Effect of temperature and packaging materials on the shelf-life stability and in vitro properties of microencapsulated and spray-dried synbiotic legume-based instant beverage powder
Received
22nd June 2023
, Accepted 26th October 2023
First published on 27th October 2023
Abstract
The aim of the work was to investigate the effect of different packaging materials and storage temperatures on the stability and in vitro properties of microencapsulated spray-dried synbiotic kidney-mung bean beverage (KMB) powder. The formulation for the synbiotic KMB powder has been explored in our previous work. The present study focuses on the evaluation of the shelf-life of the same synbiotic KMB powder. The synbiotic powder was packed in polypropylene and aluminum laminates and stored at 25, 30, and 35 °C. The survivability of probiotic Lacticaseibacillus casei in encapsulated spray-dried powder was studied under simulated gastric conditions for 70 days. At all three storage temperatures, the count remained >108 CFU mL−1 for the first 21 days. Additionally, moisture content increased and color value (L*) decreased with increasing storage time, irrespective of packaging material. The encapsulated synbiotic powder in Al-laminate pouches also showed better prebiotic activity when compared to PP laminates. An acceptable sensory score (6 out of 10) and low microbial load (<106 CFU mL−1) also suggested that the shelf life of synbiotic KMB powder in polypropylene and aluminum laminates was 63, 35, and 35 days at 25, 30, and 35 °C, respectively.
Sustainability spotlight
Recently, consumers' awareness towards more sustainable and healthy food products has led to major advances in the food industry. Among these, the incorporation of functional foods like synbiotic products into daily dietary patterns has received acceptance by most consumers. However, the market lacks options concerning synbiotic food items owing to the limited prebiotic sources. Nonetheless, with the use of legumes as a rich source of prebiotic components, a synbiotic ready-to-reconstitute beverage powder can be developed. The presented research work investigated the shelf life of developed synbiotic legume-based instant beverage powder to come up with the best packaging materials and storage temperatures for maintaining the required stability and in vitro properties of the microencapsulated spray-dried synbiotic beverage powder. Such a product not only serves as an instant food item but also provides dietary options to those who have conditions like lactose intolerance and dairy allergy, or those who follow veganism in general. In addition, the health benefits associated with probiotic components also enhance the gut metabolism and help maintain overall health.
|
Introduction
Recently, the functional foods industry has gained a lot of consumer attention with more focus on synbiotic foods. Such foods are a combination of two components, namely, probiotics and prebiotics, where the former is a viable beneficial microorganism that exhibits numerous health benefits to humans. At the same time, the latter provides a source of nutrition to probiotics and a matrix for delivery. While both components exhibit a synergistic mechanism, the product as a whole can be utilized as a healthy, nutritious functional food. The most common food matrices used to carry probiotics include dairy products such as milk, yogurt, ice creams, and fruit & vegetable juices.1,2 However, recent studies have also explored the potential of non-dairy matrices belonging to the food groups of cereals and legumes.3 This has also been discussed extensively in the review by Chaturvedi and Chakraborty (2021)4 and Rasika et al. (2021).5 Among these, legumes are still considered a less examined food group, with most of the work on selected legumes like soybean,6 chickpea,7 pea,8etc. Interestingly, the latest studies have identified the ability of certain legumes like mung beans and kidney beans as a potential medium for the delivery of probiotics in food products.9,10 The prebiotics in these legumes can also be utilized to develop a synbiotic system. These legumes are a rich source of protein and prebiotic components like raffinose, stachyose, etc., enabling a synbiotic system.11 Moreover, such products are vegan and can be used as a food option for lactose-intolerant patients or those suffering from dairy and cholesterol-related allergies.
A few studies have shown that the shelf life of synbiotic beverages made using these legumes is good and higher than that of their dairy counterparts.12,13 In a recent study by Mousavi et al. (2023),14 the formulated non-dairy gluten-free beverage made from buckwheat and lentil and fermented using Lactobacillus plantarum and Bifidobacterium bifidum showed an acceptable shelf life of 15 days with >7
log CFU mL−1 viable probiotic cells. Nonetheless, incorporating drying technology into such products also opens options for developing a non-dairy instant ready-to-reconstitute powder. Apart from enhanced shelf life, such instant powders can also be utilized as a core ingredient for manufacturing other food products. Other benefits of such beverage powders include reduced storage and transportation cost, improved convenience, enhanced shelf-life with longer survivability of probiotics, and easy availability. Among different drying techniques, spray drying is one of the most widely used processes for liquid food products at a commercial scale which also allows the scope for encapsulating beneficial microorganisms such as probiotics.15 Nonetheless, the high drying temperatures in spray drying decreases probiotic viability and also causes a glassy state in the developed powder. Spray drying is also limited to liquid food products, thereby restricting the use of this technique for a variety of food products. Various studies have researched upon the optimization of spray drying parameters to develop dairy and non-dairy probiotic spray-dried powders with desirable properties and viable probiotic count. Upadhyay & Dass16 evaluated the survival of probiotic Lactobacillus plantarum in spray-dried guava juice powder using inulin and maltodextrin as drying agents. The study concluded that the developed synbiotic guava juice powder at refrigeration temperature (4 °C) showed a high probiotic survival rate and duration (45 days) with better powder yield when made using 20% maltodextrin. Similarly, Ahlawat et al. (2023)17 investigated the shelf life of spray-dried probiotic buttermilk powder. The result showed that the use of thermal protectants, i.e., whey protein concentrate (WPC), trehalose, and maltodextrin, maintained the viability of probiotic bacteria after spray drying and during storage. Hence, the use of spray drying for developing synbiotic legume-based beverage powder can be employed in the functional food industry at the commercial level.
Another important aspect associated with the improved shelf life of powdered food products is their storage at appropriate temperatures and packaging material. Several studies have reported refrigerated temperature (4 ± 1 °C) as best for maintaining better storage life. Nonetheless, a food-based powder must also retain its properties at ambient room temperature. Similarly, the type of packaging material used is also crucial in determining the shelf life of a powder food product.18 While glass bottles or jars can be expensive, the use of food grade polypropylene (PP) and polyethylene terephthalate (PET) pouches is more economical.19 Moreover, aluminum laminates can also be used because of its suitable barrier properties. Chauhan and Patil20 studied different packaging materials, namely, metal can, metalized polyester, 4-ply laminates (polyethylene–aluminium foil–polyethylene–paper), and polystyrene packages for storing mango milk powder (MMP). The authors concluded that storage in these packaging materials can preserve the MMP for 2, 4, 6 and 8 months, respectively, both at room (30 °C) and refrigerated (5 °C) temperature. Keeping in mind the different components of the powder product's composition, the types of packaging material, and storage temperatures were selected.
Extensive literature is available on the shelf-life study of various dairy-based probiotic food powders.21–23 Similarly, information on spray drying of non-dairy fruits24 and vegetable juices25 and cereal-based26 beverages is widely available. In our previous work,27 a comparative evaluation of spray-drying microencapsulation of Lacticaseibacillus casei in different synbiotic legume-based beverages was done. The results suggested that synbiotic KMB beverage encapsulated using gum acacia was the best formulated powder with desirable characteristics such as higher bulk density, lower porosity, survivability of probiotics under gastrointestinal conditions (>7
log CFU mL−1), and good encapsulation efficiency (>80%). The formulation also showed better encapsulation efficiency (>80%) and lower moisture content values (<5%) in comparison to other encapsulating agents, i.e., maltodextrin and xanthan gum. However, the work lacked data on the shelf-life study of legume-based beverage powders at different storage temperatures and in different packaging materials. Hence, the goal of this research was to assess the storage stability of the developed synbiotic kidney-mung bean beverage powder at varying storage temperatures when packaged using two different packaging materials to select the best storage parameters. The shelf life of the powder was judged based on certain quality parameters like total viable count of the probiotic, moisture content, color, and the growth of microbes like aerobic mesophiles, and yeast and mold. The sensory acceptability along with the prebiotic activity score of the synbiotic powder at the end of shelf life was also determined in different combinations of temperature and packaging materials.
