1.680 ± 0.0010b |
0.380 ± 0.0023i |
0.490 ± 0.0056h |
0.500 ± 0.0032h |
Chlorogenic acid |
0.047 ± 0.0225b |
0.044 ± 0.0011b |
0.005 ± 0.0040e |
0.028 ± 0.0022c |
0.021 ± 0.0036c |
0.013 ± 0.0045d |
0.034 ± 0.0014c |
0.005 ± 0.0017e |
0.026 ± 0.0113c |
0.061 ± 0.0055a |
Epicatechin |
1.750 ± 0.0064g |
2.590 ± 0.0017d |
3.200 ± 0.0134b |
2.160 ± 0.0012f |
1.290 ± 0.0046h |
3.100 ± 0.0020c |
5.830 ± 0.0016a |
2.400 ± 0.0018e |
1.120 ± 0.0021i |
1.730 ± 0.0042g |
Syringic acid |
0.390 ± 0.0414d |
— |
0.120 ± 0.0051e |
1.530 ± 0.0013b |
0.008 ± 0.0011g |
0.130 ± 0.0025e |
1.600 ± 0.0050a |
0.020 ± 0.0022f |
— |
0.800 ± 0.0048c |
Vanillic acid |
0.680 ± 0.0034e |
0.640 ± 0.0021f |
1.800 ± 0.0019b |
1.430 ± 0.0083c |
0.370 ± 0.0064h |
1.400 ± 0.0056d |
2.690 ± 0.0051a |
0.670 ± 0.0017e |
0.370 ± 0.0014h |
0.610 ± 0.0015g |
Caffeic acid |
0.270 ± 0.0061e |
0.530 ± 0.0036a |
0.260 ± 0.0076f |
0.520 ± 0.0017b |
0.240 ± 0.0019g |
0.470 ± 0.0026c |
0.290 ± 0.0021d |
0.190 ± 0.0015i |
0.260 ± 0.0028f |
0.230 ± 0.0022h |
Epigallocatechin |
0.012 ± 0.0052c |
0.018 ± 0.0020b |
0.021 ± 0.0114a |
0.013 ± 0.0066c |
0.013 ± 0.0026c |
0.018 ± 0.0020b |
0.018 ± 0.0097b |
0.019 ± 0.0037b |
0.021 ± 0.0017a |
0.014 ± 0.0022c |
Ferulic acid |
3.490 ± 0.0076d |
4.120 ± 0.0032b |
1.890 ± 0.0086h |
2.180 ± 0.0014g |
1.720 ± 0.0071i |
3.820 ± 0.0087c |
1.520 ± 0.0021j |
19.430 ± 0.0023a |
2.500 ± 0.0045f |
2.860 ± 0.0033e |
Myricetin |
0.370 ± 0.0016g |
0.600 ± 0.0019e |
1.260 ± 0.0010a |
0.240 ± 0.0044h |
0.170 ± 0.0035j |
0.780 ± 0.0012d |
0.790 ± 0.0073c |
0.950 ± 0.0017b |
0.180 ± 0.0034i |
0.530 ± 0.0028f |
p-Coumaric acid |
0.460 ± 0.0081d |
0.210 ± 0.0023h |
0.920 ± 0.0011a |
0.490 ± 0.0034c |
0.170 ± 0.0072i |
0.340 ± 0.0081e |
0.750 ± 0.0024b |
0.240 ± 0.0055g |
0.290 ± 0.0012f |
0.350 ± 0.0016e |
Apigenin |
0.013 ± 0.0049b |
0.018 ± 0.0032a |
0.017 ± 0.0065a |
0.015 ± 0.0006b |
0.012 ± 0.0016b |
0.018 ± 0.0046a |
0.017 ± 0.0010a |
0.017 ± 0.0023a |
0.019 ± 0.0042a |
0.019 ± 0.0016a |
Kaempferol |
0.026 ± 0.0032e |
0.065 ± 0.010a |
0.040 ± 0.0020c |
0.052 ± 0.0050b |
0.025 ± 0.0031e |
0.037 ± 0.0003c |
0.030 ± 0.0038d |
0.050 ± 0.0007b |
0.018 ± 0.0011f |
0.052 ± 0.0018b |
Rutin |
2.590 ± 0.0011c |
2.650 ± 0.0040b |
1.720 ± 0.0056d |
1.170 ± 0.0077g |
0.550 ± 0.001i |
1.540 ± 0.0010e |
4.350 ± 0.021a |
1.250 ± 0.0027f |
0.790 ± 0.0047h |
2.590 ± 0.0073c |
Hesperetin |
0.016 ± 0.0010b |
0.025 ± 0.0015a |
0.025 ± 0.0044a |
0.016 ± 0.0025b |
— |
0.024 ± 0.0036a |
0.026 ± 0.0021a |
0.027 ± 0.0014a |
0.024 ± 0.0087a |
0.018 ± 0.0022b |
Shikimic acid |
78.820 ± 0.0021g |
118.940 ± 0.0042b |
63.490 ± 0.0078i |
49.100 ± 0.0015j |
81.230 ± 0.0019e |
133.380 ± 0.0013a |
65.130 ± 0.0042h |
109.180 ± 0.0065c |
107.730 ± 0.0012d |
80.500 ± 0.0017f |
Ellagic acid |
— |
0.410 ± 0.0112b |
0.220 ± 0.0064c |
0.042 ± 0.0045e |
0.160 ± 0.0014d |
— |
— |
0.600 ± 0.0031a |
— |
— |
Genistein |
0.011 ± 0.0024b |
0.018 ± 0.0025a |
0.019 ± 0.0076a |
0.012 ± 0.0010b |
0.011 ± 0.0086b |
0.019 ± 0.0043a |
0.017 ± 0.0032a |
0.020 ± 0.0015a |
0.020 ± 0.0054a |
0.011 ± 0.0044b |
Cinnamic acid |
0.410 ± 0.0132d |
0.390 ± 0.0045e |
0.850 ± 0.0025a |
0.420 ± 0.0016d |
0.140 ± 0.0063g |
0.140 ± 0.0059g |
0.310 ± 0.0047f |
0.640 ± 0.0043b |
0.480 ± 0.0033c |
0.160 ± 0.0034f |
