DOI:
10.1039/D3RA02475J
(Paper)
RSC Adv., 2023,
13, 14698-14702
Facile synthesis of chiral phenylselenides as novel antioxidants and cytotoxic agents†
Received
13th April 2023
, Accepted 8th May 2023
First published on 15th May 2023
Abstract
Organoselenium compounds are well-known for their unique biological properties, including antioxidant, anticancer and anti-inflammatory. They result from the presence of a particular Se-moiety enclosed in a structure that provides physicochemical features necessary for effective drug–target interactions. Looking for a proper drug design that considers the influence of each structural element has to be conducted. In this paper, we have synthesized a series of chiral phenylselenides, possessing an additional N-substituted amide moiety, and evaluated their antioxidant and anticancer potential. The presented derivatives, as a group of enantiomeric and diastereomeric pairs, enabled a thorough investigation of the 3D structure–activity dependence in correlation with the presence of the phenylselanyl group as the potential pharmacophore. The N-indanyl derivatives possessing a cis- and trans-2-hydroxy group were selected as the most promising antioxidants and anticancer agents.
Introduction
Over the years, organoselenium chemistry has expanded through a multitude of research fields including medicine, catalysis and materials science. Examples of its diverse applicability include antibacterial1 and redox-responsive materials;2 utility as catalysts in oxidation, reduction and rearrangement reactions, as well as stereoselective reactions;3 and in dietary supplementation as an essential micronutrient.4 Taking into consideration the nutritional and biochemical properties of naturally abundant organoselenium compounds like selenocysteine, selenomethionine and selenium as an element itself, one of the leading directions of development in the field is medicinal chemistry. As one of the most evaluated small antioxidant molecules, organoselenium compounds can be considered leading players in the race for market-approved drugs. The ability to mimic the antioxidant selenoenzyme glutathione peroxidase (GPx) determines its high redox-modulating properties.5 Over the years, they have also been proven to exhibit various biological activities including anticancer, anti-inflammatory, and antibacterial.6–11 However, issues associated with bioavailability and side effects remain major obstacles in the design of safe Se-therapeutics.
Since the discovery of the well-known peroxide scavenger ebselen (N-phenylbenzisoselenazol-3(2H)-one) a lot has been accomplished in the search for the ideal structural modifications that would maximize the therapeutic potential of the Se-moiety and reduce its toxicity.12–16 Modifying the primary benzisoselenazolone core can significantly affect the physicochemical properties of the molecule, thus changing the pharmacokinetics and biochemical outcome of the potential drug. We have recently observed that in some cases, the exchange of the Se–N for a Se–Car bond and incorporation of an additional aromatic ring enhances the cytotoxic activity of chiral N-terpene benzisoselenazolones 1 and 3.17 The IC50 values decreased approximately six and twelve times for N-menthyl 2 and N-caranyl phenylselenide 4, respectively (Fig. 1).
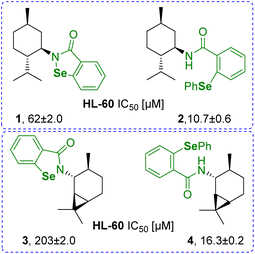 |
| Fig. 1 N-terpene phenylselenides with enhanced bio-activity. | |
These results prompted us to investigate if the presence of the phenylselanyl group can enhance the antiproliferative effect. There are few methods that enable to obtain unsymmetrical phenylselenides with a secondary amide group: starting from corresponding benzisoselenazolones through the reaction with Grignard reagent (method A);18 based on the Ru-catalyzed selenation of benzamides (method B);19 and Cu-catalyzed nucleophilic substitution of o-iodobenzamides (method C), previously reported by our research group17 (Scheme 1). Herein, we have utilized one of the known protocols to synthesize a series of chiral unsymmetrical diphenyl selenides and evaluated their antioxidant and anticancer activity. The presented derivatives are pairs of enantiomers and diastereoisomers, which additionally allows determining whether the molecule's spatial structure can, in this case, influence the drug–target interaction and increase the therapeutic potential.
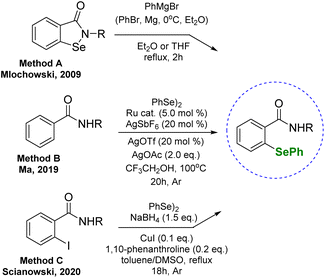 |
| Scheme 1 Methods for the preparation of diphenylselenides monosubstituted with o-amido function. | |
Results and discussion
The first stage of the research involved the synthesis of phenylselenides 6–19. For this purpose, we have slightly modified the procedure proposed by Młochowski and co-workers (Method A, Scheme 1). To a solution of phenyl magnesium bromide, formed by refluxing PhBr and Mg0 in diethyl ether, appropriate N-substituted benzisoselenazolone 5 (obtained previously through the reaction of 2-(chloroseleno)benzoyl chloride with an appropriate enantiomerically pure amine20) dissolved in Et2O or THF was added (Scheme 2).
