DOI:
10.1039/D3RA04965E
(Paper)
RSC Adv., 2023,
13, 28904-28911
Past-expiration-date liquid disinfectants to deactivate biological and chemical toxins on building material surfaces
Received
23rd July 2023
, Accepted 19th September 2023
First published on 3rd October 2023
Abstract
In this study, we evaluated the deactivating efficacy of strong basicity-based (T4-102) and hydrogen peroxide-based (DF-200) disinfectants that were past their expiration date when used to deactivate biological and chemical toxins on building material surfaces. The decontamination efficacies of DF-200 and T4-102 disinfectants against dimethyl methylphosphonate (DMMP) and 2-chloroethyl ethylsulfide (2-CEES) were studied using GC-MS analysis. The bactericidal efficacies of disinfectants against Gram-negative E. coli and P. aeruginosa, and Gram-positive B. subtilis and S. aureus, were assessed in terms of the zone of inhibition, minimum inhibitory concentration (MIC), and minimum bactericidal concentration (MBC). The results indicated that the deactivation efficacy varied significantly according to the disinfectant amount, contact time, and building material. Higher efficacy of up to 99–100% was observed for biological toxins, despite passing their expiration dates. Approximately 70–78% of deactivation efficacies were observed for disinfectants against DMMP on the tile coupon at 100 μL and 24 h contact time. Moreover, the deactivation efficacy of DF-200 was better than that of T4-102. The data presented here demonstrate that the responders may use past-expiration-date disinfectants for efficacious disinfectaion in large-scale contamination incidents.
1. Introduction
The decontamination of biological and chemical toxins from building material surfaces using liquid disinfectants with past-expiration-date has not been extensively examined in the literature. The Department of Defense (DoD) decontamination manual calls attention to the fact that the decontamination of chemical and biological agents is fundamentally different from hazardous material decontamination. Decontamination practices for biological and chemical toxins have noticed that decontamination practices should be tailored to biochemicals encountered to maximize efficacy and minimize the risk of transferring contamination from the scene.1 Chemical warfare agents (CWA) are chemical weapons used in terrorist attacks, which pose an intense threat to the environment and civilian population. Chemical warfare agent simulants (CWAS) have been developed recently; these mimic the actual CWA, carrying all the relevant chemical and physical properties without the accompanying toxicological properties. DMMP and 2-CEES are simulants of sarin and mustard CWA, respectively.2–5 Certain Gram-positive bacteria, such as Bacillus and Clostridium species, are able to protect themselves from environmental stress or nutrient depletion by a sporulation process. Bacterial spores have always been considered a threat either through their potential for use as biological weapons or owing to food and hospital contaminations.6–8
Several decontamination technologies have been proposed in the past, and different sporicidal treatments have been developed. Strong acids disrupt spore integrity;9,10 quaternary ammonia and other chemicals damage the inner membrane of the spores11,12 and peroxides disrupt the spore germination apparatus by targeting key proteins.13,14 The choice of a decontamination treatment depends on the use of technology, the biocide efficiency of the treatment, the type of the contaminated surface, and the basic materials needed for decontamination.15–18 The present study was designed to reflect, as realistically as possible, the conditions that would be encountered in decontaminating a building or outdoor area contaminated with chemical warfare agent simulants (DMMP and 2-CEES) and biological toxins (E. coli, P. aeruginosa, B. subtilis, and S. aureus). Specifically, the study used disinfectants that were past their expiration dates, test coupons of porous and nonporous building materials, and a variety of commercially available sporicidal disinfectants that were sprayed onto the test coupons in a manner similar to that used in the field. The use of past-expiration-date disinfectants to deactivate biological and chemical toxins on building material surfaces has previously not been extensively investigated.
Most of the current U.S. Army research focuses on disinfectants that can be used against biological and chemical threat agents.1 In this investigation, the decontamination efficacy of two disinfectants, DF-200 (hydrogen peroxide-based) and T4-102 (strong basicity-based) solutions, to deactivate chemical warfare agent simulants and biological toxins on common building materials was evaluated. Gas chromatography-mass spectrometry (GC-MS) analysis, zone of inhibition testing, minimum inhibitory concentrations (MICs), minimum bactericidal concentrations (MBCs), and plate-counting were used in this study to examine the decontamination efficacy, antibacterial activities, and bactericidal efficacies of DF-200 and T4-102 past their expiration dates.19,20 Moreover, the efficacies of selected commercial military disinfectants that were past their expiration date were tested for deactivating biological and chemical toxins on surfaces of the building material. This included the measurement of the efficacy of decontamination solutions at different amounts and contact times. This study was limited to an initial investigation of an expired decontamination product that would be efficacious against the targeted biochemicals on the contaminated surfaces of the building material.