When assessing the polyphenol component of all banana cultivars, it was noted that shikimic acid was the predominant compound, followed by ferulic acid and flavonoids including rutin, epicatechin, and catechin. Earlier studies reported that unripe fruit pulp contains shikimic acid.85 In addition to the above-mentioned polyphenols, lower concentrations of compounds like naringenin, vanillic acid, myricetin, syringic acid, and gallic acid were also found in some banana cultivars. Rutin, myricetin, naringenin, gallic acid, and ellagic acid were reported earlier in bananas.86 An earlier study of the phenolic and flavonoid contents in unripe and ripe Australian-grown banana types revealed the presence of p-hydroxybenzoic acid, quercetin, gallic acid, syringic acid, diosmin, chlorogenic acid, p-coumaric acid, protocatechuic acid, quercetin-3-rhamnoside, and kaempferol.75 In another study, the pulp and peel of banana cultivars were found to contain ferulic acid, ferulic acid-hexoside, epicatechin, caffeic acid-hexoside, sinapic acid, hydroxycinnamic acid, myricetin-deoxyhexose, rutin, quercetin-deoxyhexose, kaempferol-3-O-rutinoside, and other unidentified compounds.87 The dried pulp powder of Musa balbisiana has been found to contain epicatechin, catechin, rutin, quercetin, chlorogenic acid, kaempferol 3-O-sophoroside, apigenin-6-C-glucoside-7-O-glucoside, and rhamnopyranoside.88 Rutin, catechin, quinic acid, gallocatechin, propionic acid, caffeic acid-3-glucoside, kaempferol-O-rhamnosidehexoside, caffeic acid, L-dopa, sennoside A, and dopamine have been identified in the hydro-alcoholic extract of Musa Cavendish with anti-cancerous activity.89 These findings aligned with the current study on certain phenolics. Despite numerous studies conducted in this field, there is still a gap in the quantification of phenolics due to their complex structures and the way environmental factors affect the secondary metabolite levels, which differ between cultivars.
3.6 Cytotoxicity assay
The MTT assay investigated the cytotoxic effects of raw banana flour extracts on L6 myoblasts, revealing no impact on cell viability up to 500 μg mL−1. The cell lines treated with different concentrations showed above 70% cell viability. Among the flour extracts, PALA (81.78%) and PEYA (79.62%) exhibited higher viability, while YAN (69.83%) showed lower viability compared to other cultivars at 500 μg mL−1 (Fig. 5). Many previous studies have reported the poor cytotoxicity of banana extracts against normal cell lines. Dahham et al.90 tested the cytotoxic activity of Musa sapientum fruit extract against the normal human umbilical vein endothelial cell line (HUVEC) and the human colorectal carcinoma cell line HCT-116. The result revealed that the banana extracts showed lower cytotoxicity against normal cell lines than cancer cells. Extracts from 20 different fruit extracts were analysed for cytotoxicity against leukemic cell lines and normal human peripheral blood mononuclear cells (PBMCs). Some of these extracts exhibited cytotoxicity against both normal and leukemic cell lines, but some extracts specifically showed toxicity against leukemic cell lines, and others did not show any toxicity. Among these, the Musa sapientum L. extracts presented no cytotoxic effect on normal PBMC cell lines.91 The non-toxic nature of the extracts indicates that they could be used as an ingredient or additive without causing harm to normal cells. The lower cytotoxicity implies that bananas could be considered safe for a wide range of applications, particularly in the food and pharmaceutical industries.