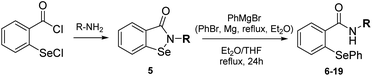 |
| Scheme 2 Synthesis of phenylselenides 6–19. | |
Due to low solubility of the starting materials 5, the reaction time had to be elongated to 24 h. All products 6–19 were obtained in good yields (Scheme 3). Surprisingly, our recently reported protocol (Method C, Scheme 1) was not efficient for these type of bulky substrates, e.g. compound 15 was obtained in only 38% yield, contrary to Method A which furnished the final phenylselenide 15 in 92%.
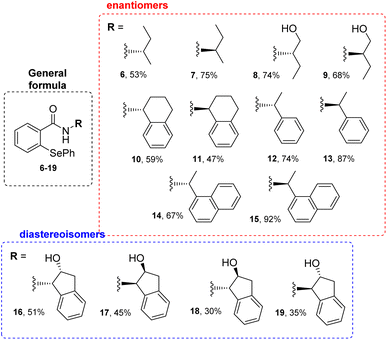 |
| Scheme 3 Synthesized phenylselenides 6–19. | |
The antioxidant activity of all synthesized compounds was evaluated by two assays. First, by the procedure presented by Iwaoka and co-workers.21 Herein, the particular selenide 6–19, serving as the Se-catalyst, is first oxidized by hydrogen peroxide to the corresponding selenooxide 20. Then, the activated catalyst 20 transforms the dithiol DTTred to the disulfide DTTox. The progress of the reaction, disappearance of signals correlated with DTTred and appearance of those associated with DTTox, is monitored by the changes in 1H NMR spectra in specific time intervals. Unfortunately, in all cases, the conversion was less efficient than in the presence of ebselen (Table 1).
Table 1 Results of the antioxidant activity measurement
Se-catalyst [0.1 eq.] |
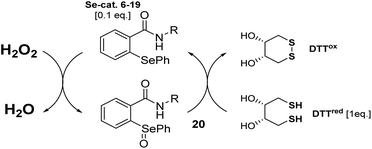
|
Remaining DTTred [%] |
5 min |
15 min |
30 min |
60 min |
Phenylselenides |
6/7 |
87 |
86 |
86 |
85 |
8/9 |
91 |
85 |
81 |
76 |
10/11 |
88 |
84 |
79 |
75 |
12/13 |
88 |
85 |
83 |
83 |
14/15 |
89 |
86 |
84 |
82 |
16/17 |
87 |
85 |
83 |
81 |
18/19 |
88 |
85 |
83 |
80 |
Ebselen |
75 |
64 |
58 |
52 |
In the second study, the popular 2,2′-diphenyl-1-picrylhydrazyl (DPPH˙) radical scavenging assay (RSA) was used to measure the total antioxidant capacity for all obtained compounds. It has been applied to quantify antioxidant activity in many samples using antioxidant standards such as ascorbic acid, butylated hydroxyl toluene, a-tocopherol, butylated hydroxyl anisole, gallic acid, and trolox.22 The results of this assay were expressed as IC50 values (Table 2). The IC50 value is defined as the antioxidant concentration required to reduce 50% of the initial DPPH concentration.23 The percentage of inhibition against the DPPH radical was calculated with the following equation: inhibition (%) = ((A − B)/A) × 100, where A and B are the absorptions of the blank samples and working standards. The DPPH˙ test is based on spectrophotometric mixed mode assays based on single electron transfer (SET), hydrogen atom transfer (HAT), and proton-coupled electron transfer (PCET) mechanisms. The DPPH radical can only be dissolved in organic media thus, this assay has a higher affinity toward lipophilic than hydrophilic antioxidants. The highest antioxidant capacity was observed for the trans-indanyl diastereoisomers 18/19. Surprisingly, the cis-indanyl conformers 16/17 were approximately 4 times less active.
Table 2 Results collected from the DPPH radical scavenging assay
Compound |
IC50DPPH [μmol L−1] |
6/7 |
7149.2 |
8/9 |
1752.6 |
10/11 |
1714.3 |
12/13 |
1715.1 |
14/15 |
1704.7 |
16/17 |
6470.4 |
18/19 |
1429.3 |
Next, the antiproliferative activity of derivatives 6–19 was evaluated towards leukemia HL-60 and breast cancer MCF-7 cell lines.24 The highest cytotoxic potential was observed for the indanyl phenylselenides possessing the cis (17) and trans (18, 19) 2-hydroxy group (Table 3).
