DOI:
10.1039/D3SC01501G
(Edge Article)
Chem. Sci., 2023,
14, 9191-9196
Copper-catalyzed propargylic C–H functionalization for allene syntheses†
Received
22nd March 2023
, Accepted 8th August 2023
First published on 9th August 2023
Abstract
Allenenitriles bearing different synthetically versatile functional groups have been prepared smoothly from 5-alkynyl fluorosulfonamides in decent yields with an excellent chemo- and regio-selectivity under redox neutral conditions. The resulting allenenitriles can be readily converted to useful functionalized heterocycles. Based on mechanistic study, it is confirmed that this is the first example of radical-based non-activated propargylic C–H functionalization for allene syntheses.
Introduction
Cross coupling reactions represent one of the most straightforward strategies for the construction of complex molecules.1 As one important class of substrates, the transformations of propargylic compounds are attracting more and more interest.2 In the past few decades, catalytic couplings of propargylic alcohol derivatives with organometallic reagents have been established as an effective and very reliable way to synthesize functionalized alkynes3,4 or allenes5,6 either via a two-electron or one-electron process (Fig. 1A). However, all these methods apply propargylic alcohol derivatives with an appropriate leaving group. In contrast, direct functionalization of the inert propargylic C–H bonds would provide a straightforward and atom economic approach. In this area, preparation of alkynes has been realized via the Kharasch–Sosnovsky-Type reaction,7 intramolecular or intermolecular nitrene insertion,8 and coordination-directed deprotonation9 (Fig. 1B). Recently Liu et al. reported the benzylic C–H activation of 1-aryl-2-alkynes for arylallene syntheses.10 We envisioned a strategy of 1,5-hydrogen atom transfer11 for the generation of a propargylic radical, which would be in rapid resonance with an allenyl radical. Trapping with an appropriate reagent would afford alkynes or allenes (Fig. 1C). Such a protocol for selective allene syntheses faces three challenges: (1) the more reactive C
C bonds may cause undesired transformations such as radical cyclization that complicates the target reaction, (2) the selectivity issue of propargylic radicals vs. allenyl radicals to form either alkyne7,12 or allene products, and (3) a matched trapping reagent. Allenenitriles could be prepared though the corresponding Wittig reaction,13 SN2′-type substitution of the in situ generated propargylic phosphates,14 cross coupling of propargylic substrates,6i,15 and difunctionalization of 1,3-enynes.16 Herein, we wish to report our results on copper-catalyzed cyanation of non-activated propargylic C–H bonds, affording di- or tri-substituted allenenitriles bearing an attractive remote sulfonamide in decent yields with an excellent chemo- and regio-selectivity tolerating many synthetically versatile functionalities (Fig. 1D).
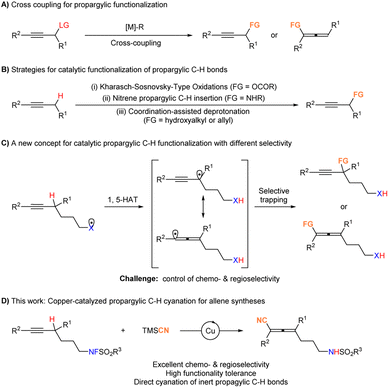 |
| Fig. 1 Catalytic functionalization of propargylic compounds. | |
Results and discussion
We began our investigation with fluorosulfonamide17,181a and TMSCN15b,16 in the presence of Cu(CH3CN)4PF6 (ref. 19) and ligand L1 in DCE at 30 °C. To our delight, 17% yield of desired allenenitrile 2a was obtained with 70% recovery of 1a (Table 1, entry 1). Then, attention was focused on the solvent effect (Table 1, entries 2–8): the reaction in CH3CN for 24 h could deliver a much better yield of 2a of 75%, with only 12% recovery of 1a. By extending the reaction time to 30 hours, 1a could be converted completely, affording 2a in 83% yield (Table 1, entry 9), and the formation of isomeric alkyne product 3a was not observed. When Cu(CH3CN)4BF4 was used instead of Cu(CH3CN)4PF6, the yield of 2a dropped to 61%, with 10% recovery of 1a. This may be attributed to the stronger coordinating ability of BF4− than that of PF6−,20 which led to a lower catalytic activity. Further screening of other copper catalysts and ligands (Table 1, entries 11–17), didn't give any better results, indicating that Cu(CH3CN4)PF6 and L1 are optimal.