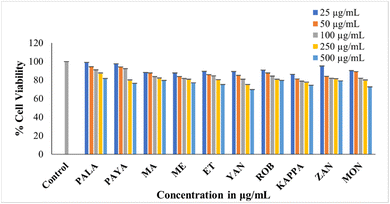 |
| Fig. 5 MTT assay showing the cytotoxicity of the ethanolic extract of ten cultivars against L6 cells. Data presented are mean value ± SD. | |
3.7 α-Amylase inhibition assay
Hyperglycemia results from the rapid breakdown of starchy meals by carbohydrate-hydrolyzing enzymes such as α-amylase and α-glucosidase. The therapeutic approach for diabetes involves synthetic inhibitors of α-amylase to impede glucose absorption, which is reported to cause undesirable side effects.92 Many dietary bioactive components are reported to inhibit these enzymes. Banana extracts (200 μg mL−1) and acarbose (100 μg mL−1) were evaluated against PPA. The findings demonstrate that the α-amylase inhibitory activity of flour extracts varied across samples, with PALA and ROB with higher inhibitory potential (66.96% and 50.50%) (Fig. 6). The standard acarbose showed 76.84% inhibition. The results are in agreement with previous reports on Musa paradisiaca's antidiabetic potential.93 The α-amylase inhibitory activity may be correlated with the bioactive phytochemicals, in line with previous reports.94,95 The results could have implications for managing postprandial blood sugar levels, making them potentially useful in the dietary management of diabetes.
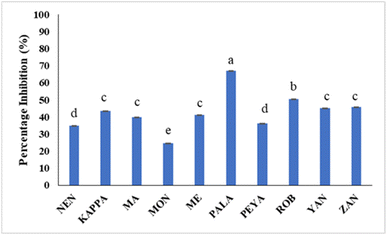 |
| Fig. 6 The percentage inhibition of PPA by different banana cultivars. Data represented are mean value ± SD, while data bars with different alphabets show significant differences (p < 0.05). | |
3.8 Molecular docking studies
According to LCMS/MS analysis, shikimic acid and ferulic acid were identified as the major phytochemicals in the raw banana flour. Both shikimic acid and ferulic acid are reported to have hypoglycemic activity due to their inhibition potential of α-amylase.96,97 We adopted molecular docking to correlate the same. The docking analysis identified the optimal poses for both, with the lowest energies at −5.45 and −5.48 kcal mol−1 respectively, for shikimic acid and ferulic acid, suggesting high binding affinity. Table 4 and Fig. 7 illustrate the hydrogen bond residues involved in their interactions with α-amylase. Earlier studies reported a binding energy of −5.3 kcal mol−1 for the ferulic acid–α-amylase complex.97 However, the binding energy was lower compared to acarbose (−8.8 kcal mol−1).98 PALA and ROB were found to have higher estimated amounts of ferulic acid and shikimic acid in comparison to the other variants. Thus, the α-amylase inhibitory activity of the banana extracts can be correlated with the presence of phytochemicals such as shikimic acid and ferulic acid, which was confirmed by docking studies.
