DOI:
10.1039/D3RA06582K
(Paper)
RSC Adv., 2023,
13, 33167-33174
Copper-promoted S-arylation reactions with triarylbismuths for the synthesis of diaryl sulfides†
Received
27th September 2023
, Accepted 6th November 2023
First published on 10th November 2023
Abstract
A simple approach for copper-promoted S-arylation reactions utilizing triarylbismuths or triarylantimonys as arylating reagents has been described. These reactions can be performed under mild conditions and exhibit remarkable functional group tolerance and chemoselectivity. The corresponding 2-arylthiopyridine 1-oxide derivatives and arylthioanilines/phenols have been successfully synthesized, achieving good to excellent yields across over 49 examples.
1. Introduction
Sulfur containing compounds are common and useful motifs in numerous natural products, functional materials, and other biologically active molecules, and also serve as versatile intermediates in organic synthesis.1,2 Especially in the pharmaceutical industry, the impact of organosulfur compounds is extraordinary (Scheme 1). A recent survey of pharmaceuticals revealed that more than 20% of the top 200 brand name drugs, as measured by U.S. retail sales and total U.S. prescriptions in 2011, contain organosulfur structures.3 Therefore, developing simple, efficient and practical methods to construct these molecules attracted considerable attention. Over the past three decades, many successful tools for ligand-assisted Cu-catalyzed Ullmann-type C–S cross-coupling reactions have been established for the preparation of organosulfur molecules, which have been well-reviewed by several groups.4–9 However, compared with C–N and C–O coupling reactions, the Cu-catalyzed C–S bond formations are less studied, partially due to the strong coordinating and adsorptive properties of sulfur-containing compounds which lead to catalyst poisoning and render the catalytic reactions ineffective.4 In addition, the thiols used as the sulfur source generally have unpleasant smell and are easy to be oxidized during the whole process.10 Much effort is then still needed to further improve the efficiency and generality of copper-based S-arylation reactions.
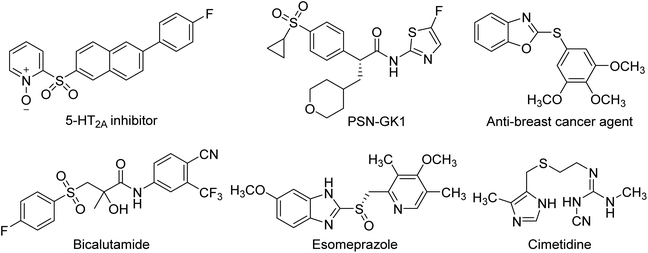 |
| Scheme 1 Some representative S-containing scaffolds in biologically active molecules. | |
Organobismuth compounds, a class of organometallic reagents, exhibit several important applications in the field of medicinal chemistry, material science and organic synthesis, attributable to their facile preparation, low or non-toxic, chemical stability and high reaction activity.11–13 Among these, trivalent organobismuths such as triarylbismuths (Ar3Bi) have proved to be a kind of effective alternative arylating agents, supplanting the frequently used aryl halides and arylboronic acids, in copper-mediated C–S cross-coupling reactions.14–17 Recently, our group reported that copper pyrithione (bis(1-hydroxy-1H-pyridine-2-thionato-O,S)copper, CuPT) could act as both the coupling partner and copper center for C–S coupling reactions with aryl iodides and bromides as the arylating reagents. In this process, CuPT could be S-arylated smoothly under conventional heating (80 °C for ArI and 100 °C for ArBr) or microwave irradiation (110 °C for ArI and 130 °C for ArBr) with high yields and excellent functional group tolerance.18 On the other side, we also found that Ar3Bi could serve as arylating reagents in Cu-promoted N-arylation of secondary and some heteroaryl and aliphatic primary amines with high efficiency.19 Based on these, we reasonably considered whether Ar3Bi instead of arylhalides could be more efficient for the C–S coupling reaction with CuPT as the green sulfur source. Therefore, we herein wish to report our results on the employment of Ar3Bi as mild and efficient arylating reagents in Cu-based C–S coupling reactions for the synthesis of 2-arylthiopyridine 1-oxide derivatives and arylthioanilines/phenols with over 49 examples (Scheme 2).