Materials and method
Materials
Red kidney beans (Phaseolus vulga L.) and green mung beans (Vigna radiata L.) were supplied via local vendors near Matunga, Mumbai, India (19 °N, 72 °E). The probiotic (food grade), Lacticaseibacillus casei ATCC 335, was procured from NCL, Pune, India.
The chemicals and reagents used in the study were analytical grade and procured from Merck, India, or Sigma-Aldrich, Germany. The media used for microbial enumeration were purchased from HIMEDIA (Mumbai, Maharashtra, India).
Sample preparation and microencapsulation
The gum acacia (GA) coated kidney-mung bean (KMB) based synbiotic beverage powder was selected for the storage study. As per the results observed in the previous work,27 spray-dried microencapsulated GA-coated KMB synbiotic powder showed higher yield (7.3%) and better encapsulation efficiency (89.37%), and the growth of probiotic Lacticaseibacillus casei was >8
log CFU mL−1 when subjected to simulated gastrointestinal conditions as well as to intestinal bile juice and gastric acid. The obtained synbiotic powder also had lower moisture content (2.5%) and good powder characteristics (bulk density, 0.15 g cm−3; tapped density, 0.19 g cm−3; particle density, 1.13 g cm−3; porosity, 83.53%; and dissolution time, 34.50 s).
For its preparation, the synbiotic kidney-mung bean based beverage28 fermented using 1% v/v probiotic Lacticaseibacillus casei (>8
log CFU mL−1) was added with 2% GA and homogenized at 1500 rpm for 5 min. The mixture was then dried in a laboratory spray dryer (Spray Mate 2011CC, JISL, India) having a counter-current flow system and an atomizer of 0.7 mm internal diameter. The outlet and inlet temperatures of the drying chamber were set at 70 °C and 130 °C, respectively. The sample was fed with a flow rate of 25 mL min−1. The obtained powder was then packed in different packaging materials in a sterile environment and kept at different storage temperatures for shelf-life study.
Storage material and conditions
The storage stability of the optimized synbiotic KMB beverage powder was assessed at three temperatures, i.e., 25, 30, and 35 °C. The temperature range for storage was selected, keeping in mind the most suitable atmosphere (available at the commercial and household level) where the powder maintains its desirable properties along with the required probiotic count. We consider 25 °C as the ambient temperature in India. 35 °C is the most suitable temperature for aerobic mesophile growth and ensures the worst scenario. In this sense, these extreme limits were considered. 30 °C was chosen to have a middle point in the storage temperature axis to obtain the kinetic information. It is recommended that for obtaining any kinetic information, we must have at least three data points of the independent variables. The range was also selected based on the results obtained from preliminary trials. The selected packaging materials were aluminum (Al) (dimensions: 8 × 15 cm; thickness: 40 μm) and polypropylene (PP) (dimensions: 8 × 15 cm; thickness: 20 μm) laminates. The packaging materials were preferred based on the high barrier and mechanical properties.
The laminates were first subjected to surface decontamination by exposing them to UV light for 24 h in a laminar airflow chamber. Then the sample was filled in several pouches for both Al and PP laminates under sterile conditions. Thereafter, the samples were kept in the corresponding incubators, which were sanitized earlier using ethyl alcohol. The shelf-life analysis was performed for the different samples till the product spoils, with a key focus on the microbiological safety of the product. The samples were considered spoiled when the AMC or YMC surpassed 6
log CFU mL−1 (ref. 29) and the probiotic count reduced below 6
log CFU g−1.30 The sampling interval was 7 days. On each sampling day, two sets of each Al and PP laminate were taken out for analysis. The sample in the opened pouch was not further considered for storage. The study focused on the ‘shelf-life until the bag is open’.
Determination of quality characteristics
Color analysis.
The L*a*b* color profile of the synbiotic powder was assessed using a Hunter-Lab colorimeter (LabScan-XE LX17375, USA) where L* indicates lightness, a* the color range from red to green, and b* the values ranging from color yellow to blue.
Moisture content.
The AOAC (2005) method was used to estimate moisture content in the synbiotic powders.31 The Petridish with known sample weight (with lid open) was placed inside the oven set at 105 °C for 4 hours. The sample was then transferred to a desiccator for cooling and the weight of the Petri dish with sample was noted. This process was repeated till a constant weight was achieved. The difference in the weight before and after drying was used to determine the moisture content in the sample.
Total viable count (TVC).
The total viable count of probiotic Lacticaseibacillus casei in the reconstituted synbiotic powder (1 g in 100 mL) was determined by a serial dilution technique and spread plate method using a selective medium, de Mann Rogosa and Sharpe (MRS) agar. The prepared plates were kept at 37 °C for incubation for 24 h. The developed colonies were counted and expressed as log CFU mL−1 (colony forming units).
Viability in simulated gastric acid (VHCl).
The viable count of L. casei in synbiotic KMB beverage powder under simulated gastric acid (VHCl) was evaluated using the protocol by Rao et al. (1989).32 In brief, 1 mL of the reconstituted sample was mixed with 10 mL of gut acid (0.08 M HCl with 0.2% (v/v) NaCl without pepsin; pH 1.55) and kept at 37 °C. The sample was taken for spread plating at an interval of every 15 min for 2 hours. The prepared plates were incubated for 24 h at 37 °C following colony counting.
Viability in bile juice (VBile).
The ability of probiotic L. casei in synbiotic KMB beverage powder to tolerate and grow in a medium with bile juice (VBile) was determined as per the protocol by Halim et al. (2017).33 The KMB powder was first reconstituted by mixing 1 g in 100 mL distilled water. 1 mL of this reconstituted beverage was then added to 10 mL of 0.6% (v/v) bile salt solution (pH 8.25) in a tube and incubated at 37 °C. The sample was then subjected to plating after every 15 minute gap for 2 hours. The prepared plates were kept at 37 °C for 24 h for incubation. The obtained colonies were stated in terms of log CFU mL−1.
Enumeration of microbial load.
The microbial quality of the synbiotic KMB beverage powder was assessed by determining the growth of aerobic mesophiles (AM) and yeasts & molds (YM) in the reconstituted sample. The growth was observed by using specific media, i.e., nutrient agar and potato dextrose agar, for AM and YM count, respectively. 1 mL of reconstituted synbiotic beverage sample used for plating was added. The agar plates for YM plates were incubated at 25 °C for 3–5 days, while AM were incubated at 37 °C for 24 h. The developed colonies were expressed in terms of log CFU mL−1.
Prebiotic activity score (PAS).
The prebiotic activity score (PAS) of L. casei in the synbiotic KMB powder to metabolize different prebiotic components apart from the control sugar (glucose) was estimated using the method of Huebner et al. (2007).34 PAS of the reconstituted sample was calculated by enumerating probiotic growth with and without prebiotic components to the growth of enteric pathogens when subjected to a medium with and without prebiotics. The plating for both the microorganisms (pathogen and probiotic) was done via the spread plate technique using MRS agar and selective agar, respectively. The cell count was stated as log CFU mL−1.
Prebiotic activity score by probiotic L. casei was determined against enteric pathogen E. coli using eqn (1).
|  | (1) |
Sensory analysis.
The sensory analysis of reconstituted synbiotic KMB beverage was done at the Institute of Chemical Technology, Mumbai, by 30 semi-trained students and staff consisting of 15 female and 10 male participants with an age group of 26–40 years. For sensory analysis, the samples were made using food grade ingredients such as sodium bicarbonate and gum acacia. The probiotic used was also isolated from a food source. The selected panel members were non-smokers, non-alcoholic, and non-allergic to any plant/legume derived milk or similar beverage. Before the analysis, the members were briefed about non-dairy plant-based beverages like almond milk, soymilk, and pea milk, their processing and sensory profile as well as different sensory parameters, score sheet, and rating scale.35 The reconstituted sample at day zero and the final day of shelf life was served along with a control sample. The samples were served in transparent glasses at room temperature with random alpha-numeric codes. The members were instructed to clean their palate with lukewarm water after subsequent tasting to avoid any overpowering tastes. The panelists were asked to score the sensory attribute of the sample (Sk) on a 9-point hedonic scale (ranging from 1 being “dislike extremely” to 9 being “like extremely”) and the relative importance (RIk) of each sensory attribute on a 5-point scale (ranging from 1 being “not important” to 5 being “extremely important”). Scores of RIk were used to find the importance of individual sensory attributes and identify the attribute which is considered most important by the members. The overall acceptability (OA) of individual samples was calculated using the obtained relative importance (RIk) values and sensory scores (Sk) (eqn (2)).36 | 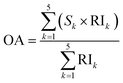 | (2) |
The OA value is used to identify the overall fondness among the different samples based on the likeness of the specific sensory attributes.
