Assessing weight loss control strategies in pomegranate (Punica granatum L.) fruit: plastic packaging and surface waxing
Received
14th June 2023
, Accepted 28th September 2023
First published on 30th September 2023
Abstract
Pomegranate fruit is highly prone to moisture loss due to the plentiful micro-pores and slits in the skin, despite having a thick rind. Water loss results in a huge financial loss to the industry through direct loss of marketable fresh weight and the associated diminished commercial value of affected fruit. Plastic packaging and surface coating are broad rivals as water loss control technologies and have attracted increased attention in the fruit industry in the last decade. In this present study, weight loss control techniques including conventional plastic packaging (liner packaging and shrink wrapping) and environmentally sustainable surface coating/waxing technologies were investigated on ‘Wonderful’ pomegranates harvested at commercial maturity with total soluble solids (TSS) of about 16.75 °Brix. Secondly, the different methods of waxing application including dipping, brushing and spraying were investigated. Furthermore, fruits were half dipped in wax by dipping only the top or bottom half of the fruit and this was to assess weight loss variation within individual fruit. Batch 1 fruits were stored at 7 °C and 90% RH for 42 d and thereafter transferred to shelf conditions of 23 °C and 58% RH for 8 d, simulating the maximum sea freight duration from South Africa to Europe across the Atlantic Ocean, followed by open-shelf marketing before consumption. Batch 2 fruits were immediately stored under shelf conditions for 16 d, to simulate prolonged shelf conditions of fruit without a cold storage regime. The fruit weight loss, decay incidence, respiration rate, external total colour difference (TCD), total soluble solids and titratable acidity were investigated and scanning electron microscopy (SEM) was carried out on waxed fruit. The weight loss was highest in control fruit (7.7%), followed by bottom-dip waxed (6.0%), top-dip waxed (5.8%), wax sprayed (4.8%), wax brushed (4.6%), wax dipped (4.0%), and liner packaged (2.1%) and least in shrink wrapped (0.8%) fruit, by the end of the 42 d of cold storage. Dipping was the best wax application method in controlling pomegranate fruit weight loss as compared to half waxing (top and bottom dipped), brushing and spraying application methods. Furthermore, shrink wrapping, liner packaging and wax dipping best maintained a lower TCD and thus better-preserved fruit appearance compared to the rest of the treatments.
Sustainability spotlight
The pomegranate fruit is highly prone to water loss due to the numerous micro-pores and slits in the skin, despite having a thick rind. Water loss is the main contributor to fruit weight loss, and when this exceeds 5%, shriveling, loss of texture, flavour, and significant loss of visual appearance quality may occur. In severe cases, these quality losses make affected fruit unmarketable. This results in postharvest losses and waste, with most of the wastage discarded into landfills. This research investigated weight loss control technologies in postharvest handling of pomegranate fruit, comparing the conventional use of plastic packaging and environmentally sustainable surface waxing applications. This work aligns well with SDG 12 on Sustainable Production and Consumption (specially, 12.3 on reducing losses and waste), SD 2 on Zero Hunger (through improved food security by minimising food losses) and SDG 15 on Life On Land (through providing environmentally sustainable solutions against land degradation).
|
Introduction
The pomegranate fruit (Punica granatum L.) is highly prone to moisture loss due to the plentiful micro-pores and slits in the skin, despite having a thick rind.1,2 A weight loss above 5% causes shrivelling.3,4 Even in the absence of any visible shrivelling, water loss can undesirably affect the visual appearance, flavour and textural properties of the fruit.5 Excessive water loss results in browning of the peel and arils and hardening of the rind6–8 and huge financial loss to the industry through direct loss of marketable fresh weight and the associated diminished commercial value of affected fruit.9 Weight loss in fresh fruit is influenced by several pre-harvest, harvest and postharvest factors including orchard practices, canopy position and cultivar effect, among others.10 Weight loss may also vary with location on the same fruit owing to variations in the surface area to volume ratio, surface waxing coverage, and distribution of surface openings such as lenticel, stomata and micro-cracks.2
Different water loss control techniques have been presented and investigated by many researchers. Storage temperature and relative humidity are important control parameters.11,12 While the use of packaging related technologies such as plastic liners and modified atmosphere packaging,13–18 individual shrink wrapping,13,19 waxing and surface coatings20,21 also influence water loss. Plastic packaging and surface coating are broad rivals as water loss control technologies and have attracted increased attention in the fruit industry in the last decade. These techniques have been applied with great success in minimising the loss of water. However, if not properly used, shrink wrapping and surface coating/waxing can cause anaerobic respiration that leads to producing off flavours,22,23 while plastic liners facilitate moisture condensation within the bags promoting fruit decay.14,24
Previous studies have investigated these weight loss control applications such as using liner packaging, shrink wrapping or surface waxing, often as single factors in comparison with untreated control. However, it is still challenging to perfectly compare results from different studies due to variabilities resulting from geographical differences, cultivar effects and differences in experimental conditions and procedures. Comparative studies with two applications have been considered. For example, Mphahlele et al.13 compared both liner and shrink wrap applications on pomegranate (cv. Wonderful) under cold storage. In this current study, the aim was to evaluate industrial postharvest applications in minimising the weight loss of pomegranate fruit, including conventional plastic packaging and environmentally sustainable surface waxing applications. Plastic packaging techniques such as liner packaging and shrink wrapping were evaluated. Secondly, the different methods of waxing application including dipping, brushing and spraying were investigated. Furthermore, fruits were half dipped in wax by dipping only the top or bottom half of the fruit and this was to assess weight loss variation on an individual fruit.
Materials and methods
Fruit acquisition
Pomegranate fruits (Punica granatum L.) of cultivar ‘Wonderful’ at commercial maturity with total soluble solids (TSS) of about 16.75 °Brix were harvested from an orchard in Porterville, Wellington (33° 38′ S, 19° 00′ E), Western Cape Province, South Africa. The fruits were packed in ventilated paper board cartons and transported to the Postharvest Research Laboratory, Stellenbosch University. Sorting of fruit was carried out to ensure size uniformity and that the fruit were free from surface defects such as cracks. The fruits were inspected, re-sorted, and packed in dozens inside single layer display type paper cartons, cushioned with paper trays at the bottom.
Packaging and waxing
A total of 576 fruits (48 cartons) were randomly divided into eight lots of 72 fruits (6 cartons) each, before applying the following different treatments: (1) the control experiment consisted of fruit with no waxing and no shrink wrapping placed in open-top display type paper cartons without plastic liner bags; (2) passive modified atmosphere packaging (MAP), with fruit enclosed within plastic liner bags (of loading capacity 5 kg, Xtend®, item code 815-PG28/m, patent no. 6190710, StePac L.A. Ltd, Israel), and placed inside open top cartons; (3) shrink film wrapping was carried out using a 19 μm thick co-extruded polyolefin heat shrinkable film (vector shrink film-19 micron polyolefin (POF) centrefold 1067 m, MIPAQ, Durban, South Africa) consisting of five layers of a mixture of low-density polyethylene (LDPE) and polypropylene. Fruits were individually wrapped using a portable I-bar sealer (Model: ME-450SP, Mercier Corporation, Taiwan) and then the film was heat-shrunk on the fruit using a blowing type and portable heat gun (Model: ME-1200-HG, Mercier Corporation, Taiwan). The fruits were then placed in open top cartons in dozens.