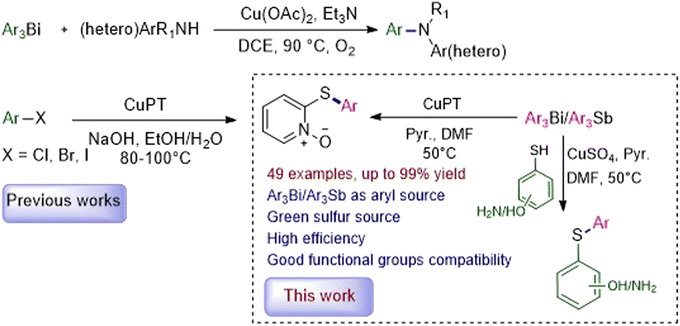 |
| Scheme 2 Previous works and our work for the S-arylatin with Ar3Bi or Ar3Sb. | |
2. Experimental
2.1 General methods
Unless otherwise stated, all reagents were purchased from commercial suppliers (such as Adamas-beta and Energy Chemical) and were used without further purification. Column chromatography and thin-layer chromatography were performed with silica gel (200–300 mesh) and GF254 plates purchased from Qingdao Haiyang Chemical Co. Ltd. 1H NMR and 13C NMR were recorded on a Bruker Avance III HD 400 instrument using TMS as the internal standard and DMSO-d6 or CDCl3 as the solvent. High-resolution mass spectrum (HRMS) was recorded on a Thermo Scientific LTQ Orbitrap XL instrument under the ESI or APCI ion source.
2.2 Ar3Bi or Ar3Sb used in this work
Ph3Bi and Ph3Sb were purchased from Adamas-beta and used without further purification. Other triarylbismuths and triarylantimonys were synthesized according to the reported methods.19–21
2.3 General procedure for the reaction between CuPT and Ar3Bi or Ar3Sb
To a 25 mL Schlenk tube was added CuPT (0.30 mmol), Ar3Bi or Ar3Sb (0.30 or 0.60 mmol), pyridine (0.30 mmol) and DMF (1 mL). The mixture was stirred at 50 or 70 °C for 12 hours under air. After cooling to room temperature, saturated brine was added and the mixture was extracted with ethyl acetate (3 × 10 mL). The organic phase was collected, dried over anhydrous Na2SO4, filtered, and concentrated under reduced pressure. The residue was purified by flash column chromatograph on silica gel (petroleum ether
:
ethyl acetate = 1
:
1 as the eluent) to afford the target products 3a–3q in Table 2.
2.4 General procedure for the reaction between NaPT and Ar3Bi or Ar3Sb
To a 25 mL Schlenk tube was added 2-mercaptopyridine N-oxide sodium (NaPT) salt (0.30 mmol), Ar3Bi or Ar3Sb (0.30 or 0.60 mmol), CuSO4·5H2O (0.15 mmol), pyridine (0.30 mmol) and DMF (1 mL). The reaction was carried out under the same conditions as the synthesis for the reaction between CuPT and Ar3Bi or Ar3Sb as mentioned before to afford the target products 3a–3j, 3l and 3m in Table 4.
2.5 General procedure for the reaction between Ar3Bi with aminobenzenethiols or mercaptophenols
To a 25 mL Schlenk tube was added aminobenzenethiol or mercaptophenol (0.30 mmol), Ar3Bi (0.30 mmol), Cu(OAc)2 (0.30 mmol), pyridine (0.30 mmol) and DMF (1 mL). The mixture was stirred at 50 °C for 12 hours. After cooling to room temperature, saturated brine was added and the mixture was extracted with ethyl acetate (3 × 10 mL). The organic phase was collected, dried over anhydrous Na2SO4, filtered and concentrated under reduced pressure. The residue was purified by flash column chromatograph on silica gel (petroleum ether
:
ethyl acetate = 20
:
1 or 5
:
1 as the eluent) to afford the target products 6a–6f and 7a–7g in Table 5.