Predicting shelf-life.
The specific death rate (Kd; day−1) of the total viable count of L. casei in the synbiotic KMB beverage stored in PET and Al-coated PP bottles at 5 °C, 15 °C and 25 °C was obtained to fit the kinetic model using eqn (3):37 |  | (3) |
where Nt and N0 are the number of viable cells (CFU mL−1) at storage time t and storage day 0, respectively. The thermal death activation energy (Ea; J mol−1) was calculated to determine the minimum energy needed for killing probiotic bacteria, and thus, the temperature sensitivity of the probiotic was expressed as per eqn (4): | 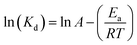 | (4) |
where A is the Arrhenius constant, T (K) is the absolute temperature, and R is the molar gas constant (8.3144 J K−2 mol−1), respectively.
FTIR analysis of synbiotic KMB powder during storage.
Fourier transform infrared (FTIR) spectroscopy was performed in attenuated total reflection (ATR) mode using a Shimadzu 8400s spectrometer (Japan). The spectra were scanned from 4000 to 400 cm−1 and measured at a 4 cm−1 resolution.
Statistical analysis
The experiments for all the protocols were performed and analyzed in triplicate. The statistical analysis was carried out by one-way analysis of variance (ANOVA) using the SPSS v20-Edition 20 (IBM SPSS Statistics Data Editor) to get the mean values of the different variables.
Results and discussion
Changes in the total viable count of the probiotic
The change in the growth of total viable count of probiotic L. casei in the synbiotic KMB powder during the storage period is depicted in Fig. 1. The effect of two different packaging materials, viz., polypropylene (PP) and aluminium (Al) laminates, on the synbiotic KMB powder when kept at three different storage temperatures, i.e., 25, 30, and 35 °C, was also studied.38 It can be observed from Fig. 1 that there was an overall decrease in the probiotic's viability during the storage period. The count in the reconstituted synbiotic powder (1 g in 100 mL water) at all three storage temperatures remained >8
log CFU mL−1 till the 35th and 21st days, when packaged in PP and Al laminates, respectively. However, the count started to lower down below 7
log CFU mL−1 (minimum probiotic population limit recommended by FAO39) after the 63rd and 56th days at 25 °C. This trend was also seen at higher storage temperatures, 30 and 35 °C, for both the packaging materials. However, it was also noticed that though the viable count remained more than 107 CFU mL−1 till the 8th week of storage, the physical structure of the synbiotic powder became coarse and difficult to reconstitute by the 35th day of storage at 30 and 35 °C. The results were similar to those of Dias et al. (2018)40 who observed that the viability of probiotic Bifidobacterium animalis ssp. Lactis BB-12 in spray-dried passion fruit juice encapsulated with maltodextrin and inulin at 25 °C was >7
log CFU mL−1 for 30 days. Massounga Bora et al. (2019)41 also observed that banana powder inoculated with probiotic L. casei exerted survival for 30 days at 25 °C with a maintained count of more than 106 CFU mL−1. This may be because of the ability of probiotic L. casei to tolerate higher storage temperatures. On the other hand, Singh et al. (2019)42 reported the highest growth (6.83
log CFU mL−1) of probiotic Lactobacillus gastricus BTM7 in alginate encapsulated-based skimmed milk powder when stored at 4 °C for 21 days. In this sense, non-dairy legume based instant powders retain better probiotic properties for longer storage periods even at room temperature.
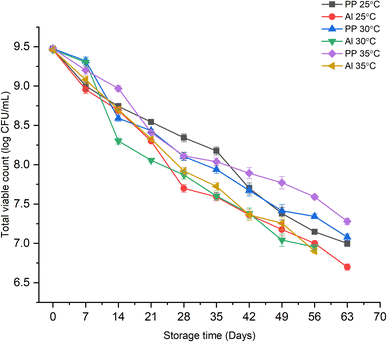 |
| Fig. 1 Effect of storage time, temperature, and packaging material on the total viable count of probiotic L. casei in synbiotic KMB powder. | |
Changes in the viability of the probiotic under simulated gastric juices
Changes under gastric acid.
The viability of probiotic microorganisms under gastrointestinal surroundings is a major barrier in proving their effectiveness. The bacteria must tolerate the harsh and vulnerable pH of the gastric juice and small intestine's fluids to exhibit their beneficial functions. Fig. 2 illustrates the effect of different storage conditions on the growth of probiotic bacteria in synbiotic KMB powder under simulated gastric acid of the human gastrointestinal tract. The viability of L. casei in synbiotic KMB powder remained up to the desirable count of 7
log CFU mL−1 of reconstituted beverage (or 9
log CFU g−1 of powder) till the 42nd day at 25 °C, and the 28th day at 30 and 35 °C. This trend was seen throughout the storage period irrespective of any significant difference in the type of packaging material used. However, at 35 °C, a sudden decrease in the viable count was seen from 8.01 ± 0.03
log CFU mL−1 on day 21 to 7.08 ± 0.02
log CFU mL−1 on day 28, after which the viability went below 107 CFU mL−1. Similarly, a significant drop (from 8.98 to ≈7.90
log CFU mL−1) in the viable count of the probiotic under gastric acid was observed at a storage temperature of 30 °C in the first week of storage for both the packaging materials.
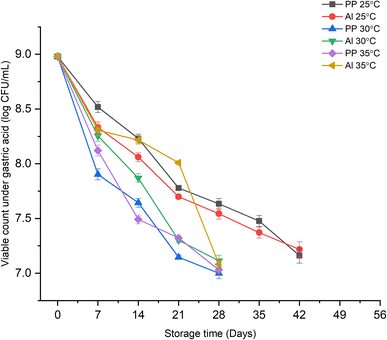 |
| Fig. 2 Effect of storage time, temperature, and packaging material on the viable count of probiotic L. casei under simulated gastric HCl in synbiotic KMB powder. | |
Dianawati et al. (2017)38 also observed that storage of different protein-based microcapsules (soy protein isolate, sodium caseinate, or pea protein) at 30 and 35 °C significantly affected (P < 0.05) the viability of probiotic Lactobacillus acidophilus as compared to that at 25 °C when exposed for 2 h to simulated gastric juice. Among the different proteins, only sodium caseinate could sustain the required count of 107 CFU mL−1 on the 8th week of storage at the different temperatures. The reason could be extremely low pH in gastric fluid together with the higher storage temperatures (30 and 35 °C) that can prove to be very distressful for probiotics when passing through it. Bradford et al. (2019)43 reported that after exposure of the probiotic milk powders to simulated gastric fluid for up to 120 min in SGF, they showed a survival count of more than 8
log CFU g−1 on the 15th day of refrigerated storage. Considering this, the survivability of our sample was also >8
log CFU mL−1 even after 15 days of storage at all three temperatures indicating the ability of the legume-based matrix as a potential probiotic carrier.
Changes under gastric bile juice.
The effect of growth of L. casei under simulated gastric bile juice at different storage conditions is shown in Fig. 3. The growth trend of the probiotic under simulated bile juice was in similar lines to that observed under gastric acid. Regardless of the packaging material type, the viable count up to 7
log CFU mL−1 was observed till day 49 at 25 °C and the 28th day at higher storage temperatures of 30 and 35 °C. Moreover, an abrupt decrease in the viable count from 8.28
log CFU mL−1 on day 7 to 7.40
log CFU mL−1 on day 14 was observed in case of both the packaging laminates. Similar results were reported by Dianawati et al. (2017)38 where the three studied protein-based microspheres were able to maintain bacterial viability in simulated intestinal fluids at 25 and 30 °C for week 4. However, a significant decrease in survival (P < 0.05) was observed at 35 °C by the end of week 8. This could be because of the effect exerted by the bile salts which emulsify the phospholipid bilayer of bacteria and cause leakage of cell cytoplasm and dissociation of integral protein, thereby decreasing the total cell count.44 Additionally, the unfavorable higher storage temperatures also interrupt the growth of the cells. Bradford et al. (2019)43 also showed that after a storage period of 15 days at 4 °C, the viable count of probiotic Lactobacillus plantarum NCIMB decreased to <107 CFU mL−1 in milk powders, when the reconstituted mixture was subjected to the intestinal fluid for 120 min. In comparison, our samples showed better tolerance and survivability to simulated bile juices even at higher storage temperatures, owing to the potential of legume-based matrices as novel synbiotic components.
