DOI:
10.1039/D3RA02407E
(Paper)
RSC Adv., 2023,
13, 14501-14505
Visible-light-promoted N–H functionalization of O-substituted hydroxamic acid with diazo esters†
Received
11th April 2023
, Accepted 4th May 2023
First published on 12th May 2023
Abstract
Herein we report an N–H functionalization of O-substituted hydroxamic acid with diazo esters under blue LED irradiation conditions. The present transformations could be performed efficiently under mild conditions without use of catalyst, additive and N2 atmosphere. Interestingly, when THF and 1,4-dioxane were employed as the reaction solvents, an active oxonium ylide involved three-component reaction and an N–H insertion of carbene species into hydroxamate occurred, respectively.
From recent decades, diazo compounds have been widely employed as an efficient reagent in numerous organic transformations.1 For instance, they could be applied for constructing cyclopropane skeletons by transferring a carbene unit to a double bond with specific diasteroselectivity.2 Moreover, diazo compounds could also be involved in transition metal catalysed carbene transfer reactions, which resulted in the development of various strategies for the construction of new C–C and C–X (X = N, O, S, Se, Si, Sn, Ge) bonds.3
Recently, visible light mediated transformations of donor/acceptor diazo compounds have been intensively studied.4 Until now, under visible light irradiation conditions, the conversion of donor/acceptor diazo compounds is mainly applied for cyclopropanation,5 insertion reaction of X–H (X = B, C, O, N, Si, S) bond6,7 via the formation of free carbene as an important intermediate, and some conversion that involves the formation of ylide intermediates.8,9 In 2018, Davies and Jurberg first revealed that aryldiazoacetates had absorption in the visible light region (λ = 400–500 nm).10 They found that aryldiazoacetates could occur cyclopropanation with styrene,10 O–H insertion reactions with oxime,6d N–H insertion reactions with amines and C–H insertion reactions with cyclic alkanes or arenes (Scheme 1a). Subsequently, Guo and Qiu reported a visible light promoted S–H insertion reaction of aryl diazoacetates and cysteine residues in batch and flow (Scheme 1b).11 Afterwards, photochemical multicomponent reactions involving aryldiazoacetates have been developed successively.12
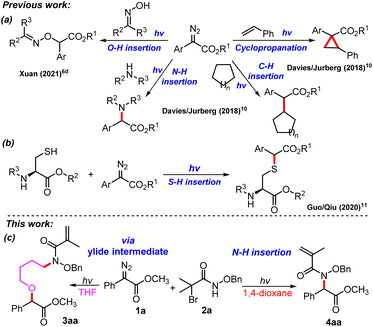 |
| Scheme 1 Visible light induced carbene insertion reaction of diazo compounds. | |
As our research interests on the development of photochemical insertion reactions of X–H-containing molecules into aryl diazoacetates, herein we report a visible-light-promoted N–H functionalization of O-substituted hydroxamates with aryldiazoacetates. It was found that when THF was the reaction solvent, a three-component coupling product was obtained, while when 1,4-dioxane was used as the solvent, an N–H insertion product was achieved (Scheme 1c). As a multicomponent-reaction between hydroxamic acid and diazo esters has been developed,13 this work will be a good supplement to the photochemical transformation of diazo compounds through the formation of free carbene as a key intermediate.
