DOI:
10.1039/D3RA04350A
(Paper)
RSC Adv., 2023,
13, 24460-24465
Chiral bifunctional organocatalysts for enantioselective synthesis of 3-substituted isoindolinones†
Received
29th June 2023
, Accepted 7th August 2023
First published on 15th August 2023
Abstract
A series of chiral bifunctional organocatalysts were prepared and used for enantioselective synthesis of 3-substituted isoindolinones from 2-formylarylnitriles and malonates through aldol-cyclization rearrangement tandem reaction in excellent yields and enantioselectivites (up to 87% yield and 95% ee) without recrystallization. In this investigation, we found that chiral tertiary-amine catalysts with a urea group can afford 3-substituted isoindolinones both in higher yields (87% vs. 77%) and enantioselectivities (95% ee vs. 46% ee) than chiral bifunctional phase-transfer catalysts.
1 Introduction
3-Substituted isoindolinones are important heterocycles present in many bioactive molecules, for examples, a anxiolytic agent (S)-pagoclone,1 a potent dopamine D4 ligand (S)-PD172938,2 antitumor agents NMS-P515 (ref. 3) and (R)-NU8165 (ref. 4) and so on (Fig. 1). Due to their versatile physiological activities, many synthetic routes to 3-substituted isoindolinones have been developed by organic chemists. Recently, the catalytic asymmetric synthesis of isoindolinones has been summarized well by Peng and colleagues.5 On one hand, metal-catalyzed asymmetric preparation of 3-substituted isoindolinones was developed rapidly, Mg(II),6 Cu(I),7 Cu(II),8 Rh(III),9 Pd(II)10 and other metals with various chiral ligands as catalysts were used to produce isoindolinones in good to excellent yields and enantioselectivities, the reaction types of aza-Wacker type cyclization, tandem Michael–Mannich reaction, intramolecular dearomatization and hydrogenation are involved. In these metal-catalyzed reactions, α,β-unsaturated ketones with N-tosyl imines,7 2-formylarylcarboxylic esters, arylamines with terminal alkynes,8 aryl hydroxamates with diazo esters,9a N-methoxy arylamides with α,α-difluoromethylene alkynes,9b were used as starting materials. On the other hand, many chiral organocatalysts including chiral phosphoric acids, ammonium salts, (thio)ureas and proline-derived silylethers have shown good chiral induction in asymmetric synthesis of 3-substituted isoindolinones with moderate to good enantio-selectivities (Fig. 2).11 In some organocatalyzed synthetic routes to chiral 3-substituted isoindolinones, excellent ee values of products were obtained through recrystallization,11a which resulted in a loss of yield. Therefore, it is necessary to find an effective method to prepare 3-substituted isoindolinones with high yields and enenatioselectivities in one pot without tedious recrystallization. We have reported asymmetric amination of nitrooleins by chiral phase-transfer catalysts (CPTCs) under neutral and water-rich conditions with high yields and enantioselectivities.12 The precursors of these CPTCs are chiral bifunctional organocatalysts. For our continuous interest on the application of these CPTCs and bifunctional organocatalysts,13 herein, we have demonstrated the comparison of chiral induction of them for preparation of 3-substituted isoindolinones from 2-formylarylnitriles and malonates through aldol-cyclization rearrangement in good yields and excellent enantioselectivites (up to 87% yield and 95% ee) without recrystallization.