Table 3 The antiproliferative activity of compounds 6–19
Compound |
IC50 [μM] ± SEM |
HL-60 |
MCF-7 |
6 |
>150 |
>150 |
7 |
>150 |
>150 |
8 |
>150 |
>150 |
9 |
>150 |
>150 |
10 |
>150 |
>150 |
11 |
>150 |
>150 |
12 |
>150 |
>150 |
13 |
>150 |
>150 |
14 |
>150 |
>150 |
15 |
>150 |
>150 |
16 |
>150 |
>150 |
17 |
>150 |
16.65 ± 1.36 |
18 |
69.50 ± 0.71 |
83.38 ± 1.94 |
19 |
55.45 ± 3.01 |
63.75 ± 0.35 |
As presented in our previous papers,20 the presence of the indanyl substituent attached to the nitrogen atom of benzisoselenazolones and corresponding diselenides also enhances the cytotoxic effect. A comparison of all 3 types of indanyl Se-derivatives is presented in Table 4. Benzisoselenazolones (A–D) show a moderate cytotoxic activity and it is similar towards both tested cell lines with IC50 values ranging from 7.9 ± 0.3 to 28.8 ± 2.1 μM. In the case of all diselenides (A–D), the cytotoxicity against MCF-7 cells is low (IC50 > 100 μM), whereas HL-60 cell line is far more sensitive (IC50 < 20 μM).
Table 4 The antiproliferative activity of N-indanyl Se-derivatives
|

|
A |
B |
Se-derivative |
MCF-7 |
HL-60 |
MCF-7 |
HL-60 |
Phenylselenide |
>150 |
>150 |
16.6 ± 1.3 |
>150 |
Benzisoselenazolone |
14.9 ± 0.9 |
7.9 ± 0.3 |
28.8 ± 2.1 |
28.2 ± 1.1 |
Diselenide |
>100 |
20.0 ± 0.1 |
>100 |
21.7 ± 2.1 |
![[thin space (1/6-em)]](https://www.rsc.org/images/entities/char_2009.gif) |
|
C |
D |
Se-derivative |
MCF-7 |
HL-60 |
MCF-7 |
HL-60 |
Phenylselenide |
83.3 ± 1.9 |
69.5 ± 0.7 |
63.7 ± 0.3 |
55.4 ± 3.0 |
Benzisoselenazolone |
22.1 ± 3.1 |
11.4 ± 1.0 |
27.6 ± 2.1 |
16.2 ± 1.8 |
Diselenide |
>100 |
12.4 ± 0.3 |
>100 |
13.0 ± 0 |
Phenylselenides with a trans-hydroxy group (C and D) on the indanyl scaffold present similar low activity to both cell lines, however, in the case of cis-hydroxy-indanyl derivative B the MCF-7 cell line is inhibited more effectively (IC50 = 16.7 ± 1.4 μM). Thus, bio-selectivity seems to be strongly related to the type of the Se-moiety, regardless of the chirality of the indanyl substituent.
Conclusions
In conclusion, we have developed a facile synthesis of chiral unsymmetrical diphenylselenides possessing an additional o-amide group on one of the aromatic rings. The derivatives were N-substituted with chiral groups forming a series of enantiomeric and diastereomeric pairs. Although the phenylselanyl group seems not to be improving the bio-activity of the organoselenium derivatives, in contrary to corresponding benzisoselenazolones with Se–N moiety and diselenides equipped with a Se–Se bond, the obtained results confirm that also for these type of Se-derivatives, the presence of the N-indanyl scaffold enhance the antioxidant and anticancer properties. Thus, the cis- and trans-indanyl moiety can be considered a pharmacophore necessary for the optimal drug–target interaction.
Experimental
General
NMR spectra were recorded on Bruker Avance III/400 or Bruker Avance III/700 (Karlsruhe, Germany) for 1H and 176.1 MHz or 100.6 MHz for 13C (see ESI†). Chemical shifts were recorded relative to SiMe4 (δ 0.00) or solvent resonance (CDCl3 δ 7.26, CD3OD δ 3.31). Multiplicities were given as: s (singlet), d (doublet), dd (double doublet), ddd (double double doublet), t (triplet), dt (double triplet), and m (multiplet). The 77Se NMR spectra were recorded on Bruker Avance III/400 or Bruker Avance III/700 with diphenyl diselenide as an external standard. NMR spectra were carried out using ACD/NMR Processor Academic Edition. Melting points were measured with a Büchi Tottoli SPM-20 heating unit (Büchi Labortechnik AG, Flawil, Switzerland) and were uncorrected. Elemental analyses were performed on a Vario MACRO CHN analyzer. Optical rotations were measured in 10 mm cells with a polAAr 3000 polarimeter. Column chromatography was performed using Sigma Aldrich 60 Å (52–73 A) 63–200 μm silica gel (Merck, Darmstadt, Germany).