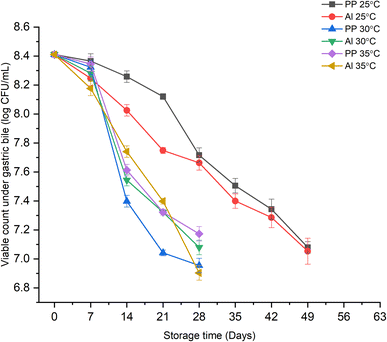 |
| Fig. 3 Effect of storage time, temperature, and packaging material on the viable count of probiotic L. casei under simulated bile juice in synbiotic KMB powder. | |
Microbiological activity
The trend of aerobic mesophiles count (AMC) and yeast and mold count (YMC) for the synbiotic powder during the storage period at different temperatures (25, 30, and 35 °C) in different packaging materials (Al and PP laminates) is depicted in Fig. 4. At a storage temperature of 25 °C, the growth of aerobic mesophiles (AM) remained less than 1
log CFU mL−1 till day 49 (Fig. 4(a)). After this, the viability reached 3
log CFU mL−1 on the 63rd day. However, the attained count was still less than 6
log CFU mL−1, which is the limit set for AM. In contrast, at higher storage temperatures (30 and 35 °C), the count was below 1
log CFU mL−1 till day 21, after which an increase in the count to 4.6
log CFU mL−1 was observed till the end of the storage period (63rd day) though it remained <6
log CFU mL−1. A similar trend for growth was observed in the case of yeast and mold (YM) for all three storage temperatures when the synbiotic KMB powder was packaged in two different packaging materials (Fig. 4(b)). An insignificant difference among the growth values was observed when different laminate pouches were used, indicating the negligible effect of packaging material on the growth of microorganisms. Thus, in this sense, the shelf life of the synbiotic KMB powder, irrespective of packaging material, can be considered to be above 70 days if considering only the growth of AM and YM. Similar findings were reported by El-Sayed et al. (2020)45 for probiotic yogurt powder wherein the product was free of mold and yeast for three months, after which the count increased to 1.70
log CFU g−1.
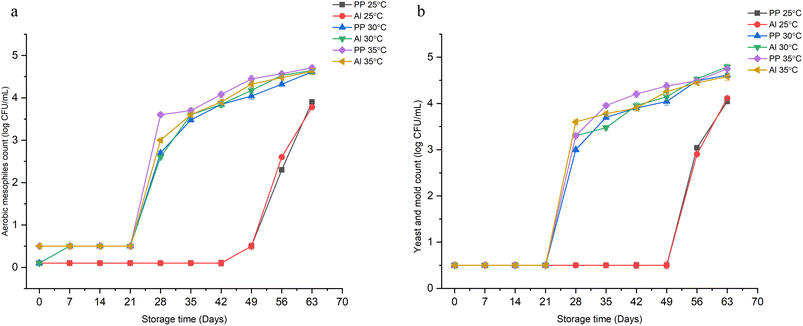 |
| Fig. 4 Effect of storage time, temperature, and packaging material on the growth of (a) aerobic mesophiles and (b) yeast and mold, in synbiotic KMB powder. | |
Change in color profile
Fig. 5 depicts the effect of storage time and temperature on the color profile in terms of the lightness value (L*) of the prepared synbiotic KMB powder. The color of the powder has shown an overall decrease in the lightness value from 70.44 to 37.76. This indicates that as the storage time increases, the powder becomes darker in color, irrespective of the type of packaging material at all three storage temperatures. The dark or brownish color of the powder might be attributed to the formation of degradation products.46 Dias et al. (2018)40 reported similar results for microencapsulated probiotic passion fruit juice. The authors concluded that the color change could be because of the oxidation of the pigments present in the product along with the additive effect exerted by heat, humidity, and exposure to oxygen. The lightness value (L*) increased from 90.85 on day 1 of storage to 92.54 on day 30 at 25 °C. Moreover, at the storage temperature of 25 °C, the lightness values for PP and Al-laminates were 43.58 ± 0.03 and 44.25 ± 0.05, respectively, by the end of the shelf life (day 70), which was the highest among all the storage temperatures indicating its desirability for the product. The type of packaging material, at an individual storage temperature, also has not shown any significant impact on changing the color of the synbiotic KMB powder.
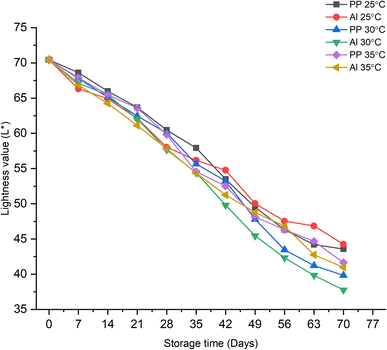 |
| Fig. 5 Effect of storage time, temperature, and packaging material on the lightness of synbiotic KMB powder. | |
Changes in moisture content
The effect of various storage conditions on the moisture content of the developed synbiotic KMB powder is shown in Fig. 6. It can be noticed that as the storage period increases, the moisture content of the powder also increases. Moreover, the type of packaging material did not show any significant effect on the increase or decrease in the moisture content of the powder. The values lie close to each other throughout the storage period when considering a specific storage temperature. For example, at 25 °C, the moisture content of the synbiotic powder remained less than 3% (w.b., wet basis) till the 6th week of storage when stored in PP and Al-laminates with values of 2.8% and 2.9% on the 42nd day. After this, the product gained some more moisture and reached a value of 4.4% and 4.5% for PP and Al-pouches, respectively, by the end of 70 days of storage. Nonetheless, the value remained at less than 5%, considered acceptable for a powder food product as per the recommended guidelines by CODEX standards.47 On the other hand, at storage temperatures of 30 and 35 °C, the moisture content remained less than 5% till days 63 and 56, respectively.
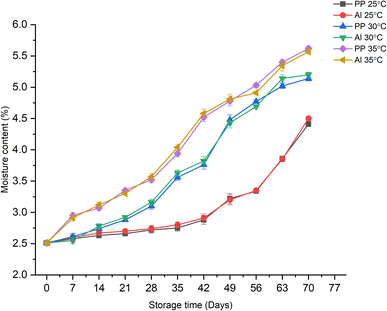 |
| Fig. 6 Effect of storage time, temperature, and packaging material on the moisture content values of synbiotic KMB powder. | |
Moreover, lumpiness and coarseness in the powder particles were also observed after day 35 for both temperatures and the packaging materials (Fig. 7). This may be due to the retention of moisture by the powder at higher temperatures and interaction with the encapsulating material, i.e., gum acacia, which caused disintegration in the powder structure resulting in its poor reconstitution properties. Algaithi et al.44 noticed similar results for the infant formula of Lactobacillus reuteri fortified camel milk. They observed an increase in lactose crystallinity and surface fat content leading to a drastic increase in caking at higher storage temperatures (30–45 °C) on the 30th day. In contrast, storage at room temperature (25 °C) prevented lactose crystallization and caking owing to smaller lump particle size even on the 60th day of storage. The changes in moisture in the powder inside the packaging material without being opened varied maximum up to 1.5% (wet basis). This might be due to the absorption of moisture by the hygroscopic sugars inside the synbiotic KMB powder. The humidity of the air inside the package was not measured in the study.
