DOI:
10.1039/D3RA01614E
(Paper)
RSC Adv., 2023,
13, 13616-13623
Catenane formation of a cyclic poly(alkyl sorbate) via chain-growth polymerization induced by an N-heterocyclic carbene and ring-closing without extreme dilution†
Received
12th March 2023
, Accepted 18th April 2023
First published on 3rd May 2023
Abstract
1,3-Di-tert-butylimidazol-2-ylidene (NHCtBu), a typical N-heterocyclic carbene (NHC), was previously found to induce the anionic chain-growth polymerization of ethyl sorbate (ES) in the presence of an aluminum Lewis acid, i.e., methylaluminum bis(2,6-di-tert-butyl-4-methylphenoxide) (MAD), in which the neighboring of α-terminal dienolate with a propagating anion induced cyclization without highly diluted conditions, after monomer depletion, to give the cyclic poly(ES). In this paper, we report that catenane formation occurs by two-step polymerization of ethyl sorbate (ES), in which, after complete monomer (ES) consumption ([ES]0/[NHCtBu]0 = 100/1) in toluene followed by purification by reprecipitation, a second addition of ES monomer ([ES]0/[ NHCtBu]0 = 20/1) in another pot (in toluene or tetrahydrofuran (THF)) resulted in catenane formation, namely a polycatenane. TEM images of a sample from the second step polymerization in THF revealed particles of polycatenane structure consisting of cyclic poly(ES) with sizes ranging from 200 to 1000 nm, showing that this NHCtBu triggered chain polymerization and successive cyclization without highly diluted conditions enabled us to fabricate the intended polycatenane in the successive two-step polymerization.
Introduction
Fully developed precision control of polymer molecules1,2 will enable us to use various polymeric materials in the most effective ways. Aiming at the design of more exotic structures, topological control of polymer molecules3–5 to produce structures such as cyclic polymers, rotaxanes, and catenanes, is essential. Cyclic polymers are, therefore, key materials for such kinds of advanced material design. One of the most reliable synthetic procedures for cyclic polymers is ring-closure, but it needs highly diluted conditions in order to avoid intermolecular coupling.6,7 In general, template synthesis using non-covalent interactions is used to design catenane structures.8,9 However, template synthesis requires complex molecular design, which will inevitably make the synthetic process more complicated. In 2017, we found that the typical N-heterocyclic carbene, 1,3-di-tert-butylimidazol-2-ylidene (NHCtBu), initiated the anionic chain-growth polymerization of methyl sorbate (MS) with the aluminum Lewis acid, methylaluminum bis(2,6-di-tert-butyl-4-methylphenoxide) (MAD).10 After monomer depletion, the carbene also acted as a leaving group to give the cyclic poly(MS). The ring closure did not require highly diluted conditions (initial monomer concentration ([M]0) of ca. 1.0 M) thanks to the neighboring α-terminal NHCtBu during the polymerization (Scheme 1). Recently, Chen et al. reported the revised initiation mechanism in the same polymerization, in which NHCtBu abstracted the ε-methyl proton as the base and the produced dienolate acted as propagating center to give cyclic poly(MS) via Michael addition of the anion towards α-terminal MS unit as shown in Scheme 1 (bottom).11 They called the corrected synthetic route as basic pathway11 in contrast to our reported nucleophilic initiation pathway by NHCtBu,10 and the latter is generally accepted now.
 |
| Scheme 1 Anionic polymerization of alkyl sorbate via basic pathway that reported by Chen et al. in 2022 to give corresponding cyclic poly(alkyl sorbate). | |
In the work reported here we report preliminary results of catenane formation by two-step polymerization of ethyl sorbate (ES). In this case, after complete monomer (ES) consumption ([ES]0/[NHCtBu]0 = 100/1), a second addition of ES monomer ([ES]0/[NHCtBu]0 = 20/1 or 100/1) resulted in the formation of polycatenane (Scheme 2). Following thiol–ene click grafting with 1-dodecanethiol,12 transmission electron microscopy (TEM) proved the formation of the catenane. The TEM images of the samples from the two-step polymerization in tetrahydrofuran (THF) revealed polycatenane particles consisting of cyclic poly(ES) with sizes ranging from 200–1000 nm. This result showed that we had fabricated the desired polycatenane in a two-step synthesis via this NHCtBu triggered chain polymerization, without requiring highly diluted conditions (Fig. 1).
