DOI:
10.1039/D3RA00588G
(Paper)
RSC Adv., 2023,
13, 12072-12079
Thevetia peruviana leaves, HPLC profile, isolation, and in vitro cytotoxicity†
Received
28th January 2023
, Accepted 5th April 2023
First published on 18th April 2023
Abstract
Phytochemicals have played a significant role in the discovery of many clinically anticancer medicines. Thevetia peruviana being famous as an ornamental plant, widely cultivated, and including a wide variety of secondary metabolites this motivated us to explore more about its potential medical applications. In this study, the total methanol extract of T. peruviana leaves (family Apocyanaceae) was sequentially fractionated into hexane, methylene chloride, ethyl acetate, and butanol fractions. The total phenolic content of the methanol extract of T. peruviana was determined spectrophotometrically and found to be 72.37 mg g−1, while the total flavonoid content was found to be 12.47 mg g−1. Fourteen polyphenolic compounds were detected by the HPLC analysis of the methanol extract, in addition to five phenolic compounds, which were isolated and identified for the first time from the EtOAc extract. They were identified as gallic acid (C1), chlorogenic acid (C2), p-coumaric acid (C3), quercetin (C4), and rutin (C5). The structures were elucidated by NMR and EI/MS spectroscopic techniques. The cytotoxic activity of the methanol extract was evaluated in vitro by cell viability assay. The cytotoxicity results showed significant anticancer activity against human colon carcinoma HCT-116 with IC50 39.3 μg mL−1 and lesser activity against human lung carcinoma A-549 and human breast carcinoma MCF-7 cell lines with IC50 93.4 and 110.3 μg mL−1, respectively.
1. Introduction
In recent years, there has been a significant increase in interest in natural pharmaceuticals, herbal teas, and other health-promoting substances. Medicinal plants have a promising future owing to their medical activities, which in turn helps in curing a variety of human diseases.1 The natural products derived from medicinal plants have been proven to be an abundant source of biologically active compounds, many of which have been used as the basis for the development of new pharmaceuticals.2
Thevetia peruviana is a shrub belonging to the Apocynaceae family, also known as yellow oleander.3 It is native to Mexico and Central America.4 In the West Indies, the seeds are called ‘lucky nuts’ and are sometimes carried in the pocket to bring good luck because of their curious form and shape, which resembles a fortune cookie.5 T. peruviana is a small ornamental tree that grows to about 1.5–2.3 m high and is grown in gardens for its beautiful yellow flowers. Its leaves are spirally arranged, linear, and about 13–15 cm in length.6 All parts of the plant contain a milky juice.7
The ethnomedical uses of T. peruviana are evident in treating external wounds, ringworms, and tumors. The ground leaves of T. peruviana have excellent therapeutic values as cardiovascular effects, anticancer, antimicrobial, antioxidant, immune-modulatory, and anti-inflammatory.8
Polyphenols are micronutrients that naturally occur in plants. Recent studies show that polyphenols are powerful antioxidants. They prevent or reverse the damage caused by aging, environment, or lifestyle. They are regarded as “lifespan essentials” for their potential to reduce the risk of chronic diseases. Studies show that people who have consumed more than 650 milligrams of polyphenol-rich diets per day have lower death risks than those who get less than 500 milligrams per day.9 From all the previous studies, we were interested to investigate the total polyphenolic compounds present in the leaves of T. peruviana cultivated in Egypt.
Cancer is one of the major causes of mortality in humans throughout the world. In 2018, there were an estimated 18.1 million new cancer cases and 9.6 million cancer deaths worldwide.10 In Egypt, cancer represents the second most common cause of mortality (14%) after cardiovascular diseases (46%).