2. Materials and methods
2.1 Biological and chemical toxins, disinfectants, and test materials
Chemical warfare agent simulants, DMMP and 2-CEES, were obtained from Sigma-Aldrich Co., Ltd. and used as the targets for the decontamination of chemical toxins. E. coli (ATCC 25922), P. aeruginosa (ATCC 27853), B. subtilis, and S. aureus (ATCC 25923) were obtained from the Food Industry Research and Development Institute, Taiwan, and were used as the reference strains in antibacterial testing. All decontamination solutions were prepared immediately prior to decontamination testing. DF-200 solution was prepared as per the manufacturer's instructions through proportional mixing of benzyl-C12-C16 alkyl dimethyl chlorides, liquid hydrogen peroxide, and diacetin. T4-102 was prepared in 100 mL batches according to the formulation described in the military reports, namely 70% diethylenetriamine NH2(CH2)2NH(CH2)2NH2, 28% propylene glycol methyl ether CH3CHOHCH2OCH3, and 2% NaOH. The two disinfectants were past their expiration dates by about 5 years. The chemical contents of the past-expiration-date liquid disinfectants were identical to those of pristine disinfectants. Common building materials, including tarmacadam, unpainted concrete, and tiles, were used as substrates for decontamination testing. The materials (Fig. 1) were cut into equally-sized coupons and then sterilized using an autoclave (120 °C, 15 psi, 50 min), with the exception of the tarmacadam coupons, which were sterilized using UV irradiation for 24 h.
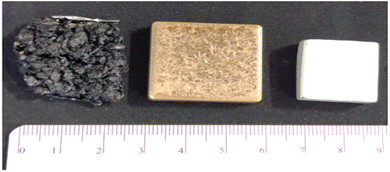 |
| Fig. 1 Photograph of representative material coupons. From left to right, tarmacadam, tile and concrete coupons were cut to size from larger pieces of stock materials and sterilized prior to use in decontamination tests. | |
2.2 Test of the decontamination and antibacterial properties
DMMP and 2-CEES were used to inoculate the surfaces of the test and positive control coupons. During inoculation, coupons were placed flat in a 60 mL wide-mouthed bottle and inoculated with 20 μL of DMMP or 2-CEES using a micropipette drop by drop across the surface of the coupon. Inoculated coupons were maintained for 30 min in the wide-mouthed bottle prior to being extracted (positive controls) or exposed to disinfectants. After the chemical contact time of 30 min with the coupons, the positive control coupon was extracted in 20 mL of the solvent. The extraction solvent was methanol for DMMP and isopropanol for 2-CEES.1 Coupons kept in the 60 mL wide-mouthed bottle were ultrasonically agitated for 10 min. Following sonication, the extract was transferred to a vial for GC-MS analysis.
In addition, the test coupons were maintained in contact with 100 μL of the disinfectant for 60 min, after which the extraction solution employed for the test coupons (those exposed to a disinfectant) was similar to that used for the positive control coupons. The decontamination efficacy is defined as the percentage decrease in the mean mass (corresponding to the GC-MS abundance value) of a chemical extracted from the test coupons (
TC) compared to the mean mass of chemicals extracted from the positive control coupon (
PC). To account for the possible losses of natural attenuation, positive control coupons contaminated and extracted under the same conditions as the test coupons were used to exclude such a decrease. Averages of three replicates of each were used to determine the decontamination efficacy for each material and decontaminant according to the following eqn:
|
Decontamination efficacy = 100% × ( PC − TC)/ PC
| (1) |
where
PC is the GC-MS abundance of the positive control coupon, and
TC is the abundance of test coupons. The GC-MS abundance tests of decontaminants against DMMP and 2-CEES on each material were performed three times, and the averages of the three replicates of each were used to determine the GC-MS abundance values for each material. The standard deviation of the mean mass recovered from the test and positive control coupons was used to derive the standard deviation of the decontamination efficacy.