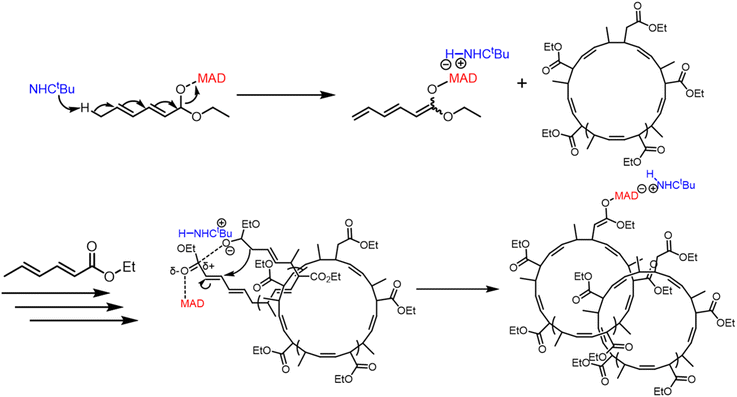 |
| Scheme 2 Synthesis scheme of poly(ES)-based polycatenane via NHCtBu-initiated two step polymerization of ES. | |
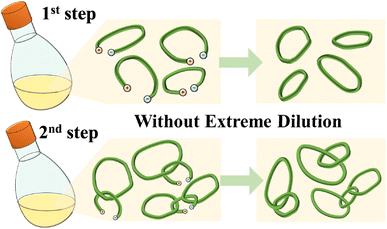 |
| Fig. 1 Schematic image of catenane or polycatenane formation via this NHCtBu initiated chain polymerization without requiring highly diluted conditions. | |
Experimental section
Materials
1,3-Di-tert-butylimidazol-2-ylidene (NHCtBu) (>98%), methyl sorbate (MS) (>98%), ethyl sorbate (ES) (>98%), methylaluminum bis(2,6-di-tert-butyl-4-methylphenoxide) (MAD) (0.4 M toluene solution), 1-dodecanethiol (>95%), 3-mercaptopropionic acid (>98%), and 2,2-dimethoxy-2-phenylacetophenone (DMPA, > 98%) were purchased from Tokyo Kasei Co. (Tokyo, Japan). Hydrogen chloride in methanol was purchased from Sigma-Aldrich Japan (Tokyo, Japan). THF (deoxidized), toluene (deoxidized) and toluene (super dehydrated) were purchased from Wako Co. (Osaka, Japan) and used for the reactions and TEM observations, respectively. Chloroform, hexane and 0.5 N NaOH aq. were purchased from Nacalai Tesque Co. (Kyoto, Japan).
First step polymerization of ES using NHCtBu as the initiator species
All polymerization reactions were carried out under N2 conditions. First, a solution of ES (5.49 mL, 37.5 mmol) and MAD (1.875 mL, 0.75 mmol) in toluene (12.5 mL) was prepared in an eggplant flask. A solution of NHCtBu (68 mg, 0.375 mmol) in toluene (12.5 mL) was prepared in another eggplant flask. After cooling the two solutions at −20 °C for 1 hour, the initiator solution was added to the monomer solution and the reaction was carried out at −20 °C for 24 hours. The addition of hydrogen chloride–methanol solution (0.5 mL) was regarded as a termination reaction. The resulting polymer was purified by reprecipitation with chloroform and hexane, as good and poor solvents, respectively. Data for poly(ES): 1H NMR (400 MHz, CDCl3, δ, ppm): 0.95 (brd, 3H, –CH–CH3), 1.17–1.27 (brm, 3H, –CH2–CH3), 2.38–2.65 (brm, 1H,
CH–CH–), 2.65–2.86 (brm, 1H,
CH–CH–), 4.00–4.15 (brm, 2H, –O–CH2–), 5.22–5.43 (brm, 2H, –CH
CH–).