Table 1 Optimization of the reaction conditionsa
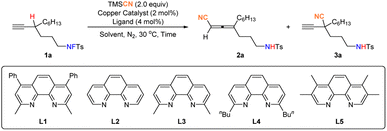
|
Entry |
Solvent |
Copper catalyst |
Ligand |
Time (h) |
Yield of 2ab (%) |
Recovery of 1ab (%) |
All reactions were run on a 0.1 mmol scale in solvent (1 mL) at 30 °C under a nitrogen atmosphere.
Determined by 1H NMR analysis with CH3NO2 as the internal standard.
|
1 |
DCE |
Cu(CH3CN)4PF6 |
L1
|
24 |
17 |
70 |
2 |
Toluene |
Cu(CH3CN)4PF6 |
L1
|
24 |
10 |
67 |
3 |
Ethyl acetate |
Cu(CH3CN)4PF6 |
L1
|
24 |
29 |
35 |
4 |
MTBE |
Cu(CH3CN)4PF6 |
L1
|
24 |
13 |
56 |
5 |
THF |
Cu(CH3CN)4PF6 |
L1
|
24 |
13 |
24 |
6 |
Dioxane |
Cu(CH3CN)4PF6 |
L1
|
24 |
38 |
17 |
7 |
DMF |
Cu(CH3CN)4PF6 |
L1
|
24 |
44 |
— |
8 |
CH3CN |
Cu(CH3CN)4PF6 |
L1
|
24 |
75 |
12 |
9 |
CH3CN |
Cu(CH3CN)4PF6 |
L1
|
30 |
83 |
— |
10 |
CH3CN |
Cu(CH3CN)4BF4 |
L1
|
36 |
61 |
10 |
11 |
CH3CN |
CuCN |
L1
|
36 |
69 |
— |
12 |
CH3CN |
CuOAc |
L1
|
36 |
66 |
— |
13 |
CH3CN |
CuTc |
L1
|
36 |
61 |
— |
14 |
CH3CN |
Cu(CH3CN)4PF6 |
L2
|
36 |
49 |
47 |
15 |
CH3CN |
Cu(CH3CN)4PF6 |
L3
|
36 |
75 |
7 |
16 |
CH3CN |
Cu(CH3CN)4PF6 |
L4
|
36 |
71 |
5 |
17 |
CH3CN |
Cu(CH3CN)4PF6 |
L5
|
36 |
3 |
67 |
With the optimized conditions in hand, we set out to explore the scope of this propargylic C–H cyanation reaction. As shown in Fig. 2, a variety of fluorosulfonamides bearing a highly sensitive terminal alkyne could be converted to trisubstituted allenes exclusively in decent yields. The R1 group may be alkyl, cycloalkyl, chloroalkyl, or methoxyalkyl, and 2a–2f were furnished in 77–83% yields. In addition, terminal olefin and differently substituted benzyl groups, such as halide atoms (F, Cl, and Br), methoxy, and trifluoromethyl may be tolerated, affording 2g–2m in 67–76% yields, and the related allyl or benzyl cyanation products were not formed, indicating a perfect chemoselectivity. Furthermore, important heteroaryl groups including thiophene and pyridine are compatible, affording heteraryl-containing products 2n and 2o in 66% and 55% yields.