Cancer prevention and therapy are and will remain huge challenges, especially in underdeveloped countries. Breast cancer is the leading malignancy among females, according to the National Cancer Registry Program of Egypt. The conventional treatment of cancer is mainly based on a combination of surgery, chemotherapy, and radiotherapy, with advanced alternative treatments like targeted therapy and immunotherapy. The current therapy for cancer has limitations in terms of efficacy and associated side effects, the eventual development of multidrug resistance, and high treatment costs. Therefore, there is a great need to improve cancer therapy, and there has been a recent rise in the use of natural products to replace the current treatment.11 Many researchers have investigated the medicinal potentials of various herbs in treating cancer or even reducing its side effects.12 For the above-mentioned purposes, we chose three cell lines, viz., A-549, HCT-116, and MCF-7, to be investigated by the methanol extract of T. peruviana leaves.
Cytotoxicity is one of the most important measures of the in vitro biological assessment framework for the observation of cell growth, reproduction, and morphological effects. Using chemicals and the advancement of cell biology, experimental methods for the assessment of cytotoxicity are also being continuously established and improved.13,14
The aim of this study is to explore the in vitro cytotoxic activities of T. peruviana leaves and the spectrophotometric determination of total phenolics and flavonoids. It also involves the investigation of the qualitative and quantitative polyphenolic compounds using RP-HPLC, as well as the isolation and identification of the major phytochemical compounds from the leaves ethyl acetate (EtOAc) fraction.
2. Materials and methods
2.1. Plant material
The fresh leaves of T. peruviana (Pers.) K. schum. were collected during the flowering stage in June 2019 from the Experimental Station of Medicinal and Aromatic Plants, Faculty of Pharmacy, Cairo University, Giza, Egypt. The identity was verified by Professor Dr Wafaa Mohamed Amer, Professor of Taxonomy, Faculty of Science, and Cairo University, Egypt. The plant was separately air-dried and kept in a tightly closed container. Voucher specimens of T. peruviana were deposited in the herbarium of the Department of Pharmacognosy, Faculty of Pharmacy, October 6 University (No. Th 2019-178).
2.2. Extraction and isolation
A sample of the air-dried powdered leaves (2 kg) T. peruviana was extracted by cold maceration in 70% aqueous methanol till exhaustion and the solvent was evaporated under reduced pressure and low temperature (50 °C) to yield dark green semi-solid sticky residue. The residue was suspended in the least amount of distilled water and successively extracted by liquid–liquid fractionation with n-hexane for defatting, methylene chloride, ethyl acetate and n-butanol saturated with water. Extracting solvents were evaporated under vacuum at 40 °C and yielded (25.23, 13.77, 10.50, and 2.89 gm) from n-hexane, methylene chloride, ethyl acetate, and n-butanol, respectively. The successive extractives were further screened and compared to the total methanol extract through chemical tests and TLC profiling. Identification of the compounds was done by NMR analysis and mass spectroscopy. 1H and 13C-NMR analyses were operated on 1H-NMR (400 MHZ) and 13C-NMR (100 MHZ) measured on a Bruker AVIIIHD 400 FT-NMR spectrometer (400/3) instrument (Japan) in suitable deuterated solvents (CD3OD or DMSO) using TMS as internal standard and chemical shift values expressed in δ ppm. (NMR Central Laboratory, Faculty of Pharmacy, Cairo University). EI/MS [40.00–1000.0].
2.3. Determination of the total phenolics
The concentration of total phenolics of the methanol extract of T. peruviana leaves was determined using the Folin–Ciocalteu method as described by Attard.20 Briefly, the procedure consisted of mixing 10 μL of sample/standard with 100 μL of Folin–Ciocalteu's reagent in a 96-well microplate, using gallic acid as a reference standard. Then, 80 μL of 1 M Na2CO3 was added and incubated at room temperature (25 °C) for 20 min in the dark. At the end of the incubation time, the resulting blue complex color was measured at 630 nm. For the quantification, a calibration curve of gallic acid was prepared by making serial dilutions in the concentration of (50–1000) mg mL−1. Gallic acid stock solution of 1 mg mL−1 in methanol was prepared, and 6 serial dilutions were prepared in the concentrations of (50, 100, 400, 600, 800 and 1000) μg mL−1. The results were expressed as mg of GAE per g of the tested extract.