Surface waxing of fruit was carried using a ready-to-use lac-resin based wax (Endura-Fresh™ 6100, John Bean Technologies Corporation, Cape Town, South Africa). The fruits were first rinsed with tap water and then allowed to dry under ambient conditions (23 °C) for six hours. Different wax application methods were simulated: (4) the whole fruit was dipped in wax for 5 s, (5) only the top half of the fruit was dipped in wax, (6) only the bottom half of the fruit was dipped in wax, (7) fruits were individually brushed thinly with wax using a general-purpose handheld paintbrush and (8) fruits were sprayed with wax using a general-purpose handheld spray can with a nozzle aperture of 0.55 mm. Care was taken to ensure that fruits were positioned at the same distance from the nozzle and that all sides of the fruit were uniformly sprayed. All waxed fruits were left to air dry under ambient temperature 23 °C for 12 hours and then placed inside open top cartons in dozens.
Fruit storage
Twelve fruits were used to assess the initial quality of fruit before storage and the 72 fruits of each treatment were stored in two batches each of 36 fruits. Batch 1 fruits were stored at 7 °C and 90% RH for 42 d and thereafter transferred to shelf conditions of 23 °C and 58% RH for eight days. This was to mimic the maximum sea freight duration of pomegranate fruit from South Africa to Europe across the Atlantic Ocean, followed by open shelf marketing before consumption. Twelve fruits were selected for quality assessment after 42 d of cold storage and again after an additional eight days of shelf storage. However, fruits packed in plastic (liner and shrink wrap) were removed from their packages when moved to shelf conditions to avoid sudden build-up of moisture condensate within the packages as noticed in preliminary studies (condensate on the roof inside the liners and in the air pockets around the crown inside shrink wraps).24
Batch 2 fruits were immediately stored under shelf conditions of 23 °C and 58% RH. Then twelve fruits were sampled for quality assessment at the eight and sixteen days of shelf storage. This procedure mimics harvested fruit directly placed under shelf storage without cold storage regime.
Measurements and evaluation
Fruit weight, external colour change and decay incidence.
Twelve fruits were randomly selected from each batch and labelled for weight and external peel colour monitoring. Fruit weight was determined using an electronic scientific scale (Mettler Toledo, Model ML3002E, Switzerland, 0.0001 g accuracy). Fruit peel colour was monitored using a digital colorimeter (Minolta, Model CR-400, Tokyo, Japan) at the same storage time interval as fruit weight and size. To establish the change in colour, follow up measurements were carried out at the same marked positions on two opposite sides of each fruit. The lightness (L*), redness (a*) and yellowness (b*) colour properties were measured according to Commission Internationale de l'Eclairage (CIE), 1976. Measurements for fruit weight and peel colour were taken before storage and at intervals of seven days throughout the 42 d under cold storage and afterwards at intervals of two days of under the additional shelf period of eight days of batch 1. For batch 2, measurements were taken at two and four day intervals for fruit weight and peel colour, respectively. Fruit decay incidence was visually assessed.13,25 Fruits with visible external decay and/or mould growth were counted at each sampling time by the researcher and results were presented as a percentage. Decayed and/or moulded fruits were discarded after each sampling.
Headspace gas composition.
The headspace gas composition (O2 and CO2) was determined using a closed system.8 Two fruits were enclosed in an equilibrated hermetically sealed glass jar, in triplicate, for each set of storage conditions. Measurements were taken before and after two hours using a calibrated handheld gas headspace analyser (CheckPoint, PBI-Dansensor A/S, Denmark) at 23 °C.
Chemical attributes.
Fresh juice was extracted from the arils using a blender (Mellerware, South Africa). Total soluble solids (TSS) of the fruit juice were measured using a digital refractometer (Atago, Tokyo, Japan). The titratable acidity (TA) of pomegranate juice (PJ) was determined potentiometrically by titration with 0.1 N NaOH to an endpoint of pH 8.2 using a Compact Auto Titrosampler (Metrohm 862, Herisau, Switzerland). Titratable acidity was expressed in milligrams of citric acid (CA) per a hundred millilitres of juice.
Scanning electron microscopy (SEM).
Samples of the control, dipped, brushed, and sprayed fruit were examined under a SEM to evaluate the waxing efficiency. This qualitative analysis was carried out in triplicate before fruit storage. A cuboid-shaped sample of dimensions 7 × 7 × 3 mm each was excised from the mid (equatorial region) location on each fruit using a sharp blade. The cuboids were fixed by submerging them in a 2.5% glutaraldehyde solution buffered with 0.1 M phosphate buffer and stored at 4 °C overnight. Samples were washed three times for ten minutes in 0.1 M phosphate buffer (at a pH of 7.2). Post-fixation was carried out with 1% osmium tetroxide in 0.1 M phosphate buffer at room temperature in light-proof vails for two hours. Osmium tetroxide has good fixative and excellent stain abilities for lipids in membranous structures. Samples were then washed three times for ten minutes with distilled water. The samples were slowly dried using filter paper in an oven at 37 °C for 48 hours, instead of the conventional dehydration using ethanol and hexamethyldisilazane (HMDS) to minimise the destabilisation and loss of the natural surface wax. The dehydrated samples were mounted on metal specimen stubs, and sputter-coated with gold for 3.5 min using a sputter coater to improve the electron emission of the samples.
Calculations
Weight loss.
Cumulative water loss was calculated with respect to the unit fruit mass (eqn (1)). | 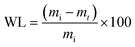 | (1) |
where WL is the water loss per unit fruit mass (%), mi (g) is the initial fruit mass, and mt (g) is the mass of fruit after storage days.
Respiration rate.
The respiration rate (RR) was calculated in terms of the oxygen consumption rate (RO2) and carbon dioxide production rate (RCO2) in mL kg−1 h−1 by fitting experimentally obtained data into eqn (2), respectively.8 The respiration quotient (RQ) was then calculated as the ratio of carbon dioxide production rate to oxygen consumption rate of the fruit (eqn (3)). | 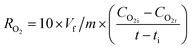 | (2) |
where CO2t and CO2i are the concentrations (%) of O2 at a time t (h) and initial time ti (h), respectively. Likewise, CCO2t and CCO2i are the concentrations (%) of CO2 at a time t (h) and initial time ti (h), respectively. In this study (t − ti) is a constant and equals to 2 h. Vf is the free volume (mL) in the jar which is the total volume minus the volume occupied by the fruit and m (g) is the mass of fruit inside the jar, and the constant 10 is a unit conversion factor (g kg −1).
Total colour difference.
The change in external peel colour was calculated as the total colour difference (TCD) using eqn (4). |  | (4) |
where
and
are the reference values and L*, a* and b* are the respective values of lightness, redness and yellowness colour parameters at a given time.9 Fruit decay incidence was calculated using eqn (5). | Decay incidence = 100 × nd/N | (5) |
where N is the total number of fruits in a carton (N = 12) and nd is the number of fruits with visible external decay and/or mould growth.
