DOI:
10.1039/D4DT01188K
(Paper)
Dalton Trans., 2024,
53, 11417-11425
Exploring structural and optical properties of a new series of soft salts based on cyclometalated platinum complexes†
Received
22nd April 2024
, Accepted 17th June 2024
First published on 18th June 2024
Abstract
A series of nine new soft salts based on two platinum(II) complexes, namely ([Pt(C^N)(CN)2]−[Pt(C^N)(en)]+) (en = ethane-1,2-diamine), has been developed and synthesized. Their photophysical properties in both solution and the solid state were described. All soft salt complexes exhibit phosphorescence emission with PLQY in the solid state up to 0.36. Most of these materials displayed aggregation-induced emission (AIE) or aggregation-induced emission enhancement (AIEE) in water/DMSO solutions as the water ratio increased. Structure–property relationships were analyzed in relation to emission properties. The presence of the free nitrogen atoms in soft salt complexes with a C^N pyrimidine-based ligand allowed for reversible sensitivity to acidic vapors, resulting in the quenching of phosphorescence emission. Additionally, for selected soft salts, we described reversible vapochromism behaviour, making these new materials interesting for multi-detection purposes in anti-counterfeiting applications.
Introduction
Over the past few decades, emissive materials with tunable luminescence colors have been of great importance for their optical applications. To meet the growing demand in various optoelectronic applications, there has been increasing interest in stimuli-responsive luminescent materials, as they can exhibit tunable emissive properties under different external stimuli, such as light,1 temperature,2 mechanical force,3 organic solvent vapors,4 or an electric field.5 In this context, small molecules,6 metal complexes,7 and polymers8 have been developed as materials with photochromic,9 mechanochromic,10 vapochromic,11 electrochromic,12 and thermochromic properties.2a,13 These luminescent materials are present in wide applications in displays,14 photocatalysis,15 data recording and storage,16 chemical sensing and cellular imaging,17 and security technologies.18
Due to their high photoluminescence quantum yield (PLQY), remarkable photostability, easy tunability of the emission wavelength, and increased sensitivity to external stimuli, phosphorescent transition metal complexes are potential candidates for constructing stimuli-responsive luminescent materials.19 Among them, phosphorescent soft salt complexes have attracted increasing attention due of their interesting structural and photophysical properties, making them valuable as phosphorescent materials in diverse optoelectronic applications.20 Consequently, studies of the potential of soft salts complexes have emerged in various applications such as organic light-emitting diodes,21 bioimaging,22 photodynamic therapy,23 and anti-counterfeiting areas.24 Soft salt complexes are composed of two or more photoactive organometallic complexes with complementary charges linked by electrostatic attractions and van der Waals forces.25 Desirable photophysical properties, such as emission tuning, improved photoluminescence quantum efficiency, and longer excited state lifetimes, can be achieved by controlling both the molecular engineering of the ligands of the ionic complexes and transition metals (Ru, Ir, Pt, Cu, Zn, and Au) present in these soft salts.20a,b,26
Among this class of ion-paired complexes, soft salts based on cyclometalated platinum(II) complexes have garnered growing interest as potential candidates for the development of stimuli-responsive luminescent materials.27 Recently, three accounts of closely related homometallic luminescent ion pairs involving cyclometalated bidentate platinum complexes have been reported.24,27b,c The square-planar coordination geometry of the d8 platinum center as well as the flat π ligands provides access to axial coordination sites and leads to possible π–π and Pt⋯Pt intermolecular interactions allowing the salt complexes to exhibit unique optical properties in solution and solid state. As extensive studies on iridium(III) salts demonstrate, by altering the chemical structure of the ligands, the photophysical properties of soft salt complexes can be easily controlled.20a,b,28 Despite the excellent photophysical properties and ease of phosphorescence tuning in isolated examples of ion-paired platinum complex, the development of a comparative study of a series of ligand-modifiable platinum soft salt complexes has been relatively unexplored until now.
In the continuation of our work on phosphorescent bidentate and tridentate cyclometalated platinum(II) complexes,29 and to address this limitation, we herein present a series of novel soft salts based on two oppositely charged cyclometalated platinum complexes S1–9 (Chart 1). Our approach was to investigate the structure–property relationships within a class of Pt(II) soft salts bearing modified C^N ligands. For this purpose, the platinum(II) complexes of [Pt(C^N)(en)]+Cl− with C^N bidentate ligands (en = ethane-1,2-diamine, C^N = 2-(4-(trifluoromethyl)phenyl)-pyridine (C1) and 2-(4-(trifluoromethyl)phenyl)-pyrimidine (C2)) were chosen as the cationic components. The platinum(II) complexes of [Pt(C^N)(CN)2]−Bu4N+A1–7 containing various cyclometalating ligands were selected as the anionic components. The cationic and anionic components were combined to construct the corresponding soft salt complexes S1–9 ([Pt(C^N)(en)]+[Pt(C^N)(CN)2]−) via van der Waals forces and electrostatic interactions. These new soft salt complexes S1–9 exhibited interesting changes in their optical properties compared to their analogous complex S0 (Chart 1) previously designed by Wong et al.24 Finally, the response to external stimuli, particularly vapochromism and halochromism properties has been successfully explored in selected soft salts, demonstrating the utility of these complexes as potential materials for anti-counterfeiting applications.