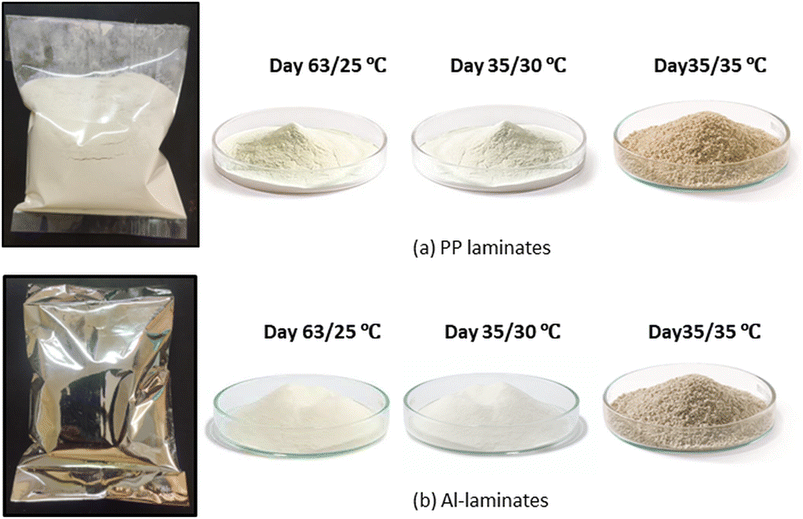 |
| Fig. 7 Physical appearance of synbiotic KMB powder by the end of shelf-life at both the storage temperatures for both the packaging materials (a) PP laminates and, (b) Al-laminates. | |
Change in prebiotic activity score (PAS)
The effect of different storage conditions on the prebiotic activity score of the synbiotic KMB powder is shown in Table 1. It can be observed that among the two-packaging materials used for storage of synbiotic KMB powder, a better PAS was observed by Al-laminate pouches at the three storage temperatures when compared to PP laminates. Moreover, the synbiotic powder showed the highest PAS at 30 °C on day 35. However, the PAS at 25 °C was 0.13 on day 63, which is comparatively better than the values at higher storage temperatures (30 and 35 °C). This could be because of the better barrier properties (low WVTR and OTR, 0.29 and 0.21 cm3 m−2/24 h/25 °C, respectively) exhibited by aluminum in comparison to polypropylene laminates (WVTR and OTR, 1.4 and 2701.73 cm3 m−2/24 h/25 °C, respectively) that allowed the preservation of the synbiotic KMB powder's probiotic and prebiotic properties. Similarly, Zhu et al. (2019)48 investigated the prebiotic activity of infant formula milk powder samples with oligosaccharides during storage of 48 days. The results concluded that with the increasing storage period, the growth of the probiotic decreased owing to the uptake of prebiotics by the probiotics.
Table 1 Effect of storage conditions on the prebiotic activity score of the synbiotic KMB powdera
Prebiotic activity score (PAS) at different storage temperatures |
|
RT |
25 °C |
30 °C |
35 °C |
Values are presented as mean ± standard error at a 95% confidence interval. Abbreviations: RT, room temperature (25 ± 2 °C); PET, polyethylene terephthalate; Al-PP, aluminum-coated polypropylene.
|
Packaging material |
Day 0 |
Day 63 |
Day 35 |
Day 35 |
PP laminate |
0.18 ± 0.12 |
0.12 ± 0.05 |
0.15 ± 0.01 |
0.15 ± 0.07 |
Al laminate |
0.19 ± 0.04 |
0.13 ± 0.10 |
0.16 ± 0.08 |
0.14 ± 0.17 |
Change in overall sensory acceptability
Table 2 describes the effect of different storage conditions, packaging materials, and storage temperatures on the overall sensory acceptability of the synbiotic KMB powder on reconstitution on day 0 and at the end of shelf life. It can be observed that synbiotic KMB powder showed slightly better OA values on reconstitution when stored in Al-laminates (5.03–6.09) in comparison to that packed in PP laminates (5.04–5.87). The highest OA value of 6.09 was shown by synbiotic KMB reconstituted powder drink packed in Al-laminate on day 35 and stored at 30 °C. The reconstituted drink from synbiotic KMB powder stored at 25 °C showed an OA of 5.03 on the last day of the shelf life. Marcela de Souza Pereira et al. (2018)49 conducted a similar study on sensory evaluation of dairy beverages made using reconstituted goat whey powder and fermented using Streptococcus thermophilus and Lactobacillus casei. The authors reported that the sensory acceptability did not differ significantly even after 7, 14, and 21 days of storage (P > 0.05). In contrast, Atwaa et al. (2022)50 reported a decrease in the overall acceptance of probiotic yogurt made from whole milk powder reconstituted in aqueous fennel extract during storage at 5–7 °C for 21 days because of an increased acidic flavor. This may be due to the probiotic bacteria producing high amounts of acetic acid.
Table 2 Effect of storage conditions on the overall acceptability (OA) sensory values of the synbiotic KMB powdera
Overall acceptability (OA) (out of 9) at different storage temperatures |
|
RT |
25 °C |
30 °C |
35 °C |
Values are presented as mean ± standard error at a 95% confidence interval. Abbreviations: RT, room temperature (25 ± 2 °C); PET, polyethylene terephthalate; Al-PP, aluminum-coated polypropylene.
|
Packaging material |
Day 0 |
Day 63 |
Day 35 |
Day 35 |
PP laminate |
6.70 ± 0.13 |
5.04 ± 0.01 |
5.87 ± 0.08 |
5.80 ± 0.05 |
Al laminate |
6.90 ± 0.08 |
5.03 ± 0.08 |
6.09 ± 0.13 |
5.92 ± 0.04 |
Shelf-life prediction of synbiotic legume-based beverage powder
The rate constant (kd) represents the rate at which probiotic growth declined during storage at different temperatures and in different packaging materials. The Arrhenius model (refer to eqn (4)) (Table 3) for different packaging materials explained the temperature dependence of probiotic survival rate. It can be observed from Table 3 that as the storage temperature increased, the value of kd also increased for synbiotic KMB powder in Al-laminates, indicating faster probiotic sensitivity at higher temperatures. A higher thermal diffusivity of Al-laminates may positively influence the probiotic sensitivity to temperature. This could be correlated with the fact that higher temperatures allow the disintegration of the probiotic's cell material leading to its inactivity and reduced count with increasing number of storage days.51 On the other hand, kd decreased with increasing storage temperature for synbiotic KMB powder in PP-laminates indicating a limited probiotic sensitivity at higher temperatures. The maximum rate constant value for L. casei in synbiotic KMB powder (0.045 day−1) was observed in Al-laminates stored at 30 and 35 °C. In all samples, the curves were explained by the linear regression (Table 4) with different slopes, and thus, diverse Ea (4.73–11.16 J mol−1) values were obtained for both the packaging materials.
Table 3 Shelf-life prediction model of the synbiotic KMB powdera
Storage temperature |
Packaging material |
k
d (day−1) |
R
2
|
E
a (J mol−1) |
Values are presented as mean ± standard error at a 95% confidence interval. Abbreviations: kd, specific death rate value; Ea, activation energy; PET, polyethylene terephthalate; Al-PP, aluminum-coated polypropylene.
|
PP |
25 °C |
0.038 |
0.98 |
11.16 |
30 °C |
0.038 |
0.96 |
35 °C |
0.033 |
0.95 |
Al |
25 °C |
0.042 |
0.96 |
4.73 |
30 °C |
0.045 |
0.93 |
35 °C |
0.045 |
0.98 |
Table 4 Estimated shelf-life of the synbiotic KMB beverage based on the probiotic viability and microbial safety values when stored at different temperatures and under different packaging conditionsa
Packaging material |
Estimated shelf life (in days) based on different criteria and storage temperature |
TVC (≥7 log CFU mL−1 of reconstituted drink) |
V
HCl & Vbile (≥6 log CFU mL−1 of reconstituted drink) |
AMC & YMC (≤6 log CFU g−1 powder) |
* indicates the shelf life of the powder till viable count remained ≥7 log CFU mL−1 but with lumpy powder structure while the values in brackets indicate the actual shelf life of the powder with viable count ≥7 log CFU mL−1 and desirable powder structure. Abbreviations: TVC, total viable count; VHCl & Vbile, viability in gastric acid and bile; AMC, aerobic mesophilic count; YMC, yeast and mold count; PET, polyethylene terephthalate; PP, polypropylene; Al, aluminium.