1
The antibacterial spectra of the samples were evaluated using zone of inhibition testing. A standard inoculum of the test organism with 106–107 colony-forming units (CFU)/plate was swabbed onto the surface of a Muller–Hinton (MH) agar plate, and a disc of the filter paper impregnated with disinfectant (20 μL) was placed on the agar. Plates were incubated overnight at 37 °C, after which the clear zones around the discs were measured. The antibacterial effects of the disinfectants were evaluated by determining MICs and MBCs using the broth dilution method. Tubes containing 10 mL MH broth with 2-fold dilutions of the disinfectant were inoculated with about 6.0 × 106 CFU mL−1 of bacteria. The inoculated tubes were then incubated at 37 °C for 24 h, following which they were examined without shaking for visible turbidity. The MIC was determined as the lowest dilution of disinfectant that produced no visible turbidity.21 To determine the MBCs of the disinfectants, the viability of bacteria was assessed in tubes with no visible turbidity. Twenty microliters were drawn from each of the tubes and spread evenly on an MH agar plate, followed by incubation at 37 °C for 18 h. The number of bacterial colonies was counted using the plate-counting method.21 Zone of inhibition testing, MIC, and MBC methods were used to examine the differences in bactericidal properties between the different disinfectants, DF-200 and T4-102. The zone of inhibition, MIC, MBC, and plate-counting methods were performed three times for each strain, and the results in agreement on two or more occasions were adopted due to the strain. The counts of the three plates corresponding to a particular sample were averaged.22
2.3 Disinfectants and application procedures
The stock suspension (∼1 × 109 CFU mL−1) was used to inoculate the surfaces of the test and positive control coupons. During inoculation, coupons were placed flat in a 60 mL wide-mouthed bottle and inoculated with a 100 μL aliquot of stock suspension using a micropipette drop by drop across the surface of the coupon, yielding ∼1 × 108 CFU per coupon. Inoculated coupons were maintained for 15 min in the wide-mouthed bottle prior to extraction(positive controls), or exposed to disinfectants. Positive control coupons were extracted in 20 mL PBS. Coupons contained within the 60 mL wide-mouthed bottle were agitated for 15 min at room temperature on an orbital shaker set to 150 rpm. In addition, the test coupons were kept in contact with 100 μL of the disinfectant for 30 min, after which the extraction buffer formulations employed for the test coupons (those exposed to a disinfectant) were similar to those used for the positive control coupons. The following agitation, the liquid extract was removed and a series of 1
:
10 dilutions was prepared in PBS. An aliquot (0.1 mL) of each serial dilution was spread-plated on the appropriate solid growth media in triplicate and incubated at 37 °C for 24 h. Following incubation, colonies were manually counted and the abundance of CFU recovered from each sample was determined. The results of the persistence tests are presented as both the recovery and reduction; recovery data are presented as the mean log10 of CFU recovered from three replicate coupons for each exposure duration. Surface reduction efficacy was quantified by determining the difference in recovered viable cells between the positive control coupons and test coupons for each material and expressed as LR. Averages of the three replicates of each were used to determine the LR values for each material and decontaminant according to the following eqn: |
% Efficacy = (Log10 CFUDec)/(Log10 CFUCon) × 100%
| (2) |
where CFUCon is the abundance of colonies recovered from the positive control samples, and CFUDec (positive control sample CFUCon–test sample CFUCon) is the abundance of the colonies recovered from the test samples decontaminated at each exposure duration.