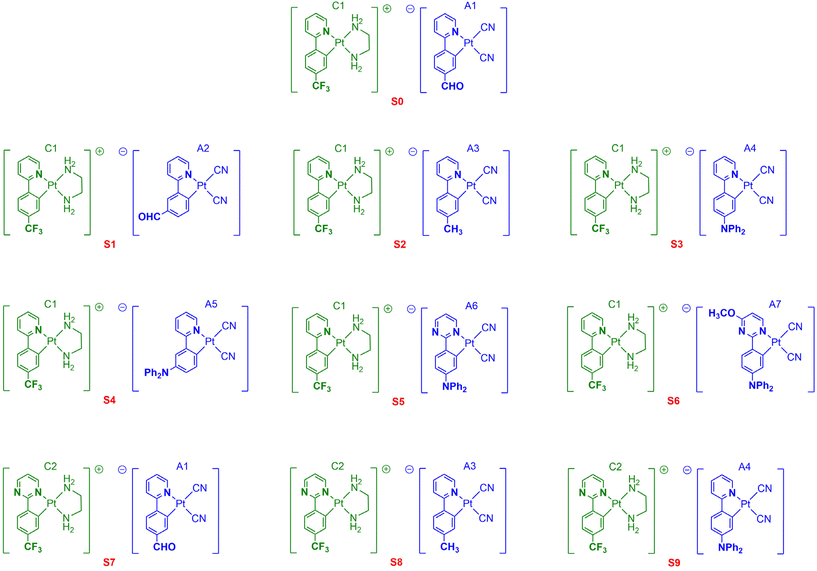 |
| Chart 1 Chemical structures of the cyclometalated soft salt platinum complexes S0–9 investigated in this work. | |
Results and discussion
Synthesis and characterization
The synthesis of the anionic A1–7 and cationic C1–2 platinum(II) complexes requires the cyclometalating bidentate C^N ligands L1-9, which are commercially available or synthetized in moderate-to-good yields by Suzuki–Miyaura cross-coupling reaction of 2-bromopyridine, 2-bromo-4-methoxypyrimidine, or 2-chloropyrimidine with appropriate phenylboronic acid derivatives under classical conditions (ESI, Scheme S1†).30 The reaction of K2PtCl4 with the corresponding bidentate C^N ligands L1-9 leads to the intermediate Pt(II) μ-chloro-bridged dimer complexes, intermediate as previously reported (Scheme 1a).31 Anionic A1–7 and cationic C1–2 platinum(II) complexes were prepared according to a well-stablished “chloride substitution” reaction.32 The cationic platinum(II) complexes C1–2 were synthesized by stirring the Pt(II) μ-chloro-bridged dimer complexes with ethylenediamine (3 equiv.) in dichloromethane in good yields, while the anionic platinum(II) complexes A1–7 were prepared in low to moderate yields by stirring with tetrabutylammonium cyanide (4 equiv.) in dichloromethane at 50 °C for 5 h. The soft salt complexes S1–9 were obtained by mixing oppositely charged platinum(II) complexes A1–7 and C1–2 in ethanol through metathesis reactions in moderate-to-good yields (Scheme 1b).24 The desired platinum(II) complexes were characterized by electrospray ionization high resolution mass spectrometry (ESI-HRMS) as well as 1H and 13C nuclear magnetic resonance (NMR) spectroscopy. The characterization data were found to be in complete agreement with the proposed structures as detailed in the ESI.†
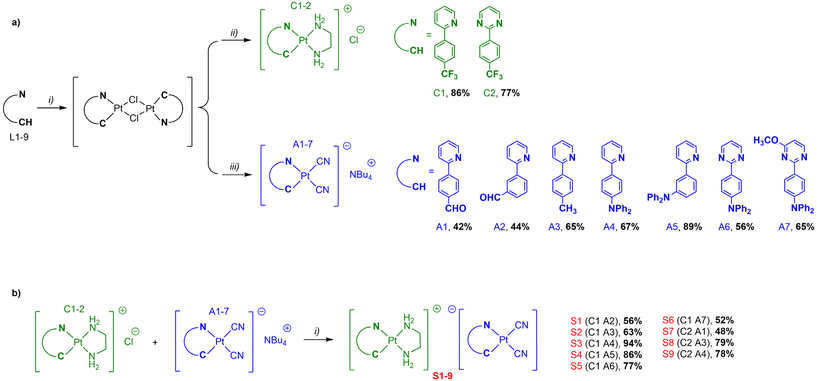 |
| Scheme 1 (a) Synthesis of the anionic and cationic complexes C1–2 and A1–7. Reagents and conditions: (i) K2PtCl4, ethoxyethanol/H2O: 3/1, N2, 80 °C, 24 h, (ii) ethylenediamine, CH2Cl2, N2, r.t., 3 h, (iii) nBu4NCN, CH2Cl2, N2, 50 °C, 5 h; (b) synthesis of soft salt platinum complexes S1–9. Reagents and conditions: (i) EtOH, N2, sonic bath, r.t., 10 min. | |
Photophysical properties
The photophysical properties of soft salts S0–9 and their precursors were measured in DMSO and the data are presented in Tables 1 and S1,† respectively. As illustrated in Fig. 1 for soft salt S5, absorption and photoluminescence maxima globally align with the data of the anionic precursor. Soft salts composed of an anionic part with a cyclometalating ligand bearing a diphenylamino group (S3–S6 and S9) exhibit a broad emission band (Fig. S53–S55 and S58†), whereas other soft salts display a structured emission profile with vibrational spacing of about 1300 cm−1 (Fig. S52–S53 and S56–57†) as often observed for Pt(II) complexes.29b,c,33 When the electron-donating group is in a conjugated position with respect to the Pt(II) center in the A4 anion of the S4 salt, a significant red-shift in emission is observed, in accordance with what is observed in neutral complexes (S4: λem = 619 nm; S3: λem = 513 nm).29a Moreover, a pyrimidine-based C^N cyclometalating ligand on the anionic complex induces a red-shift in emission (S5: λem = 567 nm and S6: λem = 546 nm vs.S3: λem = 513 nm). Nevertheless, the presence of an electron-donating methoxy group on the pyrimidine ring in case of soft salt S6 restrains the red-shift due to the reduction of the electron withdrawing character of the pyrimidine.29d In term of PLQY, only the soft salts S3–S6 and S9 with NPh2 based cationic part exhibit ΦPL > 1%. Pyrimidine-based cyclometalating C^N ligands in the A6 and A7 anions of S5 and S6 soft salts contribute to an increase in the PLQY value, with the soft salt S6 bearing a methoxy-substituted pyrimidine cyclometalating ligand exhibiting the highest value in the series (ΦPL = 20%). On the other hand, the localization of the NPh2 group in a conjugated position to the Pt(II) center (S4) or the presence of a pyrimidine cyclometalating C^N ligand on the cationic part (S9) decrease the PLQY.