2.4. Determination of the total flavonoids
The concentration of the total flavonoid content of the methanol extract of T. peruviana leaves was determined using the aluminum chloride colorimetric assay as described by Kiranmai21 with slight modifications to be carried out in microplates. Briefly, 15 μL of sample/standard was placed in a 96-well microplate, using rutin as a reference standard. Then, 175 μL of methanol was added, followed by 30 μL of 1.25% aluminum chloride.
Finally, 30 μL of 0.125 M of sodium acetate was added and incubated for 5 min. At the end of the incubation time, the resulting yellow color was measured at 420 nm. For quantification, a calibration curve of rutin was prepared by serial dilutions in the concentration of (10–1000) mg mL−1. While rutin stock solution of 1 mg mL−1 in methanol was prepared, and 7 serial dilutions were prepared in the concentrations of (10, 50, 100, 200, 400, 600, and 1000) μg mL−1. Tested sample of methanolic extract of the leaves of T. peruviana was prepared at NAWAH scientific Research Center at a concentration of (4 mg mL−1) in methanol. The results were expressed as mg of RE (rutin equivalent) per g of the extract.
2.5. RP HPLC analysis
RP HPLC analysis was carried out using an Agilent 1260 series. The separation was carried out using (Eclipse C18) reversed phase column (4.6 mm × 250 mm i.d., 5 μm). The mobile phase consisted of water (A) and 0.05% trifluoroacetic acid in acetonitrile (B) at a flow rate of 1 mL min−1. The mobile phase was programmed consecutively in a linear gradient as follows: 0 min (82% A); 0–5 min (80% A); 5–8 min (60% A); 8–12 min (60% A); 12–15 min (85% B) and 15–16 min (82% B). The multi-wavelength detector was adjusted at 280 nm. The injection volume was 10 μL for each of the sample solutions. The column temperature was maintained at 35 °C.
2.6. Cytotoxic activity
Dimethyl sulfoxide (DMSO), MTT, and trypan blue dyes were purchased from Sigma (St. Louis, Mo., USA). Fetal Bovine serum, DMEM, RPMI-1640, HEPES buffer solution, L-glutamine, gentamycin, and 0.25% trypsin-EDTA were purchased from Lonza. Human colon carcinoma (HCT-116), human lung carcinoma (A-549), and human breast carcinoma (MCF-7) cell lines were obtained from the American Type Culture Collection (ATCC, Rockville, MD). The cells were grown on RPMI-1640 medium supplemented with 10% inactivated fetal calf serum and 50 μg mL−1 gentamycin. The cells were maintained at 37 °C in a humidified atmosphere with 5% CO2 and were sub-cultured two to three times a week. For the antitumor assays, the tumor cell lines were suspended in the medium at a concentration of 5 × 104 cells per well in Corning® 96-well tissue culture plates and then incubated for 24 h. The tested samples were then added to 96-well plates (three replicates) to achieve twelve concentrations for each extract. Six vehicle controls with media or 0.5% DMSO were run for every 96 well plates as a control.
After 48 h of incubation, the number of viable cells was determined by the MTT test. Briefly, the media was removed from the 96 well plates and replaced with 100 μL of fresh culture RPMI 1640 medium without phenol red, followed by 10 μL of the 12 mM MTT stock solution (5 mg of MTT in 1 mL of PBS) to each well, including the untreated controls.
The 96 well plates were then incubated at 37 °C and 5% CO2 for 4 h and 85 μL aliquot of the media was removed from the wells, and 50 μL of DMSO was added to each well and mixed thoroughly with the pipette and incubated at 37 °C for 10 min. Then, the optical density was measured at 590 nm with the microplate reader (SunRise, TECAN, Inc., USA) to determine the number of viable cells, and the percentage of viability was calculated as [1 − (ODt/ODc)] × 100% where ODt is the mean optical density of wells treated with the tested sample and ODc is the mean optical density of untreated cells. The relation between surviving cells and drug concentration is plotted to get the survival curve of each tumor cell line after treatment with the specified extract. The 50% inhibitory concentration (IC50), the concentration required to cause toxic effects in 50% of intact cells, was estimated from graphic plots of the dose–response curve for each concentration using Graph pad Prism software (San Diego, CA. USA).