|
Powder
|
25 °C
|
30 °C
|
35 °C
|
25 °C
|
30 °C
|
35 °C
|
25 °C
|
30 °C
|
35 °C
|
PP laminate |
63 |
63* (35) |
70* (35) |
49 |
28 |
28 |
>63 |
>63 |
>70 |
Al laminate |
63 |
49* (35) |
70* (35) |
49 |
28 |
28 |
>63 |
>63 |
>70 |
Shelf-life estimation
The estimated shelf-life of synbiotic KMB powder based on the probiotic viability and microbial safety values when stored at different temperatures and under different packaging conditions is presented in Table 4. The shelf-life of synbiotic powder in PP and Al-laminates was 63, 35, and 35 days at 25, 30, and 35 °C, respectively, wherein both the packaging materials showed similar storage time. The powder at these estimated shelf life days showed the total probiotic count >7
log CFU mL−1 or >9
log CFU g−1 of powder, as suggested by FAO/WHO (2001). Similarly, the viability of the probiotic under simulated intestinal fluids, i.e., gastric acid and bile juice, should be >6
log CFU mL−1 of reconstituted drink (or >8
log CFU g−1 of powder).52 In this sense, the powder showed resistance and survivability of L. casei with a count of more than 106 CFU mL−1 for 49, 28, and 28 days at 25, 30, and 35 °C, respectively, after which the count gradually decreased which may be due to the increased acidity of the probiotic itself together with the gut's acidic fluids.53
In the case of microbial contamination, the maximum tolerable limit for yeast and molds as well aerobic mesophiles is <6
log CFU mL−1.52 The sample was safe from the microbiological point of view with YMC and AMC less than 106 CFU mL−1 and the count remained below this limit even beyond 70 days of storage at all three temperatures (25, 30 and 35 °C). Moreover, the type of packaging material did not show any significant influence on the growth of microorganisms, indicating use of either PP or Al-pouches for packaging of synbiotic powders.
FTIR spectra of synbiotic KMB powder during storage
The Fourier-transform infrared (FTIR) spectra of synbiotic KMB powder at the different storage temperatures were studied at the wavenumber range of 400 to 4000 cm−1 to detect the main components in the samples. The recorded spectra are shown in Fig. 8. Attenuated total reflection (ATR)-FTIR analysis showed the presence of organic functional groups (hydrogen bonds and O–H stretching (3000–3500 cm−1), C–H bonds (2850–3000 cm−1), C–O stretching (1138 and 1056 cm−1), C–H bending (927 cm−1), amide I & II (1539 and 1633 cm−1), COOH (1449 cm−1)) and interactions between the beverage powder and carrier in the synbiotic KMB powder on day 0 as well as the end of storage period at room temperature (25 °C) (Fig. 8(a) and (b)). Similar results were observed by Kouamé et al. (2023)54 for millet based yogurt powder wherein the band at 2860 cm−1 corresponds to the distinctive peak of the C–H stretching vibration of millet starch polysaccharides (amylose, amylopectin).
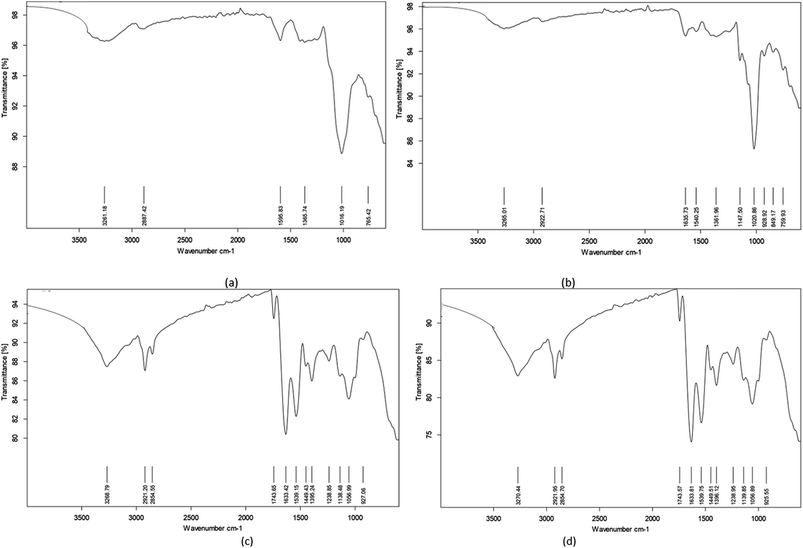 |
| Fig. 8 FTIR spectra of synbiotic KMB powder at (a) room temperature (25 °C), day 0; (b) 25 °C, day 63; (c) 30 °C, day 35; and (d) 35 °C, day 35. | |
On the other hand, a new peak at the wavenumber range of 1600 to 1750 cm−1 was noticed at higher storage temperatures of 30 and 35 °C by the end of the storage period (35 days) (Fig. 8(c) and (d)). This was attributed to carbonyl group C
O double bond stretching.55 Algaithi et al.44 also observed similar peaks for probiotic camel milk infant formula and noticed the development of new peaks by the end of storage as a result of product crystallization. Hence, the bands at 1000 cm−1 in the spectra are characteristic of carbohydrates owing to the presence of encapsulating material, namely, gum acacia. Thus, it can be concluded that the presence of different compounds as shown by the different bands allows the formulation of food with added benefits from legume-based food substrates to serve as an ideal medium for allowing the desirable growth of probiotics, thereby making a synbiotic food product.
Conclusion
The processing of liquid products to powder form has often been considered as one of the most common methods to extend the shelf life of a product in the food industry. However, the shelf life and the associated quality attributes of such powder products also depend on the type of packaging material and storage conditions. Hence, the prepared microencapsulated and spray-dried synbiotic KMB powder was subjected to storage at three temperatures (25 °C, 30 °C, and 35 °C) when packed in PP and Al laminates. A gradual decrease in TVC of the probiotic L. casei throughout the storage period was observed though the product maintained the acceptable limit (107 CFU mL−1) till the end. Similarly, VHCl and VBile also showed decreased count. AM and YM counts were <3
log CFU mL−1 till day 70, which was below the recommended guidelines. The change in color of the powder showed that as the storage time increases, the powder tends to become darker in color. The powder gained moisture and became lumpy when kept at higher temperatures (30 °C and 35 °C) for a longer duration, though it maintained its structure at 25 °C. The PAS (0.19–0.13) and OA (6.90–5.03) values of the product suggested that among the two packaging materials, the Al-laminate pouch was better. The FTIR analysis also showed the development of a new functional group (carbonyl group C
O double bond stretching). Thus, based on the calculated kinetic value of the chief parameters, and overall quality attributes, the shelf life of the synbiotic KMB powder was 63, 35, and 35 days at 25, 30, and 35 °C, respectively, wherein both the packaging materials showed similar storage time. Future studies can be carried out to understand the morphological changes in the instant powder before, after, and during storage at different storage temperatures. Hence, it can be concluded that the longer shelf-life of synbiotic KMB powder at appropriate temperatures makes it a suitable matrix for probiotic delivery with preserved probiotic and prebiotic properties to be used as an instant-function food powder which holds the potential to exhibit several health benefits to humans.
Ethical approval
Ethical approval was not required for this research. There was no coercion to participate for sensory evaluation, full disclosure of study requirements and risks, written or verbal consent of participants, no release of participant data without their knowledge, and the ability to withdraw from the study at any time.
Data availability
Research data are not shared.
Author contributions
Smriti Chaturvedi: conceptualization, methodology, software, formal analysis, data curation, investigation, resources, visualization, funding acquisition, writing – original draft, writing – review & editing. Snehasis Chakraborty: conceptualization, supervision, validation, writing – review & editing.
Conflicts of interest
The authors have declared no conflicts of interest for this article.
Acknowledgements
S. Chaturvedi wants to thank the National Doctoral Fellowship scheme under the All-India Council for Technical Education, Government of India, for providing fellowship during the research.
References
- C. S. Ranadheera, J. K. Vidanarachchi, R. S. Rocha, A. G. Cruz and S. Ajlouni, Probiotic delivery through fermentation: Dairy vs. non-dairy beverages, Fermentation, 2017, 3, 1–17 CrossRef.