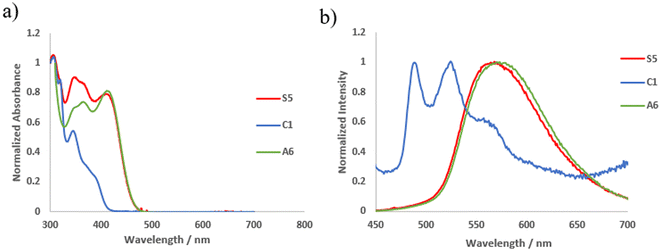 |
| Fig. 1 (a) Normalized UV-Vis absorption spectra of S5, C1, and A6 in degassed DMSO solution (10−5 M); (b) normalized emission spectra of S5, C1, and A6 in degassed DMSO solution (10−5 M). | |
Table 1 Photophysical properties of soft salts S0–S9 in degassed DMSO solution (10−5 M)
Soft salts |
Absorption λmax/nm (ε/mM−1 cm−1) |
Emission λmax/nm |
Φ
PL a |
PLQY (±10%) measured relative to 9,10-bisphenylethynylanthracene in cyclohexane (ΦPL = 1.00).34
|
S0 24 |
280 (50.3), 298 (41.1), 322 (29.4), 335 (24.6), 382 (7.5) |
491, 530, 567 |
0.01 |
S1
|
321 (15.0), 331 (9.7), 350 (10.5), 388 (2.7) |
479, 515sh |
<0.01 |
S2
|
317 (24.4), 334 (21.4), 341 (15.4), 387 (6.0) |
487, 523, 559sh |
<0.01 |
S3
|
340 (28.2), 377 (14.5), 413 (18.4) |
513
|
0.09 |
S4
|
342 (14.1), 388 (4.7) |
619
|
0.05 |
S5
|
324 (28.3), 352 (32.2), 368 (31.3), 380 (27.5), 410 (31.0) |
567
|
0.14 |
S6
|
338 (27.5), 374 (16.6), 410 (23.0) |
501sh, 546 |
0.20 |
S7
|
323 (23.1), 334 (22.5), 400 (4.4) |
480, 513, 560sh |
<0.01 |
S8
|
322 (21.1), 334 (21.4), 381 (5.7) |
489, 523, 560sh |
<0.01 |
S9
|
329 (43.4), 342 (40.0), 408 (29) |
512
|
0.03 |
The effect of aggregation on the photophysical properties of soft salts was studied by registering absorption and emission spectra in a mixture of DMSO and water with an increasing water ratio. As illustrated in Fig. 2, S5 exhibits aggregation-induced emission (AIE): when the water fraction is increased up to 80%, a slight red-shift of emission is observed with a decrease in emission intensity, explained by an increase in the polarity of the solvent. With a higher water fraction, a new red-shifted band centered at 683 nm emerges, accompanied by a dramatic increase in emission intensity. This phenomenon is attributed to the formation of aggregates and metal–metal-to ligand-charge transfer (MMLCT) excited state. Additional evidence is provided by the 1H NMR spectra obtained at different DMSO–water compositions (10−4 M) in order to examine the aggregation behavior of the two ionic components. The soft salt complex S5 showed aggregation in DMSO when the water content increased. As shown in Fig. S50,† well-resolved proton signals with clear splitting patterns corresponding to S5 can be observed in DMSO-d6. Protons signals became poorly resolved and broad when the D2O content was increased, reaching complete obscurity at 50%. This result is in accordance with the conclusions obtained in previous reports,24,27a showing the presence of a substantial amount of π–π stacking interactions between the two oppositely charged ions at high water contents. AIE has already been described for S0
24 and is also observed for soft salts S1, S7, and S8 (Fig. S59, S64, and S65†). For soft salt S4, in contrast to other cases, AIE is associated with a blue shift in emission, that might be attributed to a transition that is not related to MMLCT due to the formation of another type of aggregate (Fig. S62†). In case of S3, S6, and S9, only a slight increase in emission intensity is observed (Fig. S61, S63, and S66†), and this phenomenon can be considered as aggregation-induced emission enhancement (AIEE). Similar experiments were performed on anionic and cationic precursors A4 and C1 (Fig. S67†): whereas cationic complex C1 shows AIE, the emission intensity remained low. On the other hand, anionic complex A4 exhibits aggregation caused quenching (ACQ) indicating that AIE and AIEE observed is related to soft salt structure.