Table 4 Molecular docking results of banana cultivars phenolics towards α-amylase (PDB ID: 4W93)
Ligands |
PubChem CID |
Structure |
H-bonded residues |
Binding energy (kcal mol−1) |
Shikimic acid |
8742 |
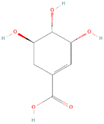 |
Asn137 |
−5.45 |
Leu170 |
Asp206 |
Trp203 |
His201 |
Asn167 |
Asn100 |
Ferulic acid |
445 858 |
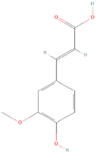 |
Arg421 |
−5.48 |
Gly403 |
Arg252 |
Ser289 |
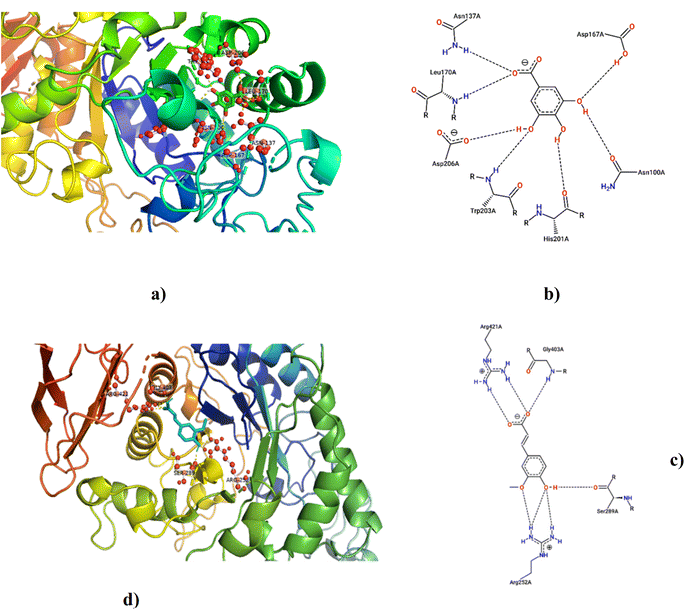 |
| Fig. 7 Molecular docking for shikimic acid and ferulic acid with α-amylase amino acid residues; (a and b) 3D & 2D interaction image of shikimic acid; (c and d) 3D & 2D interaction image of ferulic acid. | |
4 Conclusion
This study delved into the nutritional composition, starch, resistant starch, phytochemicals, antioxidants, and cytotoxicity of 10 raw banana cultivars at stage II maturity. While proximate composition remained comparable across varieties, distinctions in phenolic, flavonoid, and antioxidant potential were noted, reflecting variations in genomic and chemical constituents. LC-MS/MS analysis quantified significant polyphenols (shikimic acid, ferulic acid, epicatechin, and rutin), amino acids (phenylalanine, histidine, glutamine, lysine, and arginine), and vitamins (pyridoxine, ascorbic acid, and nicotinic acid) among cultivars. Unripe banana flour emerged as a notable source of resistant starch, which can play an important role in contributing to gut health. With the presence of bioactive phytochemicals and hypoglycemic properties enriched with resistant starch, there is great scope for the value addition of unripe bananas. The study underscores the potential of raw banana flour as a functional food ingredient, enriching food products with enhanced nutritional properties. The findings present raw banana flour as a viable substitute, offering sustainable and nutritious alternatives for food development. Future studies in the direction of identifying and quantifying the bioactive components in green bananas that are responsible for health benefits and their potential uses as functional ingredients in nutraceuticals and functional foods are warranted. Development of gluten-free products and meal replacements looking into their potential in diabetic management, prebiotic potential and effects on gut microbiota can also be investigated and established by in vivo studies. Exploring the untapped potential of these cultivars opens avenues for innovative and sustainable food production, aligning with current trends in health-conscious and environmentally friendly consumer choices.
Abbreviations
ABTS | 2,2′-Azino-bis(3-ethylbenzothiazoline-6-sulphonic acid) |
AlCl3 | Aluminum chloride |
CH3COOK | Potassium acetate |
DPPH | 2,2-Diphenyl-1-picrylhydrazyl |
ESI | Electrospray ionisation |
HCl | Hydrochloric acid |
HPLC | High-performance liquid chromatography |
I2 | Iodine |
KI | Potassium iodide |
LC-MS/MS | Liquid chromatography-tandem mass spectrometry |
MA | Malayannan |
ME | Mysore Ethan |
MON | Monthan |
MRM | Multiple reaction-monitoring |
MTT | 3-(4,5-Dimethylthiazol-2-yl)-2,5-diphenyl tetrazolium bromide |
NaOH | Sodium hydroxide |
NEN | Nendran |
PALA | Palayankodan |
PBMCs | Peripheral blood mononuclear cells |
PEYA | Peyan |
PPA | Porcine pancreatic amylase |
RADD | Refrigerated adsorption dehumidified drier |
ROB | Robusta |
RS | Resistant starch |
SD | Standard deviation |
TFC | Total flavonoid content |
TPC | Total phenolic content |
YAN | Yangambi |
ZAN | Zanzibar |
Data availability
Data will be made available on request.
Author contributions
SVS: conceptualization, data curation, methodology, investigation, formal analysis, and writing – original draft; AB: investigation, writing – review and editing; AS: investigation; PN: conceptualization, supervision, resources, writing – review and editing.
Conflicts of interest
The authors declare no conflict of interest in this work.
Acknowledgements
The authors extend their gratitude to the University Grants Commission (UGC) and Council of Scientific and Industrial Research (CSIR) of India for their financial support for the research work. The banana cultivars have been provided by the College of Agriculture, Kerala, which is also acknowledged by the authors.