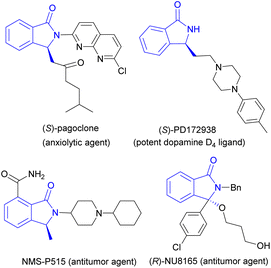 |
| Fig. 1 Representative bioactive compounds containing 3-substituted isoindolinone core. | |
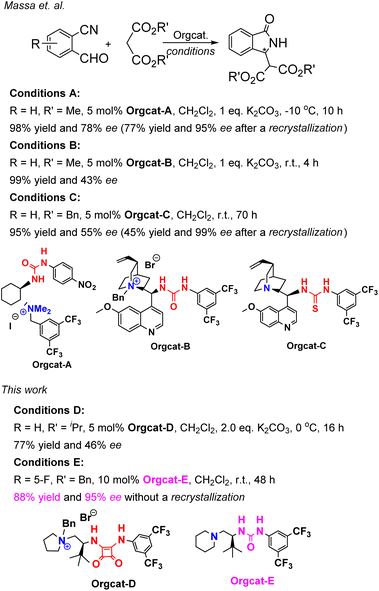 |
| Fig. 2 The synthesis of chiral 3-substituted isoindolinones by organocatalyzed aldol-cyclization rearrangement reaction. | |
2 Results and discussion
2.1. Chiral bifunctional phase-transfer catalysts for synthesis of 3-substituted isoindolinones
Massa and colleagues have developed catalytic enantioselective synthesis of 3-substituted isoindolinones by chiral phase-transfer catalysts (CPTCs).11 These CPTCs are derived from 1,2-diaminocyclohexane and cinchona alkaloids, respectively. The enantiomeric 3-substituted isoindolinones were obtained in excellent yields (98%) and good ee values (up to 78% ee at −10 °C). The ee values of products can be promoted to be 99% ee after a recrystallization with a sacrifice for half original chemical yields. Chiral bifunctioanl phase-transfer catalysts with various privileged scaffolds have been developed in recent years for many enantioselective transformations.14 we have reported catalytic asymmetric amination of β-nitrostyrenes by CPTCs in high yields and enantioselectivties. Inspired by these above-mentioned researches, herein, we have demonstrated a group of CPTCs for enantioselective synthesis of 3-substituted isoindolinones from 2-formylbenzonitriles and malonates. The chemical structures of CPTCs are illustrated in Fig. 3. At first, we choose the substrates 2-formylbenzonitrile 1a and diisopropyl malonate 2c as model substrates to investigate the enanatio-induction of all listed CPTCs. The catalytic results are shown in Table 1. It was found that all CPTCs can catalyze this reaction under standard conditions (entries 2–17), 3ac was obtained in moderate to good yields and low to moderate ee values. Among them, CPTC-12 is the best catalyst to provide 3ac in good yield (77%) but with a moderate enantioselectivity (46% ee, entry 13). When the reaction was performed at room temperature (r. t.), the enantioselectivity is decreased to 19% ee (entry 18). The solvent was switched to THF and toluene, 3ac was obtained as racemate respectively (entries 19 and 20). CHCl3 was used as a solvent to give 3ac in both low yield and enantioselectivity (entry 21). No reaction was found when EtOH was used as a solvent (entry 22). More basic additive Cs2CO3 can spoil the reaction to produce 3ac in high yield (78%) but very low ee (7% ee, entry 23), and less basic additive Na2CO3 resulted in no reaction (entry 24). Due to unfavourable results were obtained under phase-transfer catalysis conditions, no further substrate-extending was carried out.