Second step polymerization of ES using NHCtBu as the initiation species
The experiment was carried out in the same way as above. First, a solution of ES (0.549 mL, 7.5 mmol), MAD (0.9374 mL, 0.75 mmol) and poly(ES) synthesized in the above section (737.9 mg) in toluene (1.75 mL) was added to an eggplant flask. A solution of NHCtBu (33.81 mg, 0.375 mmol) in toluene (1.25 mL) was prepared in another eggplant flask. After cooling the two solutions at −20 °C for 1 hour, the initiator solution was added to the monomer solution and the reaction was carried out at −20 °C for 24 hours. The addition of hydrogen chloride–methanol solution (0.5 mL) was regarded as a termination reaction. The resulting polymer was purified by reprecipitation with chloroform and hexane, as good and poor solvent, respectively. The polymerization were also performed in THF according to similar procedure, in which ratio of [ES]0/[I]0/[MAD]0 was same. Data for poly(ES): 1H NMR (400 MHz, CDCl3, δ, ppm): 0.95 (brd, 3H, –CH–CH3), 1.17–1.27 (brm, 3H, –CH2–CH3), 2.38–2.65 (brm, 1H,
CH–CH–), 2.65–2.86 (brm, 1H,
CH–CH–), 4.00–4.15 (brm, 2H, –O–CH2–), 5.22–5.43 (brm, 2H, –CH
CH–).
Thiol–ene click reaction with 1-dodecanethiol
Poly(ES)-based polycatenane synthesized via the 2 step polymerization in the above section (Mn = 24.4 kg mol−1/5.32 kg mol−1, Mw/Mn = 1.16/1.22, 121.1 mg, 0.86 mmol of repeating unit), 1-dodecanethiol (1.04 mL, 4.3 mmol), DMPA (221.4 mg, 0.86 mmol), and toluene (5 mL) were added to the eggplant flask under an N2 atmosphere. The mixture was UV irradiated for 2 hours with stirring at room temperature. The resulting polymer was purified by reprecipitation with chloroform and a mixed solvent of methanol and ethanol (9/1, v/v), as good and poor solvents, respectively. Data for cyclic poly(ES)-graft-1-dodecanethiol (Mn = 9.88 kg mol−1 (Fig. S1†), Mw/Mn = 1.51, grafting ratio: 55.1%): 1H NMR (400 MHz, CDCl3, δ, ppm): 0.88 (t, 6H, –CH3 6.8 Hz), 1.20–1.41 (brm, 20H, –S–CH2–(CH2)10–), 1.67 (q, 2H, –S–CH2–CH2– 7.3 Hz), 2.68 (d, 3H, –CH–S–CH2– 7.4 Hz), 4.05–4.20 (brs, 2H, –O–CH2–), 5.30–5.50 (brs, 2H, –CH
CH–).
Measurements
1H NMR (400 MHz) spectra were measured using a Bruker Analytik DPX400 equipment (27 °C). The number average molecular weight (Mn) and molecular weight distribution (Mw/Mn) values of the synthesized polymers were measured by a size exclusion chromatography (SEC) system including a JASCO PU4185 pump system, equipped with JASCO RI-4035 differential refractometer and JASCO CO-2065 Plus Intelligent Column Oven [eluent, tetrahydrofuran (THF), flow rate, 0.35 mL min−1, temperature, 40 °C; Tosoh Inc.], which was calibrated using poly(styrene) standards. Matrix-assisted laser desorption/ionization time-of-flight (MALDI-TOF) spectra were gained using a Spiral TOF JMS-S3000 (JEOL) spectrometer with α-cyano-4-hydroxycinnamic acid as the matrix reagent. In order to give sodium-cationized ions of the poly(MS) ([M + Na]+), NaI was used. For direct observation of the produced cyclic polymers, a transmission electron microscope (TEM, JEM-Z2500, JEOL. Inc.) optioned with an Ultra Scan CCD camera (Orius Camera, Gatan Inc.) was used. The samples for TEM were prepared in accordance with our reported protocol12 as follows; the synthesized materials were first dissolved in toluene (0.1 μg mL−1). A drop of toluene solution added dropwise into a polystyrene Petri dish filled with ultrapure water to form a molecular layer on the water surface, into which a carbon-coated TEM (STEM) grid (ELS-C10, Okenshoji Co.) was dipped. TEM observations were carried out with the unstained samples at 200 kV accelerating voltage. The particle size was measured by dynamic light scattering using a Zetasizer Nano ZS (Malvern Instruments, UK). The measurement temperature was 25 °C, THF was used as the solvent, and a glass two-sided transparent cell purchased from SANSYO (Tokyo, Japan) was used as the cell. The measurement was carried out by diluting the stock solution (4 mg mL−1) in toluene.