- F. Fratianni, F. Cardinale, I. Russo, C. Iuliano, P. Tremonte, R. Coppola and F. Nazzaro, Ability of synbiotic encapsulated Saccharomyces cerevisiae boulardii to grow in berry juice and to survive under simulated gastrointestinal conditions, J. Microencapsulation, 2014, 31, 299–305 CrossRef CAS.
- A. Atia, A. Gomaa, I. Fliss, E. Beyssac, G. Garrait and M. Subirade, A prebiotic matrix for encapsulation of probiotics: physicochemical and microbiological study, J. Microencapsulation, 2016, 33, 89–101 CrossRef CAS PubMed.
- S. Chaturvedi and S. Chakraborty, Review on potential non-dairy synbiotic beverages: a preliminary approach using legumes, Int. J. Food Sci. Technol., 2021, 56, 2068–2077 CrossRef CAS.
- D. M. D. Rasika, J. K. Vidanarachchi, S. F. Luiz, D. Rosane, P. Azeredo, A. G. Cruz and C. S. Ranadheera, Probiotic Delivery through Non-Dairy Plant-Based Food Matrices, Agriculture, 2021, 11, 599 CrossRef CAS.
- O. L. Mondragón-Bernal, J. G. L. F. Alves, M. A. Teixeira, M. F. P. Ferreira and F. Maugeri Filho, Stability and functionality of synbiotic soy food during shelf-life, J. Funct. Foods, 2017, 35, 134–145 CrossRef.
- M. K. Sidhu, F. Lyu, T. P. Sharkie, S. Ajlouni and C. S. Ranadheera, Probiotic yogurt fortified with chickpea flour: Physico-chemical properties and probiotic survival during storage and simulated gastrointestinal transit, Foods, 2020, 9, 1–13 Search PubMed.
- F. Zare, J. I. Boye, C. P. Champagne, V. Orsat and B. K. Simpson, Probiotic Milk Supplementation with Pea Flour: Microbial and Physical Properties, Food Bioprocess Technol., 2013, 6, 1321–1331 CrossRef CAS.
- L. Mojica and E. G. de Mejía, Characterization and Comparison of Protein and Peptide Profiles and their Biological Activities of Improved Common Bean Cultivars (Phaseolus vulgaris L.) from Mexico and Brazil, Plant Foods Hum. Nutr., 2015, 70, 105–112 CrossRef CAS.
- D. Hou, Q. Zhao, L. Yousaf, Y. Xue and Q. Shen, In Vitro Starch Digestibility and Estimated Glycemic Index of Mung Bean (Vigna radiata L.) as Affected by Endogenous Proteins and Lipids, and Exogenous Heat-Processing Methods, Plant Foods Hum. Nutr., 2020, 75, 547–552 CrossRef CAS PubMed.
- M. A. Nawaz, M. Tan, S. Øiseth and R. Buckow, An Emerging Segment of Functional Legume-Based Beverages: A Review, Food Rev. Int., 2020, 1–39 Search PubMed.
- Y. Y. Murevanhema and V. A. Jideani, Shelf-life characteristics of Bambara groundnut (Vigna subterranea (L.)Verdc) probiotic beverage, Afr. J. Sci. Technol. Innov. Dev., 2020, 12, 591–599 CrossRef.
- C. González-Ferrero, J. Manuel Irache, B. Marín-Calvo, L. Ortiz-Romero, R. Virto-Resano and C. Javier González-Navarro, Encapsulation of probiotics in soybean protein-based microparticles preserves viable cell concentration in foods all along the production and storage processes, J. Microencapsulation, 2020, 37, 242–253 CrossRef.
- M. H. Mousavi, M. Gharekhani, K. Alirezalu, L. Roufegarinejad and S. Azadmard-Damirchi, Production and characterization of nondairy gluten-free fermented beverage based on buckwheat and lentil, Food Sci. Nutr., 2023, 11, 2197–2210 CrossRef CAS.
- F. Mainente, A. Piovan, F. Zanoni, R. Chignola, S. Cerantola, S. Faggin, M. C. Giron, R. Filippini, R. Seraglia and G. Zoccatelli, Spray-drying Microencapsulation of an Extract from Tilia tomentosa Moench Flowers: Physicochemical Characterization and in Vitro Intestinal Activity, Plant Foods Hum. Nutr., 2022, 77, 467–473 CrossRef CAS PubMed.
- R. Upadhyay and F. P. Dass J, Physicochemical analysis, microbial survivability, and shelf life study of spray-dried synbiotic guava juice powder, J. Food Process. Preserv., 2021, 45, 1–8 Search PubMed.
- A. Ahlawat, S. Basak and L. Ananthanarayan, Formulation of a probiotic buttermilk powder using cell protectants by spray drying and estimation of its shelf-stability, Int. Dairy J., 2023, 141, 105616 CrossRef CAS.
- D. Saha, S. K. Nanda and D. N. Yadav, Optimization of spray drying process parameters for production of groundnut milk powder, Powder Technol., 2019, 355, 417–424 CrossRef CAS.
- Z. Zorić, Z. Pelaić, S. Pedisić, I. Elez Garofulić, D. Bursać Kovačević and V. Dragović-Uzelac, Effect of storage conditions on phenolic content and antioxidant capacity of spray dried sour cherry powder, LWT--Food Sci. Technol., 2017, 79, 251–259 CrossRef.
- A. K. Chauhan and V. Patil, Effect of packaging material on storage ability of mango milk powder and the quality of reconstituted mango milk drink, Powder Technol., 2013, 239, 86–93 CrossRef CAS.
- M. E. Herkenhoff, I. U. Dantas De Medeiros, L. Henrique, G. Garutti, M. K. Salgaço, K. Sivieri, S. Marta and I. Saad, Cashew By-Product as a Functional Substrate for the Development of Probiotic Fermented Milk, Foods, 2023, 12, 3383 CrossRef CAS PubMed.
- Y. H. How, M. Y. M. Teo, L. L. A. In, S. K. Yeo, B. Bhandari and L. P. Pui, Powder characteristics, moisture sorption isotherm, and shelf-life prediction of freeze-dried recombinant Lactococcus lactis NZ3900-fermented milk powder, Drying Technol., 2023, 1–15 Search PubMed.
- S. Yadav and S. Mishra, Moisture sorption isotherms and storage study of spray-dried probiotic finger millet milk powder, J. Stored Prod. Res., 2023, 102, 102128 CrossRef CAS.
- D. Kalita, S. Saikia, G. Gautam, R. Mukhopadhyay and C. L. Mahanta, Characteristics of synbiotic spray dried powder of litchi juice with Lactobacillus plantarum and different carrier materials, LWT--Food Sci. Technol., 2018, 87, 351–360 CrossRef CAS.
- Y. F. Looi, S. P. Ong, A. Julkifle and M. S. Alias, Effects of pretreatment and spray drying on the physicochemical properties and probiotics viability of Moringa (Moringa oleifera Lam) leaf juice powder, J. Food Process. Preserv., 2019, 43, 1–15 Search PubMed.
- D. P. M. Anitha and P. S. Sellamuthu, Microencapsulation of probiotics in finger millet milk complex to improve encapsulation efficiency and viability, Food Sci. Technol. Int., 2022, 28, 216–232 CrossRef CAS PubMed.
- S. Chaturvedi and S. Chakraborty, Comparative analysis of spray-drying microencapsulation of Lacticaseibacillus casei in synbiotic legume-based beverages, Food Biosci., 2022, 50, 102139 CrossRef CAS.
- S. Chaturvedi and S. Chakraborty, Optimization of fermentation conditions of synbiotic legume-based beverages and study of their antimicrobial and proteolytic activity, J. Food Sci., 2022, 87, 5070–5088 CrossRef CAS.
- S. Chakraborty, P. S. Rao and H. N. Mishra, Changes in Quality Attributes During Storage of High-Pressure and Thermally Processed Pineapple Puree, Food Bioprocess Technol., 2016, 9, 768–791 CrossRef.