References
- P. K. Dash and R. Rai, Front. Plant Sci., 2016, 7, 1–7 Search PubMed.
- G. Aurore, C. Ginies, B. Ganou-Parfait, C. M. G. C. Renard and L. Fahrasmane, Food Chem., 2011, 129, 28–34 CrossRef CAS.
- H. Strosse, H. Schoofs, B. Panis, E. Andre, K. Reyniers and R. Swennen, Plant Sci., 2006, 170, 104–112 CrossRef CAS.
- Bananas | FAO | Food and Agriculture Organization of the United Nations, https://www.fao.org/markets-and-trade/commodities/bananas/en/, accessed 9 June 2023.
- FAOSTAT, https://www.fao.org/faostat/en/#data/QCL, accessed 9 June 2023.
- O. Gibert, D. Dufour, A. Giraldo, T. Sánchez, M. Reynes, J. P. Pain, A. González, A. Fernández and A. Díaz, J. Agric. Food Chem., 2009, 57, 7857–7869 CrossRef CAS PubMed.
- Z. A. Salih, A. Siddeeg, R. TATaha, M. Bushra, A.-F. Ammar and A. O. Ali, Int. J. Agric. Sci. Res., 2017, 4, 348–353 Search PubMed.
- M. P. Maziarz, S. Preisendanz, S. Juma, V. Imrhan, C. Prasad and P. Vijayagopal, Nutr. J., 2017, 16, 14 CrossRef PubMed.
- M. Iroaganachi and C. Eleazu, J. Pancreas, 2015, 16, 167–170 Search PubMed.
- T. A. Anyasi, A. I. O. Jideani and G. A. Mchau, Food Sci. Nutr., 2015, 3, 221–232 CrossRef CAS PubMed.
- Y. Wang, M. Zhang and A. S. Mujumdar, Lwt, 2012, 47, 175–182 CrossRef CAS.
- A. Amini Khoozani, J. Birch and A. E. D. A. Bekhit, J. Food Sci. Technol., 2019, 56, 548–559 CrossRef CAS PubMed.
- K. B. Arun, F. Persia, P. S. Aswathy, J. Chandran, M. S. Sajeev, P. Jayamurthy and P. Nisha, J. Food Sci. Technol., 2015, 52, 6355–6364 CrossRef CAS PubMed.
- AOAC 930.15-1930(1999), Loss on drying (Moisture) for feeds at – $14.15 : AOAC Official Method, http://www.aoacofficialmethod.org/index.php?main_page=product_info%26products_id=2702, accessed 12 June 2023.
- AOAC 923.03-1923, Ash of flour. Direct method – $14.15 : AOAC Official Method, http://www.aoacofficialmethod.org/index.php?main_page=product_info%26products_id=1190, accessed 12 June 2023.
- AOAC 983.23-1984, Fat in Foods – Chloroform-Methanol Extraction – $14.30 : AOAC Official Method, http://www.aoacofficialmethod.org/index.php?main_page=product_info%26products_id=1665, accessed 13 June 2023.
- AOAC 984.13-1994(1996), Protein (Crude) in animal feed and pet f – $14.30 : AOAC Official Method, http://www.aoacofficialmethod.org/index.php?main_page=product_info%26products_id=1044, accessed 13 June 2023.
- F. Noor, Int. J. Nutr. Food Sci., 2014, 3, 347 CrossRef CAS.
- Y. Zhang, H. Zeng, Y. Wang, S. Zeng and B. Zheng, Food Chem., 2014, 155, 311–318 CrossRef CAS PubMed.
- A. Escarpa, M. C. González, M. D. Morales and F. Saura-Calixto, Food Chem., 1997, 60, 527–532 CrossRef CAS.
- K. B. Arun, R. Dhanya, J. Chandran, B. Abraham, S. Satyan and P. Nisha, J. Food Sci. Technol., 2020, 57, 3221–3231 CrossRef CAS PubMed.
- R. Re, N. Pellegrini, A. Proteggente, A. Pannala, M. Yang and C. Rice-Evans, Free Radical Biol. Med., 1999, 26, 1231–1237 CrossRef CAS PubMed.
- B. Abraham, T. R. Reshmitha, M. M. Navami, L. George, V. V. Venugopalan and P. Nisha, Ind. Crops Prod., 2020, 151, 112451 CrossRef CAS.
- M. M. Navami, B. Abraham, H. Archana and P. Nisha, JSFA Rep., 2023, 3, 377–386 CrossRef CAS.
- T. Mosmann and J. Immunological, Methods, 1983, 65, 55–63 CAS.