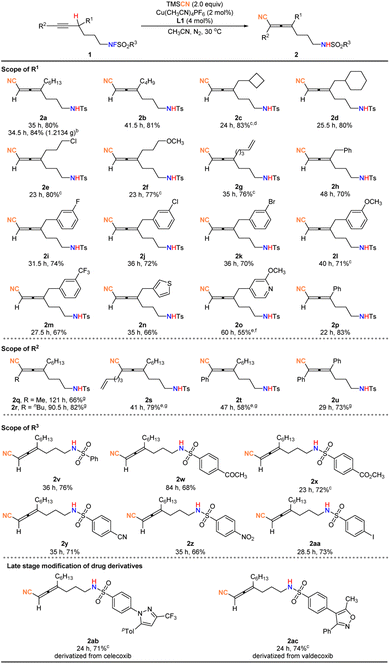 |
| Fig. 2 Scope. aReaction conditions: 1 (0.5 mmol), TMSCN (1.0 mmol), Cu(CH3CN)4PF6 (2 mol%), and L1 (4 mol%) in CH3CN (5 mL) at 30 °C. bReaction on a 4.0 mmol scale. cCu(CH3CN)4PF6 (4 mol%), L1 (8 mol%). dThe product was a mixture of 2c and its isomer 2g (2c/2g = 97/3), originated from the starting material 1c (1c/1g = 96/4). eReaction on a 0.2 mmol scale. fAdditional Cu(CH3CN)4PF6 (2 mol%) and L1 (4 mol%) were added to the reaction mixture after stirring for 36 h. gCu(CH3CN)4PF6 (10 mol%), L2 (20 mol%). | |
Compared to the alkyl-substituted substrates, the reaction of the substrate with R1 being phenyl proceeded more smoothly, affording 2p in 83% yield. The scope could be further expanded to internal alkynes to prepare tetrasubstituted allenes 2q–2u in 58–82% yields after adjusting the reaction conditions slightly. Besides, the R3 group with synthetic versatile functional groups such as acetyl, ester, cyano, nitro, and iodo groups were all intact under the optimal mild reaction conditions (2v–2aa, 66–76% yields). The cyanation reaction could be smoothly implemented for the modification of drug molecules, providing allenenitriles 2ab and 2ac in satisfactory yields. Finally, the reaction may be easily scaled up to the gram-scale (2a), demonstrating the practicality of the protocol.
Moreover, substrates 1ad, 1ae, and 1af were prepared on purpose to check the possibility of 1,4-, 1,6-, or even 1,7-HAT: the reaction of 1ad was complicated (Fig. 3A); the reaction of 1ae underwent 1,6-HAT to result in allenenitrile 2ae in 60% yield with an excellent regioselectivity (Fig. 3B); the reaction of 1af still gave the 1,5-HAT non-allene product 5 in a poor yield (Fig. 3C). Thus, 1,4-HAT and 1,7-HAT do not work while 1,6-HAT works.
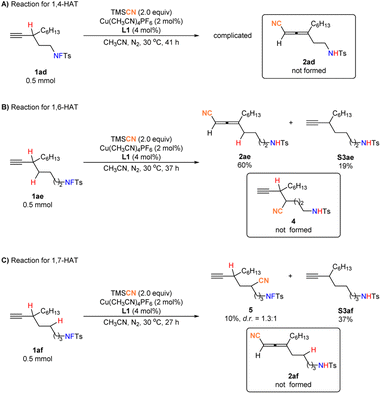 |
| Fig. 3 The reactions for 1,n-HAT (n ≠ 5). | |
Such highly functionalized allenes show great synthetic potential (Fig. 4): 2a may undergo conjugate addition exclusively under a set of mild conditions to afford nitrile 6 bearing a tetrahydropyridine in 88% yield; 2a could also be cyclized with the nitrile group being hydrolyzed to afford amide 7 in 53% yield with NaOH and H2O2; NBS promoted electrophilic bromocyclization21 of 2a would afford stereodefined brominated alkenenitrile (Z)-8 bearing a tetrahydropyrrole ring in 70% yield with an excellent stereoselectivity, which has been clearly identified by X-ray analysis. The amine functionality could also be easily modified to afford the corresponding N-tosylbenzamide 9 in an excellent yield.