Results and discussion
Two-step anionic polymerization of ethyl sorbate (ES) using NHCtBu as initiaton trigger
Using our reported protocol,10 NHCtBu initiated the anionic polymerization of ES in toluene at −20 °C in the presence of MAD as a bulky aluminum Lewis acid to provide cyclic poly(ES)s with an Mn of 15.9 × 103 and polydispersity index (Mw/Mn = 1.37). Recently, Lewis pair polymerization (LPP) has received much attention in polymer chemistry, which showed that LPP of polar vinyl monomers such as methyl methacrylate (MMA) were polymerized rapidly.13–21 Results of the polymerizations are summarized in Table 1, in which the first feed ratio of monomer to initiator ([M]0/[NHCtBu]0 = 100/1) was higher than second addition ([M]0/[NHCtBu]0 = 20/1) in the presence of MAD ([MAD]0/[NHCtBu]0 = 2/1). Toluene (run 1) and THF (run 2) were chosen as the solvents for the second step polymerization when the second monomer was added (Table 1). From the dielectric constant of the two solvents, we expected that THF is better solvent for poly(ES), which might influenced on the catenane formation because flexibility and interminglement of the polymer chains are key factors for the catenane formation. In both cases, the SEC curves of parent poly(ES) shifted to the high molecular weight region accompanied with another SEC curve (Fig. 2). The results indicated that catenane formation as well as a simple cyclization occurred in the second ES addition. The initiation efficiency may have been influenced by differences in the concentration of the prepared polymers and solvents. THF showed higher polarity than toluene. This interfered with the catalytic effect of MAD, resulting in inferior monomer activation compared to toluene. As a result, we found that the initiation efficiency of toluene (run 1) was higher.
Table 1 Two-step anionic polymerization of ethyl sorbate (ES) using NHCtBu as initiaton trigger
Run |
Solvent (2nd step) |
Poly(ES) |
Second time [ES]0/[I]0/[MAD]0 |
Conva (%) |
Mnb (kg mol−1) |
Mw/Mnb |
Ieffc (%) (2nd step) |
Mnb (kg mol−1) |
Mw/Mnb |
[ES (repeating unit)] (mol L−1) |
Monomer conversion measured by 1H NMR in CDCl3. Relative value determined by SEC with a standard series of PS. Initiation efficiency (%) = Mn(calculated)/Mn(observed) × 100, where Mn(calculated) = [molecular weight (ES)] × ([ES]0/[I]0) × (conversion). |
1 |
Toluene |
15.9 |
1.37 |
1.13 |
20/1/2 |
100 |
24.0/4.15 |
1.19/1.27 |
67.6 |
2 |
THF |
15.9 |
1.37 |
1.17 |
20/1/2 |
100 |
24.3/5.32 |
1.16/1.22 |
52.7 |
3 |
Toluene |
14.0 |
1.16 |
0.92 |
100/1/2 |
100 |
18.5 |
1.34 |
75.8 |
4 |
THF |
16.2 |
1.25 |
1.24 |
100/1/2 |
100 |
21.2 |
1.27 |
66.1 |
5 |
Toluene |
42.1 |
1.36 |
0.33 |
100/1/3 |
100 |
76.9/21.7 |
1.10/1.17 |
64.5 |
6 |
THF |
21.8 |
1.22 |
1.03 |
100/1/2 |
100 |
21.1 |
1.37 |
75.8 |
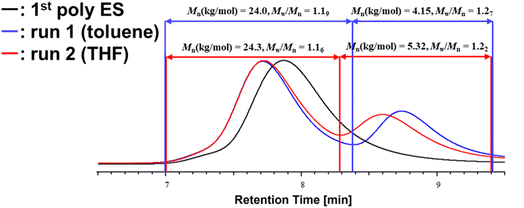 |
| Fig. 2 SEC traces of NHCtBu-catalyzed two step polymerization of ES in toluene and THF. | |
When we increased the feed molar ratio ([ES]0/[I]0) of 20/1 to 100/1 in the second monomer addition in toluene (runs 3 and 5) and THF (run 4), the SEC curves shifted to high molecular region with broadening of the Mw/Mn (runs 3 and 4, Fig. S2 and S3†). The results indicated that cyclic as well as catenane structure was formed. Using higher molecular weight poly(ES) and diluted concentration of [ES] of 0.33 mol L−1 (run 5), it seems that the expected catenane formation was not attained from the SEC curves (Fig. S4†).