3. Results and discussion
3.1 Decontamination efficacy
The decontamination efficacy of disinfectants DF-200 and T4-102 against chemical warfare agent simulants, DMMP, and 2-CEES, were identified in GC-MS analysis. Fig. 2 and 3 provide the details of the relative product and GC-MS abundance of the disinfectants at 100 μL/1 h contact time against chemical toxins on coupons. After contaminating and extracting, the GC-MS peaks observed at 5.7 min and 6.3 min were for DMMP and 2-CEES, respectively. The other peaks may be due to the disinfectant and neutralization/oxidation products of the disinfectants and chemical toxins. When disinfectants were introduced, the DMMP and 2-CEES peaks were reduced for three coupons, which was especially significant for the concrete coupon. According to eqn (1), the overall test was performed three times, and averages of the three replicates of each were used to determine the GC-MS abundance values for each material. Based on the observed GC-MS results, the low abundance values may be due to the disinfectant concentration being too low for a fast neutralizing/oxidizing reaction. Strong bases effectively neutralize DMMP and 2-CEES; aqueous T4-102 was used as a standard method for the decontamination of bulk CWA in munitions. Solutions of DF-200 with the addition of hydrogen peroxide activators, cosolvents, and surfactants produced a rapid nucleophilic/oxidative decontamination of CWA. The T4-102 and DF-200 solutions decontaminated a broad spectrum of CWA, yielding nontoxic products.23
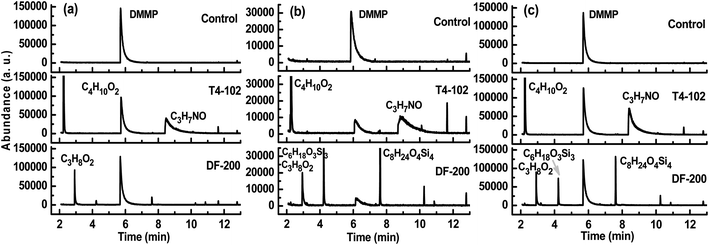 |
| Fig. 2 GC-MS abundance of disinfectants against DMMP on (a) tarmacadam, (b) concrete and (c) tile coupons. | |
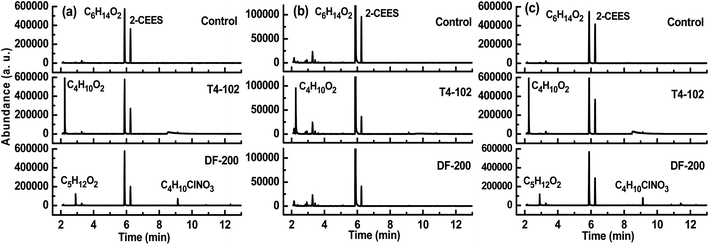 |
| Fig. 3 GC-MS abundance of disinfectants against 2-CEES on (a) tarmacadam, (b) concrete and (c) tile coupons. | |
The overall decontamination efficacy of disinfectants against DMMP and 2-CEES is summarized in Fig. 4. After using 100 μL of the disinfectant inoculation for 1 h contact time, the decontamination efficacy of two disinfectants on the concrete coupons was significantly higher (57–85%) than those observed on tarmacadam and tile coupons (14–44% and 8–31%). Both DF-200 and T4-102 exhibited decontamination efficacy against the targeted chemicals, depending on the coupon type. Micropores in the concrete coupon may have absorbed some of the more permeable disinfectants and chemical toxins, making these chemicals more accessible to the decontaminants.8 Both tarmacadam (large-pores) and tile (non-porous) may have insufficient amounts of disinfectants, and the contact time made these chemicals less accessible for decontamination. Based on the above observations, the decontamination efficiency of disinfectants against DMMP on the tile coupons with different amounts and contact times is shown in Fig. 5. The decontamination efficiency under 300 μL, 1 h, and 100 μL; 24 h conditions for T4-102 and DF-200 against DMMP were 46%, 70%, and 52%, 78%, respectively. The short contact time and limited amount of disinfectant on the contaminated surface limit the expected efficacy. Therefore, the contribution of neutralization/oxidation to the decontamination process while responders are preparing to doff the contaminated surface may be limited. In the case of DMMP, DF-200 was found to be more efficacious than T4-102 after 300 μL/1 h and 100 μL/24 h decontamination procedures. This means that DF-200 and T4-102 disinfectants would perform decontamination of chemical toxins even though they have passed their expiration date. Hydrogen peroxide and strong basicity disinfectants would be closely related to the decontamination efficiency, which depends on the amount of disinfectants and the contact time.