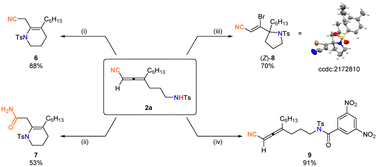 |
| Fig. 4 Transformations of products. Reaction conditions: (i) Cs2CO3 (1.0 equiv.), CH3CN, rt, 17 h. (ii) NaOH (2.0 equiv.), H2O2 (4.0 equiv.), EtOH, 80 °C, 7 h. (iii) NBS (1.2 equiv.), DCM/THF = 4/1, rt, 23 h. (iv) 3,5-Dinitrobenzoyl chloride (1.2 equiv.), DMAP (0.1 equiv.), Et3N (1.5 equiv.), rt, 4 h. | |
In addition, we found with R1 being H, both reactions of 1ag and 1ah delivered a mixture of allene 2 and alkyne 3. However, the reactions of substrates with R1 being a non-H substituent, all afforded the allenenitriles 2 exclusively (Fig. 5A). Thus, we reasoned that the steric effect of R1 is critical for the observed regioselectivity. To unveil the mechanism, a set of experiments were conducted. Initially, BHT was employed as a radical trapping reagent in the reaction of 1a: the formation of 2a was inhibited gradually with the increase of BHT (Fig. 5B), suggesting a possible radical pathway. To further confirm the existence of any radical intermediates, the reaction of 1ag was conducted under an oxygen atmosphere: the cyanation was inhibited completely, and alkynone 10 was isolated in 27% yield, which couldn't be afforded by the direct oxidation of amine S3ag, confirming the existence of propargylic radical intermediates22 (Fig. 5C). Finally, we investigated the reaction rate of 1a/1a-d to 2a by NMR monitoring (see the ESI†), showing that the primary kinetic isotope effect (KIE) was 1.5 (Fig. 5D).
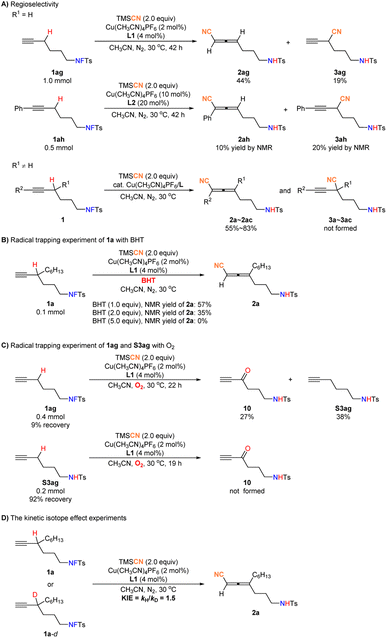 |
| Fig. 5 Regioselectivity and mechanistic studies. | |
On the basis of these experiments, a catalytic cycle involving radical intermediates has been proposed (Fig. 6): firstly, the LCuI species would reduce the N–F bond of 1 to produce the LCuIIF species and the N-centered radical Int 1. Then Int 1 undergoes a radical 1,5-HAT process to generate the resonance hybrid of propargylic/allenyl radical Int 2, and the LCuIIF species is converted to the LCuIICN species by ligand exchange with TMSCN. Subsequently, the allenyl radical, one of the resonance structures of Int 2 with less steric hindrance would be selectively trapped by the LCuIICN species to afford the allenyl LCuIIICN species Int 3. Reductive elimination would deliver the desired allene 2 and regenerate the catalytically active LCuI species.
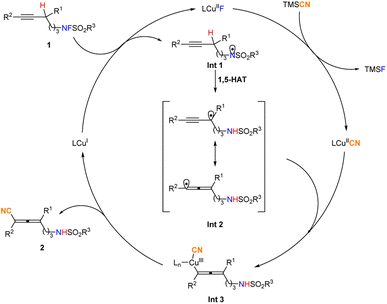 |
| Fig. 6 Proposed mechanism. | |
With this approach in hand, we also attempted the enantioselective reaction with different chiral bis(oxazoline) and pyrine-oxazoline ligands. After a series of efforts (for details see Table S6 in the ESI†), the reaction of fluorosulfonamide 1a by using (S,S)-L15* gave (−)-2a in 79% yield and −36% ee. Furthermore, non-terminal alkyne 1r was also subjected to such conditions, which delivered (+)-2r in 33% yield and −23% ee (Fig. 7).
