DOI:
10.1039/D3RA03998F
(Paper)
RSC Adv., 2023,
13, 24466-24473
An efficient, catalyst-free and aqueous ethanol-mediated synthesis of 5-((2-aminothiazol-5-yl)(phenyl)methyl)-6-hydroxypyrimidine-2,4(1H,3H)-dione derivatives and their antioxidant activity†
Received
14th June 2023
, Accepted 7th August 2023
First published on 16th August 2023
Abstract
In this study, we effectively developed a catalyst-free multicomponent synthesis of 5-((2-aminothiazol-5-yl)(phenyl)methyl)-6-hydroxypyrimidine-2,4(1H,3H)-dione derivatives employing 2-aminothiazole, N′,N′-dimethyl barbituric acid/barbituric acid and different aldehydes at 80 °C in an aqueous ethanol medium (1
:
1) using group-assisted purification (GAP) chemistry. The essential characteristics of this methodology include superior green credential parameters, metal-free multicomponent synthesis, faster reaction times, greater product yields, simple product purification without column chromatography and higher product yields. All of the synthesized compounds were analyzed against the HepG2 cell line. Compounds 4j and 4k shows good anti-proliferative effects on HepG2 cells. Furthermore, the ABTS and DPPH scavenging assays were used to determine the antioxidant activity of all compounds (4a–r). In both ABTS and DPPH radical scavenging assays, compounds 4e, 4i, 4j, 4o and 4r exhibit excellent potency compared to the standard ascorbic acid.
1. Introduction
Reactive oxygen species (ROS) are integral to the emergence of several serious illnesses, such as cataracts, diabetes, arteriosclerosis, and cancer.1 Oxidative stress refers to the negative effects of free radicals that may result in biological damage. Free radicals play a pathogenic role in the development of numerous chronic degenerative diseases in the human body, including cancer, autoimmune, inflammatory, and cardiovascular neurodegenerative diseases.2,3 One or more molecules that donate unpaired electrons are known as free radicals; they have a short half-life, low stability, and significant chemical reactivity.4,5 These radicals are produced naturally or as a result of biological processes such as phagocytosis, cell growth control, substance synthesis, and signalling between cells. Lipids, proteins, and DNA are damaged by radicals.6 It is essential to make the free radicals ineffective to protect the tissue from these harms.7 Any substance that can either delay or prevent the oxidation of a substrate when it is present in a lower concentration than the substrate is considered to be an antioxidant.8 Antioxidant function relates to reducing oxidative stress, malignant transformation, DNA mutations, and damage parameters.9 Generally, the human body protects itself from oxidative stress by manufacturing antioxidants naturally and receiving exogenous antioxidants from the diet.10
The multicomponent synthetic approach was used to synthesize the tri-substituted methane derivative. Multicomponent synthetic strategies are most important for the synthesis of bioactive heterocyclic compounds due to the construction of complex target molecules to be derived from three or more reactants with greater efficiency, simplicity, and higher atom economy than the corresponding conventional multi-step approaches.11–19 The field of organic synthetic strategies GAP (group-assisted purification) chemistry is used for greener synthesis because it excludes classical purification techniques like column chromatography and recrystallization.20 It has driven the synthetic community to look for better ecologically friendly syntheses and reactions to speed up preparation and use less energy and effort.21–23
2-Aminothiazole has an important core structure that attracts researchers due to its extensive biological activity, such as antidiabetic,24 antitubercular,25 anticonvulsant,26 antioxidant,27 antimicrobial,28 antiviral,29 anticancer,30 anti-inflammatory31 and antifungal.32 It is a natural product found in vitamin thiamine (B1) and other natural sources.33 Therefore, they show a significant role in pharmacological applications and are also used for the development of drugs to treat various disorders. Specifically, the well-known medicine Famotidine, Cefdinir and Abafungin all contain 2-aminothiazole core. Barbituric acid and its derivatives are important compounds due to their therapeutic benefits. They exhibited a wide range of pharmacological activity such as sedative, local anesthetic drugs, anti-inflammatory, antibacterial, antisclerotics, antioxidant, and anticancer as well.34 They are well known for therapeutic medicines such as Veronal, bucolome, sodium pentothal Seconal, and Phenobarbital.35 Fig. 1 represents a glimpse of some of the 2-aminothiazole and barbituric acid molecular scaffolds of potential interest.34,36
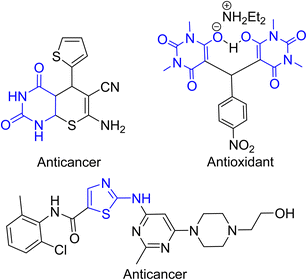 |
| Fig. 1 Some biologically active compounds. | |
In this study, we developed the catalyst-free synthesis of 5-((2-aminothiazol-5-yl)(phenyl)methyl)-6-hydroxypyrimidine-2,4(1H,3H)-dione derivatives using the GAP technique, which is important from a sustainability point of view. This tri-substituted methane derivative was derived from 2-aminothiazole, barbituric acid and various aromatic aldehydes using aqueous ethanol as solvent at 80 °C. All the derivatives (4a–r) were screened for their antioxidant (DPPH and ABTS) and cytotoxic activity.
2. Result and discussion
Initially, to optimize reaction conditions we chose 2-aminothiazole 1 (1.0 mmol), N,N′-dimethyl barbituric acid 2a (1.0 mmol) and p-chlorobenzaldehyde 3a (1.0 mmol) as our model substrate. We performed a series of trial reactions between all the above starting materials with different solvents and temperatures to check the successful reaction transformation with a higher yield. We found the best results for this multicomponent reaction in preparing desired product 4a with 92% yield at 30 min when we used aqueous ethanol (1
:
1, v/v) as a solvent at heating conditions (80 °C) (Table 1, entry 7). However, this reaction was also performed at room temperature but only an intermediate was observed on TLC.