At the outset, we evaluated the reaction using methyl 2-diazo-2-phenylacetate 1a and N-(benzyloxy)-2-bromo-2-methylpropanamide 2a as the model substrates. Initially, under the irradiation of 30 W blue LEDs, the reaction was conducted in THF in the presence of NaHCO3 (Table 1, entry 1), but only trace amount of product 3aa was observed. Next, some stronger bases were employed in the reaction system. To our delight, the THF incorporated product methyl (R)-2-(4-(N-(benzyloxy)methacrylamido)butoxy)-2-phenylacetate 3aa could be isolated in good yields (Table 1, entries 2–4). Especially, when KOtBu was taken as the base, product 3aa could be isolated in 90% yield (Table 1, entry 4). Characterization of product 3aa by 1H NMR, 13C NMR and HRMS indicated that an intramolecular elimination of one molecular HBr occurred. Furthermore, control experiment under dark conditions was conducted, the result of which demonstrated the necessity of visible light irradiation condition (Table 1, entry 5). The impact of water on this transformation was studied, when 1.0 equiv. of water was added into the reaction system, except for product 3aa (28% yield), an N–H insertion product 10 that still brominated was generated in 46% yield. (For the details of the conditional optimization, please see the ESI, Page S3 and S4.†)
Table 1 Optimization of reaction conditionsa
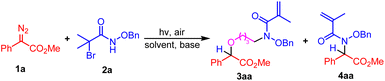
|
Entry |
Base |
Solvent |
Yieldb (%) |
3aa |
4aa |
Reaction conditions: 1a (0.2 mmol), 2a (1.5 equiv.), base (1.2 equiv.), solvent (2 mL), 14 h, room temperature, 30 W blue LEDs (λ ≈ 440–450 nm). Isolated yields. Without light irradiation. n.d. = no desired product was detected. |
1 |
NaHCO3 |
THF |
Trace |
n.d. |
2 |
NaOH |
THF |
63 |
n.d. |
3 |
DBU |
THF |
50 |
n.d. |
4 |
KOtBu |
THF |
90 |
Trace |
5c |
KOtBu |
THF |
n.d. |
n.d. |
6 |
KOtBu |
1,4-Dioxane |
n.d. |
60 |
7 |
Cs2CO3 |
1,4-Dioxane |
n.d. |
49 |
8 |
NaOH |
1,4-Dioxane |
n.d. |
43 |
9 |
NaHCO3 |
1,4-Dioxane |
n.d. |
Trace |
10 |
KOtBu |
DCE |
n.d. |
n.d. |
11 |
KOtBu |
DMF |
n.d. |
n.d. |
12 |
KOtBu |
CH3CN |
n.d. |
n.d. |
13c |
KOtBu |
1,4-Dioxane |
n.d. |
n.d. |
Interestingly enough, N–H insertion product methyl (R)-2-(N-(benzyloxy)methacrylamido)-2-phenylacetate 4aa was produced in 60% yield when 1,4-dioxane was employed as the solvent (Table 1, entry 6). Further exploration of different kinds of bases including Cs2CO3, NaOH and NaHCO3 didn't bring better results (Table 1, entries 7–9). Inferior results were observed when the reaction solvent was changed to DCE, DMF or CH3CN (Table 1, entries 10–12). Control experiment was also performed, the result showed that visible light irradiation was necessary for the generation of product 4aa (Table 1, entry 13).
With the optimal reaction conditions in hand, we started to explore the substrate scope of the solvent-controlled N–H functionalization reaction of hydroxamates 2 with aryl diazoacetates 1. The results are shown in Schemes 2 and 3. Scheme 2 presented the three-component reaction of aryl diazoacetates 1, hydroxamates 2 and THF. Methyl 2-diazo-2-phenylacetate with both electron-donating and electron-withdrawing groups like Me, CF3, F, Cl, Br at the ortho-, meta-, para-position of the benzene ring were all tolerated, resulting in the formation of products 3aa–3ia in good to excellent yields. For instance, the three-component reaction of methyl 2-(4-chlorophenyl)-2-diazoacetate, N-(benzyloxy)-2-bromo-2-methylpropanamide 2a and THF gave rise to the product 3ea in 87% yield. Additionally, the naphthyl-containing substrate 1j was also compatible with this protocol, generating the corresponding product 3ja in 79% yield. Subsequently, substituent modification of the ester group in aryl diazoacetates was investigated. The results showed that the replacement of methyl group with ethyl, n-hexyl, isopropyl, benzyl, cycloalkyl and other sensitive functional groups (1q and 1r) was feasible, leading to the corresponding products 3ka–3ra in good yields (51–91%). Unfortunately, the α-aryl diazo substrate 1s was failed to react with 2a and THF.