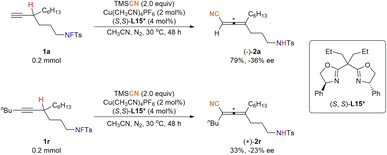 |
| Fig. 7 Preliminary results of catalytic asymmetric propargylic C–H cyanation. | |
In summary, we have developed the first example of 1,5-HAT-based propargylic C–H activation, providing a highly chemo- and regioselective approach for the construction of allenenitriles, featuring great functionality compatibility. The resulting products may be readily converted to different functionalized heterocycles. Mechanistic studies support the catalytic cycle of CuI/CuIII involving a propargylic radical and allenyl radical. Further studies on other types of 1,5-HAT based propargylic C–H functionalization for allene syntheses and further investigations on the asymmetric version of this propargylic C–H cyanation are ongoing in our laboratory.
Data availability
All detailed procedures, characterization data, and spectra are available in the ESI.†
Author contributions
S. M., X. W. and D. Z. designed the experiments. D. Z., J. F., Y. S and Y. H performed the experiments. D. Z. and S. M. wrote the manuscript. D. Z., C. F. and S. M. checked the experimental data.
Conflicts of interest
The authors declare no competing interests.
Acknowledgements
Financial support from the National Key R&D Program of China (2021YFA1500100) is greatly appreciated. Shengming Ma is a Qiu Shi Adjunct Professor at Zhejiang University. We also thank Qian Zhang in our group for reproducing the results of 2c, 2j, and 2r presented in Fig. 2.
Notes and references
-
(a) M. Kumada, Pure Appl. Chem., 1980, 52, 669 CrossRef CAS;
(b) N. Miyaura and A. Suzuki, Chem. Rev., 1995, 95, 2457 CrossRef CAS;
(c) R. Chinchilla and C. Nájera, Chem. Rev., 2007, 107, 874 CrossRef CAS PubMed;
(d) A. Suzuki, Angew. Chem., Int. Ed., 2011, 50, 6722 CrossRef CAS PubMed;
(e) R. Jana, T. P. Pathak and M. S. Sigman, Chem. Rev., 2011, 111, 1417 CrossRef CAS PubMed;
(f) C. Liu, H. Zhang, W. Shi and A. Lei, Chem. Rev., 2011, 111, 1780 CrossRef CAS PubMed;
(g) P. Ruiz-Castillo and S. L. Buchwald, Chem. Rev., 2016, 116, 12564 CrossRef CAS PubMed;
(h) A. Biffis, P. Centomo, A. Del Zotto and M. Zecca, Chem. Rev., 2018, 118, 2249 CrossRef CAS PubMed;
(i) Q. Yang, Y. Zhao and D. Ma, Org. Process Res. Dev., 2022, 26, 1690 CrossRef CAS.
-
(a) J. Tsuji and T. Mandai, Angew. Chem., Int. Ed. Engl., 1995, 34, 2589 CrossRef CAS;
(b) S. Ma, Eur. J. Org. Chem., 2004, 2004, 1175 CrossRef;
(c) C.-H. Ding and X.-L. Hou, Chem. Rev., 2011, 111, 1914 CrossRef CAS PubMed;
(d) L. Guo, X. Duan and Y. Liang, Acc. Chem. Res., 2011, 44, 111 CrossRef CAS;
(e) X.-Z. Shu, D. Shu, C. M. Schienebeck and W. Tang, Chem. Soc. Rev., 2012, 41, 7698 RSC;
(f) D.-Y. Zhang and X.-P. Hu, Tetrahedron Lett., 2015, 56, 283 CrossRef CAS;
(g) R. Roy and S. Saha, RSC Adv., 2018, 8, 31129 RSC;
(h) C. Q. O'Broin and P. J. Guiry, J. Org. Chem., 2020, 85, 10321 CrossRef PubMed;
(i) X.-R. Song, R. Yang and Q. Xiao, Adv. Synth. Catal., 2021, 363, 852 CrossRef CAS.