Table 1 Optimization reaction conditiona
To investigate the effectiveness, generality and scope of this method, we conducted multi-component reactions between 1, 2 and 3 (various aromatic aldehydes) under the standard optimized condition. The electron-withdrawing group (EWG) containing aromatic aldehydes (p-Cl, o-Cl, p-Br, p-F, p-NO2, o-NO2, m-OCH3) was performed smoothly to furnish the desired 5-((2-aminothiazol-5-yl)(phenyl)methyl)-6-hydroxypyrimidine-2,4(1H,3H)-dione derivatives in good to excellent yields (80–92%). While the electron-donating group (EDG) containing aromatic aldehydes (p-CH3, p-OH, p-OCH3) provides a lower yield compared to EWG containing aldehydes as well as takes more time for reaction completion. The results are demonstrated in Table 2.
Table 2 Synthesis of 5-((2-aminothiazol-5-yl)(phenyl)methyl)-6-hydroxypyrimidine-2,4(1H,3H)-dione derivativesa
We expanded our research to assess the method's viability, so we replaced N,N′-dimethyl barbituric acid with barbituric acid. The multi-component reaction of 2-aminothiazole, barbituric acid and various aromatic aldehydes was processed smoothly and provided a higher product yield (Table 2, entry 12 to 18) under the same reaction condition rather than when we used N,N′-dimethyl barbituric acid.
The proposed mechanisms for the synthesis of 5-((2-aminothiazol-5-yl)(phenyl)methyl)-6-hydroxypyrimidine-2,4(1H,3H)-dione derivatives 4(a-r) has shown in Scheme 1. First, we assumed that barbituric acid (2) reacted with an Aldehyde (3) and formed an intermediate Knoevenagel adduct [A] which undergoes Michael addition reaction with 2-aminothiazole and followed by deprotonation result in product 4 was obtained.
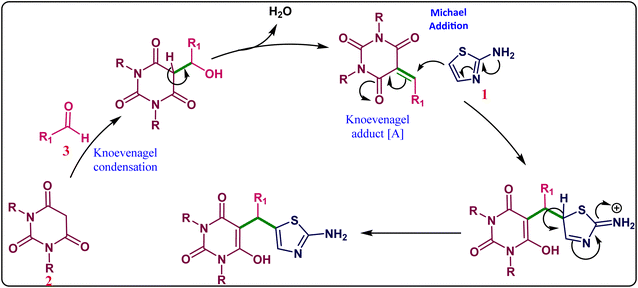 |
| Scheme 1 Proposed reaction mechanism. | |
3. Biological applications of synthesized compounds
3.1. Cytotoxicity effect of compounds at various concentrations in HepG2 cells
18 compounds (4a–r) of the 5-((2-aminothiazol-5-yl)(phenyl)methyl)-6-hydroxypyrimidine-2,4(1H,3H)-dione derivatives were chemically synthesized and screened for their antiproliferative activity on HepG2 (hepatocarcinoma) cell lines using MTT assay. The molecular weight of the chemical compounds ranged between 316.33 and 423.28. HepG2 cells were subjected to 1 hour serum starvation, followed by treatment with varying concentrations (20, 40, 60, 80, and 100 μM) of all the compounds for 24 and 48 hours. The cells treated with 20 μM of 5-fluorouracil were used as the positive control. Cells treated with the highest dose (100 μM) of the compounds exhibited viability of less than 50% after 24- and 48 hours treatment. Dose- and duration-dependent curves were estimated to determine the IC50 value which suggested that all the compounds exhibited dose-dependent cytotoxic effects on HepG2 cells. The IC50 value for the compounds was 100 ± 2 μM at 24- and 48 hours. These findings indicated that these compounds had gradually entered the cells and caused cell death. Due to the increased cell death, 100 μM concentration (24 hours) was chosen for further processing. Two compounds 4j and 4k exhibited the most potent anti-proliferative effects on HepG2 cells in a dose-dependent manner among the 18 compounds tested. At a concentration of 100 μM, these compounds demonstrated a reduction of 33.8% and 30.5% in viability after 24 hours, and 29.9% and 21.5, after 48 hours (Fig. 2).
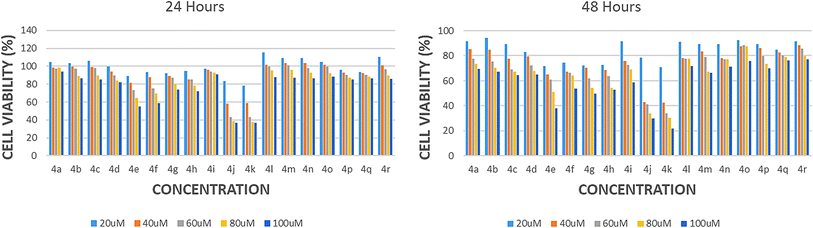 |
| Fig. 2 Histogram illustrating the survival of HepG2 cells at 24- and 48- hours after treatment with 4(a-r) compounds. | |
3.2. In vitro antioxidant activity
All the tri-substituted methane derivatives 4(a-r) were tested for their in vitro antioxidant (DPPH and ABTS) activity as seen in Table 3. All the compounds show good antioxidant activity among them compounds 4e, 4i, 4j, 4o and 4r showed outstanding ABTS and DPPH radical scavenging capabilities than standard (ABTS, IC50 = 60.2 ± 0.25 μM; DPPH, IC50 = 12.65 ± 0.74 μM).