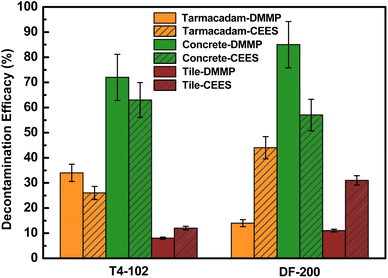 |
| Fig. 4 Decontamination efficacies for tested disinfectants against DMMP and 2-CEES. Error bars in efficacy are one standard deviation from the mean (n = 3). | |
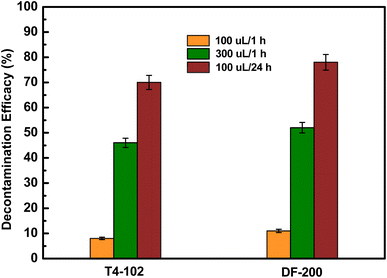 |
| Fig. 5 Decontamination efficacies of disinfectants against DMMP on tile coupon with different amount and contact time. Error bars in efficacy are one standard deviation from the mean (n = 3). | |
3.2 Bactericidal efficacy
The antibacterial efficacies of disinfectants DF-200 and T4-102 against bacteria were assessed by the zone of bactericidal testing, MIC, and MBC. Fig. 6 and Table 1 detail the relative retention of the activity (zone of inhibition) of the disinfectants against bacteria. After 24 h of incubation, the zones of inhibition of DF-200 against bacteria were significantly greater (20.20–22.63 mm) than those observed for T4-102 (18.92–20.20 mm). Both DF-200 and T4-102 exhibited significant efficacy against bacteria of the Gram class, especially P. aeruginosa and S. aureus. The MIC and MBC values, and photographs of the disinfectants acting against bacteria, are shown in Fig. 7 and Table 2. The MIC/MBC values of DF-200 and T4-102 against bacteria were 0.02/0.03 and 0.33–0.72/0.67–1.44, respectively, indicating that the antibacterial activity of the disinfectants was of the order DF-200 > T4-102. This means that the hydrogen peroxide-based (DF-200) disinfectant performed better than the strong basicity-based (T4-102) disinfectant against bacteria as an antimicrobial agent. Following the incubation of MIC and MBC colonies, the CFUs of each sample were obtained, as shown in Fig. 7. The number of bacterial colonies grown on MH plates was similar to that obtained from the MIC/MBC values. The results of the zone of bactericidal testing, MIC, and MBC demonstrated a difference in the antimicrobial properties of DF-200 and T4-102. Hydrogen peroxide and strong basicity were closely related to the antibacterial activity, which depended on the disinfectant content and expiration date of the disinfectant. It is believed that DNA loses its replication ability and cellular proteins become inactivated upon the disinfectant treatment.22 In addition, it has also been shown that, following the catalytic oxidation of the disinfectant with nascent oxygen, the reaction occurs with bacterial cell membranes, leading to cell death.24 Antimicrobial action in water is usually achieved upon contact with the following sequence of the elementary processes: adsorption onto the microbial cell surface, diffusion through the cell wall, binding and disruption of the cytoplasmic membrane, and release of the cytoplasmic constituents, resulting in cell death.25
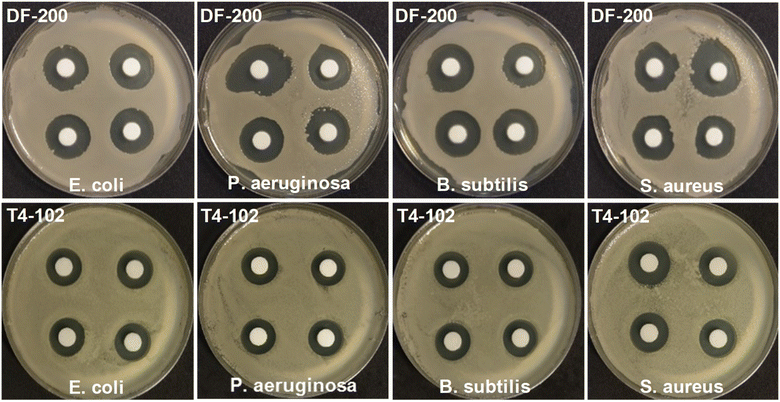 |
| Fig. 6 Photographs of the bactericidal test results of these microbes in the DF-200 and T4-102 disinfectants. | |
Table 1 Zone of inhibition (mm) against bacteria of the DF-200 and T4-102 disinfectantsa
Bacteria |
E. coli |
P. aeruginosa |
B. subtilis |
S. aureus |