3. Results and discussion
As shown in Table 1, the reaction conditions were initially explored using CuPT (1) and Ph3Bi (2a) as the model substrates. Being a bispyrithione–copper(II) complex of CuPT, 2 equiv. of Ph3Bi relative to CuPT was firstly used as the arylating reagent and without the addition of any other copper source for this transformation. Screening the solvent indicated that DMF was the best one and exhibited an excellent yield (99%) of the desired product 3a (entries 1–5). Among various bases examined, organic bases, such as pyridine, Et3N and DIPEA demonstrated higher yields than inorganic bases, but decreasing the amount led to inferior results (entries 6–13). Interestingly, we were pleased to find that reducing the Ph3Bi amount to 1.0 equiv. did not decrease the efficiency and gave the coupling product in 97% yield (entry 14). This result indicated that all the phenyl groups could be transferred from Ph3Bi in the reaction; however, further reducing the amount resulted in an obviously decreased yield (entry 15). Lower results were observed when dropping the reaction temperature or shortening the reaction time (entries 16–19). Finally, in the C–S coupling reaction between CuPT and Ph3Bi, a yield of 96% was gained when 1.0 equiv. Ph3Bi as well as 1.0 equiv. of pyridine were used in DMF at 50 °C for 12 h in the open air (entry 18), which was chosen as the optimal conditions.
Table 1 Optimization of the reaction conditions between CuPT and Ph3Bia

|
Entry |
Solvent |
Base (equiv.) |
Temp. (°C) |
Time (h) |
Yieldb (%) |
Reaction conditions: 1 (0.3 mmol), 2a (0.6 mmol, 2.0 equiv.), base, solvent (1 mL), open air. Isolated yield (based on CuPT with double pyrthione). 2a (0.3 mmol, 1.0 equiv.). 2a (0.2 mmol, 0.67 equiv.). |
1 |
CH2Cl2 |
Pyridine (2.0) |
50 |
20 |
32 |
2 |
MeCN |
Pyridine (2.0) |
50 |
20 |
93 |
3 |
DMSO |
Pyridine (2.0) |
50 |
20 |
98 |
4 |
DMF |
Pyridine (2.0) |
50 |
20 |
99 |
5 |
Toluene |
Pyridine (2.0) |
50 |
20 |
54 |
6 |
DMF |
K2CO3 (2.0) |
50 |
20 |
58 |
7 |
DMF |
Cs2CO3 (2.0) |
50 |
20 |
35 |
8 |
DMF |
K3PO4 (2.0) |
50 |
20 |
44 |
9 |
DMF |
Et3N (2.0) |
50 |
20 |
98 |
10 |
DMF |
KOH (2.0) |
50 |
20 |
75 |
11 |
DMF |
DIPEA (2.0) |
50 |
20 |
95 |
12 |
DMF |
Pyridine (0.5) |
50 |
20 |
82 |
13 |
DMF |
Pyridine (1.0) |
50 |
20 |
93 |
14 |
DMF |
Pyridine (1.0) |
50 |
20 |
97c |
15 |
DMF |
Pyridine (1.0) |
50 |
20 |
73d |
16 |
DMF |
Pyridine (1.0) |
40 |
20 |
94c |
17 |
DMF |
Pyridine (1.0) |
r.t. |
20 |
71c |
18 |
DMF |
Pyridine (1.0) |
50 |
12 |
96c |
19 |
DMF |
Pyridine (1.0) |
50 |
7 |
46c |
With the optimal conditions in hand, the substrate scopes of Ar3Bi were subsequently investigated. As indicated in Table 2, a wide range of diverse triarylbismuths could be coupled with CuPT efficiently, giving the corresponding 2-arylthiopyridine 1-oxide derivatives with high yields in general (3a–3q), which needed to increase the reaction temperature and/or the loading of triarylbismuths for some cases. It is clear that the electronic factor has only a slight impact on the efficiency, as triarylbismuths bearing electron-donating, electron-withdrawing, or electron-neutral groups at the para-, meta-, or ortho- positions of the aromatic ring performed well under the optimal conditions. Notably, sterically hindered substrates could be converted without any difficulty in the protocol, including 2-methyl, 2,4-dimethyl, 2-methoxy, and 1-naphthyl substituted compounds (3b, 3e, 3f and 3q). In view of hypervalent organoantimony compounds previously used as aryl donors in some coupling reactions,22,23 we explored the feasibility of Ar3Sb in the current reaction. Fortunately, it is notable that Ar3Sb could act as an efficient arylating partner for the C–S crossing coupling as well, affording the desired products in 93–99% yields under the optimal reaction conditions (3a, 3c and 3l).