As a result of MALDI-TOF mass analysis, the peak pattern with an interval of 140.18 (M. W. of ES unit) was observed (Fig. 3). The result suggested the presence of cyclic structures.
 |
| Fig. 3 MALDI-TOF mass signal pattern of the poly(ES)-based catenanes via NHCtBu-initiated two step polymerization of ES. The data is for the sample “run 1” in Table 1. | |
The TEM photographs (Fig. 4, 5, and S5–S7†) also indicated cyclic-catenane topology same as the SEC measurements. In order to perform TEM observations, a thiol–ene click reaction of the internal double bond of poly(ES) was employed (Scheme 3).12 Grafting using 5 eq. of 1-dodecanethiol, resulted in ca. 55.1% of internal double bonds being consumed. The grafted sample was soluble in toluene and after being poured into distilled water the surface membrane was transferred to a TEM-grid (elastic carbon). Using our reported procedure,12 the TEM image of the sample from two-step polymerization in toluene was observed (Fig. 4). An image of a large circle of catenane that was ca. 30 nm in diameter threaded through a small circle was observed.
 |
| Fig. 4 TEM image of poly(ES) based catenane via two step polymerization in toluene. | |
 |
| Scheme 3 Introduction of 1-dodecanethiol into poly(ES)-based polycatenane as side chains. | |
On the other hand, in the TEM image of a similar sample from the second-step polymerization of ES in THF, images of large, 200–1000 nm in size, polycatenane-like linked circles were observed (Fig. 5), indicating that NHCtBu-initiated anionic polymerization can also produce the expected polycatenane formation, without the need for highly dilute conditions. Considering the results of TEM observations, the formation of polycatenane is more likely to occur in THF. Furthermore, contamination by linear counterparts was not observed to the best of our ability in a TEM survey (see also Fig. S5–S7†). Therefore, the peaks of MALDI-TOF mass spectra supported the cyclic structures (Fig. 3). The size distribution was confirmed by dynamic light scattering measurement in THF, as shown in Fig. 6, in which the bimodal size distribution was observed. It seems that the large (150–1500 nm) and small peaks (5.0–30.0 nm) are ascribed to polycatenane and cyclic-catenane poly(ES), respectively, the weight fraction was 30/70, wt/wt.
 |
| Fig. 5 TEM image of poly(ES) based catenane via two step polymerization in THF. | |
 |
| Fig. 6 Comparison of before and after DLS measurement of poly(ES) based catenane via two step polymerization in THF. | |
Next, we attempted to distinguish the observed catenane structure from the aggregated cyclic structures. We expected that the ionic repulsion by introducing ionic side chains would dissociate the self-assembled structures. Using a similar procedure to that used for the click reaction, 3-mercaptopropionic acid was grafted onto the cyclic poly(ES) (Scheme 4). The incorporation ratio was 52.4%, the degree being consistent with the result using 1-dodecanethiol (vide supra). In order to ionize the carboxylic acid side chain, the grafted sample was soluble in methanol mixed with 49.05 μl of 0.5 N NaOH aq. ([NaOH]/[–COOH] = 2/1) and after being poured into chloroform the surface membrane was transferred to a TEM-grid (elastic carbon).
 |
| Scheme 4 Introduction of ionic 3-mercaptopropionic acid into poly(ES)-based polycatenane as side chains. | |
In the TEM image, isolated catenane consisting of cyclic poly(ES) was observed as shown in Fig. 7, supporting that the catenane structure was formed during the ring-closure without highly diluted conditions after NHCtBu-initiated anionic polymerization. We compared the visualized diameters in the TEM images with theoretical values calculated according to previous literature.12 We obtained a theoretical value of 15.3 nm. To calculate 15.3 nm, we first calculated the diameter of a 6-mer cyclic poly(MS) (L6mer = 0.836 nm) based on the molecular mechanics method (MM2). The MM2 method does not indicate the value of the diameter of a fully elongated chain, but rather the diameter under energetically optimized conditions. The L6mer value was then multiplied by n/6 (where n represents the degree of polymerization (n = 15.9 kDa/140.18 ≓ 110)) to obtain Ld = 15.3 nm. We considered the side chains to be attached to both sides of the main chain as zig–zag conformation. We obtained values of approximately 20–30 nm from a randomly selected objects. Also, the prepared polymers have a molecular weight distribution of 1.37. Therefore, we concluded that the observed value did not show any discrepancy with the theoretical value.