3. Results and discussion
3.1. Identification of the isolated compounds
In this study, five compounds, mainly polyphenolics were identified in the ethyl acetate fraction of T. peruviana leaves. Compounds were characterized by NMR and EI/MS. Also, identification was carried out by comparison with previously published data and/or authentic reference compounds. They were identified as: three phenolic acids (C1–C3) besides flavonol glycosides and their aglycone (C5 & C4) (Fig. 1; Tables 1 and 2). The following data demonstrated the spectroscopic and chromatographic behaviour of the isolated compounds.
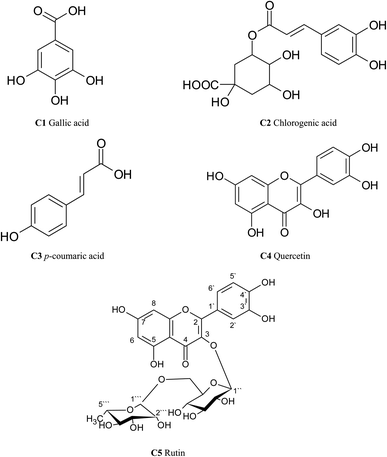 |
| Fig. 1 Chemical structures of the isolated compounds (C1–C5) of the Thevetia peruviana leaves. | |
Table 1 1HNMR spectroscopic data for T. peruviana isolated compounds C1–C5 a
Position |
C1 |
C2 |
C3 |
C4 |
C5 |
δ in ppm, J in Hz, (s): singlet, (brs): broad singlet (d): doublet, (dd): doublet of doublet, all compounds in DMSO. |
1 |
|
— |
— |
|
|
2 |
6.97(s) |
1.96(2H, brd,J = 5 Hz) |
7.78(1H,d,J = 8.6 Hz) |
— |
— |
3 |
|
5.25(1H, brd,J = 5 Hz) |
7.35(1H,d,J = 8.5 Hz) |
— |
— |
4 |
|
3.63(1H, dd,J = 8.6,3 Hz) |
— |
— |
— |
5 |
|
4.07(1H,ddd,J = 9.1,8.9,4.4 Hz) |
7.35(1H,d,J = 8.5 Hz) |
— |
— |
6 |
6.97(s) |
2.13(2H, m, H-6 quinic a') |
7.78(1H,d,J = 8.6 Hz) |
6.08(1H,d,J = 1.96 Hz) |
6.22(1H,d,J = 2.04 Hz) |
7 |
— |
— |
7.51(1H,d,J = 15.8 Hz) |
— |
— |
8 |
|
|
6.20(1H,d,J = 15.8 Hz) |
6.28(1H,d,J = 1.96 Hz) |
6.41(1H,d,J = 2.04 Hz) |
9 |
|
|
— |
— |
— |
10 |
|
|
|
— |
— |
1′ |
|
— |
|
— |
— |
2′ |
|
6.95(1H,d,J = 1.8 Hz) |
|
7.63(1H,d,J = 1.96 Hz) |
7.69(1H,d,J = 2.08 Hz) |
3′ |
|
— |
|
— |
— |
4′ |
|
— |
|
— |
— |
5′ |
|
6.68(1H,d,J = 8 Hz) |
|
6.79(1H,d,J = 8.4 Hz) |
6.90(1H,d,J = 8.4 Hz) |
6′ |
|
6.86(1H,dd,J = 8.2 Hz) |
|
7.54(1H,dd,J = 8.4,1.96 Hz) |
7.66(1H,dd,J = 8.4,2.1 Hz) |
7′ |
|
7.47(1H,d,J = 15.9 Hz) |
|
|
|
8′ |
|
6.18(1H,d,J = 15.9 Hz) |
|
|
|
9′ |
|
— |
|
|
|
1′′ |
|
|
|
|
5.13(1H,d,J = 7.56 Hz) |
2′′ |
|
|
|
|
3.65(1H,d,J = 1.4 Hz) |
3′′ |
|
|
|
|
3.41(1H,d,J = 9.8 Hz) |
4′′ |
|
|
|
|
3.55(1H,d,J = 11.04 Hz) |
5′′ |
|
|
|
|
3.37(1H,d,J = 14.9 Hz) |
6′′ |
|
|
|
|
a-3.66(1H,dd,J = 3.2,1.5 Hz) b-3.83(1H,d,J = 10.3 Hz) |
1′′′ |
|
|
|
|
4.54 |
2′′′ |
|
|
|
|
3.57(1H,d,J = 9.4 Hz) |
3′′′ |
|
|
|
|
3.32(1H,dd,J = 1.6,4.1 Hz) |
4′′′ |
|
|
|
|
3.49(1H,dd,J = 14.4,9.4 Hz) |
5′′′ |
|
|
|
|
3.45(1H,d,J = 8.4 Hz) |
6′′′ |
|
|
|
|
1.15(1H,d,J = 6.2 Hz) |
3.1.1. Compound C1. Yellowish-white needle crystals (30 mg), Rf value: 0.56 (BAW). It gives shine violet fluorescence under short UV light, turned to a deep blue color with FeCl3. Positive EI/MS: m/z 170.22 [M + H]+, which corresponds to the molecular formula C7H6O5. Also, the presence of characteristic fragment ion of gallic acid at m/z 125 is attributed to the loss of CO2 moiety [−m/z 44 m.u.] [M + H − CO2]+. 1H and 13C NMR data are presented in Tables 1 and 2. The compound was characterized as 3,4,5-trihydroxy-benzoic acid (gallic acid).15