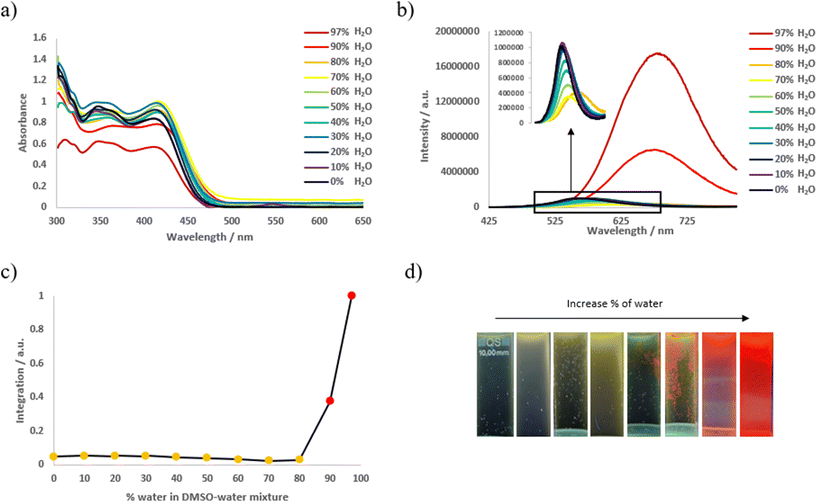 |
| Fig. 2 (a) UV-Vis absorption spectra of S5 in DMSO–water mixtures (10−3 M) with different water ratio; (b) emission spectra of S5 in DMSO–water mixtures (10−3 M) with different water ratio; (c) integration of emission band vs. percentage of water in DMSO/water mixtures; (d) pictures of S5 solutions in DMSO/water mixtures with various percentages of water taken in the dark upon irradiation with a handheld UV lamp (λem = 254 nm). | |
The photophysical properties of the series of soft salt complexes S0–9 were also measured in the solid state (1 wt% in KBr pellets), and the results are summarized in Table 2. All soft salts exhibited red/near-infrared (NIR) emission with broad, non-structured emission bands (Fig. 3 and S68†), as well as emission lifetimes ranging from 6.3 to 37.2 μs, characteristic of phosphorescence emission. The red-shifted emission of soft salts observed in solid state with regards to anionic precursors is attributed to MMLCT excited state. The highest radiative constants kr were observed for soft salts S4 and S5, which are based on an anionic complex with a NPh2-substituted cyclometalating ligand. As far as the substituents on the N^C cyclometalating pyridine ligand are concerned, when comparing soft salts S0, S2, and S3, the presence of an electron-withdrawing formyl group (S0) induces a significant red shift in emission (Fig. 4a) associated with a decrease in PLQY. Soft salts S3 and S4, which differ in the position of the NPh2 group in the phenyl ring of the C^N ligand of the anionic complex, were compared. It is observed that S4, with the NPh2 group in a conjugated position to the Pt(II) center, exhibits a blue-shifted emission (Fig. 4b) with an increased PLQY. This is contrary to what is observed in solution but comparable to AIE phenomenon. This tends to indicate that metal-to-ligand charge transfer (MLCT) plays a minor role in solid-state emission. The presence of a pyrimidine C^N ligand, either in the anionic or cationic complexes within the structure of S5 and S9, respectively, induces a red-shift in emission compared to the pyridine analogue S3 (Fig. 4c and d). Regarding the PLQY, it is in the same order when the pyrimidine cyclometalating ligand is in the anionic part and is 2.7 fold lower when the pyrimidine ligand is in the cationic part. An opposite trend is observed for soft salts with an anionic complex based on a methyl-substituted cyclometalating ligand (S8vs.S2).
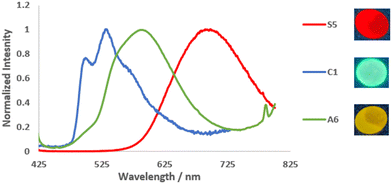 |
| Fig. 3 Left: PL spectra in the solid state of S5, C1, and A6 at 298 K. Right: Pictures of KBr pellets of S5, C1, and A6 in the dark upon irradiation with a handheld UV lamp (λem = 365 nm). | |
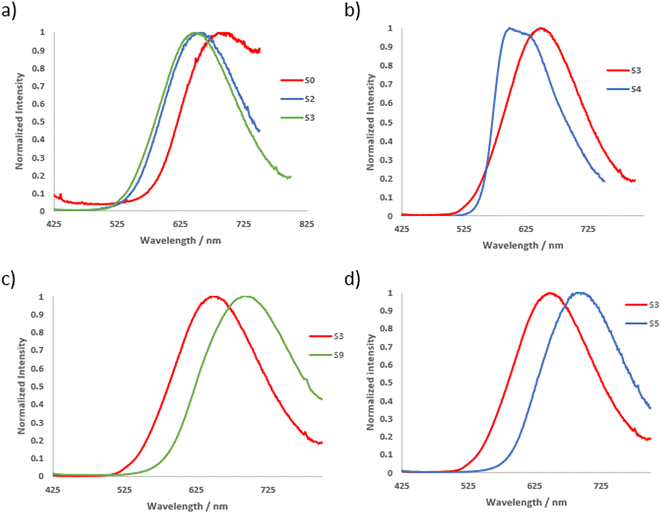 |
| Fig. 4 (a) Normalized emission spectra in the solid state for S0, S2, and S3; (b) normalized emission spectra in the solid state for S3 and S4; (c) normalized emission spectra in the solid state for S3 and S9; (d) normalized emission spectra in the solid state for S3 and S5. | |
Table 2 Photophysical properties of S0–S9 in the solid state (1 wt% KBr pellets)
Soft salt complexes |
Emission λmax/nm |
Φ
PL a (τ0/μs) |
k
r (s−1) |
k
nr (s−1) |
CIE chromaticity coordinates (x; y) |
Measured as powder with an integrating sphere.
|
S0
|
692 |
0.06 (9.2) |
6.5 × 103 |
1.0 × 105 |
(0.56; 0.32) |
S1
|
688 |
0.02 (21.5) |
0.9 × 103 |
4.6 × 104 |
(0.62; 0.36) |
S2
|
656 |
0.19 (37.2) |
5.1 × 103 |
2.2 × 104 |
(0.61; 0.38) |
S3
|
650 |
0.19 (19.2) |
9.9 × 103 |
4.2 × 104 |
(0.60; 0.39) |
S4
|
629 |
0.36 (14.3) |
2.5 × 104 |
4.5 × 104 |
(0.60; 0.40) |
S5
|
694 |
0.17 (10.4) |
1.6 × 104 |
7.9 × 104 |
(0.66; 0.33) |
S6
|
679 |
0.13 (15.8) |
8.2 × 103 |
5.5 × 104 |
(0.57; 0.41) |
S7
|
727 |
0.03 (6.3) |
6.2 × 103 |
8.2 × 104 |
(0.59; 0.34) |
S8
|
652 |
0.09 (13.8) |
6.5 × 103 |
6.6 × 104 |
(0.61; 0.34) |
S9
|
695 |
0.07 (11.3) |
4.8 × 103 |
1.5 × 105 |
(0.65; 0.33) |
Response to external stimuli
The sensitivity to external stimuli of selected soft salts was also investigated. Incorporating solvent molecules into the solid-state structure provides a means to manipulate the metal–metal distances of the Pt(II) complexes, as documented in the literature.27a,35 Vapochromic behavior was observed in the case of S3 (Fig. 5). Upon exposure to MeOH vapor, the solid-state emission shifted from 650 to 690 nm, with a similar effect observed with CHCl3 vapors. This suggests a closer proximity between Pt(II) units and stronger Pt⋯Pt interactions, which favor MMLCT transitions.19e No further changes in emission were observed after a few hours, even under reduced pressure. In the presence of acetone vapors, the initial emission was restored, as was the case with DCM or THF, or after grinding. Four cycles were conducted. It is noteworthy that after the first cycle, the red-shift induced by MeOH vapor was slightly reduced (the emission band is centered at 677 nm instead of 690 nm). Soft salt S4 exhibited similar vapochromic properties (Fig. S69†): the presence of acetone vapors induced an irreversible change in the emission band from 600 nm to 634 nm. This emission was red-shifted to 670 nm in the presence of CHCl3, and this phenomenon was reversible in the presence of acetone vapors. Similar experiments were performed on cationic and anionic precursors C1 and A4 and no vapochromism were observed in that cases (Fig. S70†), highlighting the crucial effect of the soft salt structure of S3.