- C. S. Ranadheera, C. A. Evans, S. K. Baines, C. F. Balthazar, A. G. Cruz, E. A. Esmerino, M. Q. Freitas, T. C. Pimentel, A. E. Wittwer, N. Naumovski, J. S. Graça, A. S. Sant'Ana, S. Ajlouni and T. Vasiljevic, Probiotics in Goat Milk Products: Delivery Capacity and Ability to Improve Sensory Attributes, Compr. Rev. Food Sci. Food Saf., 2019, 18, 867–882 CrossRef CAS.
-
AOAC, Determination of Moisture, Ash, Protein and Fat, Washington DC, 2005 Search PubMed.
- A. V. Rao, N. Shiwnarain and I. Maharaj, Survival of Microencapsulated Bifidobacterium pseudolongum in Simulated Gastric and Intestinal Juices, Can. Inst. Food Sci. Technol. J., 1989, 22, 345–349 CrossRef.
- M. Halim, N. A. Mohd Mustafa, M. Othman, H. Wasoh, M. R. Kapri and A. B. Ariff, Effect of encapsulant and cryoprotectant on the viability of probiotic Pediococcus acidilactici ATCC 8042 during freeze-drying and exposure to high acidity, bile salts and heat, LWT--Food Sci. Technol., 2017, 81, 210–216 CrossRef CAS.
- J. Huebner, R. L. Wehling and R. W. Hutkins, Functional activity of commercial prebiotics, Int. Dairy J., 2007, 17, 770–775 CrossRef CAS.
-
Sensory Testing Methods: American Society for Testing and Materials Manual, Astm Intl, ed. E. Chambers & M. B. Wolf, 2nd edn, January 1, 1996, 1996 Search PubMed.
- S. Chakraborty, P. S. Rao and H. N. Mishra, Response Surface Optimization of Process Parameters and Fuzzy Analysis of Sensory Data of High Pressure-Temperature Treated Pineapple Puree, J. Food Sci., 2015, 80, E1763–E1775 CrossRef CAS.
- S. Seyedzadeh-Hashemi, V. Mofid, S. M. Hosseini, S. M. T. Gharibzahedi, A. M. Mortazavian and S. Shojaee-Aliabadi, Characterization of synbiotic films based on carboxymethyl cellulose/β-glucan and development of a shelf life prediction model, Food Biosci., 2023, 51, 102228 CrossRef CAS.
- D. Dianawati, S. F. Lim, Y. B. H. Ooi and N. P. Shah, Effect of Type of Protein-Based Microcapsules and Storage at Various Ambient Temperatures on the Survival and Heat Tolerance of Spray Dried Lactobacillus acidophilus, J. Food Sci., 2017, 82, 2134–2141 CrossRef CAS PubMed.
-
FAO/WHO, Joint FAO/WHO Working Group Report on Drafting Guidelines for the Evaluation of Probiotics in Food, London, Ontario, Canada, 2001 Search PubMed.
- C. O. Dias, J. dos Santos Opuski de Almeida, S. S. Pinto, F. C. de Oliveira Santana, S. Verruck, C. M. O. Müller, E. S. Prudêncio and R. D. de Mello Castanho Amboni, Development and physico-chemical characterization of microencapsulated bifidobacteria in passion fruit juice: A functional non-dairy product for probiotic delivery, Food Biosci., 2018, 24, 26–36 CrossRef CAS.
- A. F. Massounga Bora, X. Li, Y. Zhu and L. Du, Improved Viability of Microencapsulated Probiotics in a Freeze-Dried Banana Powder During Storage and Under Simulated Gastrointestinal Tract, Probiotics Antimicrob. Proteins, 2019, 11, 1330–1339 CrossRef CAS PubMed.
- M. Singh, D. Sharma, R. Chauhan and G. Goel, Skimmed Milk-Based Encapsulation for Enhanced Stability and Viability of Lactobacillus gastricus BTM 7 Under Simulated Gastrointestinal Conditions, Probiotics Antimicrob. Proteins, 2019, 11, 850–856 CrossRef CAS.
- R. Bradford, V. Reyes, F. Bonilla, F. Bueno, B. Dzandu, C. Liu, A. Chouljenko and S. Sathivel, Development of milk powder containing Lactobacillus plantarum NCIMB 8826 immobilized with prebiotic hi-maize starch and survival under simulated gastric and intestinal conditions, Food Prod., Process. Nutr., 2019, 1, 2–8 CrossRef.
- M. Algaithi, P. Mudgil, M. Hamdi, A. A. Redha, T. Ramachandran, F. Hamed and S. Maqsood, Lactobacillus reuteri-fortified camel milk infant formula: Effects of encapsulation, in vitro digestion, and storage conditions on probiotic cell viability and physicochemical characteristics of infant formula, J. Dairy Sci., 2022, 105, 8621–8637 CrossRef CAS.
- H. S. El-Sayed, H. H. Salama and A. E. Edris, Survival of Lactobacillus helveticus CNRZ32 in spray dried functional yogurt powder during processing and storage, J. Saudi Soc. Agric. Sci., 2020, 19, 461–467 Search PubMed.
- M. I. L. Neves, S. Desobry-Banon, I. T. Perrone, S. Desobry and J. Petit, Encapsulation of curcumin in milk powders by spray-drying: Physicochemistry, rehydration properties, and stability during storage, Powder Technol., 2019, 345, 601–607 CrossRef CAS.
-
CODEX, Codex Standard for Milk Powders and Cream Powder, Rome. Italy, 2011 Search PubMed.
- R. Zhu, M. Hong, C. Zhuang, L. Zhang, C. Wang, J. Liu, Z. Duan, F. Shang, F. Hu, T. Li, C. Ning and G. Chen, Pectin oligosaccharides from hawthorn (Crataegus pinnatifida Bunge. Var. major) inhibit the formation of advanced glycation end products in infant formula milk powder, Food Funct., 2019, 10, 8081–8093 RSC.
- Á. Marcela de Souza Pereira, G. dos Santos Silva, R. Duarte Almeida, H. Oliveira Salles, K. Maria Olbrich dos Santos, E. Rolim Florentino and F. Carolina Alonso Buriti, Instrumental texture and sensory evaluation of fermented dairy beverages processed with reconstituted goat whey powder and a co-culture of Streptococcus thermophilus and Lactobacillus casei, Mljekarstvo, 2018, 68, 21–29 CrossRef.
- E. S. H. Atwaa, M. R. Shahein, E. S. A. El-Sattar, H. H. A. Hijazy, A. Albrakati and E. K. Elmahallawy, Bioactivity, Physicochemical and Sensory Properties of Probiotic Yoghurt Made from Whole Milk Powder Reconstituted in Aqueous Fennel Extract, Fermentation, 2022, 8, 52 CrossRef CAS.
- T. Naksing, P. Hatawee, A. Wanlapa, K. Torpol, C. ChiuHsia, J. Teeka and A. Areesirisuk, Thermal death evaluation of multi-strains probiotic inoculant for shelf-life prediction, Malays Appl. Biol. J., 2019, 48, 147–152 Search PubMed.
- E. M. Padma, D. P. J. Rao, D. L. Edukondalu, D. K. Aparna and D. G. R. Babu, Storage studies of probiotic rice milk during refrigerated conditions, Int. J. Chem. Stud., 2019, 7, 1114–1117 Search PubMed.
- M. Naissinger da Silva, B. L. Tagliapietra, V. do A. Flores and N. S. Pereira dos Santos Richards, In vitro test to evaluate survival in the gastrointestinal tract of commercial probiotics, Curr. Res. Food Sci., 2021, 4, 320–325 CrossRef CAS PubMed.
- K. J. E. P. Kouamé, A. F. M. Bora, X. Li, Y. Sun, S. Tian, M. Hussain, L. Liu and I. Coulibaly, Development and characterization of probiotic (co)encapsulates in biopolymeric matrices and evaluation of survival in a millet yogurt formulation, J. Food Sci., 2023, 88, 9–27 CrossRef PubMed.
- L. Du, W. Lu, B. Gao, J. Wang and L. L. Yu, Authenticating Raw from Reconstituted Milk Using Fourier Transform Infrared Spectroscopy and Chemometrics, J. Food Qual., 2019, 2019, 1–6 CrossRef.
|
This journal is © The Royal Society of Chemistry 2024 |
Click here to see how this site uses Cookies. View our privacy policy here.