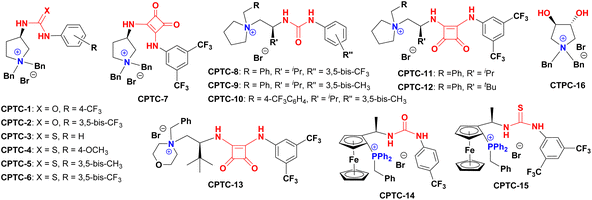 |
| Fig. 3 The chemical structures of CPTCs. | |
Table 1 The screening of CPTCs
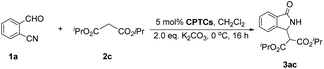
|
Entrya |
CPTC |
Yieldb (%) |
eec (%) |
Config.d |
1.0 mmol of 1a and 1.1 mmol of 2c in 5 mL dry CH2Cl2 were stirred under inert atmosphere. Isolated yield based on 1a. Determined by chiral HPLC. Compare to literature report. r. t. reaction. THF as solvent. Toluene as solvent. CHCl3 as solvent. EtOH as solvent, N. R. is no reaction. 2.0 eq. Cs2CO3 as additive. 2.0 eq. Na2CO3 as additive. |
1 |
None |
67 |
0 |
— |
2 |
CPTC-1 |
79 |
32 |
S |
3 |
CPTC-2 |
72 |
26 |
S |
4 |
CPTC-3 |
53 |
15 |
S |
5 |
CPTC4 |
48 |
11 |
S |
6 |
CPTC-5 |
56 |
25 |
S |
7 |
CPTC-6 |
78 |
24 |
S |
8 |
CPTC-7 |
70 |
34 |
S |
9 |
CPTC-8 |
75 |
13 |
S |
10 |
CPTC-9 |
51 |
15 |
S |
11 |
CPTC-10 |
74 |
22 |
S |
12 |
CPTC-11 |
73 |
11 |
S |
13 |
CPTC-12 |
77 |
46 |
S |
14 |
CPTC-13 |
76 |
44 |
S |
15 |
CPTC-14 |
76 |
13 |
S |
16 |
CPTC-15 |
79 |
17 |
S |
17 |
CPTC-16 |
71 |
10 |
S |
18e |
CPTC-12 |
71 |
19 |
S |
19f |
CPTC-12 |
78 |
0 |
— |
20g |
CPTC-12 |
52 |
0 |
— |
21h |
CPTC-12 |
49 |
27 |
S |
22i |
CPTC-12 |
N. R. |
— |
— |
23j |
CPTC-12 |
78 |
7 |
S |
24k |
CPTC-12 |
N. R. |
— |
— |
2.2. Chiral bifunctional organocatalysts for synthesis of 3-substituted isoindolinones
Cinchona-derived ureas and thioureas have been used as chiral organocatalysts for preparation of chiral 3-substituted isoindolinones in excellent yield and moderate enantioselectivities by Massa and colleagues.11a Inspired by their research work, we thought that chiral bifunctional organocatalysts (Bif-OCs) containing one tertiary amine and one hydrogen-bonding donor group (such as hydroxyl, amide, urea, thiourea and squaramide group) can sever as catalysts in this aldol-cyclization rearrangement reaction to prepare enantio-rich 3-substituted isoindolinones. Then, the catalytic activities of sixteen chiral bifunctional organocatalysts based privileged scaffolds (Fig. 4) were investigated in aldol-cyclization rearrangement reaction for preparation of chiral 3-substituted isoindolinones, and the results are listed in Table 2. L-Proline-derived Bif-OC-1 with a secondary amine group can not catalyze this reaction at standard conditions (10 mol% of catalyst, r. t., 48 h, in CH2Cl2). Neither Bif-OC-2 nor Bif-OC-3 can provide the corresponding product 3ac even possessing a tertiary amine group (entries 2 and 3). Thiourea Bif-OC-4 and squarmide Bif-OC-5 with 3-amino pyrrolidine backbone can afford 3ac in good yield but with low ees (entries 4 and 5). 