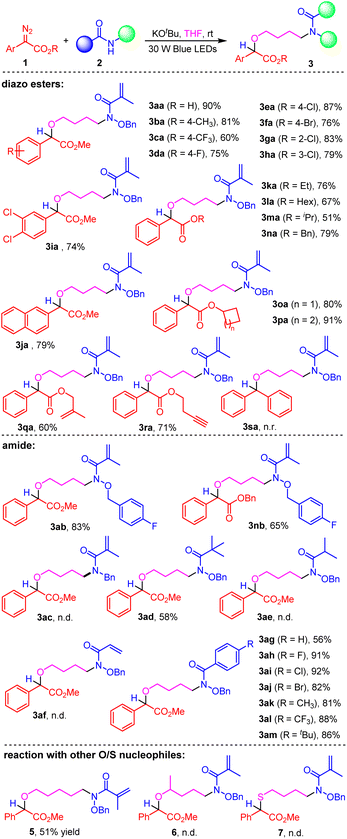 |
| Scheme 2 Substrate scope with THF as the solvent. Reaction conditions: 1a (0.2 mmol), 2a (0.3 mmol), KOtBu (0.24 mmol), THF (2 mL), r.t., 30 W blue LEDs (λ ≈ 440–450 nm), 14 h. Isolated yield. n.d. = no desired product was detected. n.r. = no reaction. | |
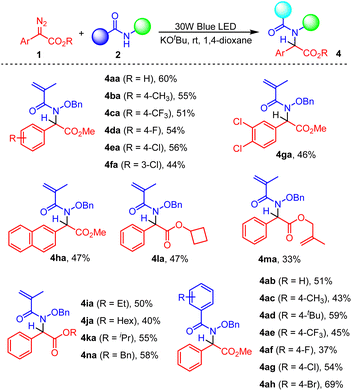 |
| Scheme 3 Substrate scope with 1,4-dioxane as the solvent. Reaction conditions: 1 (0.2 mmol), 2 (0.3 mmol), KOtBu (0.24 mmol), 1,4-dioxane (2 mL), r.t., 30W blue LEDs (λ ≈ 440–450 nm), 14 h. Isolated yield. | |
Then, we investigated the substrate scope of amide 2. The fluoro-group containing 2b was found to react with 1a or 1n smoothly, giving the corresponding products 3ab and 3nb in good yields. However, changing the OBn group to Bn group, no desired product 3ac was detected. To our delight, the tert-butyl substituted amide 2d worked well to produce product 3ad in 58% yield. Unfortunately, when N-(benzyloxy)isobutyramide 2e or N-(benzyloxy)-2-bromopropanamide 2f were taken as the substrate, both of the reaction were failed, no desired products (3ae, 3af) was observed. Additionally, aromatic amides bearing either electron-withdrawing or electron-donating groups were also tolerated, resulting in the formation of the corresponding products 3ag–3am in good yields. Moreover, using tetrahydropyran as the reaction solvent, the corresponding product 5 was isolated in 51% yield. But, when 2-methyltetrahydrofuran or tetrahydrothiophene were taken as the reaction solvent, reactions were failed.
We further expanded the reaction scope of aryl diazoacetates 1 and hydroxamates 2 in 1,4-dioxane (Scheme 3). It was found that the incorporation of both electron-donating and electron-withdrawing groups on the phenyl ring of aryl diazoacetates generally afforded the corresponding N–H insertion product in 44–60% yields (Scheme 3, 4aa–4ga). In addition, the reaction of methyl 2-diazo-2-(naphthalen-2-yl)acetate 1h and N-(benzyloxy)-2-bromo-2-methylpropanamide 2a also proceeded very well under the standard conditions, giving rise to 4ha in 47% yield. Moreover, a series of aryl diazoacetates were also demonstrated to react with 2a smoothly, leading to the formation of products 4la–4na. As expected, various amides 2 reacted with methyl 2-diazo-2-phenylacetate 1a successfully, giving rise to the corresponding products 4ab–4ah in moderate yields. Different functional groups including F, Cl, Br, CF3, Me, tBu were all tolerated under the reaction conditions.