Table 3 In vitro ABTS and DPPH Radical Scavenging Activities of 5-((2-aminothiazol-5-yl)(phenyl)methyl)-6-hydroxypyrimidine-2,4(1H,3H)-dione derivative 4(a-r)
Sr. no. |
Compound |
R1 |
ABTS radical scavenging activity |
DPPH radical scavenging activity |
IC50 ± SEMa (μM) |
IC50 ± SEMa (μM) |
SEM(Standard error mean). Standard for ABTS (2,2-azino-bis-3-ethylbenzothiazoline-6-sulphonic acid) and DPPH (2,2-diphenyl-1-picrylhydrazyl) radical scavenging activity. Bold values show the lowest IC50s. |
1 |
4a |
H |
78.93 ± 0.45 |
18.19 ± 0.87 |
2 |
4b |
Cl |
78.71 ± 0.67 |
20.09 ± 0.56 |
3 |
4c |
Br |
81.69 ± 0.34 |
24.81 ± 1.34 |
4 |
4d |
F |
76.99 ± 0.65 |
18.4 ± 0.76 |
5 |
4e |
p-CH3 |
66.62 ± 0.87 |
09.25 ± 0.76 |
6 |
4f |
p-NO2 |
97.62 ± 0.34 |
24.06 ± 0.46 |
7 |
4g |
o-NO2 |
77.59 ± 0.87 |
26.05 ± 0.35 |
8 |
4h |
o-Cl |
74.41 ± 0.23 |
13.76 ± 0.65 |
9 |
4i |
m-OCH3 |
88.62 ± 0.54 |
09.77 ± 0.24 |
10 |
4j |
p-OH |
66.02 ± 0.85 |
04.19 ± 0.56 |
11 |
4k |
p-OCH3 |
78.79 ± 0.67 |
11.36 ± 0.75 |
12 |
4l |
p-Cl |
87.93 ± 0.56 |
19.22 ± 0.47 |
13 |
4m |
p-Br |
94.5 ± 0.78 |
12.9 ± 0.28 |
14 |
4n |
p-F |
84.26 ± 1.08 |
06.93 ± 0.84 |
15 |
4o |
p-CH3 |
64.87 ± 1.34 |
03.58 ± 0.52 |
16 |
4p |
p-NO2 |
80.74 ± 0.65 |
20.45 ± 0.64 |
17 |
4q |
o-Cl |
74.15 ± 0.76 |
16.62 ± 0.85 |
18 |
4r |
m-OH |
67.35 ± 0.48 |
06.06 ± 0.52 |
19 |
Ascorbic acid b |
67.2 ± 0.98 |
12.65 ± 0.84 |
3.3. Structure–activity relationship (SAR) for DPPH and ABTS radical scavenging activities
All the compounds show good to excellent antioxidant activity (ABTS and DPPH). At a para position with electron-donating groups containing compounds 4e (ABTS, IC50 = 66.62 ± 0.87 μM; DPPH, IC50 = 09.25 ± 0.76 μM), 4j (ABTS, IC50 = 66.02 ± 0.85 μM; DPPH, IC50 = 04.19 ± 0.56 μM) and 4o (ABTS, IC50 = 64.87 ± 1.34 μM; DPPH, IC50 = 03.58 ± 0.52 μM) were the most active scavengers against both ABTS and DPPH which showed excellent scavenging activities to standard ascorbic acid (ABTS, IC50 = 67.2 ± 0.98 μM; DPPH, IC50 = 12.65 ± 0.84 μM). Also, the presence of the OH group on the phenyl ring increased the antioxidant activities which shows in compounds 4j (ABTS, IC50 = 66.02 ± 0.85 μM; DPPH, IC50 = 04.19 ± 0.56 μM) and 4r (ABTS, IC50 = 67.35 ± 0.48 μM; DPPH, IC50 = 06.06 ± 0.52 μM). The parent analog 4a (ABTS, IC50 = 78.93 ± 0.45 μM; DPPH, IC50 = 18.19 ± 0.87 μM) without any substitution, showed weak antioxidant activity. The presence of electron-withdrawing groups at a para position such as chloro, bromo, fluoro and nitro shows the least antioxidant activity such as 4b (ABTS, IC50 = 78.71 ± 0.67 μM; DPPH, IC50 = 20.09 ± 0.56 μM), 4l (ABTS, IC50 = 87.93 ± 0.56 μM; DPPH, IC50 = 19.22 ± 0.47 μM), 4c (ABTS, IC50 = 81.69 ± 0.34 μM; DPPH, IC50 = 24.81 ± 1.34 μM), 4m (ABTS, IC50 = 94.5 ± 0.78 μM; DPPH, IC50 = 12.9 ± 0.28 μM), 4d (ABTS, IC50 = 76.99 ± 0.65 μM; DPPH, IC50 = 18.4 ± 0.76 μM), 4n (ABTS, IC50 = 84.26 ± 1.08 μM; DPPH, IC50 = 06.93 ± 0.84 μM), 4f (ABTS, IC50 = 97.62 ± 0.34 μM; DPPH, IC50 = 24.06 ± 0.46 μM) and 4p (ABTS, IC50 = 80.74 ± 0.65 μM; DPPH, IC50 = 20.45 ± 0.64 μM) respectively. The electron-withdrawing groups at the ortho position also show the least antioxidant activity 4h (ABTS, IC50 = 74.41 ± 0.23 μM; DPPH, IC50 = 13.76 ± 0.65 μM), 4q (ABTS, IC50 = 74.15 ± 0.76 μM; DPPH, IC50 = 16.62 ± 0.85 μM). The SAR study shows that electron-donating and methoxy groups attached to phenyl rings show excellent antioxidant activity rather than the electron-withdrawing group in the phenyl ring.