Table 2 Scope for the coupling reactions between CuPT and Ar3Bi or Ar3Sba
Reaction conditions: 1 (0.3 mmol), 2 (0.3 mmol, 1.0 equiv.), pyridine (0.3 mmol, 1.0 equiv.), DMF (1 mL), 50 °C, 12 h, open air; isolated yield (based on CuPT with double pyrthione). Reaction at 70 °C. Ar3Bi (0.6 mmol, 2.0 equiv.). Yield for Ar3Sb (0.6 mmol, 2 equiv.). |
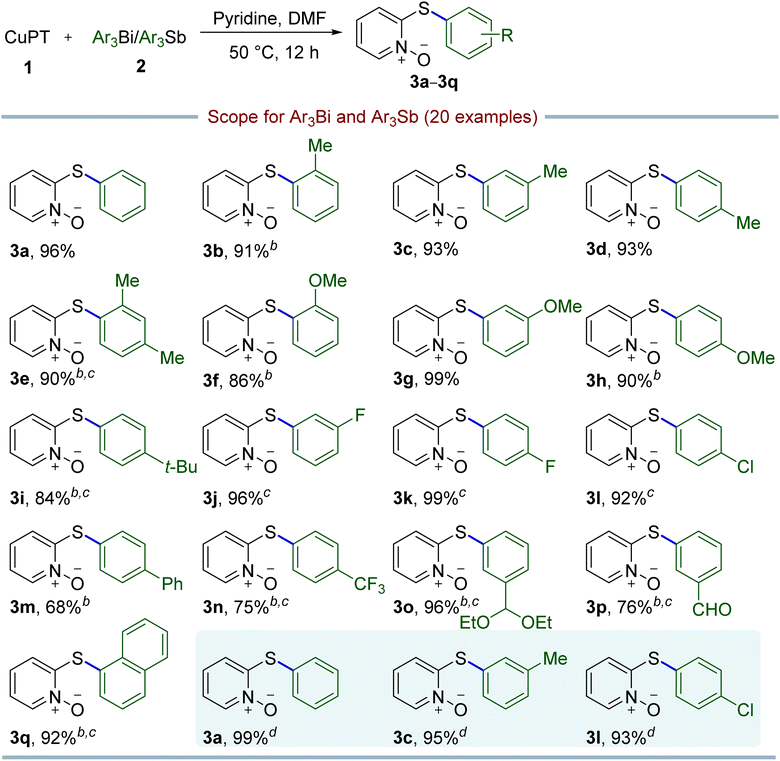 |
Considering that the CuPT complex can be easily synthesized from NaPT and CuSO4 in alkali solution, a mixture of NaPT and external copper source was then tested as an alternative to CuPT for both the catalyst and coupling partner in the reaction. As shown in Table 3, after evaluating the effects of the copper sources and base, it could be seen that the optimal conditions employed 0.5 equiv. of CuSO4·5H2O, 1.0 equiv. of Ph3Bi and 0.5 equiv. of pyridine relative to the amount of NaPT in DMF at 50 °C for 12 h under ambient conditions, generating the 95% yield of the coupling product 3a (entry 11). As summarized in Table 4, triarylbismuths with a variety of functional groups have also been successfully applied to the S-arylation of NaPT and exhibited a broad substrate scope and compatibility, giving the similar high yields with CuPT. Moreover, the Ar3Sb compounds have also been demonstrated to be suitable for these coupling reactions with 70–96% yields (3a, 3c, 3j and 3l).