Statistical analysis
Measured and calculated data on fruit physical and physio-chemical attributes were analysed using Statistica software (Statistica 14.0, Statsoft, USA). The data were also subjected to analysis of variance (ANOVA) to assess the main effects of weight loss control treatments and the storage duration. Duncan's Multiple Range Test was carried to test for statistical significance at p < 0.05.
Results and discussion
Weight loss
Fig. 1a–c show the weight loss profiles of pomegranate fruit under different weight loss control treatments (packaging and waxing) and storage conditions. The main experimental factors of treatment, storage conditions (cold and shelf storage) and storage duration as well as their interaction significantly (P < 0.05) influenced fruit weight loss. Generally, other treatments greatly minimised fruit weight loss relative to the no-wax-no-package control across all tested conditions. The weight loss was highest in control fruit (7.7%), followed by bottom-dip waxed (6.0%), top-dip waxed (5.8%), wax sprayed (4.8%), wax brushed (4.6%), wax dipped (4.0%), and liner packaged (2.1%) and least in shrink wrapped (0.8%) fruit, by the end of the 42 d of cold storage. A similar trend is observed during the subsequent additional eight days of shelf storage as well as during the independent 16 d of prolonged shelf storage. A weight loss of 19.8, 15.2, 14.8, 14.0, 14.0, 11.2, and 0.9% was observed in the control, bottom-dipped, top-dipped, wax sprayed, wax brushed, wax dipped and shrink wrapped fruit, respectively, at the end of the 16 d of prolonged shelf storage.
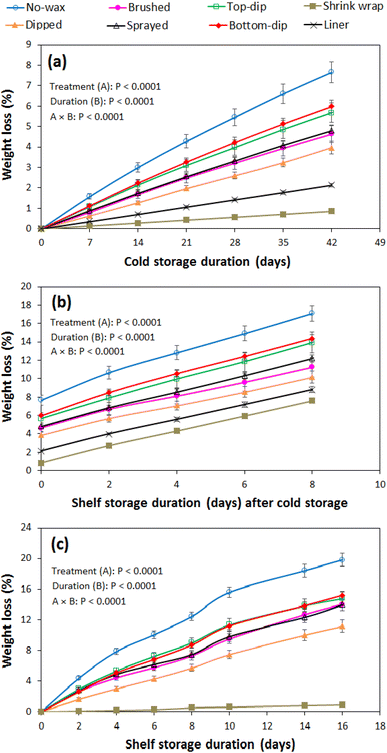 |
| Fig. 1 Weight loss profile of pomegranate fruit (cv. Wonderful) under different weight loss control treatments during storage: (a) at 7 °C/90% RH for 42 d, (b) followed by an additional 8 d of shelf storage at 23 °C/58% RH and (c) under immediate prolonged shelf storage of 16 d. The data points are means (n = 12) and the vertical lines represent the standard error of the mean. Numerical values of A and B are p-values. | |
A significantly higher weight loss was observed in all waxed fruits as compared to the packaged fruit. This is attributed to higher water vapour permeability and diffusivity in the wax material than in the plastic liners and shrink wrap. Similar results have been reported previously.21 In waxed fruit, weight loss was lower in dipped fruit than in half waxed (top and bottom dipped), brushed and sprayed fruit. This is attributed to differences in wax thickness resulting from the different application methods. Spraying and brushing methods result in thinner wax deposits and coverage compared to fruit dipping. This is discussed further in the SEM section below. The slightly higher weight loss in the bottom waxed as compared to the top waxed fruit can be attributed to more surface micro-openings (lenticels) on the top side of the fruit than on the bottom side of the fruit. This is further supported by findings from Lufu et al.2 In addition, this could be facilitated by a higher porosity (void space) and permeability in the inner peel tissues at the top than the bottom location of the fruit.
Individual shrink wrapping was the best treatment in minimising weight loss in pomegranate fruit compared to plastic liner packaging using (MAP) and surface waxing under cold and storage conditions. Quite similar to the current findings, a previous study reported a weight loss of less than 2% in shrink wrap and MAP treatments as compared to 16.3% in control treatment.13 This is attributed to the combined effect of the high resistance (barrier) properties against moisture diffusion in the shrink wrap plastic film and its ability to block the surface opening of the fruit.
Total colour difference (TCD) of the peel
External peel colour is a very important quality attribute of fresh fruit during marketing. It influences visual appeal and acceptance of pomegranate during marketing.4,26 The overall change in peel colour was expressed as the TCD (Fig. 2). Generally, the TCD was significantly (P < 0.05) influenced by the different treatments, storage duration and their interactions. The TCD progressively increased with the storage duration during the 42 d of cold storage and the subsequent 8 d of shelf storage. Fig. 2a shows the TCD results during the additional shelf storage period, where all packaging and waxing treatments significantly minimised the overall change in colour compared to the control treatment (with no wax and packaging). At zero days of the additional shelf storage, a distinctively lower TCD is observed in the shrink wrapped and liner packaged fruit as compared to the waxed fruit. This is attributed to the generally lower moisture loss rates in the former than in the latter, during the previous cold storage regime. The rapid increase in the TCD for the shrink wrapped and liner packaged fruit during the additional shelf storage is because of an accelerated rate of moisture loss resulting from un-wrapping and un-packaging of fruit from the plastics to prevent induced moisture condensation inside plastic liners and accelerated fruit decay.