1,2-Diaminocyclohexane based ureas Bif-OC-6 and Bif-OC-7 demonstrate good chiral induction in this model reaction to furnish 3ac in 76% and 75% yields with 69% and 68% ee values (entries 6 and 7). However, 1,2-diaminocyclohexane derived squaramides Bif-OC-8 and Bif-OC-9 with a bulky tertiary amine group can lead to a significant decrease both in yield and enantioselectivity of 3ac (entries 8 and 9). Cinchona alkaloid-derived Bif-OC-10, Bif-OC-11 and Bif-OC-12 are very attractive organocatalysts for this aldol-cyclization rearrangement reaction to give 3ac both in high yield (68–72%) and enantioselectivity (66–69% ee, entries 10 to 12). Chiral bifunctional urea organocatalysts from tert-leucine containing an electron-withdrawing aryl group (3,5-bis-CF3C6H3) Bif-OC-13 and Bif-OC-16 have shown the same and satisfactory catalytic activity in this model reaction, and 3ac was obtained in high yield (85%) and good enantioselectivity (71% ee, entries 13 and 16). Urea Bif-OC-14 with an electron-donating aryl group (3,5-bis-MeC6H3) can make a sharp decline in yield and ee of 3ac which compared to Bif-OC-16 (entry 14). Thiourea Bif-OC-15 has provided a inferior result to Bif-OC-16 in this aldol-cyclization rearrangement reaction (entry 15). Therefore, Bif-OC-16 was chosen as the best catalyst for this reaction. When THF was used as a solvent, no reaction was found at the same time (entry 17), while 3ac was afforded in 49% yield and 34% ee in CH3CN (entry 18). The solvent was switched to toluene, 3ac was obtained in higher enantioselectivity (73% ee) than in CH2Cl2 but with a low yield (34%, entry 19). The reaction was performed at 0 °C, no significant change in yield and ee of 3ac was found (entry 20). The yield and enantioselectivity of 3ac were decreased along with a decrease of amount of Bif-OC-16 (entries 21 and 22, 5 mol% and 1 mol% Bif-OC-16 was used, respectively). Additives Cs2CO3 and K2CO3 can overwhelm catalyst and lead to rac-3ac in high yield (entries 23 and 24). The optimal reaction conditions are listed here: 10 mol% of Bif-OC-16, CH2Cl2 as a solvent, and the reaction mixture was stirred at r. t. in 48 h.
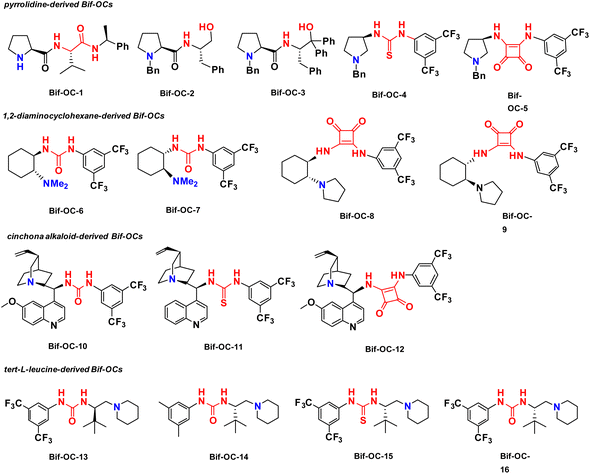 |
| Fig. 4 The chemical structures of Bif-OCs. | |
Table 2 The screening of Bif-OCs
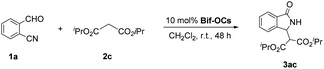
|
Entrya |
CPTC |
Yieldb (%) |
eec (%) |
Config.d |
1.0 mmol of 1a and 1.1 mmol of 2c in 5 mL dry CH2Cl2 were stirred under inert atmosphere. N. R. is no reaction. Isolated yield based on 1a. Determined by chiral HPLC. Compare to literature report. THF as solvent. CH3CN as solvent. Toluene as solvent. At 0 °C. 5 mol% Bif-OC-16 was used. 1 mol% Bif-OC-16 was used. 2.0 eq. Cs2CO3 as additive. 2.0 eq. K2CO3 as additive. |
1 |
Bif-OC-1 |
N. R. |
— |
— |
2 |
Bif-OC-2 |
N. R. |
— |
— |
3 |
Bif-OC-3 |
N. R. |
— |
— |
4 |
Bif-OC-4 |
73 |
37 |
S |
5 |
Bif-OC-5 |
77 |
10 |