Table 3 Optimization of the copper-promoted coupling reaction between NaPT and Ph3Bia

|
Entry |
[Cu] (equiv.) |
Base (equiv.) |
Yieldb (%) |
Reaction condition: 5 (0.3 mmol), Ph3Bi (0.3 mmol), pyridine, DMF (1 mL), 50 °C, 12 h, open air. Isolated yields. In a sealed tube. |
1 |
Cu(OAc)2 (1.0) |
Pyridine (1.0) |
66 |
2 |
CuBr2 (1.0) |
Pyridine (1.0) |
90 |
3 |
Cu (1.0) |
Pyridine (1.0) |
64 |
4 |
CuO (1.0) |
Pyridine (1.0) |
Trace |
5 |
CuSO4 (1.0) |
Pyridine (1.0) |
Trace |
6 |
CuSO4·5H2O (1.0) |
Pyridine (1.0) |
91 |
7 |
CuSO4·5H2O (1.0) |
Pyridine (1.0) |
56c |
8 |
— |
Pyridine (1.0) |
NR |
9 |
CuSO4·5H2O (0.5) |
Pyridine (1.0) |
95 |
10 |
CuSO4·5H2O (0.25) |
Pyridine (1.0) |
61 |
11 |
CuSO4·5H2O (0.5) |
Pyridine (0.5) |
95 |
Table 4 Scope for the coupling reaction between NaPT and Ar3Bi or Ar3Sba
Reaction conditions: 5 (0.3 mmol), Ar3Bi (0.3 mmol, 1.0 equiv.), CuSO4·5H2O (0.5 equiv.), pyridine (0.5 equiv.), DMF (1 mL), 50 °C, 12 h, open air; isolated yield. Reaction at 70 °C. Yield for Ar3Sb (0.6 mmol, 2 equiv.). |
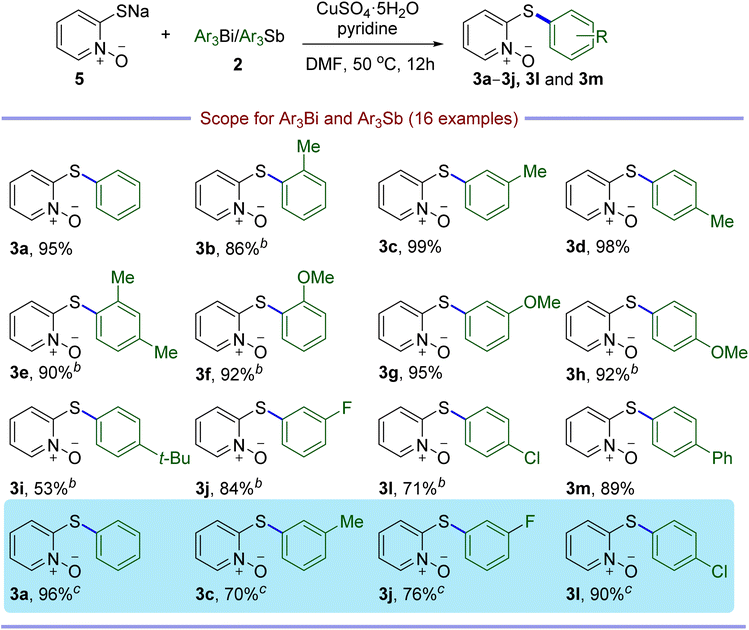 |
Having established the method for S-arylation of CuPT or NaPT with triarylbismuths or triarylantimonys, the possibilities of chemoselective arylation of aminobenzenethiols or mercaptophenols were evaluated under the same reaction conditions, which was listed in Table 5. Similar to the S-arylation of NaPT, the present protocol tolerates various functional groups in the substrate along with high levels of chemoselectivity (chemoselectivity order S > N > O).24 The –OH or –NH2 groups bearing at ortho-, meta- or para- position of aminobenzenethiol or mercaptophenol all did not influence the activity under the optimized conditions, further showing the non-obvious steric effect of the present catalytic system.