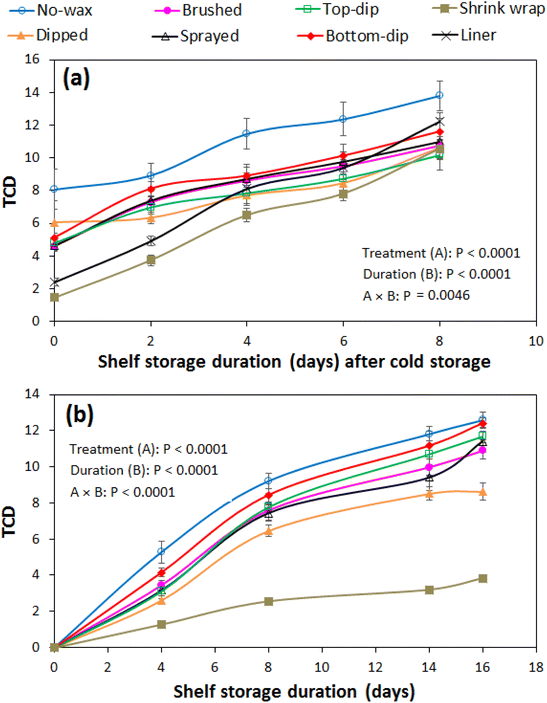 |
| Fig. 2 Total colour difference (TCD) profiles of pomegranate (cv. Wonderful) under different weight loss control treatments during storage: (a) the additional 8 d of shelf storage at 23 °C/58% RH after the 42 d of cold storage at 7 °C/90% RH and (b) under immediate prolonged shelf storage of 16 d. The data points are means (n = 12) and the vertical lines represent the standard error of the mean. Treatments include fruit with no-package and no-wax control, fruit waxed in carnauba wax by dipping, brushing, spraying, dipping only the top half of the fruit, dipping only the bottom half of the fruit, and fruit packaged by individual shrink wrapping and in plastic liners. Numerical values of A and B are p-values. | |
A similar pattern of TCD variation with treatment–duration interaction was observed for fruit under prolonged shelf storage (Fig. 2b). In this case, the TCD increased steadily at a higher rate for the first 8 d, followed by a progressive increase at a lower rate to the end of the 16 d. Fig. 3 shows that generally the TCD was linearly related to fruit weight loss, as demonstrated by the very high positive correlations of R2 = 0.965–0.991 during cold storage plus additional shelf storage, and R2 = 0.936–0.989 during prolonged shelf storage (Table 1). This suggests that the TCD is a very good predictor parameter of water loss in pomegranate fruit during storage and marketing conditions. The loss of water during storage facilitates the degradation of colour pigments due to water stress.27
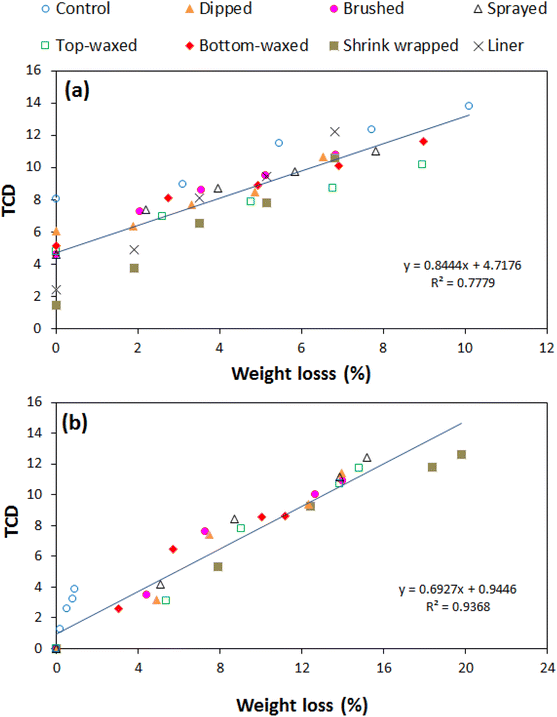 |
| Fig. 3 Correlations between the total colour difference (TCD) and weight loss of pomegranate fruit (cv. Wonderful) under different weight loss control treatments during storage: (a) the additional 8 d of shelf storage at 23 °C/58% RH after the 42 d of cold storage at 7 °C/90% RH and (b) under immediate prolonged shelf storage of 16 d. A general trend line is plotted through all data points for each respective storage period. | |
Table 1 This correlation coefficient (R2) values of the linear relationship between the peel total colour difference (TCD) and fruit weight loss
Treatment |
42 d [7 °C] + 8 d [23 °C] |
16 d [23 °C] |
No-wax |
0.9662 |
0.9886 |
Dipped |
0.922 |
0.9506 |
Brushed |
0.9684 |
0.9359 |
Sprayed |
0.9648 |
0.9665 |
Top-dip |
0.9817 |
0.9844 |
Bottom-dip |
0.9707 |
0.9859 |
Shrink wrap |
0.9913 |
0.9737 |
Liner |
0.9885 |
|
Overall |
0.7779 |
0.9368 |
Respiration rate (RR)
The results of the respiration rate of pomegranate fruit during the 42 d of cold storage and the subsequent shelf storage of eight days are summarised in Fig. 4a and b. Both the rates of oxygen consumption (RO2) and carbon dioxide production (RCO2) followed a similar trend across all tested treatments. In the study, the experimental factors of the treatment type and storage duration significantly (P < 0.05) influenced RO2 and RCO2, unlike their interactive effect. Before fruit storage, RO2 and RCO2 were 21.8 and 21.0 mL kg−1 h−1, respectively. A slight increase is observed in most treatments at the end of cold storage, and thereafter it continued to decrease during the subsequent shelf storage. At 42 d of cold storage, all waxing and packaging treatments exhibited a relatively lower RCO2 than the control treatment with significantly lower results observed in the shrink wrapped and liner packaged fruit (Fig. 4b). At 4 d and 8 d of subsequent shelf storage, all treatments exhibited relatively lower RCO2 than the control treatment but with no significant difference.
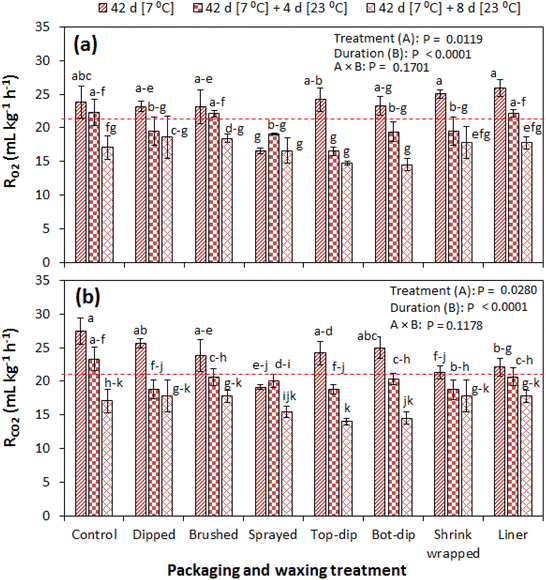 |
| Fig. 4 The rate of O2 (RO2) production (a) and CO2 (RCO2) production (b) of pomegranate fruit (cv. Wonderful) under different weight loss control treatments during storage at 7 °C/90% RH for 42 d followed by an additional 8 d of shelf storage at 23 °C/58% RH. The bars are means (n = 3) and the vertical lines represent the standard error of the mean. The dotted horizontal line represents values before storage. Numerical values of A and B are p-values. | |
Fig. 5a and b show the RR of the fruit during prolonged shelf storage, where RO2 and RCO2 decreased with the storage duration. At 8 d of shelf storage, all treatments significantly had a lower RCO2 than the control, except for the fruit sprayed with wax. In this case, RCO2 was lowest in the shrink wrapped fruit with a value of 12.40 mL kg−1 h−1 compared to 16.34 mL kg−1 h−1 in the control fruit. Thereafter, the RR did not significantly change to the end of the 16 d in all waxed and packaged fruit except for the control fruit. The tremendous decrease in RCO2 observed in the control fruit at the end of the 16 d could be attributed to a change in fruit physiology due to hardening of the rind resulting from excessive water loss. Other studies have reported a decrease in the RR of pomegranate fruit with the storage duration.20,28,29 Rao29 observed a decreasing RR of shrink wrapped and non-wrapped pomegranate fruit (cvs. Mridula and Bhagwa) during the 28 d of shelf storage at 25–32 °C and 49–67% RH. A similar situation was observed in a climacteric fruit (pear) stored at different temperature and relative humidity combinations.30 In contrast, an increase of the RR with the storage duration has been reported in uncoated and coated pomegranate fruit.31 The increase in other studies is attributed to tissue senescence.32
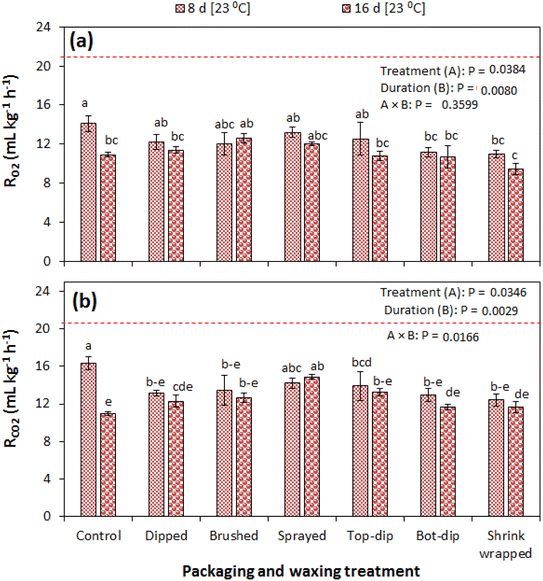 |
| Fig. 5 The rate of O2 (RO2) production (a) and CO2 (RCO2) production (b) of pomegranate fruit (cv. Wonderful) under different weight loss control treatments during prolonged shelf storage of 16 d at 23 °C/58% RH. The bars are means (n = 3) and the vertical lines represent the standard error of the mean. The dotted horizontal line represents values before storage. Numerical values of A and B are p-values. | |
Respiratory quotient (RQ)
The RQ is an important metabolic index in assessing the characteristic respiratory kinetics of fresh produce. The RQ is the ratio of the CO2 production rate of the product to the rate of O2 consumed.33Fig. 6a and b show the RQ of pomegranate fruit under all tested conditions. The treatments, storage duration and their interactive effect significantly influenced the RQ during the cold storage period and the subsequent shelf regime (Fig. 6a). A RQ of 0.96 was observed in the control fruit before storage, which significantly increased to 1.16 at 42 d of cold storage, followed by a decrease to 1.00 by the end of the 8 d subsequent shelf storage. A similar trend was observed in the bottom waxed fruit (bot-dip) and sprayed fruit. On the other hand, the RQ decreased from 0.96 to 0.85 in the shrink wrapped and liner packaged fruit at the end of cold storage followed by an increase to RQ = 1. However, treatments, the storage duration and their interactions did not have a significant influence on the RQ during the prolonged shelf storage period (Fig. 6b).