4. Conclusion
In conclusion, we successfully developed a GAP-assisted green synthetic protocol for the synthesis of diversely functionalized 5-((2-aminothiazol-5-yl)(phenyl)methyl)-6-hydroxypyrimidine-2,4(1H,3H)-dione derivatives via a multicomponent reaction of 2-aminothiazole, N′,N′-dimethyl barbituric acid/barbituric acid and aryl aldehyde in catalyst-free condition at 80 °C and ethanol as a solvent with a focus on a greener approach, shorter reaction time, good yields, mild condition, and no column chromatographic purifications. Moreover, all the newly synthesized compounds were screened for their in vitro antiproliferative and antioxidant activities. Two compounds 4j and 4k exhibited the most potent anti-proliferative effects on HepG2 cells. Five compounds 4e, 4i, 4j, 4o, and 4r were found most potent than standard ascorbic acid (ABTS and DPPH).
5. Experimental
5.1. Materials and apparatus
All reagents used in this synthesis were purchased from commercially available sources and used without any further purification. Melting points were resolute using the open capillary tube method and were uncorrected. NMR spectra (1H NMR & 13C NMR) were recorded on Bruker 500 MHz, JEOL 500 MHz, and JEOL 400 MHz NMR spectrometer using solvent peak as CDCl3/DMSO-d6 solvent. LCMS analyses were performed on an MS-Agilent 6120 quadrupole spectrometer and HRMS was determined on Waters Micro mass Q-Tof Micro 4000 quadrupole spectrometer. TLC analyses were performed on aluminium plates precoated with F254 silica gel 60.
5.2. General procedure for the synthesis of 2-aminothiazole-tethered tri-substituted methane derivative
In a dry round-bottom flask (50 ml), a mixture of 2-aminothiazole (1.0 mmol), barbituric acid or N,N′-dimethyl barbituric acid (1.0 mmol) and aromatic substituted aldehydes (1.0 mmol) in ethanol was stirred at 80 °C for 30 minutes and the reaction progress was monitored by TLC. After the reaction was completed, the solid precipitate was filtered out and washed thoroughly twice with aqueous ethanol (2 × 5 ml). All compounds were confirmed by NMR and mass spectroscopic methods.
5.2.1. 5-((2-Aminothiazol-5-yl)(4-chlorophenyl)methyl)-6-hydroxy-1,3-dimethylpyrimidine-2,4(1H,3H)-dione (4a). White solid, mp: 228–230 °C; 1H NMR (500 MHz, DMSO-d6) δ 12.33 (s, 1H, OH), 8.79 (s, 2H, NH2), 7.25–7.20 (m, 4H, ArH), 7.08 (s, 1H, ArH), 5.60 (s, 1H, CH), 3.06 (s, 6H, 2CH3); 13C NMR (126 MHz, DMSO-d6) δ 170.01, 161.65, 152.64, 143.12, 129.73, 129.19, 128.84, 127.34, 122.22, 85.84, 38.41, 26.81; HRMS(+ESI) 379.0652 [M + H]+.
5.2.2. 5-((2-Aminothiazol-5-yl)(phenyl)methyl)-6-hydroxy-1,3-dimethylpyrimidine-2,4(1H,3H)-dione (4b). Light pink solid, mp: 218–220 °C; 1H NMR (400 MHz, DMSO-d6) δ 12.37 (s, 1H, OH), 8.78 (s, 2H, NH2), 7.21–7.16 (m, 4H, ArH), 7.11–7.07 (m, 1H, ArH), 7.05 (s, 1H, ArH), 5.60 (s, 1H, CH), 3.06 (s, 6H, 2CH3); 13C NMR (101 MHz, DMSO-d6) δ 170.58, 162.32, 153.29, 144.61, 130.40, 128.11, 127.65, 125.81, 122.59, 86.63, 39.51, 27.48; HRMS(+ESI) 345.1059 [M + H]+.
5.2.3. 5-((2-Aminothiazol-5-yl)(4-bromophenyl)methyl)-6-hydroxy-1,3-dimethylpyrimidine-2,4(1H,3H)-dione (4c). Light pink solid, mp: 230–232 °C; 1H NMR (400 MHz, DMSO-d6) δ 12.41 (s, 1H, OH), 8.81 (s, 2H, NH2), 7.37 (d, J = 8.1 Hz, 2H, ArH), 7.13 (d, J = 8.4 Hz, 2H, ArH), 7.11 (s, 1H, CH), 5.57 (s, 1H, CH), 3.05 (s, 6H, 2CH3); 13C NMR (101 MHz, DMSO-d6) δ 170.65, 162.29, 153.26, 144.22, 130.92, 129.87, 129.60, 122.93, 118.83, 86.50, 39.05, 27.47; HRMS(+ESI) 423.0145 [M + H]+.