S |
6 |
Bif-OC-6 |
76 |
69 |
S |
7 |
Bif-OC-7 |
75 |
68 |
R |
8 |
Bif-OC-8 |
41 |
19 |
S |
9 |
Bif-OC-9 |
48 |
21 |
R |
10 |
Bif-OC-10 |
68 |
69 |
S |
11 |
Bif-OC-11 |
72 |
68 |
S |
12 |
Bif-OC-12 |
73 |
66 |
S |
13 |
Bif-OC-13 |
83 |
70 |
R |
14 |
Bif-OC-14 |
52 |
32 |
S |
15 |
Bif-OC-15 |
77 |
36 |
S |
16 |
Bif-OC-16 |
85 |
71 |
S |
17e |
Bif-OC-16 |
N. R. |
— |
— |
18f |
Bif-OC-16 |
49 |
33 |
S |
19g |
Bif-OC-16 |
34 |
73 |
S |
20h |
Bif-OC-16 |
78 |
70 |
S |
21i |
Bif-OC-16 |
52 |
42 |
S |
22j |
Bif-OC-16 |
44 |
30 |
S |
23k |
Bif-OC-16 |
86 |
rac- |
— |
24l |
Bif-OC-16 |
82 |
rac- |
— |
With the optimal conditions in hand, the substrate scope of this reaction was investigated and the results are shown in Scheme 1. 3-Substituted isoindolinones 3aa–3cd were obtained in good to excellent yields and enantioselectivities under standard conditions (up to 94% yield and 95% ee) except 3ae (trace yield) due to its bulky tert-Bu group. Dibenzyl malonate is a good substrate for this reaction to provide the corresponding products 3ad and 3bd in satisfactory yields (89% and 86% yields) and excellent ees (93% and 95% ees) without recrystallization, however, when 5-chloro-2-formylbenzonitrile was used as substrate, 3cd was obtained in somewhat low enantioselectivity (73% ee of 3cd vs. 95% ee of 3bd). In order to show the practical use of this method, 3bd was prepared in 10 mmol scale in 89% yield and 95% ee. This product 3bd can be transformed to the corresponding chiral acid through hydrolysis and decarboxylation, and this chiral acid can be used as a building block for preparation of F-containing Pazinaclone and (S)-PD-172938 (Scheme 2).11a No corresponding products were obtained when malononitrile and acetylacetone were used as substrates under the same conditions. There is a limitation of functional group transformation (FGT) when activated symmetric methylene compounds (such as dialkyl malonate or malononitrile) were used, decarboxylation following hydrolysis is common conversion of products 3aa–3cd. It should be more interesting and useful to take activated unsymmetric methylene compounds (such as ethyl acetoacetate, ethyl 2-nitroacetate, ethyl 2-cyanoacetate, or nitromethane) as substrates. And then, we have investigated the performance of these activated unsymmetric methylene compounds 4 in this aldol-cyclization rearrangement reaction, and the results are listed in Scheme 3. All activated unsymmetric methylene compounds 4 used can take place the aldol-cyclization rearrangement reaction to provide corresponding products 5 in good yields (67–83%) but with poor diastereoselectivities (d. r. is about 1
:
1), and the enantioselectivities of major product are moderate (53–67% ee) except 5ae.