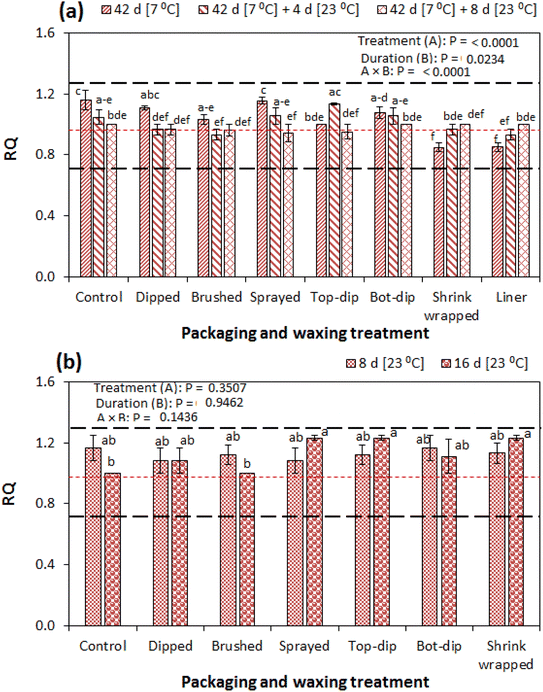 |
| Fig. 6 Respiratory quotient (RQ) of pomegranate fruit (cv. Wonderful) under different weight loss control treatments during storage: (a) at 7 °C/90% RH for 42 d followed by an additional 8 d of shelf storage at 23 °C/58% RH and (b) under an immediate prolonged shelf storage of 16 d. The bars are means (n = 3) and the vertical lines represent the standard error of the mean. The dotted horizontal red line represents TA before storage, while the black dotted lines are the lower (0.7) and upper (1.3) characteristic threshold values.40 Numerical values of A and B are p-values. | |
Generally, for all tested conditions, the RQ ranged from 0.85 to 1.16 during the cold storage plus shelf storage regime and 1.00 to 1.23 during prolonged shelf storage. The characteristic values of RQ are often in the range of 0.7 to 1.3 with respect to the respiratory substrate.34 The RQ value above 1.3 is indicative of anaerobic respiration in the product.35 Shrink-wrapping and waxing/coating is often associated with the development of noticeable off flavours in fruit under storage.22,23 It is important to note that this is dependent of the degree of coverage and thickness of the wax, which ultimately affects the skin permeance to water vapour and gases.36 In the current study, the shrink wraps were further removed upon transferring fruit to shelf conditions, and this could have minimised the building up of off flavour. Therefore, the RQ results of the current study are well within the expected range and further indicate that the fruit could be free from anaerobic respiration and its associated off flavour development that is often attributed to shrink wrapping and surface coating/waxing.22,23
TSS and TA
Chemical attributes of TSS and TA are important in describing the sweetness and sourness of fruit juice taste, respectively.37,38 The changes in the TSS of the fruit juice with storage time is presented in Fig. 7a and b. The different treatments did not have a significant influence on the TSS with respect to the control treatment during the 42 d of cold storage and subsequent shelf storage (Fig. 7a). Similar results of no significant difference were observed in pomegranate fruit (cv. Primosole) treated and untreated with lecithin during storage at 8 °C and 90–95% RH for 84 d and an additional 7 d of shelf storage at 20 °C and 60–65% RH.39
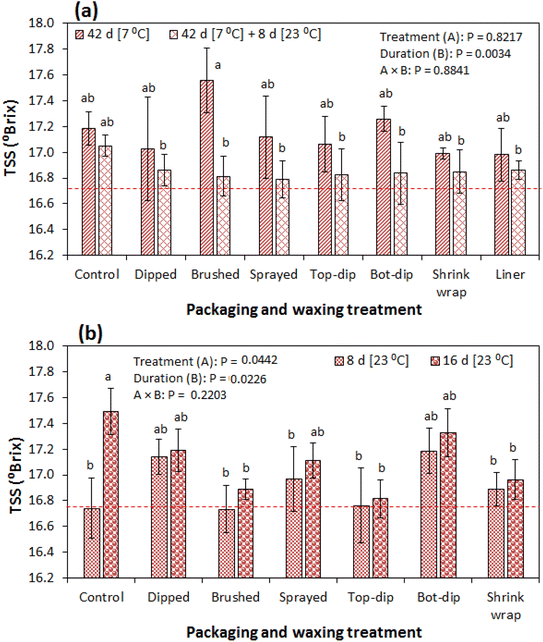 |
| Fig. 7 Total soluble solids (TSS) of pomegranate fruit juice (cv. Wonderful) for fruit under different weight loss control treatments during storage: (a) at 7 °C/90% RH for 42 d followed by an additional 8 d of shelf storage at 23 °C/58% RH and (b) under immediate prolonged shelf storage of 16 d. The bars are means (n = 12) and the vertical lines represent the standard error of the mean. The dotted horizontal line represents TSS before storage. Numerical values of A and B are p-values. | |
However, TSS varied significantly with storage conditions increasing from 16.75 °Brix before storage to 16.98 °Brix in the shrink wrapped and liner packaged fruit, 17.03–17.06 °Brix in the dipped and top-dip waxed fruit and 17.12–17.19 °Brix in the control and the rest of the wax treated fruit. This was followed by an insignificant decrease in TSS across all treatments when the fruit was transferred to shelf conditions for 8 d. The increase in TSS is attributed to the hydrolysis of starch and polysaccharides into respiration substrate sugars40,41 rather than the concentration of total solids due to moisture loss as observed in a previous study on pomegranate (cv. Hicrannar).42 This is because water loss in pomegranate fruit occurs mainly and primarily from the peel fraction rather than the arils.19
On the other hand, a significantly lower TSS was observed in the shrink wrap, top-dip and brushed fruit as compared to the control fruit by the end of the 16 d of prolonged shelf storage (Fig. 7b). A previous study on ‘Wonderful’ reported that coated and liner packaged fruit significantly minimised the increase in TSS compared to control fruit.21 Quite similar to our results, the packaging of fruit in liners was observed to minimise the increase in the TSS of pears (cv. Punjab Beauty) compared to no-packaging treatment in the first 60 d of cold storage.43