 |
| Fig. 5 (a) Vapochromic behavior of S3 in the solid state (KBr powder with 1% wt of soft salt). Pictures were taken in the dark upon irradiation with a handheld UV lamp (λem = 365 nm). Conditions: (i) MeOH vapors, (ii) Acetone vapors; (b) normalized PL spectra of S3 in the initial state and in presence of MeOH/acetone vapors; (c) emission wavelength variations measured after 5 repeating fuming cycles with MeOH/acetone. | |
Pyrimidine chromophores are known for the sensitivity of their photophysical properties to the presence of acid.36 In pyrimidine-based Pt(II) complexes, one of the nitrogen atoms is not involved in complexation and is available for interaction with a proton. This was examined using soft salt S5. In the presence of HCl vapors, the emission is fully quenched, and this phenomenon was qualitatively reversible in the presence of basic ammonia vapors. Although the initial intensity was not fully recovered, the emission intensity of the neutral species could be maintained after three protonation/deprotonation cycles (Fig. 6). The soft salt S9, where the pyrimidine-based ligand is part of the cationic complex, showed a similar behavior, remaining slightly emissive after exposure to HCl vapors (Fig. S72†). On the other hand, in the case of the pyridine analogue S3, after an initial slight decrease, the emission intensity was not influenced by HCl and NH3 vapors, indicating that the emission quenching is due to the protonation of the pyrimidine ring (Fig. S71†).
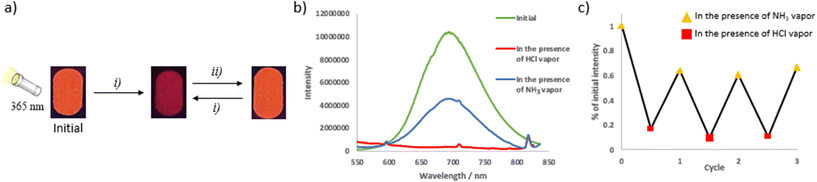 |
| Fig. 6 (a) Response of S5 to acids in the solid state (KBr powder with 1% wt of soft salt). Pictures were taken in the dark upon irradiation with a handheld UV lamp (λem = 365 nm). Conditions: (i) HCl vapors (ii) NH3 vapors; (b) PL spectra of S5 in the initial state and in presence of HCl/NH3 vapors; (c) percentage variation in initial emission intensity measured after 3 repeated exposure cycles to HCl/NH3 vapors. | |
Conclusion
In conclusion, a new series of phosphorescent soft salts, S1–S9, composed of platinum(II) complexes with opposite charges, was synthesized through a metathesis reaction involving specific anionic and cationic Pt(II) complexes. The phosphorescence emission of both soft salts and their precursor complexes was studied to analyze the color tuning of emission resulting from the association of complementary charge species. Absorption and emission wavelengths, PLQYs, and emission lifetimes (in solid state) were determined for all soft salt complexes in both degassed solution and the solid state. These complexes exhibit phosphorescent emission in degassed DMSO solution with low to moderate PLQY. In the solid state, more intense and tunable emissions were observed, with red-shifted emission detected for most soft salt complexes compared to the values obtained for the corresponding anionic emissive complexes. AIE was observed for some soft salt complexes in water/DMSO solutions as the water fraction increased. With a higher water fraction, a new red-shifted emission band emerged, corresponding to the emission maxima observed in the solid state. This series of Pt(II) complexes-based soft salt materials serves to elucidate structure–properties relationships in terms of azaheterocycles (pyridine vs. pyrimidine), as well as the type and position of electron-withdrawing or electron-donating groups on the cyclometalating ligands.
The incorporation of a pyrimidine ring into the cyclometalating ligand, unlike traditional pyridine, is another noteworthy aspect of this study. The soft salt complexes exhibit a red-shift due to the presence of the pyrimidine group in the anionic Pt(II) complex, and reversible quenching of solid-state emission occurs in the presence of acidic vapors. The demonstrated reversible vapochromic response of selected soft salt complexes indicates the potential of these new materials for anti-counterfeiting applications.
Data availability
The data supporting this article have been included as part of the ESI.†
Conflicts of interest
There are no conflicts to declare.
Acknowledgements
A. R. acknowledges the support from Région Bretagne France, and Conseil Départemental des Côtes d'Armor, France for his PhD funding (INIMITABLE project). The authors are grateful to the EUR LUMOMAT project and the Investments for the Future program ANR-18-EURE-0012.
References
-
(a) Z. Li, H. Chen, B. Li, Y. Xie, X. Gong, X. Liu, H. Li and Y. Zhao, Adv. Sci., 2019, 6, 1901529 CrossRef CAS PubMed;
(b) K. Mutoh, N. Miyashita, K. Arai and J. Abe, J. Am. Chem. Soc., 2019, 141, 5650 CrossRef CAS PubMed;
(c) Y. Zhuang, X. Ren, X. Che, S. Liu, W. Huang and Q. Zhao, Adv. Photonics, 2021, 3, 014001 Search PubMed.