As is shown in Scheme 4, a key intermediate 8 which was generated from an intramolecular elimination reaction of substrate 2a was detected in the reaction mixture (both in THF and 1,4-dioxane) by HRMS. Additionally, in the THF reaction system, the ylide intermediate 9 was also detected by HRMS (Scheme 4a). When we performed the reaction of 1a and 2a in the absence of base (Scheme 4c), it was found that the N–H insertion reaction still worked but without in situ dehydrobromination occurring, product 10 could be obtained in 68% yield, while in this reaction system product 3aa could not be detected. Then, we prepared N-(benzyloxy)methacrylamide 8 as the substrate, and under the irradiation with 30 W blue LEDs we conducted the reaction of 8 with 1a in THF which resulted in the formation of product 3aa in 68% yield (Scheme 4d).
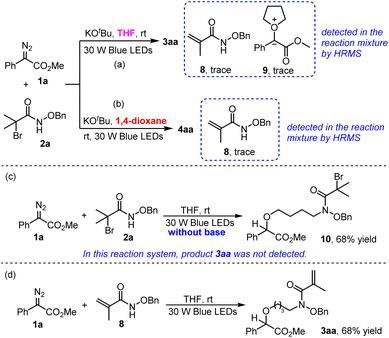 |
| Scheme 4 Mechanistic studies. | |
Based on the results of control experiments and previous reports,12 a plausible mechanism for this transformation is illustrated in Scheme 5. Initially, in the presence of base, N-(benzyloxy)-2-bromo-2-methylpropanamide 2a was transformed into intermediate 8 through elimination of one molecular HBr. In the meantime, with the release of molecule nitrogen, a carbene intermediate A was generated from aryl diazoacetates 1 upon the irradiation with blue LEDs.4–6 When the reaction was performed in 1,4-dioxane, trapping of the carbene species A by the intramolecular elimination product 8 afforded the N–H insertion product 4. However, when THF was employed as the reaction solvent, an active oxonium ylide intermediate 9 would be generated. Then, intermolecular nucleophilic attack of the nitrogen anion B on the intermediate 9 would lead to the formation of intermediate C, which further delivered the final product 3.
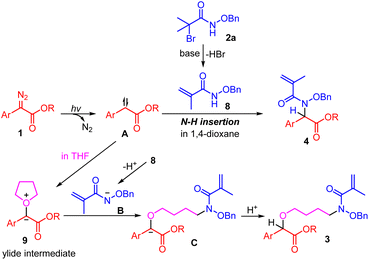 |
| Scheme 5 Possible reaction mechanism. | |
Conclusions
In summary, a visible light promoted N–H functionalization of O-substituted hydroxamic acids with diazo esters under mild conditions was developed. The reactions proceeded efficiently to afford two series of N–H functionalized products of O-substituted hydroxamic acids in moderate to excellent yields with good functional group compatibility. When 1,4-dioxane was applied as the solvent, N–H insertion of the photo-generated free carbene species into hydroxamic acid derivatives occurred directly. While, when the reaction was performed in THF, it was found that THF can participate in the reaction system through a formation of an active oxonium ylide intermediate. Taken THF as a covalent linker, this strategy can be applied in medicinal chemistry to providing a new approach for antibody-drug conjugation.14
Conflicts of interest
There are no conflicts to declare.
Acknowledgements
The authors gratefully acknowledge the financial support from the NSFC (grant no. 22201130), the Natural Science Foundation of the Jiangsu Higher Education Institutions of China (21KJB150012).