- For selected reviews, see:
(a) N. Ljungdahl and N. Kann, Angew. Chem., Int. Ed., 2009, 48, 642 CrossRef CAS PubMed;
(b) Y. Miyake, S. Uemura and Y. Nishibayashi, ChemCatChem, 2009, 1, 342 CrossRef CAS;
(c) Y. Nishibayashi, Synthesis, 2012, 44, 489 CrossRef CAS;
(d) H. Tsuji and M. Kawatsura, Asian J. Org. Chem., 2020, 9, 1924 CrossRef CAS.
- For recent examples, see:
(a) A. J. Oelke, J. Sun and G. C. Fu, J. Am. Chem. Soc., 2012, 134, 2966 CrossRef CAS PubMed;
(b) X. Huang, S. Wu, W. Wu, P. Li, C. Fu and S. Ma, Nat. Commun., 2016, 7, 12382 CrossRef PubMed;
(c) K. Zhang, L.-Q. Lu, S. Yao, J.-R. Chen, D.-Q. Shi and W.-J. Xiao, J. Am. Chem. Soc., 2017, 139, 12847 CrossRef CAS PubMed;
(d) A. Shemet and E. M. Carreira, Org. Lett., 2017, 19, 5529 CrossRef CAS PubMed;
(e) K. Watanabe, Y. Miyazaki, M. Okubo, B. Zhou, H. Tsuji and M. Kawatsura, Org. Lett., 2018, 20, 5448 CrossRef CAS PubMed;
(f) F.-D. Lu, D. Liu, L. Zhu, L.-Q. Lu, Q. Yang, Q.-Q. Zhou, Y. Wei, Y. Lan and W.-J. Xiao, J. Am. Chem. Soc., 2019, 141, 6167 CrossRef CAS PubMed;
(g) Z.-J. Zhang, L. Zhang, R.-L. Geng, J. Song, X.-H. Chen and L.-Z. Gong, Angew. Chem., Int. Ed., 2019, 58, 12190 CrossRef CAS PubMed;
(h) L. Peng, Z. He, X. Xu and C. Guo, Angew. Chem., Int. Ed., 2020, 59, 14270 CrossRef CAS PubMed;
(i) S. Liu, Y. Tanabe, S. Kuriyama and Y. Nishibayashi, Angew. Chem., Int. Ed., 2021, 60, 11231 CrossRef CAS.
- For a review, see: S. Du, A.-X. Zhou, R. Yang, X.-R. Song and Q. Xiao, Org. Chem. Front., 2021, 8, 6760 RSC.
- For selected recent examples, see:
(a) Y. Wang, W. Zhang and S. Ma, J. Am. Chem. Soc., 2013, 135, 11517 CrossRef CAS;
(b) S. Wu, X. Huang, W. Wu, P. Li, C. Fu and S. Ma, Nat. Commun., 2015, 6, 7946 CrossRef PubMed;
(c) S. N. Kessler and J.-E. Bäckvall, Angew. Chem., Int. Ed., 2016, 55, 3734 CrossRef CAS PubMed;
(d) J. Ruchti and E. M. Carreira, Org. Lett., 2016, 18, 2174 CrossRef CAS PubMed;
(e) Q. Lu, S. Greßies, F. J. R. Klauck and F. Glorius, Angew. Chem., Int. Ed., 2017, 56, 6660 CrossRef CAS PubMed;
(f) I. Scheipers, C. Mück-Lichtenfeld and A. Studer, Angew. Chem., Int. Ed., 2019, 58, 6545 CrossRef CAS PubMed;
(g) W.-F. Zheng, W. Zhang, C. Huang, P. Wu, H. Qian, L. Wang, Y.-L. Guo and S. Ma, Nat. Catal., 2019, 2, 997 CrossRef CAS;
(h) H. Wang, H. Luo, Z.-M. Zhang, W.-F. Zheng, Y. Yin, H. Qian, J. Zhang and S. Ma, J. Am. Chem. Soc., 2020, 142, 9763 CrossRef CAS PubMed;
(i) Q. Liu, J. Zheng, X. Zhang and S. Ma, Nat. Commun., 2022, 13, 3302 CrossRef CAS PubMed;
(j) H. Wang, H. Qian, J. Zhang and S. Ma, J. Am. Chem. Soc., 2022, 144, 12619 CrossRef CAS PubMed.