- Z. Xiao, R. Storms and A. Tsang, Anal. Biochem., 2006, 351, 146–148 CrossRef CAS PubMed.
- A. O. Oko, A. C. Famurewa and J. O. Nwaza, Curr. J. Appl. Sci. Technol., 2015, 6(3), 285–294 Search PubMed.
- C. Bangwaek, Acta Hortic., 2014, 1026, 61–66 CrossRef.
- G. I. Onwuka and N. D. Onwuka, Int. J. Food Prop., 2005, 8, 347–353 CrossRef.
- P. S. Kumar, A. Saravanan, N. Sheeba and S. Uma, Lwt, 2019, 116, 108524 CrossRef CAS.
- M. Khoza, E. Kayitesi and B. C. Dlamini, Foods, 2021, 10, 1–15 CrossRef PubMed.
- A. Amini Khoozani, B. Kebede, J. Birch and A. E.-D. A. Bekhit, Foods, 2020, 9, 152 CrossRef PubMed.
- A. S. Babu, M. Mahalakshmi and R. Parimalavalli, Trends Carbohydr. Res., 2014, 6, 38–44 Search PubMed.
- F. Carlos-Amaya, P. Osorio-Diaz, E. Agama-Acevedo, H. Yee-Madeira and L. A. Bello-Pérez, J. Agric. Food Chem., 2011, 59, 1376–1382 CrossRef CAS PubMed.
- M.-C. Li, C.-F. Chou, S.-C. Hsu and J.-S. Lin, Int. J. Food Prop., 2020, 23, 1168–1175 CrossRef.
- E. Juarez-Garcia, E. Agama-Acevedo, S. G. Sáyago-Ayerdi, S. L. Rodríguez-Ambriz and L. A. Bello-Pérez, Plant Foods Hum. Nutr., 2006, 61, 131–137 CrossRef CAS PubMed.
- Z. Li, K. Guo, L. Lin, W. He, L. Zhang and C. Wei, Molecules, 2018, 23, 2312 CrossRef PubMed.
- N. Vatanasuchart, B. Niyomwit and K. Wongkrajang, Maejo Int. J. Sci. Technol., 2012, 6(02), 259–271 CAS.
- H. Marta, Y. Cahyana, M. Djali, J. Arcot and T. Tensiska, Int. J. Food Prop., 2019, 22, 1562–1575 CrossRef CAS.
- A. Mohamed, J. Xu and M. Singh, Food Chem., 2010, 118, 620–626 CrossRef CAS.
- B. Cordenunsi, J. Nascimento, V. Castro-Alves, E. Purgatto, J. Fabi and F. Peroni-Okita, Front. Plant Sci., 2019, 7, 391 CrossRef PubMed.
- C. V. Bezerra, A. M. d. C. Rodrigues, E. R. Amante and L. H. M. da Silva, Rev. Bras. Frutic., 2013, 35, 1140–1146 CrossRef.
- T. Nguyen Minh, T. Beverly Cheruto, V. Kieu Minh, L. Phan Thi Truc, T. Phan Thi Thanh, T. Nguyen Bich, V. Le Thi Tuong, T. Le Ngoc and T. Ngo Van, J. Appl. Microbiol. Biotechnol., 2022, 10, 181–188 Search PubMed.
- G. Zhang and B. R. Hamaker, Crit. Rev. Food Sci. Nutr., 2009, 49, 852–867 CrossRef CAS PubMed.
- FoodData Central, https://fdc.nal.usda.gov/fdc-app.html#/food-details/173944/nutrients, accessed 20 November 2023.
- H. S. Lee, Y. B. Kim, C. Seo, M. Ji, J. Min, S. Choi, H. B. Kim, H. J. Park, G. Lee, W. Lee and M. J. Paik, Anal. Lett., 2019, 52, 2496–2505 CrossRef CAS.
- M. S. Mokbel and F. Hashinaga, Am. J. Biochem. Biotechnol., 2005, 1, 125–131 CrossRef.
- H. Ito, H. Kikuzaki and H. Ueno, J. Nutr. Sci. Vitaminol., 2019, 65, 264–271 CrossRef CAS PubMed.
- A. Islary, J. Sarmah and S. Basumatary, Asian J. Chem., 2019, 31, 825–828 CAS.
- H. Maeda and N. Dudareva, Annu. Rev. Plant Biol., 2012, 63, 73–105 CrossRef CAS PubMed.
- H. Hu, J. Wang, Y. Hu and J. Xie, Food Funct., 2020, 11, 8286–8296 RSC.
- M. M. Wall, J. Food Compos. Anal., 2006, 19, 434–445 CrossRef CAS.