Notes and references
-
(a) Y. Xia, Y. Zhang and J. Wang, Catalytic cascade reactions involving metal carbene migratory insertion, ACS Catal., 2013, 3, 2586–2598 CrossRef CAS;
(b) N. R. Candeias, R. Paterna and P. M. P. Gois, Homologation reaction of ketones with diazo compounds, Chem. Rev., 2016, 116, 2937–2981 CrossRef CAS PubMed;
(c) D. Zhu, L. Chen, H. Fan and S. Zhu, Recent progress on donor and donor-donor carbenes, Chem. Soc. Rev., 2020, 49, 908–950 RSC.
- C. Damiano, P. Sonzini and E. Gallo, Iron catalysts with N-ligands for carbene transfer of diazo reagents, Chem. Soc. Rev., 2020, 49, 4867–4905 RSC.
-
(a) Y. He, Z. Huang, K. Wu, J. Ma, Y.-G. Zhou and Z. Yu, Recent advances in transition-metal-catalyzed carbene insertion to C–H bonds, Chem. Soc. Rev., 2022, 51, 2759–2852 RSC;
(b) Y. Xia, D. Qiu and J. Wang, Transition-metal-catalyzed cross-couplings through carbene migratory insertion, Chem. Rev., 2017, 117, 13810–13889 CrossRef CAS PubMed.
-
(a) C. Empel, C. Pei and R. M. Koenigs, Unlocking novel reaction pathways of diazoalkanes with visible light, Chem. Commun., 2022, 58, 2788–2798 RSC;
(b) B. Cai and J. Xuan, Visible light-promoted transformation of diazo compounds via the formation of free carbene as key intermediate, Chin. J. Org. Chem., 2021, 41, 4565–4574 CrossRef CAS;
(c) T. Xiao, M. Mei, Y. He and L. Zhou, Blue light-promoted cross-coupling of aryldiazoacetates and diazocarbonyl compounds, Chem. Commun., 2018, 54, 8865–8868 RSC;
(d) C. Ye, B.-G. Cai, J. Lu, X. Cheng, L. Li, Z.-W. Pan and J. Xuan, Visible-light-promoted polysubstituted olefins synthesis involving sulfur ylides as carbene trapping reagents, J. Org. Chem., 2021, 86, 1012–1022 CrossRef CAS PubMed;
(e) F. He, C. Pei and R. M. Koenigs, Photochemical fluoro-amino etherification reactions of aryldiazoacetates with NFSI under stoichiometric conditions, Chem. Commun., 2020, 56, 599–602 RSC;
(f) L. W. Ciszewski, K. Rybicka-Jasińska and D. Gryko, Recent developments in the photochemical reactions of diazo compounds, Org. Biomol. Chem., 2019, 17, 432–448 RSC;
(g) D. L. Priebbenow, Silicon-derived singlet nuclephilic carbene reagents in organic synthesis, Adv. Synth. Catal., 2020, 362, 1927–1946 CrossRef CAS.
-
(a) Y. Xu, G. Lv, K. Yan, H. He, J. Li, Y. Luo, R. Lai, L. Hai and Y. Wu, Blue light-promoted carbene transfer reactions of tosylhydrazones, Chem.–Asian J., 2020, 15, 1945–1947 CrossRef CAS PubMed;
(b) Y. Guo, T. V. Nguyen and R. M. Koenigs, Norcaradiene synthesis via visible-light-mediated cyclopropanation reactions of arenes, Org. Lett., 2019, 21, 8814–8818 CrossRef CAS PubMed;
(c) S. Zhao, X.-X. Cheng, N. Gao, M. Qian and X. Chen, Visible-light-mediated cyclopropanation reactions of 3-diazooxindoles with arenes, J. Org. Chem., 2021, 86, 7131–7140 CrossRef CAS PubMed;
(d) R. Hommelsheim, Y. Guo, Z. Yang, C. Empel and R. M. Koenigs, Blue-light-induced carbene-transfer reactions of diazoalkanes, Angew. Chem., Int. Ed., 2019, 58, 1203–1207 CrossRef CAS PubMed;
(e) V. Klöpfer, R. Eckl, J. Floß, P. M. C. Roth, O. Reiser and J. P. Barham, Catalyst-free, scalable heterocyclic flow photocyclopropanation, Green Chem., 2021, 23, 6366–6372 RSC;
(f) S. Guha, S. Gadde, N. Kumar, D. S. Black and S. Sen, Orthogonal syntheses of γ-carbolinone and spiro[pyrrolidinone-3,3′]indole derivatives in one
pot through reaction telescoping, J. Org. Chem., 2021, 86, 5234–5244 CrossRef CAS PubMed.