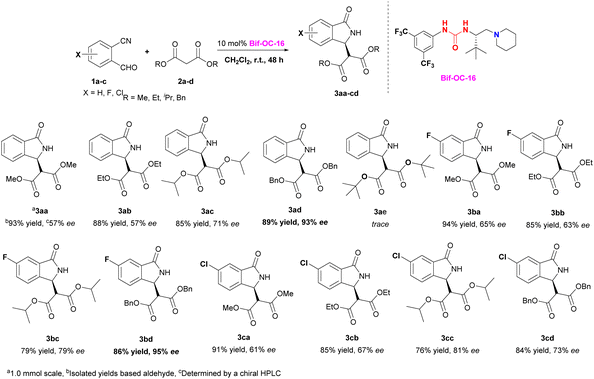 |
| Scheme 1 Bif-OC-16 catalyzed aldol-cyclization rearrangement reactions. | |
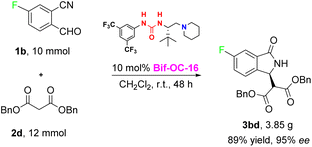 |
| Scheme 2 Gram-scale preparation of 3bd. | |
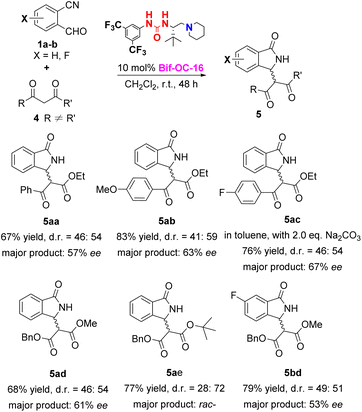 |
| Scheme 3 Unsymmetric methylene compounds 4 in preparation of 3-substituted isoindolinones. | |
A plausible mechanism for the formation of enantiomeric 3-substituted isoindolidinones 3 in the presence of Bif-OC-16 is shown in Fig. 5. Initially, the aldol-cyclization of 2-formylarylnitriles 1 and malonates 2 took place in the presence of Bif-OC-16 to form intermediate A, which underwent elimination and rearrangement to provide intermediate B. Bif-OC-16 may play cooperative action mode with intermediate B through H-bonding between amide moiety and carboxylic group to activate both Michael donor and acceptor. The facial contact with the nucleophiles could be assisted by this cooperative action. The effect of this urea-based catalyst (Bif-OC-16) was significant, providing the desired products 3 in up to 95% ee. This catalytic mode is different from the chiral phase-transfer catalyst (CPTCs), and the chiral induction of CPTCs mainly depends on the electrostatic attraction of chiral ion pair between substrate and CPTCs.
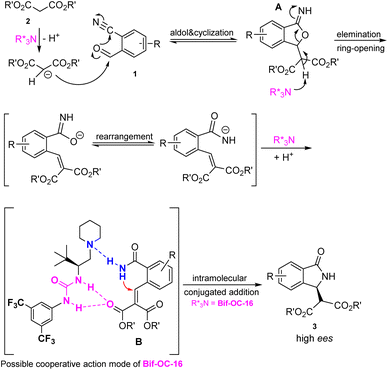 |
| Fig. 5 The proposed mechanism for Bif-OC-16 catalyzed aldol-cyclization. | |
3 Experimental
3.1. Typical procedure for synthesis of compound 3aa
To a dried reaction tube, 2-formylbenzonitrile 1a (1 mmol), dimethyl malonate 2a (1.1 mmol), Bif-OC-16 (45 mg, 0.1 mmol) and 5.0 mL of dry CH2Cl2 were added successively. The mixture is stirred at room temperature for 48 h, and the reaction was monitored by TLC. When TLC indicates that 1a was consumed, solvent CH2Cl2 was removed under reduced pressure to yield a yellow foam, which was purified by a flash column chromatography to give pure 3aa as white solid.
4 Conclusions
In conclusion, we have developed a facile access to enantiomeric 3-substituted isoindolidinones through aldol-cyclization rearrangement reaction catalyzed by chiral bifunctional organocatalysts in high yields and excellent enantioselectivities without recrystallization. It was found that chiral tertiary-amine urea catalyst can afford 3-substituted isoindolinones both in higher yields (87% vs. 77%) and enantioselectivities (95% ee vs. 46% ee) than chiral bifunctional phase-transfer catalysts.
Author contributions
P. A. W., Y. Y. Jia and G. Q. B. guided the research. X. M. H., H. D. and R. Z. conducted the experiments, analysed the results, and wrote the ESI and manuscript. Y. Y. J. and G. Q. B. helped revise the ESI and manuscript.
Conflicts of interest
There are no conflicts to declare.
Acknowledgements
We thank the National Science Foundation of China (no. 21372259) and The Fourth Military Medical University (2019XB069) for financial support of this work.