- M. M. A. N. Ranjha, S. Irfan, M. Nadeem and S. Mahmood, Food Rev. Int., 2022, 38, 199–225 CrossRef CAS.
- S. Qamar and A. Shaikh, Trends Food Sci. Technol., 2018, 79, 1–9 CrossRef CAS.
- S. Siji and P. V. Nandini, Int. J. Adv. Eng. Manage. Sci., 2016, 2, 964–968 Search PubMed.
- K. Dakshinamurti, S. Dakshinamurti and M. P. Czubryt, in Handbook of Famine, Starvation, and Nutrient Deprivation: from Biology to Policy, ed. V. Preedy and V. B. Patel, Springer International Publishing, Cham, 2017, pp. 1–23 Search PubMed.
- S. Kabeer, N. Govindarajan, R. Preetha, K. Ambrose, M. Essa and M. Qoronfleh, J. Food Sci. Technol., 2022, 60, 1–10 Search PubMed.
- L. Englberger, G. Lyons, W. Foley, J. Daniells, B. Aalbersberg, U. Dolodolotawake, C. Watoto, E. Iramu, B. Taki, F. Wehi, P. Warito and M. Taylor, J. Food Compos. Anal., 2010, 23, 624–632 CrossRef CAS.
- R. Kamal, M. Kharbach, Y. Vander Heyden, Z. Doukkali, R. Ghchime, A. Bouklouze, Y. Cherrah and K. Alaoui, J. Food Biochem., 2019, 43, e13066 CrossRef PubMed.
- E. W. Menezes, C. C. Tadini, T. B. Tribess, A. Zuleta, J. Binaghi, N. Pak, G. Vera, M. C. T. Dan, A. C. Bertolini, B. R. Cordenunsi and F. M. Lajolo, Plant Foods Hum. Nutr., 2011, 66, 231–237 CrossRef CAS PubMed.
- A. M. Aboul-Enein, Z. A. Salama, A. A. Gaafar and H. F. Aly, J. Chem. Pharm. Res., 2016, 8, 46–55 CAS.
- F. R. T. Suniati and H. Purnomo, Food Res., 2019, 3, 678–683 Search PubMed.
- J. Kim, J.-Y. Choi, J. Kim, K.-D. Moon and J. Korean, Food Preserv., 2021, 28, 13–22 CrossRef.
- R. Tsao and J. McCallum, in Fruit and Vegetable Phytochemicals: Chemistry, Nutritional Value and Stability, ed. L. A. de la Rosa, Alvarez-Parrilla and G. A. Gonzalez-Aguilar, WILEY-Blackwell, Ames, 2009, pp. 131–153 Search PubMed.
- J. Dai and R. J. Mumper, Molecules, 2010, 15, 7313–7352 CrossRef CAS PubMed.
- J. Xiang, M. Zhang, F. B. Apea-Bah and T. Beta, Food Chem., 2019, 295, 214–223 CrossRef CAS PubMed.
- C. Dong, H. Hu, Y. Hu and J. Xie, Front. Plant Sci., 2016, 7, 1291 Search PubMed.
- F. A. Tomás-Barberán, A. González-Sarrías, R. García-Villalba, M. A. Núñez-Sánchez, M. V. Selma, M. T. García-Conesa and J. C. Espín, Mol. Nutr. Food Res., 2017, 61, 1500901 CrossRef PubMed.
- A. Braune and M. Blaut, Gut Microbes, 2016, 7(3), 216–234 CrossRef CAS PubMed.
- R. Yan and X. Zhang, Lett. Appl. Microbiol., 2022, 74, 555–563 CrossRef CAS PubMed.
- H. Li, L. M. Christman, R. Li and L. Gu, Food Funct., 2020, 11, 4878–4891 RSC.
- L. Zhao, Z. Qi, L. Yi, J. Li, Y. Cui, F. U. Rehman, J. Yang, J. Liu, Y. Li and J. Zhang, Food Funct., 2021, 12, 7836–7850 RSC.
- R. Duan, X. Guan, K. Huang, Y. Zhang, S. Li, J. Xia and M. Shen, J. Agric. Food Chem., 2021, 69, 7629–7640 CrossRef CAS PubMed.
- S. Siji and P. V. Nandini, Int. J. Adv. Res. Comput. Sci. Software Eng., 2017, 4, 118–123 Search PubMed.
- Y. M. Bashmil, A. Ali, B. K. Amrit, F. R. Dunshea and H. A. R. Suleria, Antioxidants, 2021, 10, 1521 CrossRef CAS PubMed.