Titratable acidity was not significantly influenced by the treatments during cold storage and the subsequent shelf storage regime (Fig. 8a). Quite similarly, Mphahlele et al.13 observed no significant TA results among treatments (control, plastic liner packaging and shrink wrapping) at 30 days of storage. However, in the current study, TA significantly (P < 0.005) decreased with the duration from 1.62 mg.100 mL−1 before storage to between 0.78 and 0.92 mg.100 mL−1 at the end of the additional shelf storage. Comparably, a decrease in TA has been reported in different cultivars of pomegranate including ‘Wonderful’, ‘Hicrannar’ and ‘Hicaznar’12,42,44 under different storage conditions, attributed to the utilisation of organic acids in the metabolic process. Similarly, a decrease in TA is reported for pomegranate fruit (cv. Primosole) treated and untreated with lecithin during storage at 8 °C and 90–95% RH for 84 d and an additional 7 d of shelf storage at 20 °C and 60–65% RH.39 Furthermore, comparable to our results, Mphahlele et al.13 reported a decreasing TA with no significant difference between the control and the shrink wrapped pomegranate (cv. Wonderful) fruit at the end of 90 d of storage at 7 °C and 92% RH. However, the authors observed a significant difference in TA between the control and the liner packed fruit after the 90 d of storage. Likewise, D'Aquino et al.45 observed a significantly lower TA in the shrink wrapped fruit (cv. Primosole) than in the control fruit at the end of the 42 d at 8 °C and 85–90% RH and an additional 7 d of shelf storage at 20 °C and 65–70% RH. These differences between the findings of the current study and previous studies could be attributed to differences in cultivars and material properties of the packaging investigated. In our study, TA was significantly lower in the dipped (17.14 mg.100 mL−1) and shrink wrapped (16.89 mg.100 mL−1) fruit than in the rest on the treatments (1.42–1.74 mg.100 mL−1) at 8 d of shelf storage (Fig. 8b).
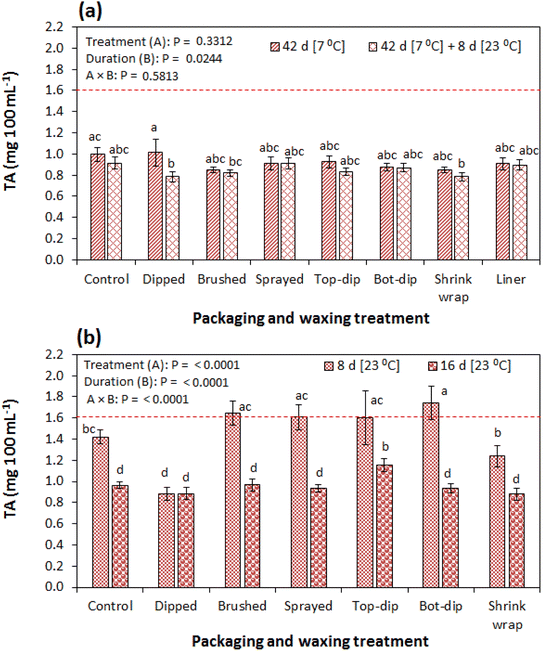 |
| Fig. 8 Titratable acidity (TA) of pomegranate fruit juice (cv. Wonderful) for fruit under different weight loss control treatments during storage: (a) at 7 °C/90% RH for 42 d followed by an additional 8 d of shelf storage at 23 °C/58% RH and (b) under immediate prolonged shelf storage of 16 d. The bars are means (n = 12) and the vertical lines represent the standard error of the mean. The dotted horizontal line represents TA before storage. Numerical values of A and B are p-values. | |
Fruit decay incidence
The results on fruit decay are presented in Fig. 9a and b. Decay incidence was evaluated by visual count of all fruits with signs of decay and/or mould growth. There was no visible fruit decay on fruit from all treatments except for shrink wrapped fruit. Signs of mould growth were first identified in the shrink-wrapped fruit after 42 d of storage at 7 °C and 90% RH with a decay incidence of 5.6%, followed by an increase to 11.1% by the end of the subsequent 8 d of shelf storage at 13 °C and 58% RH (Fig. 9a). Likewise, there was no observed fruit decay in fruit from other treatments except in the shrink-wrapped fruit (13.89%) at the end of the 16 d of prolonged shelf storage (Fig. 9b). This could be attributed to the high moisture barrier properties of the shrink-wrap films creating favourable conditions for spore germination especially in micro-cracks and micro-pores on the fruit peel surface. Mould mycelial growth has been observed in micro-cracks and lenticels on the surface of pomegranate (cv. Wonderful) fruit.2,46 Comparable to our findings, Mphahlele et al.13 observed a decay incidence of 0.00 and 4.17% in the liner packed and shrink wrapped pomegranate (cv. Wonderful) fruit, respectively, after 30 d of cold storage at 7 °C and 92% RH. However, the authors also observed a 4.17% decay incidence in the control (without liner and shrink wrap) fruit. Furthermore, the authors observed an increase in decay incidence to 29.17 and 33.93, 29.17 and 16.68% in the liner packed, shrink wrapped and control fruit at the end of the 90 d of storage. Likewise, Laribi et al.47 observed higher decay in liner packed pomegranate fruit (cv. Mollar de Elche) as compared to the control fruit with no liner.