Notes and references
-
(a) T. L. Stuk, B. K. Assink, R. C. Bates, D. T. Erdman, V. Fedij, S. M. Jennings, J. A. Lassig, R. J. Smith and T. L. Smith, Org. Process Res. Dev., 2003, 7, 851 CrossRef CAS;
(b) Y. Zhang, H.-Q. Zhu, Y.-T. Huang, Q. Hu, Y. He, Y.-H. Wen and G.-G. Zhu, Org. Lett., 2019, 21, 1273 CrossRef CAS PubMed;
(c) M.-D. Chen, M.-Z. He, L.-Q. Huang, Y.-P. Ruan and P.-Q. Huang, Chin. J. Chem., 2002, 20, 1149 CrossRef CAS;
(d) R. A. Hemming, M. Bell, L. J. Duffy, J. Bristow, J. D. Wallis, S. M. Allin and P. C. Bulman, Tetrahedron, 2019, 75, 121 CrossRef CAS;
(e) S. A. Ali, A. Bera, M. R. Molla and S. Samanta, ChemistrySelect, 2021, 6, 5603 CrossRef.
-
(a) S. Royo, R. S. L. Chapman, A. M. Sim, L. R. Peacock and S. D. Bull, Org. Lett., 2016, 18, 1146 CrossRef CAS PubMed;
(b) S. K. Kulkarni and I. Ninan, Fundam. Clin. Pharmacol., 2009, 14, 529 CrossRef PubMed;
(c) Z.-P. Cao, H.-B. Zhu, X. Meng, J. Guan, Q. Zhang, L.-J. Tian, X.-J. Sun, G. Chen and J.-M. You, Chem. - Eur. J., 2016, 22, 16979 CrossRef CAS PubMed;
(d) S. Yuan, S.-X. Wang, M. Zhao, D.-Q. Zhang, J.-J. Chen, J.-X. Li, J.-Y. Zhang, Y.-H. Song, J.-Y. Wang, B. Yu and H.-M. Liu, Chin. Chem. Lett., 2020, 31, 349 CrossRef CAS.
-
(a) G. Papeo, P. Orsini, N. R. Avanzi, D. Borghi, E. Casale, M. Ciomei, A. Cirla, V. Desperati, D. Donati, E. R. Felder, A. Galvani, M. Guanci, A. Isacchi, H. Posteri, S. Rainoldi, F. Riccardi-Sirtori, A. Scolaro and A. Montagnoli, ACS Med. Chem. Lett., 2019, 10, 534 CrossRef CAS PubMed;
(b) A. Borja-Miranda, F. Valencia-Villegas, J. A. Lujan-Montelongo and L. A. Polindara-García, J. Org. Chem., 2021, 86, 929 CrossRef CAS PubMed;
(c) G. Papeo, H. Posteri, D. Borghi, A. A. Busel, F. Caprera, E. Casale, M. Ciomei, A. Cirla, E. Corti, M. D'Anello, M. Fasolini, B. Forte, A. Galvani, A. Isacchi, A. Khvat, M. Y. Krasavin, R. Lupi, P. Orsini, R. Perego, E. Pesenti, D. Pezzetta, S. Rainoldi, F. Riccardi-Sirtori, A. Scolaro, F. Sola, F. Zuccotto, E. R. Felder, D. Donati and A. Montagnol, J. Med. Chem., 2015, 58, 6875 CrossRef CAS PubMed;
(d) H. Shen, Y.-R. Ge, J.-W. Wang, H. Li, Y.-G. Xu and Q.-H. Zhu, Bioorg. Med. Chem. Lett., 2021, 47, 128169 CrossRef CAS PubMed.
-
(a) I. R. Hardcastle, J.-F. Liu, E. Valeur, A. Watson, S. U. Ahmed, T. J. Blackburn, K. Bennaceur, W. Clegg, C. Drummond, J. A. Endicott, B. T. Golding, R. J. Griffin, J. Gruber, K. Haggerty, R. W. Harrington, C. Hutton, S. Kemp, X.-H. Lu, J. M. McDonnell, D. R. Newell, M. E. M. Noble, S. L. Payne, C. H. Revill, C. Riedinger, Q. Xu and J. Lunec, J. Med. Chem., 2011, 54, 1233 CrossRef CAS PubMed;
(b) A. Lemos, M. Leão, J. Soares, A. Palmeira, M. Pinto, L. Saraiva and M. E. Sousa, Med. Res. Rev., 2016, 36, 789 CrossRef CAS PubMed.