General procedure for the synthesis of phenylselenides 6–19
To a suspension of elemental magnesium in diethyl ether, phenyl bromide was slowly added (during this time, the mixture was continuously heated to 40 °C). The reaction mixture was stirred and refluxed until all magnesium was dissolved (≈2 h) at 40 °C under anaerobic conditions. Appropriate N-substituted benzisoselenazol-3(2H)-one, dissolved in diethyl ether or THF THF (Et2O: 6, 7, 8, 9, 12, 13, 14, 15; THF: 10, 11, 16, 17, 18, 19) was slowly added. The reaction was stirred at 40 °C for 24 h, poured on water, and extracted with DCM. The combined organic layers were dried over anhydrous magnesium sulfate and evaporated. The crude product was purified by column chromatography (silica gel, DCM). Characterization of all obtained products is presented in ESI file.†
Antioxidant activity assays
DTT-assay. To a solution of compounds 6–19 (0.015 mmol) and dithiothreitol DTTred (0.15 mmol) in 1.1 mL of CD3OD, 30% H2O2 (0.15 mmol) was added. 1H NMR spectra were measured right after the addition of hydrogen peroxide and then in specific time intervals. The concentration of the substrate was determined according to the changes in the integration on the 1H NMR spectra.21
The 2,2′-diphenyl-1-picrylhydrazyl (DPPH˙) method. The antioxidant capacity of each compound was determined by spectrophotometric DPPH˙ method. The discussed compounds 6–19 were dissolved in methanol (5 mL), next different values of the obtained solutions were added to 0.5 mL DPPH methanolic solution (0.30 mmol L−1) and made up to 2.5 mL with methanol. The mixture was shaken vigorously and left in darkness for 15 min. The absorbance was measured at 517 nm against a reagent blank (2 mL of methanol with 0.5 mL of DPPH methanolic solution) using a 1 cm quartz cell in a Shimadzu UV-1601 spectrophotometer.The 50% DPPH˙ inhibition (IC50DPPH) was calculated based on linear regression analyses (the relationship curve of %DPPH scavenging versus concentrations of the respective sample).
The calibration curve was prepared using working solutions of 6-hydroxy-2,5,7,8-tetramethylchromane-2-carboxylic acid (trolox) in methanol between 0.02–0.10 mmol mL−1 and resulted in the equation: %DPPH˙ = 0.5155CTE +1.8291.
MTT viability assay. The MTT (3-(4,5-dimethyldiazol-2-yl)-2,5 diphenyl tetrazolium bromide) assay, which measures the activity of cellular dehydrogenases, was based on the method of Mosmann.24 Briefly, cells were seeded into 24-well plates (about 8 × 104 cells per mL) and then left to adhere and grow for 24 h. Subsequently, the tested compounds in the medium were added to a final concentration of 0–150 μM for 48 h, followed by the addition of 100 μL MTT, 5 mg mL−1 in PBS, for the next 2 h. Then, the plates were centrifuged, the supernatant was discarded and remaining insoluble formazan crystals were dissolved in 1 mL DMSO. The absorbance of the blue formazan product was measured at 560 nm using FlexStation 3 Multi-Mode Microplate Reader (Molecular Devices, LLC, CA, USA) and compared with control (untreated cells). All experiments were performed three times in triplicate. The concentration of the tested compounds required to inhibit cell viability by 50% (IC50) was calculated from concentration–response curves.
Conflicts of interest
There are no conflicts to declare.
Acknowledgements
Financial support from the NCU research grant “Excellence Initiative – Debuts – III edition” number 03.01.00002702, Zad.6. IDUB/Debiuty_3_ and program for financing the costs of publication of doctoral students under the program "Initiative of excellence - Research University".
References
- M. Liu, X. Zhang, S. Chu, Y. Ge, T. Huang, Y. Liu and L. Yu, Chin. Chem. Lett., 2022, 33, 205 CrossRef CAS.
- Q. Li, Y. Zhang, Z. Chen, X. Pan, Z. Zhang, J. Zhu and X. Zhua, Org. Chem. Front., 2020, 7, 2815 RSC.
- F. V. Singh and T. Wirth, Catal. Sci. Technol., 2019, 9, 1073 RSC.