Table 2 13C NMR of the isolated compounds C1–C5 a
|
C1 |
C2 |
C3 |
C4 |
C5 |
δ in ppm, all compounds in DMSO. |
1 |
120.7 |
74.9 |
125.9 |
|
|
2 |
108.9 |
36.8 |
131.9 |
146.6 |
157.1 |
3 |
144.9 |
72.2 |
115.4 |
135.8 |
134.2 |
4 |
138.2 |
70.6 |
159.7 |
175.9 |
177.9 |
5 |
144.9 |
70.1 |
115.4 |
161.1 |
161.6 |
6 |
108.9 |
37.54 |
131.9 |
97.9 |
98.6 |
7 |
169.1 |
176 |
145.2 |
164.3 |
164.9 |
8 |
|
— |
114.7 |
93.0 |
93.5 |
9 |
|
— |
161.9 |
156.8 |
157.9 |
10 |
|
— |
|
103.1 |
104.1 |
1′ |
|
126.4 |
|
122.7 |
121.7 |
2′ |
|
113.9 |
|
114.6 |
114.7 |
3′ |
|
145.7 |
|
144.8 |
144.4 |
4′ |
|
148.2 |
|
147.4 |
148.4 |
5′ |
|
115.1 |
|
114.8 |
116.3 |
6′ |
|
121.6 |
|
120.3 |
122.2 |
7′ |
|
145.4 |
|
|
|
8′ |
|
113.8 |
|
|
|
9′ |
|
167.3 |
|
|
|
1′′ |
|
|
|
|
103.4 |
2′′ |
|
|
|
|
74.3 |
3′′ |
|
|
|
|
76.8 |
4′′ |
|
|
|
|
70.8 |
5′′ |
|
|
|
|
75.8 |
6′′ |
|
|
|
|
67.2 |
1′′′ |
|
|
|
|
101.0 |
2′′′ |
|
|
|
|
70.7 |
3′′′ |
|
|
|
|
69.9 |
4′′′ |
|
|
|
|
72.5 |
5′′′ |
|
|
|
|
68.3 |
6′′′ |
|
|
|
|
16.5 |
3.1.2. Compound C2. White amorphous powder (25 mg), Rf value: 0.76 (BAW). It gave a blue fluorescence under UV light, changed to yellowish green on exposure to ammonia vapors, and a dark blue color with FeCl3, which indicates that it is phenolic in nature. Positive EI/MS: m/z 355.23 [M + H]+, which corresponds to the molecular formula C16H18O9. The fragmentation patterns showed signals at m/z 163 (caffeoyl) corresponding to [M + H-quinic acid]+. 1H and 13C NMR data are presented in Tables 1 and 2. The compound was characterized as 3-O-E-caffeoyl quinic acid (chlorogenic acid). This is the first time this compound has been isolated from the leaves of T. peruviana.16
3.1.3. Compound C3. Yellow amorphous crystals (40 mg), Rf value: 0.76 (BAW). It gave a yellow color under UV light and a dark blue color with FeCl3, which indicates its phenolic nature. The compound C3 showed [M+] ion at m/z 165.25, which corresponds to the molecular formula C9H8O3, which was also supported by NMR data. 1H and 13C NMR data are presented in Tables 1 and 2. Compound C3 is identified as p-coumaric acid.17
3.1.4. Compound C4. Dark yellow amorphous powder (35 mg), Rf value: 0.67 (BAW). It gave dark purple [long/short UV]; yellow fluorescence [UV/NH3], turned to yellow/Naturstoff's spray reagent, and green color with FeCl3, which is characteristic of flavonoids. Also, positive EI/MS: m/z 302.3 [M + H]+ calculated for structural formula C15H10O7. 1H and 13C NMR data are presented in Tables 1 and 2. Compound C4 was identified as 3,5,7,3′,4′, penta-oxygenated flavonoid derivative, which is in good agreement with quercetin.18