5.2.4. 5-((2-Aminothiazol-5-yl)(4-fluorophenyl)methyl)-6-hydroxy-1,3-dimethylpyrimidine-2,4(1H,3H)-dione (4d). Off white solid, mp: 214–216 °C; 1H NMR (400 MHz, DMSO-d6) δ 12.38 (s, 1H, OH), 8.79 (s, 2H, NH2), 7.22 (dd, J = 8.6, 5.8 Hz, 2H, ArH), 7.06 (s, 1H, CH), 7.00 (dd, J = 10.1, 7.7 Hz, 2H, ArH), 5.58 (s, 1H, CH), 3.05 (s, 6H, 2CH3); 13C NMR (101 MHz, DMSO-d6) δ 170.58, 162.27, 153.28, 140.68, 130.33, 129.44, 129.36, 122.60, 114.78, 114.57, 86.52, 38.92, 27.44; HRMS(+ESI) 363.0974 [M + H]+.
5.2.5. 5-((2-Aminothiazol-5-yl)(p-tolyl)methyl)-6-hydroxy-1,3-dimethylpyrimidine-2,4(1H,3H)-dione (4e). Light pink solid, mp: 218–220 °C; 1H NMR (400 MHz, DMSO-d6) δ 12.35 (s, 1H, OH), 8.75 (s, 2H, NH2), 7.07 (d, J = 7.8 Hz, 2H, ArH), 7.02 (s, 1H, CH), 6.98 (d, J = 7.9 Hz, 2H, ArH), 5.55 (s, 1H, CH), 3.05 (s, 6H, 2CH3), 2.22 (s, 3H, CH3); 13C NMR (101 MHz, DMSO-d6) δ 170.53, 162.25, 153.30, 141.59, 134.58, 130.78, 128.66, 127.58, 122.35, 86.66, 39.16, 27.44, 21.09; HRMS(+ESI) 359.1241 [M + H]+.
5.2.6. 5-((2-Aminothiazol-5-yl)(4-nitrophenyl)methyl)-6-hydroxy-1,3-dimethylpyrimidine-2,4(1H,3H)-dione (4f). Light yellow solid mp. 206–208 °C; 1H NMR (400 MHz, DMSO-d6) δ 12.38 (s, 1H, OH), 8.79 (s, 2H, NH2), 7.26–7.17 (m, 2H, ArH), 7.06 (s, 1H, CH), 7.04–6.93 (m, 2H, ArH), 5.58 (s, 1H, CH), 3.05 (s, 6H, 2CH3). 13C NMR (101 MHz, DMSO-d6) δ 170.08, 161.77, 152.77, 140.18, 129.83, 128.94, 128.86, 122.10, 114.28, 114.07, 86.02, 38.42, 26.94; HRMS(+ESI) 390.0909 [M + H]+.
5.2.7. 5-((2-Aminothiazol-5-yl)(2-nitrophenyl)methyl)-6-hydroxy-1,3-dimethylpyrimidine-2,4(1H,3H)-dione (4g). Light yellow solid, mp: 200–202 °C; 1H NMR (400 MHz, DMSO-d6) δ 12.38 (s, 1H, OH), 8.86 (s, 2H, NH2), 7.87–7.82 (m, 1H, ArH), 7.64 (dd, J = 8.0, 1.4 Hz, 1H, ArH), 7.54 (td, J = 7.6, 1.4 Hz, 1H, ArH), 7.38 (td, J = 7.7, 1.4 Hz, 1H, ArH), 6.76 (s, Hz, 1H, CH), 5.93 (s, 1H, CH), 3.02 (s, 6H, 2CH3); 13C NMR (101 MHz, DMSO-d6) δ 170.00, 162.24, 153.22, 149.82, 137.73, 132.44, 131.49, 129.79, 127.74, 123.67, 122.41, 86.81, 35.64, 27.47; HRMS(+ESI) 390.0909 [M + H]+.
5.2.8. 5-((2-Aminothiazol-5-yl)(2-chlorophenyl)methyl)-6-hydroxy-1,3-dimethylpyrimidine-2,4(1H,3H)-dione (4h). White solid, mp: 234–236 °C; 1H NMR (400 MHz, DMSO-d6) δ 12.30 (s, 1H, OH), 8.79 (s, 2H, NH2), 7.79 (dd, J = 7.5, 2.1 Hz, 1H, ArH), 7.31 (dd, J = 7.4, 1.9 Hz, 1H, ArH), 7.19 (pd, J = 7.3, 1.9 Hz, 2H, ArH), 6.63 (s, 1H, CH), 5.84 (s, 1H, CH), 3.04 (s, 6H, 2CH3); 13C NMR (101 MHz, DMSO-d6) δ 169.78, 162.43, 153.30, 141.74, 133.19, 131.84, 131.03, 129.25, 128.32, 127.16, 121.76, 86.65, 37.50, 27.50; HRMS(+ESI) 379.0652 [M + H]+.
5.2.9. 5-((2-Aminothiazol-5-yl)(3-methoxyphenyl)methyl)-6-hydroxy-1,3-dimethylpyrimidine-2,4(1H,3H)-dione (4i). White solid, mp: 218–220 °C; 1H NMR (400 MHz, DMSO-d6) δ 12.36 (s, 1H, OH), 8.76 (s, 2H, NH2), 7.11 (t, J = 7.9 Hz, 1H, ArH), 7.04 (s, 1H, CH), 6.82–6.73 (m, 2H, ArH), 6.69 (dd, J = 8.2, 2.6 Hz, 1H, ArH), 5.56 (s, 1H, CH), 3.66 (s, 3H, CH3), 3.05 (s, 6H, 2CH3); 13C NMR (101 MHz, DMSO-d6) δ 170.55, 162.29, 159.38, 153.29, 146.37, 130.35, 129.06, 122.57, 120.24, 114.25, 110.39, 86.54, 56.56, 55.34, 27.45, 19.10; HRMS(+ESI) 375.1210 [M + H]+
5.2.10. 5-((2-Aminothiazol-5-yl)(4-hydroxyphenyl)methyl)-6-hydroxy-1,3-dimethylpyrimidine-2,4(1H,3H)-dione (4j). White solid, mp: 216–218 °C; 1H NMR (500 MHz, DMSO-d6) δ 12.31 (s, 1H, OH), 9.05 (s, 1H, OH), 8.72 (s, 2H, NH2), 7.01 (d, J = 8.2 Hz, 2H, ArH), 6.93 (s, 1H, CH), 6.58 (d, J = 8.2 Hz, 2H, ArH), 5.48 (s, 1H, CH), 3.05 (s, 6H, 2CH3); 13C NMR (101 MHz, DMSO-d6) δ 169.83, 161.70, 155.07, 152.74, 134.18, 131.15, 128.23, 121.44, 114.31, 86.26, 38.37, 26.89; LCMS(+ESI) 360.90 [M + H]+.