-
(a) Z.-L. Chen, C. Empel, K. Wang, P.-P. Wu, B.-G. Cai, L. Lei, R. M. Koenigs and J. Xuan, Enabling cyclopropanation reactions of imidazole heterocycles via chemoselective photochemical carbene transfer reactions of NHC-boranes, Org. Lett., 2022, 24, 2232–2237 CrossRef CAS PubMed;
(b) H. Zhang, Z. Wang, Z. Wang, Y. Chu, S. Wang and X.-P. Hui, Visible-light-mediated formal carbene insertion reaction: enantioselective synthesis of 1,4-dicarbonyl compounds containing all-carbon quaternary stereocenter, ACS Catal., 2022, 12, 5510–5516 CrossRef CAS;
(c) F. He and R. M. Koenigs, Visible light mediated, metal-free carbene transfer reactions of diazoalkanes with propargylic alcohols, Chem. Commun., 2019, 55, 4881–4884 RSC;
(d) Q. Li, B.-G. Cai and J. Xuan, Oxime ether synthesis through O–H functionalization of oximes with diazo esters under blue LED irradiation, Org. Lett., 2021, 23, 6951–6955 CrossRef CAS PubMed;
(e) D. Maiti, R. Das and S. Sen, Blue LED-mediated N–H insertion of indoles into aryldiazoesters at room temperature in batch and flow: reaction kinetics, density functional theory, and mechanistic study, J. Org. Chem., 2021, 86, 2522–2533 CrossRef CAS PubMed.
-
(a) J. Yang, J. Duan, G. Wang, H. Zhou, B. Ma, C. Wu and J. Xiao, Visible-light-promoted site-selective N1-alkylation of benzotriazoles with α-diazoacetates, Org. Lett., 2020, 22, 7284–7289 CrossRef CAS PubMed;
(b) F. He, F. Li and R. M. Koenigs, Metal-free insertion reactions of silanes with aryldiazoacetates, J. Org. Chem., 2020, 85, 1240–1246 CrossRef CAS PubMed;
(c) R. Chen, G. Ma, Y. Li, J. Zhang, R. Xia, K.-K. Wang and L. Liu, TBAI-catalyzed S–H and N–H insertion reactions of α-diazoesters with thiophenols and amines under metal-free conditions, J. Org. Chem., 2022, 87, 10990–10999 CrossRef CAS PubMed;
(d) J. Yang, G. Wang, S. Chen, B. Ma, H. Zhou, M. Song, C. Liu and C. Huo, Catalyst-free, visible-light-promoted S–H insertion reaction between thiols and α-diazoesters, Org. Biomol. Chem., 2020, 18, 9494–9498 RSC.
-
(a) Z. Wang, R. Liu, C. Qu, X.-E. Zhao, Y. Lv, H. Yue and W. Wei, Elemental sulfur as the “S” source: visible-light-mediated four-component reactions leading to thiocyanates, Org. Chem. Front., 2022, 9, 3565–3570 RSC;
(b) R. Hommelsheim, Y. Guo, Z. Yang, C. Empel and R. M. Koenigs, Blue-light-induced carbene-transfer reactions of diazoalkanes, Angew. Chem., Int. Ed., 2019, 58, 1203–1207 CrossRef CAS PubMed;
(c) J. Yang, J. Wang, H. Huang, G. Qin, Y. Jiang and T. Xiao, gem-Difluoroallylation of aryl diazoesters via catalyst-free, blue-light-mediated formal Doyle–Kirmse reaction, Org. Lett., 2019, 21, 2654–2657 CrossRef CAS PubMed.