Table 5 Selective C–S coupling of Ar3Bi with aminobenzenethiols or mercaptophenolsa
Reaction conditions: aminobenzenethiols or mercaptophenols (0.3 mmol), Ar3Bi (0.3 mmol, 1.0 equiv.), Cu(OAc)2 (0.3 mmol, 1.0 equiv.), pyridine (0.3 mmol, 1.0 equiv.), DMF (1 mL), 50 °C, 12 h, sealed tube; isolated yield. |
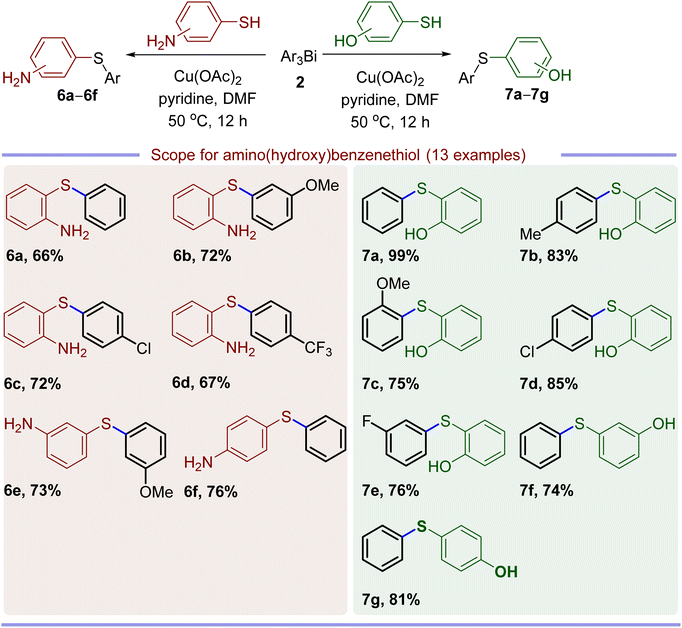 |
On the basis of the experiment results and the related reports,11,15 a plausible reaction mechanism for the Cu-promoted S-arylation reactions with triarylbismuth reagents under basic condition was illustrated in Scheme 3. The catalytic process was similar to that of the Cu-promoted N-arylation of secondary and primary amines with triarylbismuths that we reported very recently.19 The first step of the reaction would be the formation of a copper(III) intermediate I from Ar3Bi, Cu(OAc)2 and the S-containing nucleophiles (CuPT, NaPT and ArSH), through the process of disproportionation of copper(II) species, transmetallation of aryl moiety from the bismuth to copper center, and deprotonation of the S-nucleophiles by pyridine. Then, the reductive elimination of I gave the S-arylated products and copper(I) acetate.
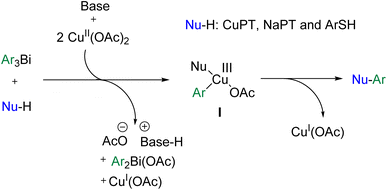 |
| Scheme 3 Proposed mechanism for Cu-promoted S-arylation reactions with Ar3Bi. | |
4. Conclusions
In summary, an efficient approach has been developed for copper-promoted C–S crossing coupling reactions by using triarylbismuth and antimony reagents under mild conditions. Applying this method, the S-arylation of CuPT or NaPT proceeded effectively and displayed good functional group compatibility in the presence of a variety of triarylbismuth or antimony reagents, giving the corresponding 2-arylthiopyridine 1-oxide derivatives in good to excellent yields. Moreover, the reaction shows effective chemoselectivity for S-arylation of aminobenzenethiols or mercaptophenols. This protocol provides a new tool for the synthesis of C–S coupling products with green sulfur sources and Ar3Bi/Ar3Sb as arylating reagents. Further applications of triarylbismuths and triarylantimonys with other coupling partners are in progress.
Author contributions
Mei Nie and Xuehao Zhou: conceptualization, data curation, investigation, writing – original draft. Jingjie Tang: funding acquisition, writing – review & editing. Dongting Huang: analysis, writing – review & editing. Xinsheng Xiao and Jianwei Xie: funding acquisition, resources, supervision, writing – review & editing.
Conflicts of interest
There are no conflicts to declare.
Acknowledgements
We gratefully acknowledge the financial support of this work from the Natural Science Foundation of Hunan Province (2021JJ30290 and 2021JJ302289), Guangzhou Science and Technology Plan Projects (2023A04J1844) and the construct program of applied characteristic discipline in Hunan University of Science and Engineering.