5.2.11. 5-((2-Aminothiazol-5-yl)(4-methoxyphenyl)methyl)-6-hydroxy-1,3-dimethylpyrimidine-2,4(1H,3H)-dione (4k). White solid, mp: 220–222 °C; 1H NMR (500 MHz, DMSO-d6) δ 12.34 (s, 1H), 8.74 (s, 2H), 7.12 (dd, J = 7.6, 1.5 Hz, 2H), 6.99 (s, 1H), 6.83–6.71 (m, 2H), 5.53 (s, 1H), 3.68 (s, 3H), 3.05 (s, 7H); 13C NMR (101 MHz, DMSO-d6) δ 169.90, 161.69, 157.14, 152.75, 136.01, 130.77, 128.23, 121.56, 112.95, 86.13, 54.93, 38.35, 26.86; HRMS(+ESI) 375.1210 [M + H]+.
5.2.12. 5-((2-aminothiazol-5-yl)(4-chlorophenyl)methyl)-6-hydroxypyrimidine-2,4(1H,3H)-dione (4l). Light pink solid, mp: 228–230 °C; 1H NMR (400 MHz, DMSO-d6) δ 9.43 (s, 2H, NH), 8.77 (m, 2H, NH2), 7.27–7.20 (m, 4H, ArH), 7.02 (s, 1H, ArH), 5.45 (s, 1H, CH); 13C NMR (126 MHz, DMSO-d6) δ 170.59, 164.32, 152.41, 143.77, 130.39, 130.25, 129.66, 127.97, 123.22, 86.03, 37.92; HRMS(+ESI) 351.0325 [M + H]+.
5.2.13. 5-((2-Aminothiazol-5-yl)(4-bromophenyl)methyl)-6-hydroxypyrimidine-2,4(1H,3H)-dione (4m). Light pink solid, mp: 226–228 °C; 1H NMR (400 MHz, DMSO-d6) δ 9.39 (s, 2H, NH), 8.75 (s, 2H, NH2), 7.38 (d, J = 8.1 Hz, 2H, ArH), 7.17 (d, J = 8.2 Hz, 2H, ArH), 7.02 (s, 1H, ArH), 5.43 (s, 1H, CH); 13C NMR (126 MHz, DMSO-d6) δ 170.59, 164.32, 152.42, 144.24, 130.88, 130.09, 123.29, 118.86, 86.00, 37.97; HRMS(+ESI) 394.9854 [M + H]+.
5.2.14. 5-((2-Aminothiazol-5-yl)(4-fluorophenyl)methyl)-6-hydroxypyrimidine-2,4(1H,3H)-dione (4n). Off white solid, mp: 222–224 °C; 1H NMR (400 MHz, DMSO-d6) δ 9.40 (s, 2H, NH), 8.76 (s, 2H, NH2), 7.22–7.18 (m, 2H, ArH), 6.99 (d, J = 10 Hz, 2H, ArH), 6.96 (s, 1H, ArH), 5.41 (s, 1H, CH); 13C NMR (126 MHz, DMSO-d6) δ 170.50, 164.32, 159.96, 152.41, 140.74, 130.86, 129.64, 129.58, 123.02, 114.72, 114.55, 86.18, 37.84; HRMS(+ESI) 335.0681 [M + H]+.
5.2.15. 5-((2-Aminothiazol-5-yl)(p-tolyl)methyl)-6-hydroxypyrimidine-2,4(1H,3H)-dione (4o). Off white solid, mp: 218–220 °C; 1H NMR (400 MHz, DMSO-d6) δ 9.29 (s, 2H, NH), 8.65 (s, 2H, NH2), 7.10 (d, J = 7.8 Hz, 2H, ArH), 7.00 (d, J = 7.8 Hz, 2H, ArH), 6.90 (s, 1H, ArH), 5.41 (s, 1H, CH), 2.22 (s, 3H, CH3); 13C NMR (126 MHz, DMSO-d6) δ 170.43, 164.29, 152.41, 141.60, 134.63, 131.25, 128.64, 127.80, 122.88, 86.31, 38.08, 21.10; HRMS(+ESI) 331.0918 [M + H]+.
5.2.16. 5-((2-Aminothiazol-5-yl)(4-nitrophenyl)methyl)-6-hydroxypyrimidine-2,4(1H,3H)-dione (4p). Yellow solid, mp: 226–228 °C; 1H NMR (400 MHz, DMSO-d6) δ 9.33 (s, 2H, NH), 8.82–8.80 (m, 2H, NH2), 8.06 (d, J = 8.5 Hz, 2H, ArH), 7.42 (d, J = 8.5 Hz, 2H, ArH), 7.14 (s, 1H, ArH), 5.57 (s, 1H, CH); 13C NMR (126 MHz, DMSO-d6) δ 170.77, 164.33, 153.43, 152.43, 145.93, 128.95, 128.77, 123.79, 123.40, 86.17, 38.50; HRMS(+ESI) 362.0602 [M + H]+.