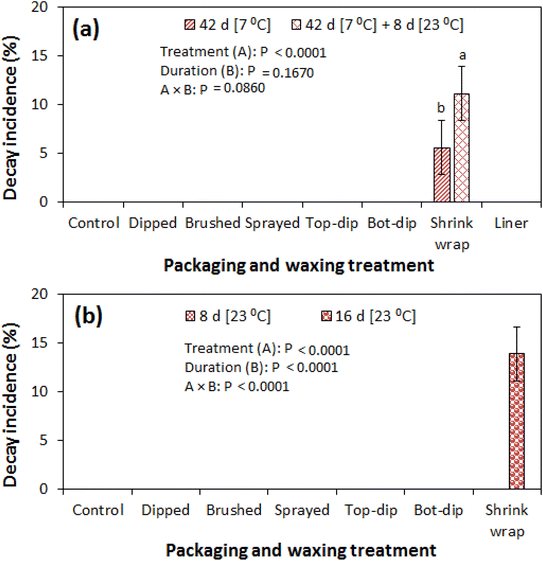 |
| Fig. 9 Decay incidence of pomegranate fruit (cv. Wonderful) under different weight loss control treatments during storage: (a) at 7 °C/90% RH for 42 d followed by an additional 8 d of shelf storage at 23 °C/58% RH and (b) under immediate prolonged shelf storage of 16 d. Numerical values of A and B are p-values. | |
SEM
Scanning electron microscopy revealed differences in the waxing thickness and waxing patterns on the surface of the fruit (Fig. 10a–d). The control sample showed the presence of widely open lenticels and micro-cracks (Fig. 10a). On the other hand, waxing by dipping, brushing and spraying covers the lenticels and micro-cracks on the surface of the fruit. The degree of wax coverage varied among the waxing methods with higher coverage (fewer gaps) observed in the dipped than in the brushed and sprayed fruit. This is attributed to the thicker wax layer in the dipped fruit (3.86 μm) as compared to the brushed fruit (2.89 μm) and sprayed fruit (2.17 μm) that can easily be cracked. Quite similar results were observed in pears coated with carnauba-based wax to varying concentrations, resulting in different wax-skin coverage and thus affecting the water and gas permeance.36 These results explain why there was a relatively lower moisture loss in the dipped fruit than in the brushed fruit and sprayed fruit during the cold storage and shelf storage regimes.
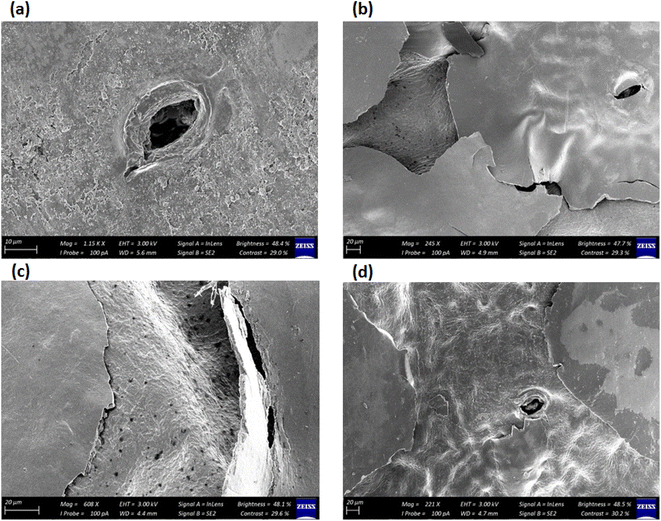 |
| Fig. 10 Scanning electron microscopy (SEM) images of pomegranate peel (cv. Wonderful) taken from samples of fruit: (a) with no wax, (b) dipped, (c) brushed and (d) sprayed with lac-resin based wax after they were stored for 42 d at 7 °C/90% RH followed by an additional 8 d of shelf storage at 23 °C/58% RH. | |
Conclusions
The aim of the study was to evaluate weight loss control capabilities of conventional plastic packaging and environmentally sustainable surface waxing applications on pomegranate fruit. Secondly, the different methods of waxing application were investigated. Weight loss was best minimised in shrink wrapped (0.8%), followed by liner packaged (2.1%), wax dipped (4.0%), wax brushed (4.6%), wax sprayed (4.8%), top-dip waxed (5.8%), and bottom-dip waxed (6.0%) fruit, compared to untreated control fruit (7.7%) by the end of the 42 d of cold storage. A similar trend was observed at the end of 16 d of prolonged shelf storage, with a weight loss of 19.8, 15.2, 14.8, 14.0, 14.0, 11.2, and 0.9% in the control, bottom-dipped, top-dipped, wax sprayed, wax brushed, wax dipped and shrink wrapped fruit, respectively.
Dipping was the best wax application method in controlling pomegranate fruit weight loss as compared to half waxing (top and bottom dipped), brushing and spraying application methods. This is attributed to differences in wax thickness resulting from the different application methods, with spraying and brushing methods resulting in thinner wax deposits and coverage compared to fruit dipping, as demonstrated through scanning electron microscopic examination.
Packaging and waxing treatments significantly minimised the external total colour difference (TCD) compared to the control, maintaining a similar trend to weight loss results, with the TCD being lowest in shrink wrapped and wax dipped fruit during the prolonged shelf storage regime. In addition, the study established a very strong positive linear correlation (R2 = 0.936–0.991) between the TCD and fruit weight loss, suggesting that the TCD is a very good predictor parameter of water loss in pomegranate fruit under storage and marketing conditions. Furthermore, the respiration quotient (RQ) results of the current study are well within the expected range and further indicate that the fruit could be free from anaerobic respiration and its associated off flavour development that is often attributed to shrink wrapping and surface coating/waxing.
Due to visually observed decay incidence in the shrink-wrapped fruit, in this study liner packaging and surface waxing by dipping are considered the best options in minimising fruit water loss and prevention of postharvest quality loss. However, the current movement towards a plastic-free packaging (remove/reduce plastics) is a growing challenge in the fruit and vegetable industry. Therefore, using edible surface waxing should be considered as a more environmentally sustainable application for minimising water loss in pomegranate fruit. The findings of this study are vital to the pomegranate industry in guiding future research on strategic water loss control using environmentally sustainable technologies like surface waxing. Furthermore, the study highlights the significance of the surface waxing application method which is a major research gap in the pomegranate fruit industry in terms of waxing and coating applications. Future research should consider determining the gas permeability of the wax and shrink wrap material and analysis of phenolics content, antioxidant capacity of fruit juice and fermentation volatiles resulting from anaerobic respiration.
Author contributions
Conceptualization, U. L. O. & A. A.; methodology, R. L. & A. A.; validation, A. A.; formal analysis, R. L.; investigation, R. L.; writing—original draft preparation, R. L.; writing—review and editing, A. A. & U. L. O.; visualization, R. L.; supervision, U. L. O. & A. A.; project administration, U. L. O. & A. A.; funding acquisition, U. L. O. All authors have read and agreed to the published version of the manuscript.
Conflicts of interest
The authors declare no competing interests.
Acknowledgements
This work is based on the research supported wholly/in part by the National Research Foundation of South Africa (grant numbers: 64813). The opinions, findings and conclusions or recommendations expressed are those of the author(s) alone, and the NRF accepts no liability whatsoever in this regard. Research reported in this publication was supported in part by the Foundation for Food and Agriculture Research under award number – grant ID: DFs-18-0000000008.
Notes and references
- U. L. Opara and M. R. Al-Ani, Br. Food J., 2010, 112, 797–810 CrossRef.
- R. Lufu, A. Ambaw and U. L. Opara, Postharvest Biol. Technol., 2021, 178, 111539 CrossRef CAS.
-
R. Lufu, Unpacking the Influence of Internal Packaging on Cooling Characteristics and Postharvest Quality of Pomegranate Fruit, Master thesis, University of Stellenbosch, South Africa, 2017.
- S. M. Elyatem and A. A. Kader, Sci. Hortic., 1984, 24, 287–298 CrossRef.
- S. Pareek, D. Valero and M. Serrano, J. Sci. Food Agric., 2015, 95, 2360–2379 CrossRef CAS.
- A. A. Kader, A. Chordas and S. Elyatem, Calif. Agric., 1984, 14–15 Search PubMed.