-
(a) H. Kropf, R. Schröder and R. Fölsing, Synthesis, 1977, 894 CrossRef CAS;
(b) L. X. Alvarez, M. L. Christ and A. B. Sorokin, Appl. Catal., A, 2007, 325, 303 CrossRef CAS.
-
(a) R. D. Grigg, J. W. Rigoli, S. D. Pearce and J. M. Schomaker, Org. Lett., 2012, 14, 280 CrossRef CAS PubMed;
(b) H. Lu, C. Li, H. Jiang, C. L. Lizardi and X. P. Zhang, Angew. Chem., Int. Ed., 2014, 53, 7028 CrossRef CAS PubMed;
(c) M. Ju, E. E. Zerull, J. M. Roberts, M. Huang, I. A. Guzei and J. M. Schomaker, J. Am. Chem. Soc., 2020, 142, 12930 CrossRef CAS PubMed;
(d) Z. Liu, Z.-Y. Qin, L. Zhu, S. V. Athavale, A. Sengupta, Z.-J. Jia, M. Garcia-Borràs, K. N. Houk and F. H. Arnold, J. Am. Chem. Soc., 2022, 144, 80 CrossRef CAS PubMed.
-
(a) T. Li and L. Zhang, J. Am. Chem. Soc., 2018, 140, 17439 CrossRef CAS PubMed;
(b) Y. Wang, J. Zhu, A. C. Durham, H. Lindberg and Y.-M. Wang, J. Am. Chem. Soc., 2019, 141, 19594 CrossRef CAS PubMed;
(c) J. Zhu, Y. Wang, A. D. Charlack and Y.-M. Wang, J. Am. Chem. Soc., 2022, 144, 15480 CrossRef CAS PubMed.
- R. Lu, T. Yang, X. Chen, W. Fan, P. Chen, Z. Lin and G. Liu, J. Am. Chem. Soc., 2021, 143, 14451 CrossRef CAS PubMed.
-
J. J. Li, in Name Reactions, Springer, Cham, 2021, pp. 259–261 Search PubMed.
- Y. Deng, R. Lu, P. Chen and G. Liu, Chem. Commun., 2023, 59, 4656 RSC.
- For selected reports, see:
(a) Y. Kita, Y. Tsuzuki, S. Kitagaki and S. Akai, Chem. Pharm. Bull., 1994, 42, 233 CrossRef CAS;
(b) Y.-F. Ao, D.-X. Wang, L. Zhao and M.-X. Wang, J. Org. Chem., 2014, 79, 3103 CrossRef CAS PubMed.
- For a selected report, see: R. Yoneda, N. Inagaki, S. Harusawa and T. Kurihara, Chem. Pharm. Bull., 1992, 40, 21 CrossRef CAS.
- For selected reports, see:
(a) Y. Tsuji, M. Taniguchi, T. Yasuda, T. Kawamura and Y. Obora, Org. Lett., 2000, 2, 2635 CrossRef CAS PubMed;
(b) F.-D. Lu, Z.-C. Shu, G.-F. He, J.-C. Bai, L.-Q. Lu and W.-J. Xiao, Org. Chem. Front., 2022, 9, 5259 RSC.