5.2.17. 5-((2-aminothiazol-5-yl)(2-chlorophenyl)methyl)-6-hydroxypyrimidine-2,4(1H,3H)-dione (4q). Off white solid, mp: 252–254 °C; 1H NMR (500 MHz, DMSO-d6) δ 9.15 (s, 2H), 8.61 (s, 2H), 7.79 (dd, J = 7.4, 2.3 Hz, 1H), 7.28 (dd, J = 7.2, 2.0 Hz, 1H), 7.16 (qt, J = 7.3, 3.5 Hz, 2H), 6.51 (s, 1H), 5.67 (s, 1H); 13C NMR (126 MHz, DMSO-d6) δ 169.69, 164.43, 152.36, 141.76, 133.16, 131.99, 131.06, 129.20, 128.34, 127.14, 86.55, 36.34; HRMS(+ESI) 351.0325 [M + H]+.
5.2.18. 5-((2-Aminothiazol-5-yl)(3-hydroxyphenyl)methyl)-6-hydroxypyrimidine-2,4(1H,3H)-dione (4r). Pink solid, mp: 246–248 °C; 1H NMR (400 MHz, DMSO-d6) δ 9.24 (s, 2H, NH), 9.08 (s, 1H, OH), 8.63 (s, 2H, NH2), 6.93 (d, J = 8.7 Hz, 2H, ArH), 6.65 (s, 1H, ArH), 6.58 (dd, J = 7.6, 1.5 Hz, 1H, ArH), 6.46 (d, J = 8.0 Hz, 1H, ArH), 5.35 (s, 1H, CH); 13C NMR (126 MHz, DMSO-d6) δ 170.50, 164.30, 157.38, 152.43, 146.20, 130.86, 128.83, 123.06, 118.58, 114.86, 112.80, 86.27, 38.30; HRMS(+ESI) 333.0706 [M + H]+.
5.3. ABTS radical scavenging assay
The ABTS free radical cation scavenging activity of the compounds was performed using a standard method. First, a 7 mM concentrated solution of ABTS was prepared, and then, a 2.45 mM concentrated solution of potassium persulfate was added to the ABTS solution. This mixture was kept in a dark place at room temperature for 14–16 h. The test compound sample solutions were prepared in absolute alcohol at concentrations ranging from 0.01 to 1 mg ml−1. The test sample was added to the ABTS solutions and incubated for 30 min at 37 °C. The absorbance was measured at a wavelength of 734 nm, and the procedure was repeated for ascorbic acid as a reference standard. The % inhibition of radical scavenging activity was determined using the given formula.
5.4. DPPH radical scavenging assay
The free radical scavenging activity of DPPH was tested using a standard procedure. 0.3 mM DPPH concentrated solution in ethanol incubated for 30 min at 37 °C with various test samples. The absorption of the sample was taken at 517 nm. The same procedure was followed for ascorbic acid as the standard. The % inhibition of radical scavenging activity was determined using the given formula.
Conflicts of interest
The authors declare no conflict of interest.
Acknowledgements
The authors P. J. P. and S. G. P. are grateful to the University Grants Commission of India (UGC), New Delhi, for the award junior research fellowship (File No. 16-9 (June 2019)/2019 (NET/CSIR); dated 10.06.2020) and (NTA Ref. no. 201610157514; dated 01.04.2021), respectively. The author is also grateful to the Authority of the Department of Chemistry, Sardar Patel University, Gujarat- 388120, India, for providing laboratory facilities.
References
- A. M. Pisoschi and A. Pop, Eur. J. Med. Chem., 2015, 97, 55–74 CrossRef CAS PubMed.
- A. Lozynskyi, V. Zasidko, D. Atamanyuk, D. Kaminskyy, H. Derkach, O. Karpenko, V. Ogurtsov, R. Kutsyk and R. Lesyk, Mol. Diversity, 2017, 21, 427–436 CrossRef CAS PubMed.
- E. N. Bentz, A. B. Pomilio and R. M. Lobayan, Comput. Theor. Chem., 2017, 1110, 14–24 CrossRef CAS.
- Y. Dai, C. Shao, Y. Piao, H. Hu, K. Lu, T. Zhang, X. Zhang, S. Jia, M. Wang and S. Man, Carbohydr. Polym., 2017, 178, 34–40 CrossRef CAS PubMed.
- H. Razzaq, F. Saira, A. Yaqub, R. Qureshi, M. Mumtaz and S. Saleemi, J. Photochem. Photobiol., B, 2016, 161, 266–272 CrossRef CAS PubMed.
- F. Sonmez, Z. Gunesli, B. Z. Kurt, I. Gazioglu, D. Avci and M. Kucukislamoglu, Mol. Diversity, 2019, 23, 829–844 CrossRef CAS PubMed.
- J. D. Trinity, R. M. Broxterman and R. S. Richardson, Free Radical Biol. Med., 2016, 98, 90–102 CrossRef CAS PubMed.
- P. J. Patel, D. M. Patel, R. M. Vala, S. G. Patel, D. B. Upadhyay, Y. Pannerselvam and H. M. Patel, ACS Omega, 2023, 8, 444–456 CrossRef CAS PubMed.