- W. Gao, M.-W. Chen, Q.-P. Ding and Y.-Y. Peng, Chem.–Asian J., 2019, 14, 1306 CrossRef CAS PubMed.
- Y. Suzuki, M. Kanai and S. Matsunaga, Chem. - Eur. J., 2012, 18, 7654 CrossRef CAS PubMed.
- S.-M. Guo, Y.-J. Xie, X.-Q. Hu, C.-G. Xia and H. Huang, Angew. Chem., Int. Ed., 2010, 49, 2728 CrossRef CAS PubMed.
- A. Suneja, V. Bisai and V. K. Singh, J. Org. Chem., 2016, 81, 4779 CrossRef CAS PubMed.
-
(a) B.-H. Ye and N. Cramer, Angew. Chem., Int. Ed., 2014, 53, 7896 CrossRef CAS PubMed;
(b) T. Li, C. Zhou, X.-Q. Yan and J. Wang, Angew. Chem., Int. Ed., 2018, 57, 4048 CrossRef CAS PubMed.
- X.-F. Bai, Q.-C. Mu, Z. Xu, K.-F. Yang, L. Li, Z.-J. Zheng, C.-G. Xia and L.-W. Xu, ACS Catal., 2019, 9, 1431 CrossRef CAS.
-
(a) A. D. Mola, M. Tiffner, F. Scorzelli, L. Palombi, R. Filosa, P. D. Caprariis, M. Waser and A. Massa, Beilstein J. Org. Chem., 2015, 11, 2591 CrossRef PubMed;
(b) M. Perillo, A. D. Mola, R. Filosa, L. Palombi and A. Massa, RSC Adv., 2014, 4, 4239 RSC;
(c) S. Tiso, L. Palombi, C. Vignes, A. D. Mola and A. Massa, RSC Adv., 2013, 3, 19380 RSC;
(d) M.-W. Chen, Q.-A. Chen, Y. Duan, Z.-S. Ye and Y.-G. Zhou, Chem. Commun., 2012, 48, 1698 RSC.
- J.-C. Zhu, D.-X. Cui, Y.-D. Li, J.-X. He, W.-P. Chen and P.-A. Wang, Org. Biomol. Chem., 2018, 16, 3012 RSC.
-
(a) D.-X. Cui, Y. D. Li, P. Huang, Z. Tian, Y.-Y. Jia and P.-A. Wang, RSC Adv., 2020, 10, 12360 RSC;
(b) Y.-D. Li, D.-X. Cui, J.-C. Zhu, P. Huang, Z. Tian, Y.-Y. Jia and P.-A. Wang, Green Chem., 2019, 21, 5231 RSC.
-
(a) S.-Q. Fang, J.-P. Tan, J.-K. Pan, H.-K. Zhang, Y. Chen, X.-Y. Ren and T.-L. Wang, Angew. Chem., Int. Ed., 2021, 60, 14921 CrossRef CAS PubMed;
(b) M. Kondo, K. Nakamura, C. G. Krishnan, S. Takizawa, T. Abe and H. Sasai, ACS Catal., 2021, 11, 1863 CrossRef CAS;
(c) J.-C. Zhu, D.-X. Cui, Y.-D. Li, R. Jiang, W.-P. Chen and P.-A. Wang, ChemCatChem, 2018, 10, 907 CrossRef CAS;
(d) S. Kaneko, Y. Kumatabara and S. A. Shirakawa, Org. Biomol. Chem., 2016, 14, 5367 RSC.
Footnotes |
† Electronic supplementary information (ESI) available. See DOI: https://doi.org/10.1039/d3ra04350a |
‡ Co-first authors. These two authors have the same contribution to this work. |
|
This journal is © The Royal Society of Chemistry 2023 |
Click here to see how this site uses Cookies. View our privacy policy here.