- M. Elmastaş, L. Ozturk, I. Gokce, R. Erenler and H. Aboul-Enein, Anal. Lett., 2011, 37, 1859–1869 CrossRef.
- R. Erenler, O. Sen, H. Aksit, I. Demirtas, A. S. Yaglioglu, M. Elmastas and İ. Telci, J. Sci. Food Agric., 2016, 96, 822–836 CrossRef CAS PubMed.
- I. Demirtas, R. Erenler, M. Elmastas and A. Goktasoglu, Food Chem., 2013, 136, 34–40 CrossRef CAS PubMed.
- M. Elmastaş, İ. Telci, H. Akşit and R. Erenler, Turk. J. Biochem., 2015, 40, 456–462 CrossRef.
- N. Savlak, B. Türker and N. Yeşilkanat, Food Chem., 2016, 213, 180–186 CrossRef CAS PubMed.
- M. Alothman, R. Bhat and A. A. Karim, Food Chem., 2009, 115, 785–788 CrossRef CAS.
- R. Erenler, I. Telci, M. Elmastaş, H. AksiT, F. Gül, A. R. Tüfekç
, I. DemiRtaş and Ö. Kayir, Turk. J. Chem., 2018, 42, 1695–1705 CrossRef CAS. - M. N. Atalar, R. Erenler, F. Turkan, M. H. Alma, I. Demirtas, A. Baran, S. Irtegun Kandemir, A. I. Kekec and F. Z. Saltan, J. Integr. Med., 2023, 62, 102290 Search PubMed.
- R. Erenler, T. Karan and İ. Hosaflioğlu, Turk J Biod, 2023, 6, 75–78 CrossRef.
- A. C. Hulme, Qual. Plant. Mater. Veg., 1958, 3–4, 468–473 CrossRef CAS.
- S. Behiry, M. Okla, S. Alamri, M. EL-Hefny, M. Salem, I. Alaraidh, H. Ali, S. Al-Ghtani, J. Monroy and A. Z. M. Salem, Processes, 2019, 7, 215 CrossRef CAS.
- C. V. Passo Tsamo, M.-F. Herent, K. Tomekpe, T. Happi Emaga, J. Quetin-Leclercq, H. Rogez, Y. Larondelle and C. M. Andre, J. Food Compos. Anal., 2015, 44, 158–169 CrossRef CAS.
- S. Kumari, P. B. Katare, R. Elancheran, H. L. Nizami, B. Paramesha, S. Arava, P. P. Sarma, R. Kumar, D. Mahajan, Y. Kumar, R. Devi and S. K. Banerjee, Oxid. Med. Cell. Longev., 2020, 2020, 1–14 CrossRef PubMed.
- W. A. Barroso, I. C. Abreu, L. S. Ribeiro, C. Q. da Rocha, H. P. de Souza and T. M. de Lima, Toxicol. In Vitro, 2019, 59, 179–186 CrossRef CAS PubMed.
- S. Dahham, M. Taleb Agha, Y. Tabana and A. M. S. Abdul Majid, Acad. J. Cancer Res., 2015, 8, 28–34 CAS.
- C. Ampasavate, S. Okonogi and S. Anuchapreeda, Afr. J. Pharm. Pharmacol., 2010, 4, 013–021 Search PubMed.
- G. Oboh, I. A. Akinbola, A. O. Ademosun, D. M. Sanni, O. V. Odubanjo, T. A. Olasehinde and S. I. Oyeleye, J. Oleo Sci., 2015, 64, 775–782 CrossRef CAS PubMed.
- I. Patel, O. Padse and Y. Ingole, Int. J. Res. Advent Technol., 2015, 13–14 Search PubMed.
- B. C. Adedayo, G. Oboh, S. I. Oyeleye and T. A. Olasehinde, Scientifica, 2016, 2016, 1–7 CrossRef PubMed.
- A. O. Ademiluyi and G. Oboh, Exp. Toxicol. Pathol., 2013, 65, 305–309 CrossRef CAS PubMed.
- J. Cao, J. Zhang, R. Cao, W. Li, G. Wang, L. Cui and L. Sun, Food Front., 2023, 4, 2058–2069 CrossRef CAS.
- Y. Zheng, J. Tian, W. Yang, S. Chen, D. Liu, H. Fang, H. Zhang and X. Ye, Food Chem., 2020, 317, 1–8 CrossRef PubMed.
- P. Okechukwu, M. Sharma, W. H. Tan, H. K. Chan, K. Chirara, A. Gaurav and M. Al-Nema, Pharmacia, 2020, 67, 363–371 CrossRef CAS.
|
This journal is © The Royal Society of Chemistry 2024 |