- W. Si, Y. P. Chen, J. Zhang, Z.-Y. Chen and H. Y. Chung, Food Chem., 2018, 239, 1117–1125 CrossRef CAS PubMed.
- P. Sivaguru, K. Parameswaran and A. Lalitha, Mol. Diversity, 2017, 21, 611–620 CrossRef CAS PubMed.
- D. B. Upadhyay, R. M. Vala, S. G. Patel, P. J. Patel, C. Chi and H. M. Patel, J. Mol. Struct., 2023, 1273, 134305 CrossRef CAS.
- D. M. Patel, M. G. Sharma, R. M. Vala, I. Lagunes, A. Puerta, J. M. Padrón, D. P. Rajani and H. M. Patel, Bioorg. Chem., 2019, 86, 137–150 CrossRef CAS PubMed.
- S. G. Patel, A. González-Bakker, R. M. Vala, P. J. Patel, A. Puerta, A. Malik, R. K. Sharma, J. M. Padrón and H. M. Patel, RSC Adv., 2022, 12, 30404–30415 RSC.
- H. M. Patel, Curr. Bioact. Compd., 2018, 14, 278–288 CrossRef CAS.
- H. M Patel, K. D Patel and H. D Patel, Curr. Bioact. Compd., 2017, 13, 47–58 CrossRef.
- H. M. Patel, Green Sustainable Chem., 2015, 5, 137 CrossRef CAS.
- P. J. Patel, R. M. Vala, S. G. Patel, D. B. Upadhyay, D. P. Rajani, F. Damiri, M. Berrada and H. M. Patel, J. Mol. Struct., 2023, 1285, 135467 CrossRef CAS.
- H. M. Patel, D. P. Rajani, M. G. Sharma and H. G. Bhatt, Lett. Drug Des. Discovery, 2019, 16, 119–126 CrossRef CAS.
- D. M. Patel, H. J. Patel, J. M. Padrón and H. M. Patel, RSC Adv., 2020, 10, 19600–19609 RSC.
- D. M. Patel, R. M. Vala, M. G. Sharma, D. P. Rajani and H. M. Patel, ChemistrySelect, 2019, 4, 1031–1041 CrossRef CAS.
- D. M. Patel, P. J. Patel and H. M. Patel, Eur. J. Org. Chem., 2022, 2022, e202201119 CrossRef CAS.
- D. M. Patel and H. M. Patel, ACS Sustainable Chem. Eng., 2019, 7, 18667–18676 CrossRef CAS.
- D. P. Vala, R. M. Vala and H. M. Patel, ACS Omega, 2022, 7, 36945–36987 CrossRef CAS PubMed.
- T. Iino, D. Tsukahara, K. Kamata, K. Sasaki, S. Ohyama, H. Hosaka, T. Hasegawa, M. Chiba, Y. Nagata, J.-i. Eiki and T. Nishimura, Biorg. Med. Chem., 2009, 17, 2733–2743 CrossRef CAS PubMed.
- M. Pieroni, B. Wan, S. Cho, S. G. Franzblau and G. Costantino, Eur. J. Med. Chem., 2014, 72, 26–34 CrossRef CAS PubMed.
- N. Siddiqui and W. Ahsan, Med. Chem. Res., 2011, 20, 261–268 CrossRef CAS.
- E. Khan, A. Khan, Z. Gul, F. Ullah, M. N. Tahir, M. Khalid, H. M. Asif, S. Asim and A. A. C. Braga, J. Mol. Struct., 2020, 1200, 127126 CrossRef.
- M. Juhás, A. Bachtíková, D. E. Nawrot, P. Hatoková, V. S. Pallabothula, A. Diepoltová, O. Janďourek, P. Bárta, K. Konečná, P. Paterová, V. Šesták and J. Zitko, Pharmaceuticals, 2022, 15(5), 580 CrossRef PubMed.
- S. Ghaemmaghami, C. H. May Barnaby, R. Renslo Adam and B. Prusiner Stanley, J. Virol., 2010, 84, 3408–3412 CrossRef CAS PubMed.
- M. Dobbelstein and U. Moll, Nat. Rev. Drug Discovery, 2014, 13, 179–196 CrossRef CAS PubMed.
- R. S. Giri, H. M. Thaker, T. Giordano, J. Williams, D. Rogers, V. Sudersanam and K. K. Vasu, Eur. J. Med. Chem., 2009, 44, 2184–2189 CrossRef CAS PubMed.
- T. A. Yousef, G. M. Abu El-Reash, O. A. El-Gammal and B. M. Sharaa, Egypt. J. Basic Appl. Sci., 2016, 3, 44–60 Search PubMed.
- X. Zhou, L. Shao, Z. Jin, J.-B. Liu, H. Dai and J.-X. Fang, Heteroat. Chem., 2007, 18, 55–59 CrossRef CAS.
- A. Barakat, H. A. Ghabbour, A. M. Al-Majid, a. Qurat ul, R. Imad, K. Javaid, N. N. Shaikh, S. Yousuf, M. Iqbal Choudhary and A. Wadood, J. Chem., 2016, 2016, 8517243 Search PubMed.
- A. M. Al-Majid, A. Barakat, H. J. Al-Najjar, Y. N. Mabkhot, H. A. Ghabbour and H.-K. Fun, Int. J. Mol. Sci., 2013, 14, 23762–23773 CrossRef PubMed.
- D. Das, P. Sikdar and M. Bairagi, Eur. J. Med. Chem., 2016, 109, 89–98 CrossRef CAS PubMed.
|
This journal is © The Royal Society of Chemistry 2023 |