3.1.5. Compound C5. Yellow crystals (40 mg), Rf value: 0.39 (BAW). It was observed as a dark purple spot under UV-light, turned yellow fluorescence with NH3 vapors, orange fluorescence with Naturstoff, and green colour with FeCl3 spray reagents. Negative ESI/MS spectrum of compound C5 showed a molecular ion peak at m/z 609.2 [M–H]−, which corresponds to the molecular formula C27H30O16. 1H and 13C NMR data are presented in Tables 1 & 2. Compound C5 was identified as quercetin 3-O-α-L-1C4 – rhamnopyranosyl-(1′′′ → 6′′)-O-β-D-4C1-glucopyranoside (Rutin).19
3.2. Results of the total phenolics and total flavonoids
The total phenolic content (TPC) was determined using the Folin–Ciocalteu method for the assessment of polyphenols.20 The results were expressed as mg of GAE per g of the dried extract. TPC of the total methanol extract of the leaves of T. peruviana was determined spectrophotometrically at 630 nm, which is found to be 72.37 mg g−1. The flavonoid content of the total methanol extract of the leaves of T. peruviana was determined using the aluminum chloride colorimetric assay as described by Kiranmai.21 The basic principle of aluminium chloride colorimetric method is that aluminium chloride forms acid-stable complexes with the C-4 keto group and either the C-3 or C-5 hydroxyl group of flavones and flavonols. In addition, it also forms acid–labile complexes with the ortho-dihydroxyl groups in the A- or B-ring of flavonoids.21 The results were expressed as mg of rutin equivalent (RE) per g of the extracts.
Total flavonoids of the total methanol extract of the leaves of T. peruviana were determined spectrophotometrically at 420 nm, which is found to be 12.47 mg g−1.
3.3. RP HPLC analysis
Reversed Phase-High Performance Liquid Chromatography is the most well-known method for assessing phytochemicals present in various plant extracts. HPLC analysis of total methanol extract of leaves of T. peruviana was studied using RP-HPLC.
Fourteen polyphenolic compounds were identified as nine phenolic acids and five flavonoids by comparison with the retention times of authentic standards investigated under identical conditions. Concerning the polyphenolic compounds investigated in the leaves of T. peruviana, chlorogenic acid was the major phenolic acid (1769.56 μg g−1), followed by gallic acid (902.14 μg g−1) and coumaric acid (180.93 μg g−1). While naringenin flavanone was the highest concentration as the flavonoid compound present (324.74 μg g−1), followed by quercetin (212.08 μg g−1) and rutin (174.30 μg g−1). The results are compiled in Tables 3 and 4. According to a survey, one of the main classes of chemicals that function as antioxidants and anticancer agents is plant phenolics.22 Consequently, it is valuable to identify the phenolic components in the extracts of the studied T. peruviana.