 |
| Fig. 7 TEM image of poly(ES) based catenane via two step polymerization in THF. | |
Finally, we tried to confirm the non-covalent cross-linking using a nuclear Overhauser effect (NOE) strategy. For this sample, we used similar two-step polymerization using ES and MS as the first and second monomer, respectively. In the NOESY spectrum at low temperature (−20 °C), correlation of ethyl and methyl signals ascribed to ES and MS [(cross-points (inside circle) of peaks a and c, peaks b and c in Fig. 8)], respectively, were not confirmed unfortunately, because the cross-linking points based on the catenane structure were fewer than those of the normal ring structures. In this measurement, we attempted to observe the correlation between the ester groups in the side chains of ES and MS. In a polymer with a degree of polymerization of 100, there are 100 ester groups. If this polymer constitutes a [2]-catenane, the correlation appears only at one or two ester groups out of the 100 ester groups. The degree of polymerization calculated from Mn of the cyclic poly(ES) used in this experiment is about 110. Therefore, we consider that the expected correlation was not observed as strong correlation peaks.
 |
| Fig. 8 NOESY spectrum of poly(alkyl sorbate) based catenane via two step polymerization of ES and MS in THF. | |
Conclusions
An expected cyclic poly(alkyl sorbate) was synthesized upon anionic polymerization of a conjugated diene (ES) initiated by NHCtBu as initiator, followed by ring-closing without the need of highly diluted conditions, thus compensating for the entropic penalty to encounter low reactivity telechelic polymer termini. In order to apply this unique polymerization system for catenane formation, we demonstrated two-step polymerization of ES at a normal concentration of ca. 1.0 M of [M]0. The TEM images after click grafting by 1-dodecanethiol revealed an unexpected large polycatenane structure because of the fully extended conformation of cyclic poly(ES) in THF. The results of this study promise further development of topological access to new functional polymeric materials.
Conflicts of interest
There are no conflicts to declare.
Acknowledgements
Akinori Takasu sincerely acknowledges the encouragement of a grant from the Ministry of Education, Science and Culture of Japan (Grant-in-Aid for Development Scientific Researches of No. of 18K19112 as well as 20H02786).
Notes and references
- M. Ouchi, T. Terashima and M. Sawamoto, Transition Metal-Catalyzed Living Radical Polymerization: Toward Perfection in Catalysis and Precision Polymer Synthesis, Chem. Rev., 2009, 109(11), 4963–5050 CrossRef CAS PubMed.
- M. Kamigaito, T. Ando and M. Sawamoto, Metal-catalyzed living radical polymerization, Chem. Rev., 2001, 101(12), 3689–3745 CrossRef CAS PubMed.
- Y. Tezuka, Cyclic and topological polymers: Ongoing innovations and upcoming breakthroughs, React. Funct. Polym., 2020, 148 Search PubMed.
- Y. Tezuka, Topological Polymer Chemistry Designing Complex Macromolecular Graph Constructions, Acc. Chem. Res., 2017, 50(11), 2661–2672 CrossRef CAS PubMed.
- Y. Muramatsu and A. Takasu, Synthetic innovations for cyclic polymers, Polym. J., 2022, 54(2), 121–132 CrossRef CAS.
- A. Deffieux and R. Borsali, in Macromolecular Engineering: Precise Synthesis, Materials Properties, Applications, ed. K. Matyjaszewski, Y. Gnanou and L. Leibler, Wiley-VCH, Weinheim, 2007, vol. 2, pp. 875–908 Search PubMed.
- B. A. Laurent and S. M. Grayson, An efficient route to well-defined macrocyclic polymers via "Click" cyclization, J. Am. Chem. Soc., 2006, 128(13), 4238–4239 CrossRef CAS PubMed.