References
- M. Feng, B. Tang, S. H. Liang and X. Jiang, Curr. Top. Med. Chem., 2016, 16, 1200–1216 CrossRef CAS PubMed.
- N. Wang, P. Saidhareddy and X. Jiang, Nat. Prod. Rep., 2020, 37, 246–275 RSC.
- E. A. Ilardi, E. Vitaku and J. T. Njardarson, J. Med. Chem., 2014, 57, 2832–2842 CrossRef CAS PubMed.
- T. Kondo and T.-a. Mitsudo, Chem. Rev., 2000, 100, 3205–3220 CrossRef CAS PubMed.
- K. Kunz, U. Scholz and D. Ganzer, Synlett, 2003, 2428–2439 CrossRef CAS.
- S. V. Ley and A. W. Thomas, Angew. Chem., Int. Ed., 2003, 42, 5400–5449 CrossRef CAS PubMed.
- I. P. Beletskaya and A. V. Cheprakov, Coord. Chem. Rev., 2004, 248, 2337–2364 CrossRef CAS.
- S. Bhunia, G. G. Pawar, S. V. Kumar, Y. Jiang and D. Ma, Angew. Chem., Int. Ed., 2017, 56, 16136–16179 CrossRef CAS PubMed.
- Q. Cai and W. Zhou, Chin. J. Chem., 2020, 38, 879–893 CrossRef CAS.
- Z. Qiao, H. Liu, X. Xiao, Y. Fu, J. Wei, Y. Li and X. Jiang, Org. Lett., 2013, 15, 2594–2597 CrossRef CAS PubMed.
- A. Gagnon, J. Dansereau and A. Le Roch, Synthesis, 2017, 49, 1707–1745 CrossRef CAS.
- S. Condon, C. Pichon and M. Davi, Org. Prep. Proced. Int., 2014, 46, 89–131 CrossRef CAS.
- J. Luan, L. Zhang and Z. Hu, Molecules, 2011, 16, 4191–4230 CrossRef CAS.
- S. Yasuike, M. Nishioka, N. Kakusawa and J. Kurita, Tetrahedron Lett., 2011, 52, 6403–6406 CrossRef CAS.
- E. Benoit, B. Bueno, C. Choiniere and A. Gagnon, J. Organomet. Chem., 2019, 893, 72–77 CrossRef CAS.
- Y. Murata, S. Asano, R. Kato, Y. Kitamura, M. Matsumura and S. Yasuike, Catal. Commun., 2019, 132, 105808 CrossRef.
- E. Benoit, A. Fnaiche and A. Gagnon, Beilstein J. Org. Chem., 2019, 15, 1162–1171 CrossRef CAS PubMed.
- B. Song, N. Cao, J. Zhang and J. Xie, Mol. Catal., 2021, 516, 111981 CrossRef CAS.
- X. Zhou, H. Ma and J. Xie, J. Mol. Struct., 2023, 1294, 136437 CrossRef CAS.
- C. Crifar, P. Petiot, T. Ahmad and A. Gagnon, Chem. – Eur. J., 2014, 20, 2755–2760 CrossRef CAS PubMed.
- P. Petiot, J. Dansereau and A. Gagnon, RSC Adv., 2014, 4, 22255–22259 RSC.
- W. Qin, N. Kakusawa, Y. Wu, S. Yasuike and J. Kurita, Chem. Pharm. Bull., 2009, 57, 436–438 CrossRef CAS PubMed.
- W. Qin, S. Yasuike, N. Kakusawa, Y. Sugawara, M. Kawahata, K. Yamaguchi and J. Kurita, J. Organomet. Chem., 2008, 693, 109–116 CrossRef CAS.
- A. Siva Reddy, K. Ranjith Reddy, D. Nageswar Rao, C. K. Jaladanki, P. V. Bharatam, P. Y. S. Lam and P. Das, Org. Biomol. Chem., 2017, 15, 801–806 RSC.
Footnote |
† Electronic supplementary information (ESI) available: Characterization data and copies of 1NMR, 13CNMR and 19F NMR spectra of compound 3, 6 and 7. See DOI: https://doi.org/10.1039/d3ra06582k |
|
This journal is © The Royal Society of Chemistry 2023 |