The test was performed three times for each strain, and the count on three plates corresponding to a particular sample was averaged. |
DF-200 |
21.07 |
22.63 |
20.20 |
21.32 |
T4-102 |
19.36 |
19.76 |
18.92 |
20.20 |
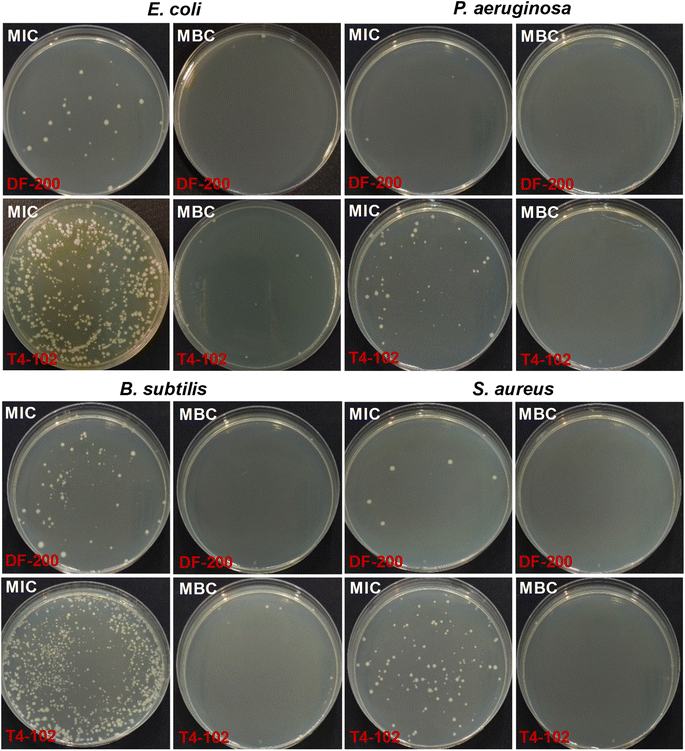 |
| Fig. 7 The photographs of MH plates incubated under the condition of MIC and MBC in Table 2. | |
Table 2 The MIC and MBC values of the DF-200 and T4-102 disinfectants on bacteriaa
Bacteria |
E. coli |
P. aeruginosa |
B. subtilis |
S. aureus |
The test was performed three times for each strain, and results in agreement on two or more occasions were adopted as the MIC(MBC) of the strain. |
Minimum inhibitory concentration (mL mL−1) |
DF-200 |
0.02 |
0.02 |
0.02 |
0.02 |
T4-102 |
0.72 |
0.33 |
0.67 |
0.33 |
![[thin space (1/6-em)]](https://www.rsc.org/images/entities/char_2009.gif) |
Minimum bactericidal concentration (mL mL−1) |
DF-200 |
0.03 |
0.03 |
0.03 |
0.03 |
T4-102 |
1.44 |
0.67 |
1.33 |
0.67 |
Decontamination testing using the complex material surfaces, such as those in the current study, is complicated by variables such as the spore recovery from control and test coupons, consistent application, penetration of the disinfectant into the material, and effective extraction of the disinfectant. With respect to the inoculated surface, one of these test materials (tile) can be considered non-porous, whereas the other two test materials (tarmacadam, unpainted concrete) can be considered porous. A summary of the bactericidal efficacy data, in terms of the mean of the LR, is presented in Table 3 for DF-200 and T4-102. Bactericidal efficacy varied narrowly, depending primarily on the test material and disinfectant, and somewhat on the test organism. The data presented in Table 3 demonstrate that the bacterial colonies of Gram-positive S. aureus and B. subtilis were completely killed after 30 min of contact time, and the average percentage reduction of bacteria was 100%, after 24 h of inoculation with DF-200 (no CFU recovered from any test coupons), demonstrating the best performance. Overall, T4-102 was less effective, with average bactericidal efficacy ranging from 98.96% to 99.85%, and a mean recovery of Gram-bacteria from test coupons lower than 1%. In addition, the effect of the material type on bactericidal efficacy was evident, as the tile was more easily decontaminated than the tarmacadam and concrete coupons with DF-200 during the bactericidal testing; however, the effect of T4-102 was less apparent. As the high CFU levels applied in this study are rarely found in real-life systems, it appears that DF-200 and T4-102 possess excellent biocidal effects and are effective in reducing bacterial growth, although the two disinfectants were past their expiration dates by about 3–5 years. The mechanism of the bactericidal action of disinfectants on microorganisms is a catalytic oxidation reaction that occurs with bacterial cell membranes, leading to cell death.9
Table 3 Bactericidal efficacy after 1 h of bactericidal treatment on material surfacea