-
(a) C.-Q. Jing, J.-H. Wu, Y.-Y. Cao, H.-X. Che, X.-M. Zhao, M. Yue, Y.-Y. Liao, C.-Y. Yue and X.-W. Lei, Chem. Commun., 2020, 56, 5925 RSC;
(b) S. Sun, J. Wang, L. Ma, X. Ma and H. Tian, Angew. Chem., Int. Ed., 2021, 60, 18557 CrossRef CAS PubMed.
-
(a) G. Huang, Y. Jiang, S. Yang, B. S. Li and B. Z. Tang, Adv. Funct. Mater., 2019, 29, 1900516 CrossRef;
(b) Y. Gong, S. He, Y. Li, Z. Li, Q. Liao, Y. Gu, J. Wang, B. Zou, Q. Li and Z. Li, Adv. Opt. Mater., 2020, 8, 1902036 CrossRef CAS;
(c) A. E. Norton, M. K. Abdolmaleki, J. Liang, M. Sharma, R. Golsby, A. Zoller, J. A. Krause, W. B. Connick and S. Chatterjee, Chem. Commun., 2020, 56, 10175 RSC.
-
(a) H. Zou, Y. Hai, H. Ye and L. You, J. Am. Chem. Soc., 2019, 141, 16344 CrossRef CAS PubMed;
(b) L. M. C. Luong, M. A. Malwitz, V. Moshayedi, M. M. Olmstead and A. L. Balch, J. Am. Chem. Soc., 2020, 142, 5689 CrossRef CAS PubMed.
-
(a) J. Chen, Z. Wang, C. Liu, Z. Chen, X. Tang, Q. Wu, S. Zhang, G. Song, S. Cong, Q. Chen and Z. Zhao, Adv. Mater., 2021, 33, 2007314 CrossRef CAS PubMed;
(b) W. Ma, L. Xu, S. Zhang, G. Li, T. Ma, B. Rao, M. Zhang and G. He, J. Am. Chem. Soc., 2021, 143, 1590 CrossRef CAS PubMed.
-
(a) Y. Guo, S. Gu, X. Feng, J. Wang, H. Li, T. Han, Y. Dong, X. Jiang, T. D. James and B. Wang, Chem. Sci., 2014, 5, 4388 RSC;
(b) Y. Zhang, Y. Li, H. Wang, Z. Zhang, Y. Feng, Q. Tian, N. Li, J. Mei, J. Su and H. Tian, ACS Appl. Mater. Interfaces, 2019, 11, 39351 CrossRef CAS PubMed.
-
(a) Y. Ma, L. Shen, P. She, Y. Hou, Y. Yu, J. Zhao, S. Liu and Q. Zhao, Adv. Opt. Mater., 2019, 7, 1801657 CrossRef;
(b) Y. Shigeta, A. Kobayashi, M. Yoshida and M. Kato, Inorg. Chem., 2019, 58, 7385 CrossRef CAS PubMed.
-
(a) Y. Ding, S. Jiang, Y. Gao, J. Nie, H. Du and F. Sun, Macromolecules, 2020, 53, 5701 CrossRef CAS;
(b) C. Calvino, A. Guha, C. Weder and S. Schrettl, Adv. Mater., 2018, 30, 1704603 CrossRef PubMed.
-
(a) P. Wei, J.-X. Zhang, Z. Zhao, Y. Chen, X. He, M. Chen, J. Gong, H. H.-Y. Sung, I. D. Williams, J. W. Y. Lam and B. Z. Tang, J. Am. Chem. Soc., 2018, 140, 1966 CrossRef CAS PubMed;
(b) A. Kometani, Y. Inagaki, K. Mutoh and J. Abe, J. Am. Chem. Soc., 2020, 142, 7995 CrossRef CAS PubMed.
-
(a) W. Zhao, Z. He, Q. Peng, J. W. Y. Lam, H. Ma, Z. Qiu, Y. Chen, Z. Zhao, Z. Shuai, Y. Dong and B. Z. Tang, Nat. Commun., 2018, 9, 3044 CrossRef PubMed;
(b) B. Hupp, J. Nitsch, T. Schmitt, R. Bertermann, K. Edkins, F. Hirsch, I. Fischer, M. Auth, A. Sperlich and A. Steffen, Angew. Chem., Int. Ed., 2018, 57, 13671 CrossRef CAS PubMed;
(c) Z. He, H. Gao, S. Zhang, S. Zheng, Y. Wang, Z. Zhao, D. Ding, B. Yang, Y. Zhang and W. Z. Yuan, Adv. Mater., 2019, 31, 1807222 CrossRef PubMed.
-
(a) P. Kar, M. Yoshida, Y. Shigeta, A. Usui, A. Kobayashi, T. Minamidate, N. Matsunaga and M. Kato, Angew. Chem., Int. Ed., 2017, 56, 2345 CrossRef CAS PubMed;
(b) C.-Y. Lien, Y.-F. Hsu, Y.-H. Liu, S.-M. Peng, T. Shinmyozu and J.-S. Yang, Inorg. Chem., 2020, 59, 11584 CrossRef CAS PubMed.
-
(a) W. Zhang, Y.-M. Zhang, F. Xie, X. Jin, J. Li, G. Yang, C. Gu, Y. Wang and S. X.-A. Zhang, Adv. Mater., 2020, 32, 2003121 CrossRef CAS PubMed;
(b) Y. Wang, S. Wang, X. Wang, W. Zhang, W. Zheng, Y.-M. Zhang and S. X.-A. Zhang, Nat. Mater., 2019, 18, 1335 CrossRef CAS PubMed.
- J. Du, L. Sheng, Q. Chen, Y. Xu, W. Li, X. Wang, M. Li and S. X.-A. Zhang, Mater. Horiz., 2019, 6, 1654 RSC.