- C. M. Weekley and H. H. Harris, Chem. Soc. Rev., 2013, 42, 8870 RSC.
- M. Obieziurska-Fabisiak, A. J. Pacuła-Miszewska, A. Laskowska and J. Ścianowski, Arkivoc, 2022, v, 212 Search PubMed.
- J. Pacuła, F. Mangiavacchi, L. Sancineto, E. J. Lenardao, J. Ścianowski and C. Santi, Curr. Chem. Biol., 2015, 9, 97 Search PubMed.
- F. V. Singh and T. Wirth, Synthesis of Organoselenium Compounds with Potential Biological Activities, in Organoselenium Compounds in Biology and Medicine: Synthesis, Biological and Therapeutic Treatments, Royal Society of Chemistry, London, UK, 2017, p. 77 Search PubMed.
- H. Chuai, S. Q. Zhang, H. Bai, J. Li, Y. Wang, J. Sun, E. Wen, J. Zhang and M. Xin, Eur. J. Med. Chem., 2021, 223, 113621 CrossRef CAS PubMed.
- W. Hou, H. Dong, X. Zhang, Y. Wang, L. Su and H. Xu, Drug Discovery Today, 2022, 27, 2268 CrossRef CAS PubMed.
- L. Sancineto, A. Mariotti, L. Bagnoli, F. Marini, J. Desantis, N. Iraci, C. Santi, C. Pannecouque and O. J. Tabarrini, J. Med. Chem., 2015, 58, 9601 CrossRef CAS PubMed.
- D. Krasowska, N. Iraci, C. Santi, J. Drabowicz, M. Cieslak, J. Kaźmierczak-Barańska, M. Palomba, K. Królewska-Golińska, J. Magiera and L. Sancineto, Molecules, 2019, 24, 2914 CrossRef CAS PubMed.
- P. Santofimia-Castaño, A. Izquierdo-alvarez, I. Casa-resino, A. Martinez-ruiz, M. Perez-lopez, J. C. Portilla, G. M. Salido and A. Gonzalez, Toxicology, 2016, 74, 357 Search PubMed.
- G. K. Azad and R. Tomar, Mol. Biol. Rep., 2014, 41, 4865 CrossRef CAS PubMed.
- J. L. Benelli, V. R. Poester, L. S. Munhoz, A. M. Melo, M. R. Trapaga, D. A. Stevens and M. O. Xavier, Med. Mycol., 2021, 59, 409 CrossRef CAS PubMed.
- M. J. Parnham and H. Sies, Biochem. Pharmacol., 2013, 86, 1248 CrossRef CAS PubMed.
- C. Santi, C. Scimmi and L. Sancineto, Molecules, 2021, 26, 4230 CrossRef CAS PubMed.
- M. Obieziurska-Fabisiak, A. J. Pacuła, L. Capoccia, J. Drogosz-Stachowicz, A. Janecka, C. Santi and J. Ścianowski, Molecules, 2020, 25, 3354 CrossRef CAS PubMed.
- R. Lisiak and J. Młochowski, Synth. Commun., 2009, 39, 3141 CrossRef CAS.
- W. Ma, Z. Weng, X. Fang, L. Gu, Y. Song and L. Ackermann, Eur. J. Org. Chem., 2019, 1, 41 CrossRef.
- A. Laskowska, A. J. Pacuła-Miszewska, M. Obieziurska-Fabisiak, J. Drogosz-Stachowicz, A. Janecka, A. Wojtczak and J. Ścianowski, Materials, 2022, 15, 2068 CrossRef CAS PubMed.
- F. Kumakura, B. Mishra, K. I. Priyadarsini and M. Iwaoka, Eur. J. Org. Chem., 2010, 3, 440 CrossRef.
- F. Martinez-Morales, A. J. Alonso-Castro, J. R. Zapata-Morales, C. Carranza-Alvarez and O. H. Aragon-Martinez, Chem. Pap., 2020, 74, 3325 CrossRef CAS.
- B. Brummelhaus de Menezes, L. Mironuk Frescura, R. Duarte, M. A. Villetti and M. Barcellos da Rosa, Anal. Chim. Acta, 2021, 1157, 338398 CrossRef PubMed.
- T. Mosmann, J. Immunol. Methods, 1983, 65, 55 CrossRef CAS PubMed.
Footnote |
† Electronic supplementary information (ESI) available: 1H, 13C and 77Se NMR spectra of phenylselenides 6–19 are available. See DOI: https://doi.org/10.1039/d3ra02475j |
|
This journal is © The Royal Society of Chemistry 2023 |
Click here to see how this site uses Cookies. View our privacy policy here.