Bacteria |
Disinfectant |
Material |
Control (Log10CFUCon) |
Decontaminated (Log10CFUDec) |
% efficacy |
% Efficacy = (Log10CFUDec)/(Log10CFUCon) × 100%. Decontaminated values are expressed as mean ± SD from triplicate samples of each test material. |
E. coli |
DF-200 |
Tarmacadam |
6.08 |
6.02 ± 0.22 |
99.01% |
|
|
Concrete |
6.04 |
6.02 ± 0.13 |
99.67% |
|
|
Tile |
6.20 |
6.20 ± 0.05 |
100% |
|
T4-102 |
Tarmacadam |
6.08 |
6.06 ± 0.23 |
99.67% |
|
|
Concrete |
6.04 |
5.98 ± 0.16 |
99.01% |
|
|
Tile |
6.20 |
6.16 ± 0.06 |
99.35% |
P. aeruginosa |
DF-200 |
Tarmacadam |
6.62 |
6.62 ± 0.19 |
100% |
|
|
Concrete |
5.70 |
5.69 ± 0.11 |
99.82% |
|
|
Tile |
5.79 |
5.79 ± 0.03 |
100% |
|
T4-102 |
Tarmacadam |
6.62 |
6.61 ± 0.20 |
99.85% |
|
|
Concrete |
5.70 |
5.67 ± 0.11 |
99.47% |
|
|
Tile |
5.79 |
5.73 ± 0.08 |
98.96% |
B. subtilis |
DF-200 |
Tarmacadam |
5.97 |
5.97 ± 0.09 |
100% |
|
|
Concrete |
6.20 |
6.20 ± 0.08 |
100% |
|
|
Tile |
6.18 |
6.18 ± 0.05 |
100% |
|
T4-102 |
Tarmacadam |
5.97 |
5.94 ± 0.11 |
99.50% |
|
|
Concrete |
6.20 |
6.18 ± 0.09 |
99.68% |
|
|
Tile |
6.18 |
6.16 ± 0.06 |
99.68% |
S. aureus |
DF-200 |
Tarmacadam |
5.80 |
5.80 ± 0.09 |
100% |
|
|
Concrete |
5.61 |
5.61 ± 0.07 |
100% |
|
|
Tile |
6.11 |
6.11 ± 0.05 |
100% |
|
T4-102 |
Tarmacadam |
5.80 |
5.75 ± 0.12 |
99.14% |
|
|
Concrete |
5.61 |
5.56 ± 0.10 |
99.11% |
|
|
Tile |
6.11 |
6.08 ± 0.07 |
99.51% |
4. Conclusions
Numerous factors influence deactivation efficacy, including, according to the results of this study, the disinfectant choice, past-expiration-date disinfectant, material type (porous or non-porous), and chem-bio toxicant type. The decontamination efficiency and bactericidal efficacy of disinfectants beyond their expiration date were highlighted in this study. Hydrogen peroxide-based (DF-200) and strong basicity-based (T4-102) disinfectants had a decontamination efficacy (57–85%) against DMMP and 2-CEES on concrete at 100 μL/1 h and had bactericidal efficacy (98.96–100%) against E. coli, P. aeruginosa, B. subtilis, and S. aureus. Thus, this study demonstrated that these disinfectants (DF-200 and T4-102) have an acceptable deactivation efficacy of chem-bio toxicants on tarmacadam, concrete, and tile surfaces, even after their expiration date. Such results may be useful in the development of the guidance to aid in the deployment of liquid disinfectant after a wide-area release of chem-bio agents in a city environment. Moreover, this study provides data for battlefield studies using DF-200 and T4-102.
Conflicts of interest
There are no conflicts to declare.
Notes and references
- C. R. Jabbour, L. A. Parker, E. M. Hutter and B. M. Weckhuysen, Nat. Rev. Chem, 2021, 5, 370–387 CrossRef PubMed.
- K. Ma, Y. H. Cheung, K. O. Kirlikovali, X. Wang, T. Islamoglu, J. H. Xind and O. K. Farha, Acc. Mater. Res., 2023, 4, 168–179 CrossRef.
- Y. H. Cheung, K. Ma, M. C. Wasson, X. Wang, K. B. Idrees, T. Islamoglu, J. Mahle, G. W. Peterson, J. H. Xin and O. K. Farha, Angew. Chem., Int. Ed., 2022, 61, e202202207 CrossRef PubMed.