-
(a) M. A. Baldo, D. F. O'Brien, Y. You, A. Shoustikov, S. Sibley, M. E. Thompson and S. R. Forrest, Nature, 1998, 395, 151 CrossRef CAS;
(b) S. Lamansky, P. Djurovich, D. Murphy, F. Abdel-Razzaq, H.-E. Lee, C. Adachi, P. E. Burrows, S. R. Forrest and M. E. Thompson, J. Am. Chem. Soc., 2001, 123, 4304 CrossRef CAS PubMed;
(c) N. T. Kalyani and S. J. Dhoble, Renewable Sustainable Energy Rev., 2012, 16, 2696 CrossRef;
(d) R.-P. Xu, Y.-Q. Li and J.-X. Tang, J. Mater. Chem. C, 2016, 4, 9116 RSC;
(e) A. Salehi, X. Fu, D.-H. Shin and F. So, Adv. Funct. Mater., 2019, 29, 1808803 CrossRef;
(f) G. Hong, X. Gan, C. Leonhardt, Z. Zhang, J. Seibert, J. M. Busch and S. Bräse, Adv. Mater., 2021, 33, 2005630 CrossRef CAS PubMed.
-
(a) J. Twilton, C. Le, P. Zhang, M. H. Shaw, R. W. Evans and D. W. C. MacMillan, Nat. Rev. Chem., 2017, 1, 0052 CrossRef CAS;
(b) J. He, Z.-Q. Bai, P.-F. Yuan, L.-Z. Wu and Q. Liu, ACS Catal., 2021, 11, 446 CrossRef CAS.
-
(a) M. Irie, T. Fukaminato, T. Sasaki, N. Tamai and T. Kawai, Nature, 2002, 420, 759 CrossRef CAS PubMed;
(b) P. Zijlstra, J. W. M. Chon and M. Gu, Nature, 2009, 459, 410 CrossRef CAS PubMed;
(c) Q.-F. Gu, J.-H. He, D.-Y. Chen, H.-L. Dong, Y.-Y. Li, H. Li, Q.-F. Xu and J.-M. Lu, Adv. Mater., 2015, 27, 5968 CrossRef CAS PubMed;
(d) C.-L. Wong, M. Ng, E. Y.-H. Hong, Y.-C. Wong, M.-Y. Chan and V. W.-W. Yam, J. Am. Chem. Soc., 2020, 142, 12193 CrossRef CAS PubMed.
-
(a) J. Xu, P. Yang, M. Sun, H. Bi, B. Liu, D. Yang, S. Gai, F. He and J. Lin, ACS Nano, 2017, 11, 4133 CrossRef CAS PubMed;
(b) V. W.-W. Yam and A. S.-Y. Law, Coord. Chem. Rev., 2020, 414, 213298 CrossRef CAS;
(c) K. K.-W. Lo, Acc. Chem. Res., 2020, 53, 32 CrossRef CAS PubMed.
-
(a) X. Hou, C. Ke, C. J. Bruns, P. R. McGonigal, R. B. Pettman and J. F. Stoddart, Nat. Commun., 2015, 6, 6884 CrossRef PubMed;
(b) Z. Mao, Z. Yang, Y. Mu, Y. Zhang, Y.-F. Wang, Z. Chi, C.-C. Lo, S. Liu, A. Lien and J. Xu, Angew. Chem., Int. Ed., 2015, 54, 6270 CrossRef CAS PubMed;
(c) J. Liu, H. Rijckaert, M. Zeng, K. Haustraete, B. Laforce, L. Vincze, I. Van Driessche, A. M. Kaczmarek and R. Van Deun, Adv. Funct. Mater., 2018, 28, 1707365 CrossRef;
(d) Y. Ma, Y. Dong, S. Liu, P. She, J. Lu, S. Liu, W. Huang and Q. Zhao, Adv. Opt. Mater., 2020, 8, 1901687 CrossRef CAS;
(e) Z. Li, X. Liu, G. Wang, B. Li, H. Chen, H. Li and Y. Zhao, Nat. Commun., 2021, 12, 1363 CrossRef CAS PubMed.
-
(a) Z.-q. Chen, Z.-q. Bian and C.-h. Huang, Adv. Mater., 2010, 22, 1534 CrossRef CAS PubMed;
(b) H. Sasabe, J.-i. Takamatsu, T. Motoyama, S. Watanabe, G. Wagenblast, N. Langer, O. Molt, E. Fuchs, C. Lennartz and J. Kido, Adv. Mater., 2010, 22, 5003 CrossRef CAS PubMed;
(c) Y. You and W. Nam, Chem. Soc. Rev., 2012, 41, 7061 RSC;
(d) H. Xiang, J. Cheng, X. Ma, X. Zhou and J. J. Chruma, Chem. Soc. Rev., 2013, 42, 6128 RSC;
(e) Y. Liu, P. Zhang, X. Fang, G. Wu, S. Chen, Z. Zhang, H. Chao, W. Tan and L. Xu, Dalton Trans., 2017, 46, 4777 RSC;
(f) T. V. Esipova, H. J. Rivera-Jacquez, B. Weber, A. E. Masunov and S. A. Vinogradov, J. Am. Chem. Soc., 2016, 138, 15648 CrossRef CAS PubMed;
(g) M. A. Soto, R. Kandel and M. J. MacLachlan, Eur. J. Inorg. Chem., 2021, 894 CrossRef CAS.
-
(a) D. R. Martir and E. Zysman-Colman, Coord. Chem. Rev., 2018, 364, 86 CrossRef;
(b) M. Yun, C. Kexin, G. Zeling, L. Shujuan, Z. Qiang and W. Wai-Yeung, Acta Chim. Sin., 2020, 78, 23 CrossRef.
-
(a) G. Nasr, A. Guerlin, F. Dumur, L. Beouch, E. Dumas, G. Clavier, F. Miomandre, F. Goubard, D. Gigmes, D. Bertin, G. Wantz and C. R. Mayer, Chem. Commun., 2011, 47, 10698 RSC;
(b) F. Dumur, G. Nasr, G. Wantz, C. R. Mayer, E. Dumas, A. Guerlin, F. Miomandre, G. Clavier, D. Bertin and D. Gigmes, Org. Electron., 2011, 12, 1683 CrossRef CAS.