- F. Artés, R. Villaescusa and A. J. Tudela, J. Food Sci., 2000, 65, 1112–1116 CrossRef.
- O. J. Caleb, P. V. Mahajan, U. L. Opara and C. R. Witthuhn, Postharvest Biol. Technol., 2012, 64, 49–54 CrossRef.
- P. B. Pathare, U. L. Opara and F. A.-J. Al-Said, Food Bioprocess Technol., 2013, 6, 36–60 CrossRef CAS.
- R. Lufu, A. Ambaw and U. L. Opara, Sci. Hortic., 2020, 272, 109519 CrossRef.
- R. Lufu, A. Ambaw and U. L. Opara, Postharvest Biol. Technol., 2019, 157, 110982 CrossRef.
- E. Arendse, O. A. Fawole and U. L. Opara, CyTA--J. Food, 2014, 12, 389–398 CrossRef CAS.
- R. R. Mphahlele, O. A. Fawole and U. L. Opara, Sci. Hortic., 2016, 211, 140–151 CrossRef CAS.
- R. Lufu, T. M. Berry, A. Ambaw and U. L. Opara, Acta Hortic., 2018, 1201, 259–263 CrossRef.
- O. J. Caleb, U. L. Opara, P. V. Mahajan, M. Manley, L. Mokwena and A. G. J. Tredoux, Postharvest Biol. Technol., 2013, 79, 54–61 CrossRef CAS.
- Z. A. Belay, O. J. Caleb, P. V. Mahajan and U. L. Opara, Food Bioprocess Technol., 2018, 11, 1478–1494 CrossRef CAS.
- K. Banda, O. J. Caleb, K. Jacobs and U. L. Opara, Postharvest Biol. Technol., 2015, 109, 97–105 CrossRef.
- R. Lufu, A. Ambaw, T. M. Berry and U. L. Opara, Food Packag. Shelf Life, 2020, 26, 100585 CrossRef.
- R. Lufu, A. Ambaw and U. L. Opara, Sustainable Food Technology, 2023, 79–91 RSC.
- S. Nanda, D. V. S. Rao and S. Krishnamurthy, Postharvest Biol. Technol., 2001, 22, 61–69 CrossRef.
- T. G. Kawhena, U. L. Opara and O. A. Fawole, Processes, 2022, 10(1), 164 CrossRef CAS.
-
S. Ben-Yehoshua and V. Rodov, in Postharvest Physiology and Pathology of Vegetables, ed. J. A. Bartz and J. K. Brecht, CRC Press, Boca Raton, 2nd edn, 2002, pp. 111–159 Search PubMed.
- E. Motamedi, J. Nasiri, T. R. Malidarreh, S. Kalantari, M. R. Naghavi and M. Safari, Sci. Hortic., 2018, 240, 170–178 CrossRef CAS.
- R. Lufu, A. Ambaw and U. L. Opara, Foods, 2021, 10, 1388 CrossRef CAS PubMed.
- J. Atukuri, O. A. Fawole and U. L. Opara, J. Food Meas. Charact., 2017, 11, 1081–1093 CrossRef.
- M. I. Gil, R. Sánchez, J. G. Marín and F. Artés, Z. Lebensm.-Unters. Forsch., 1996, 202, 481–485 CrossRef CAS.
- B. M. Muche, R. A. Speers and H. P. V. Rupasinghe, Front. Nutr., 2018, 5, 1–9 CrossRef PubMed.
- F. Artés, J. G. Marín and J. A. Martínez, Z. Lebensm.-Unters. Forsch., 1996, 203, 33–37 CrossRef.
- D. V. S. Rao, J. Food Sci. Technol., 2018, 55, 351–365 CrossRef PubMed.
- G. T. Xanthopoulos, C. G. Templalexis, N. P. Aleiferis and D. I. Lentzou, Biosyst. Eng., 2017, 158, 76–85 CrossRef.
- H. Meighani, M. Ghasemnezhad and D. Bakhshi, J. Food Sci. Technol., 2014, 52, 4507–4514 CrossRef PubMed.
- L. Sanchez-Gonzalez, C. Pastor, M. Vargas, A. Chiralt, C. Gonzalez-Martinez and M. Chafer, Postharvest Biol. Technol., 2011, 60, 57–63 CrossRef CAS.
- T. Iqbal, F. A. S. Rodrigues, P. V. Mahajan and J. P. Kerry, J. Food Eng., 2009, 91, 325–332 CrossRef CAS.
- S. C. Fonseca, F. A. R. Oliveira, J. M. Frias, J. K. Brecht and K. V. Chau, J. Food Eng., 2002, 54, 299–307 CrossRef.
- R. M. Beaudry, A. C. Cameron, A. Shirazi and D. L. Dostal-Lange, J. Am. Soc. Hortic. Sci., 1992, 117, 436–441 Search PubMed.
- C. Amarante, N. H. Banks and S. Ganesh, Postharvest Biol. Technol., 2001, 21, 291–301 CrossRef.
- F. A. Al-Said, L. U. Opara and R. A. Al-Yahyai, J. Food Eng., 2009, 90, 129–134 CrossRef.
- K. S. Tandon, E. a. Baldwin, J. W. Scott and R. L. Shewfelt, J. Food Sci., 2003, 68, 2366–2371 CrossRef CAS.
-
S. D'Aquino, M. Schirra, A. Gentile, E. Tribulato, S. La Malfa and A. Palma, in XXVIIIth IHC – IS on Postharvest Technology in the Global Market, Acta Horticulturae, ed. M. I. Cantwell and D. P. F. Almeida, ISHS, 2012, pp. 733–740 Search PubMed.
-
D. Valero and M. Serrano, Postharvest Biology and Technology for Preserving Fruit Quality, CRC Press, Boca Raton, USA, 1st edn, 2010 Search PubMed.
- H. M. Díaz-Mula, P. J. Zapata, F. Guillén, D. Martínez-Romero, S. Castillo, M. Serrano and D. Valero, Postharvest Biol. Technol., 2009, 51, 354–363 CrossRef.
- N. Selcuk and M. Erkan, Postharvest Biol. Technol., 2014, 92, 29–36 CrossRef CAS.
- K. Kaur, W. S. Dhillon and B. V. C. Mahajan, J. Food Sci. Technol., 2013, 50, 147–152 CrossRef CAS.
- N. Selcuk and M. Erkan, Postharvest Biol. Technol., 2015, 109, 30–39 CrossRef CAS.
- S. D'Aquino, A. Palma, M. Schirra, A. Continella, E. Tribulato and S. La Malfa, Postharvest Biol. Technol., 2010, 55, 121–128 CrossRef.
- R. Lufu, A. Ambaw and U. L. Opara, Acta Hortic., 2022, 1349, 261–267 CrossRef.
-
A. I. Laribi, L. Palou, V. Taberner and M. B. Pérez-gago, in Post Harvest, Food and Process Engineering, International Conference of Agricultural Engineering – CIGR-AgEng 2012: Agriculture and Engineering for a Healthier Life, Valencia, Spain, 8–12 July 2012, CIGR-EurAgEng, Valencia, Spain, 1992, p. 6 Search PubMed.
|
This journal is © The Royal Society of Chemistry 2024 |
Click here to see how this site uses Cookies. View our privacy policy here.