- Y. Song, C. Peng, Z. Iqbal, K. K. Sirkar, G. W. Peterson, J. J. Mahle and J. H. Buchanan, ACS Appl. Mater. Interfaces, 2022, 14, 31321–31331 CrossRef.
- Y. H. Cheung, K. Ma, H. C. van Leeuwen, M. C. Wasson, X. Wang, K. B. Idrees, W. Gong, R. Cao, J. J. Mahle, T. Islamoglu, G. W. Peterson, M. C. de Koning, J. H. Xin and O. K. Farha, J. Am. Chem. Soc., 2021, 143, 16777–16785 CrossRef CAS PubMed.
- Y. Kim, L. Ma, K. Huang and N. Nitin, Curr. Opin. Biotechnol., 2023, 79, 102871 CrossRef CAS.
- S. A. Bhatti, F. H. Memon, F. Rehman, Z. Bhatti, T. Naqvi and K. H. Thebo, Rev. Inorg. Chem., 2022, 42, 283–295 CrossRef CAS.
- W. R. Richter, J. P. Wood, M. Q. S. Wendling and J. V. Rogers, J. Environ. Manage., 2018, 206, 800–806 CrossRef CAS.
- K. Gallandat, R. C. Kolus, T. R. Julian and D. S. Lantagne, Am. J. Infect. Control, 2021, 49, 90–103 CrossRef CAS PubMed.
- M. W. Calfee and M. Wendling, Sci. Total Environ., 2013, 443, 387–396 CrossRef CAS.
- M. P. Wylie, R. A. Craig, S. P. Gorman and C. P. McCoy, Int. J. Antimicrob. Agents, 2021, 57, 106360 CrossRef PubMed.
- A. G. Rios-Castillo, F. Gonzalez-Rivas and J. J. Rodriguez-Jerez, J. Food Sci., 2017, 82, 2351–2356 CrossRef CAS PubMed.
- G. K. Abdel-Latef, A. N. Mohammed and J. Adv, J. Vet. Anim. Res., 2021, 8, 105–115 Search PubMed.
- F. Amini Tapouk, R. Nabizadeh, N. Mirzaei, N. H. Jazani, M. Yousefi and M. A. Valizade Hasanloei, Antimicrob. Resist. Infect. Control., 2020, 9, 115 CrossRef.
- I. A. Jones and L. T. Joshi, Molecules, 2021, 26, 2276 CrossRef CAS PubMed.
- V. Drauch, C. besich, C. Vogl, M. Hess and C. Hess, Int. J. Food Microbiol., 2020, 328, 108660 CrossRef CAS PubMed.
- T. Matsubara, S. Maki and Y. Toshimori, Sci. Rep., 2021, 11, 1072 CrossRef CAS.
- K. H. Wu, Y. C. Chang and J. C. Wang, J. Inorg. Biochem., 2019, 199, 110788 CrossRef CAS.
- H. Mohamed, E. Marusich, Y. Afanasev and S. Leonov, Front. Microbiol., 2022, 13, 844811 CrossRef PubMed.
- L. Oudejans, J. O'Kelly, A. S. Evans, B. Wyrzykowska-Ceradini, A. Touati, D. Tabor and E. G. Snyder, J. Environ. Chem. Eng., 2016, 4, 2745–2753 CrossRef CAS.
- Y. Li, P. Leung and L. Yao, J. Hosp. Infect., 2006, 62, 58–63 CrossRef CAS.
- K. H. Wu, J. C. Wang, J. Y. Huang, C. Y. Huang, Y. H. Cheng and N. T. Liu, Mater. Sci. Eng., C, 2019, 98, 177–184 CrossRef CAS.
- S. S. Talmage, A. P. Watson, V. Hauschild, N. B. Munro and J. King, Curr. Org. Chem., 2007, 11, 285–298 CrossRef CAS.
- A. Tofant, M. Vucemilo, Z. Pavicic and D. Milic, Livest. Sci., 2006, 102, 243–247 CrossRef.
- J. Song, H. Kong and J. Jang, Colloids Surf., B, 2011, 82, 651–656 CrossRef CAS.
|
This journal is © The Royal Society of Chemistry 2023 |
Click here to see how this site uses Cookies. View our privacy policy here.