- For selected reports, see:
(a) F. Wang, D. Wang, Y. Zhou, L. Liang, R. Lu, P. Chen, Z. Lin and G. Liu, Angew. Chem., Int. Ed., 2018, 57, 7140 CrossRef CAS PubMed;
(b) X. Zhu, W. Deng, M.-F. Chiou, C. Ye, W. Jian, Y. Zeng, Y. Jiao, L. Ge, Y. Li, X. Zhang and H. Bao, J. Am. Chem. Soc., 2019, 141, 548 CrossRef CAS PubMed;
(c) Y. Zeng, M.-F. Chiou, X. Zhu, J. Cao, D. Lv, W. Jian, Y. Li, X. Zhang and H. Bao, J. Am. Chem. Soc., 2020, 142, 18014 CrossRef CAS PubMed;
(d) Y. Song, C. Fu and S. Ma, ACS Catal., 2021, 11, 10007 CrossRef CAS;
(e) Y. Zhao, J.-L. Wang, Z. Zhang, X.-S. Li, Z.-J. Niu and X.-Y. Liu, J. Org. Chem., 2021, 86, 18056 CrossRef CAS PubMed;
(f) Y. Chen, J. Wang and Y. Lu, Chem. Sci., 2021, 12, 11316 RSC.
- For a seminal report using fluorosulfonamide, see: B. J. Groendyke, D. I. AbuSalim and S. P. Cook, J. Am. Chem. Soc., 2016, 138, 12771 CrossRef CAS PubMed.
- The substrates used in this study are prepared according to the following scheme:
.
-
(a) Z. Li, Q. Wang and J. Zhu, Angew. Chem., Int. Ed., 2018, 57, 13288 CrossRef CAS PubMed;
(b) H. Zhang, Y. Zhou, P. Tian and C. Jiang, Org. Lett., 2019, 21, 1921 CrossRef CAS PubMed;
(c) Z. Yin, Y. Zhang, S. Zhang and X.-F. Wu, Adv. Synth. Catal., 2019, 361, 5478 CrossRef CAS;
(d) Z. Zhang, L. M. Stateman and D. A. Nagib, Chem. Sci., 2019, 10, 1207 RSC;
(e) A. Modak, E. N. Pinter and P. S. Cook, J. Am. Chem. Soc., 2019, 141, 18405 CrossRef CAS PubMed;
(f) Z. Liu, H. Xiao, B. Zhang, H. Shen, L. Zhu and C. Li, Angew. Chem., Int. Ed., 2019, 58, 2510 CrossRef CAS;
(g) Z.-H. Zhang, X.-Y. Dong, X.-Y. Du, Q.-S. Gu, Z.-L. Li and X.-Y. Liu, Nat. Commun., 2019, 10, 5689 CrossRef PubMed;
(h) S. Shi, P. Zhang, C. Luo, S. Zhuo, Y. Zhang, G. Tang and Y. Zhao, Org. Lett., 2020, 22, 1760 CrossRef CAS PubMed;
(i) Y.-C. Wang, Z.-Y. Qin, Y.-L. Huang, Y.-M. Hou, R.-X. Jin, C. Li and X.-S. Wang, Org. Lett., 2020, 22, 4006 CrossRef PubMed;
(j) Q.-Q. Min, J.-W. Yang, M.-J. Pang, G.-Z. Ao and F. Liu, Org. Chem. Front., 2021, 8, 249 RSC.
- R. Díaz-Torres and S. Álvarez, Dalton Trans., 2011, 40, 10742 RSC.
-
(a) Z. Shi, Z. Yu and S. Ma, Tetrahedron, 1999, 55, 12137 CrossRef;
(b) C. Jonasson, A. Horváth and J. E. Bäckvall, J. Am. Chem. Soc., 2000, 122, 9600 CrossRef CAS;
(c) N. Wang, B. Chen and S. Ma, Adv. Synth. Catal., 2014, 356, 485 CrossRef CAS.
-
(a) C. Pérollier and A. B. Sorokin, Chem. Commun., 2002, 2002, 1548 RSC;
(b) C. C. Black and A. E. V. Gorden, Tetrahedron Lett., 2018, 59, 803 CrossRef CAS.
Footnotes |
† Electronic supplementary information (ESI) available. CCDC 2172810. For ESI and crystallographic data in CIF or other electronic format see DOI: https://doi.org/10.1039/d3sc01501g |
‡ These authors contributed equally: Junjie Fan, Yaqi Shi, and Yankai Huang. |
|
This journal is © The Royal Society of Chemistry 2023 |
Click here to see how this site uses Cookies. View our privacy policy here.