- Q. Wu, P. M. Rauscher, X. Lang, R. J. Wojtecki, J. J. De Pablo, M. J. A. Hore and S. J. Rowan, Poly[n]catenanes: Synthesis of molecular interlocked chains, Science, 2017, 358, 1434–1439 CrossRef CAS PubMed.
- G. C. Lydia and B. D. Paul, Halogen- and Hydrogen-Bonding Catenanes for Halide-Anion Recognition, Chem.–Eur. J., 2014, 20(27), 8379–8385 CrossRef PubMed.
- Y. Hosoi, A. Takasu, S. Matsuoka and M. Hayashi, N-Heterocyclic Carbene Initiated Anionic Polymerization of (E,E)-Methyl Sorbate and Subsequent Ring-Closing to Cyclic Poly(alkyl sorbate), J. Am. Chem. Soc., 2017, 139(42), 15005–15012 CrossRef CAS PubMed.
- M. L. McGraw, L. T. Reilly, R. W. Clarke, L. Cavallo, L. Falivene and E. Y.-X. Chen, Mechanism of Spatial and Temporal Control in Precision Cyclic Vinyl Polymer Synthesis by Lewis Pair Polymerization, Angew. Chem., Int. Ed., 2022, 61, e202116303 CrossRef CAS PubMed.
- Y. Muramatsu, Y. Oga, A. Takasu and M. Higuchi, Direct observation of cyclic poly(N-substituted maleimide)s with broad size distributions synthesized by anionic polymerization using an N-heterocyclic carbene and successive ring closure without high dilutions, Polym. J., 2020, 52(11), 1253–1261 CrossRef CAS.
- M. L. McGraw, R. W. Clarke and E. Y.-X. Chen, Synchronous Control of Chain Length/Sequence/Topology for Precision Synthesis of Cyclic Block Copolymers from Monomer Mixtures, J. Am. Chem. Soc., 2021, 143(9), 3318–3322 CrossRef CAS PubMed.
- Y. Zhang, G. M. Miyake and E. Y. X. Chen, Alane-Based Classical and Frustrated Lewis Pairs in Polymer Synthesis: Rapid Polymerization of MMA and Naturally Renewable Methylene Butyrolactones into High-Molecular-Weight Polymers, Angew. Chem., Int. Ed., 2020, 49(27), 10158–10162 Search PubMed.
- Y. Bai, H. Wang, J. He and Y. Zhang, Rapid and Scalable Access to Sequence-Controlled DHDM Multiblock Copolymers by FLP Polymerization, Angew. Chem., Int. Ed., 2020, 59(28), 11613–11619 CrossRef CAS PubMed.
- M. Hong, J. W. Chen and E. Y. X. Chen, Polymerization of Polar Monomers Mediated by Main-Group Lewis Acid-Base Pairs, Chem. Rev., 2018, 118(20), 10551–10616, DOI:10.1021/acs.chemrev.8b00352.
- M. McGraw and E. Y. X. Chen, Catalytic Lewis Pair Polymerization of Renewable Methyl Crotonate to High-Molecular-Weight Polymers, ACS Catal., 2018, 8(10), 9877–9887, DOI:10.1021/acscatal.8b03017.
- M. L. McGraw and E. Y. X. Chen, Lewis Pair Polymerization: Perspective on a Ten-Year Journey, Macromolecules, 2020, 53(15), 6102–6122, DOI:10.1021/acs.macromol.0c01156.
- Y. Zhang, G. M. Miyake, M. G. John, L. Falivene, L. Caporaso, L. Cavallo and E. Y. X. Chen, Lewis pair polymerization by classical and frustrated lewis pairs: acid, base and monomer scope and polymerization mechanism, Dalton Trans., 2012, 41, 9119–9134 RSC.
- X. J. Wang and M. Hong, Lewis-Pair-Mediated Selective Dimerization and Polymerization of Lignocellulose-Based β-Angelica Lactone into Biofuel and Acrylic Bioplastic, Angew. Chem., Int. Ed., 2020, 59(7), 2664–2668 CrossRef CAS PubMed.
- J. Chen and E. Y. X. Chen, Lewis Pair Polymerization of Acrylic Monomers by N-Heterocyclic Carbenes and B(C6F5)3, Isr. J. Chem., 2015, 55, 216–225 CrossRef CAS.
|
This journal is © The Royal Society of Chemistry 2023 |
Click here to see how this site uses Cookies. View our privacy policy here.