-
(a) J. Lu, L. Li, X.-K. He, G.-Y. Xu and J. Xuan, Visible light-promoted sulfoxonium ylides synthesis from aryl diazoacetates and sulfoxides, Chin. J. Chem., 2021, 39, 1646–1650 CrossRef CAS;
(b) S. Roy, G. Kumar and I. Chatterjee, Photoinduced diverse reactivity of diazo compounds with nitrosoarenes, Org. Lett., 2021, 23, 6709–6713 CrossRef CAS PubMed;
(c) B. Cai, S.-S. Luo, L. Li, L. Li, J. Xuan and W.-J. Xiao, Visible light promoted amide bond formation via one-pot nitrone in situ formation/rearrangement cascade, CCS Chem., 2020, 2, 2764–2771 Search PubMed.
- I. D. Jurberg and H. M. L. Davies, Blue light-promoted photolysis of aryldiazoacetates, Chem. Sci., 2018, 9, 5112–5118 RSC.
- L.-Z. Qin, X. Yuan, Y.-S. Cui, Q. Sun, X. Duan, K.-Q. Zhuang, L. Chen, J.-K. Qiu and K. Guo, Visible-light-mediated S–H bond insertion reactions of diazoalkanes with cysteine residues in batch and flow, Adv. Synth. Catal., 2020, 362, 5093–5104 CrossRef CAS.
-
(a) R. Cheng, C. Qi, L. Wang, W. Xiong, H. Liu and H. Jiang, Visible light-promoted synthesis of organic carbamates from carbon dioxide under catalyst- and additive-free conditions, Green Chem., 2020, 22, 4890–4895 RSC;
(b) B.-G. Cai, Q. Li, Q. Zhang and J. Xuan, Synthesis of trisubstituted hydroxylamines by a visible light-promoted multicomponent reaction, Org. Chem. Front., 2021, 8, 5982–5987 RSC;
(c) Y. Lv, R. Liu, H. Ding, W. Wei, X. Zhao and L. He, Metal-free visible-light-induced multi-component reactions of α-diazoesters leading to S-alkyl dithiocarbamates, Org. Chem. Front., 2022, 9, 3486–3492 RSC;
(d) C. Qu, R. Liu, Z. Wang, Y. Lv, H. Yue and W. Wei, Visible-light-driven multicomponent reactions to access S-alkyl phosphorothioates using elemental sulfur as the sulfur source, Green Chem., 2022, 24, 4915–4920 RSC;
(e) S. E. John, S. Gulati and N. Shankaraiah, Recent advances in multi-component reactions and their mechanistic insights: a triennium review, Org. Chem. Front., 2021, 8, 4237–4287 RSC.
- B.-G. Cai, Q. Li, C. Empel, L. Li, R. M. Koenigs and J. Xuan, Dark and light reactions of carbenes-merging carbene transfer reactions with N-heterocyclic carbene catalysis for the synthesis of hydroxamic acid esters, ACS Catal., 2022, 12, 11129–11136 CrossRef CAS.
-
(a) V. Kostova, P. Désos, J. Starck and A. Kotschy, The chemistry behind ADCs, Pharmaceuticals, 2021, 14, 442–487 CrossRef CAS PubMed;
(b) S. J. Walsh, J. D. Bargh, F. M. Dannheim, A. R. Hanby, H. Seki, A. J. Counsell, X. Ou, E. Fowler, N. Ashman, Y. Takada, A. Isidro-Llobet, J. S. Parker, J. S. Carroll and D. R. Spring, Site-selective modification strategies in antibody-drug conjugates, Chem. Soc. Rev., 2021, 50, 1305–1353 RSC.
|
This journal is © The Royal Society of Chemistry 2023 |
Click here to see how this site uses Cookies. View our privacy policy here.