- Y. Ma, H. Liang, Y. Zeng, H. Yang, C.-L. Ho, W. Xu, Q. Zhao, W. Huang and W.-Y. Wong, Chem. Sci., 2016, 7, 3338 RSC.
- Y. Ma, S. Zhang, H. Wei, Y. Dong, L. Shen, S. Liu, Q. Zhao, L. Liu and W.-Y. Wong, Dalton Trans., 2018, 47, 5582 RSC.
- Y. Ma, K. Chen, J. Lu, J. Shen, C. Ma, S. Liu, Q. Zhao and W.-Y. Wong, Inorg. Chem., 2021, 60, 7510 CrossRef CAS PubMed.
- C. Wu, H.-F. Chen, K.-T. Wong and M. E. Thompson, J. Am. Chem. Soc., 2010, 132, 3133 CrossRef CAS PubMed.
-
(a) M. Sandroni and E. Zysman-Colman, Dalton Trans., 2014, 43, 3676 RSC;
(b) K. N. Swanick, M. Sandroni, Z. Ding and E. Zysman-Colman, Chem. – Eur. J., 2015, 21, 7435 CrossRef CAS PubMed;
(c) Q. Liu, M. Xie, X. Chang, S. Cao, C. Zou, W.-F. Fu, C.-M. Che, Y. Chen and W. Lu, Angew. Chem., Int. Ed., 2018, 57, 6279 CrossRef CAS PubMed.
-
(a) V. C.-H. Wong, C. Po, S. Y.-L. Leung, A. K.-W. Chan, S. Yang, B. Zhu, X. Cui and V. W.-W. Yam, J. Am. Chem. Soc., 2018, 140, 657 CrossRef CAS PubMed;
(b) J. Li, Y. Ma, S. Liu, Z. Mao, Z. Chi, P.-C. Qian and W.-Y. Wong, Chem. Commun., 2020, 56, 11681 RSC;
(c) J. Li, K. Chen, J. Wei, Y. Ma, R. Zhou, S. Liu, Q. Zhao and W.-Y. Wong, J. Am. Chem. Soc., 2021, 143, 18317 CrossRef CAS PubMed.
-
(a) V. Fiorini, A. D'Ignazio, K. D. M. Magee, M. I. Ogden, M. Massi and S. Stagni, Dalton Trans., 2016, 45, 3256 RSC;
(b) S. Guo, T. Huang, S. Liu, K. Y. Zhang, H. Yang, J. Han, Q. Zhao and W. Huang, Chem. Sci., 2017, 8, 348 RSC.
-
(a) M. Fecková, S. Kahlal, T. Roisnel, J.-Y. Saillard, J. Boixel, M. Hruzd, P. le Poul, S. Gauthier, F. Robin-le Guen, F. Bureš and S. Achelle, Eur. J. Inorg. Chem., 2021, 1592 CrossRef;
(b) M. Hruzd, S. Gauthier, J. Boixel, S. Kahlal, N. le Poul, J.-Y. Saillard, S. Achelle and F. Robin-le Guen, Dyes Pigm., 2021, 194, 109622 CrossRef CAS;
(c) M. Hruzd, N. le Poul, M. Cordier, S. Kahlal, J.-Y. Saillard, S. Achelle, S. Gauthier and F. Robin-le Guen, Dalton Trans., 2022, 51, 5546 RSC;
(d) M. Hruzd, S. Kahlal, N. le Poul, L. Wojcik, M. Cordier, J.-Y. Saillard, J. Rodríguez-López, F. Robin-le Guen, S. Gauthier and S. Achelle, Dalton Trans., 2023, 52, 1927 RSC.
-
(a) S. Achelle, J. Rodríguez-López, M. Larbani, R. Plaza-Pedroche and F. Robin-le Guen, Molecules, 2019, 24, 1742 CrossRef CAS PubMed;
(b) M. Klikar, P. le Poul, A. Růžička, O. Pytela, A. Barsella, K. D. Dorkenoo, F. Robin-le Guen, F. Bureš and S. Achelle, J. Org. Chem., 2017, 82, 9435 CrossRef CAS PubMed.
- L. Ricciardi, M. La Deda, A. Ionescu, N. Godbert, I. Aiello and M. Ghedin, Dalton Trans., 2017, 46, 12625 RSC.
-
(a) A. F. Rausch, U. V. Monkowius, M. Zabel and H. Yersin, Inorg. Chem., 2010, 49, 7818 CrossRef CAS PubMed;
(b) S. S. Pasha, P. Das, N. P. Rath, D. Bandyopadhyay, N. R. Jana and I. R. Laskar, Inorg. Chem. Commun., 2016, 67, 107 CrossRef CAS.
- Z. Wang, E. Turner, V. Mahoney, S. Madakuni, T. Groy and J. Li, Inorg. Chem., 2010, 49, 11276 CrossRef CAS PubMed.
- M. Taniguchi and J. S. Lindsey, Photochem. Photobiol., 2018, 94, 290 CrossRef CAS PubMed.
-
(a) O. S. Wenger, Chem. Rev., 2013, 113, 3686 CrossRef CAS PubMed;
(b) A. Kobayashi and M. Kato, Eur. J. Inorg. Chem., 2014, 4469 CrossRef CAS;
(c) J. Ni, Z. Guo, Q. Zhu, S. Liu and J. Zhang, Dyes Pigm., 2023, 217, 111406 CrossRef CAS;
(d) M. Shiotsuka, M. Ogihara, T. Hanada and K. Kasai, J. Organomet. Chem., 2022, 965–966, 122334 CrossRef CAS.
-
(a) S. Achelle, J. Rodríguez-López, F. Bureš and F. Robin-le Guen, Chem. Rec., 2020, 20, 440 CrossRef CAS PubMed;
(b) M. Hodée, A. Lenne, J. Rodríguez-López, F. Robin-le Guen, C. Katan, S. Achelle and A. Fihey, New J. Chem., 2021, 45, 19132 RSC;
(c) O. Alévêque, S. Achelle and L. Sanguinet, ChemPhotoChem, 2023, 7, e202200201 CrossRef.
|
This journal is © The Royal Society of Chemistry 2024 |