Table 3 RP-HPLC analysis of the phenolics in the EtOAc extract of T. peruvianaa
Compound |
Retention time (min) |
Conc. of compounds (μg g−1) |
ND: not detected. |
Chlorogenic acid |
3.90 |
1769.56 |
Gallic acid |
3.09 |
902.14 |
Caffeic acid |
5.43 |
ND |
Coumaric acid |
8.24 |
180.93 |
Ellagic acid |
7.88 |
123.08 |
3,4-Dihydroxy-benzoic acid |
11.59 |
ND |
Vanillin |
8.86 |
38.69 |
Cinnamic acid |
13.27 |
8.32 |
Methyl gallate |
5.03 |
66.44 |
Ferulic acid |
9.50 |
154.09 |
Syringic acid |
5.93 |
55.53 |
Table 4 RP-HPLC analysis of the flavonoids in the EtOAc extract of T. peruvianaa
Compound |
Retention time (min) |
Conc. of compounds (μg g−1) |
ND: not detected. |
Rutin |
7.13 |
174.30 |
Quercetin |
9.40 |
212.08 |
Naringenin |
7.79 |
324.74 |
Hesperetin |
8.11 |
ND |
Apigenin |
2.36 |
ND |
Diadzein |
3.46 |
ND |
Catechin |
4.33 |
139.47 |
Luteolin |
6.92 |
ND |
Myricetin |
5.40 |
99.2 |
Kaempferol |
14.17 |
ND |
Taxifolin |
12.14 |
ND |
3.4. Cytotoxic evaluation
Cancer is an old disease that still threatens humanity. The three most common types of cancer in 2018 were lung, breast, and colorectal, according to WHO statistics.23 The American Cancer Society projects more men and women will die from lung and colorectal cancer, compared to any other cancer, in 2023. One key tool for the development of new anticancer medications with high specificity is the in vitro assessment of anticancer characteristics using cancer cell lines.24 In the present study, the cytotoxicity of different concentrations of the methanol extract of T. peruviana leaves (500, 250, 125, 62.5, 31.25, 15.6 μg mL−1), was assessed on three human cancer cell lines (HCT-116, A-549 and MCF-7). The anticancer activity was estimated in terms of percent growth inhibition (IC50 in μg mL−1) compared to untreated control cells. In comparison to cisplatin, the reference standard, the acquired data showed a direct cytotoxic effect with varied growth inhibition (Fig. 2–4). The tested extracts of T. peruviana resulted in a dose-dependent decrease in the surviving fraction of selected cancer cell lines. It reduced the cell proliferation of human lung carcinoma A-549 and human breast carcinoma MCF-7 cell lines with IC50 values of 93.4 and 110.3 μg mL−1, respectively. While it exhibited significant cytotoxic activity against human colon carcinoma HCT-116 cell line to the reference drug cisplatin, with IC50 values of 39.3 μg mL−1. This is consistent with research revealed that any extract had cytotoxic action when it had an IC50 value fewer than 1000 μg mL−1 after 24 h contact time.25 Natural phenolic substances have been found to cause cell cycle arrest by various methods at several cell stages, including G1, S, S-G2, and G2.26 Our polyphenolic HPLC results provided evidence in favour of the anticancer potential due to the existence of chlorogenic and gallic acids as the principal phenolic acids, whereas naringenin and quercetin were the main flavonoids. Chlorogenic acid can affect the cell cycle and ERK inactivation, which can lead to ROS generation and decrease cell viability in human colon cancer cells.27 The binding of annexin A2 by chlorogenic acid prevents the proliferation of human lung cancer A549 cell lines.28 Also, gallic acid has been shown to reduce the growth of colon cancer by preventing the phosphorylation of SRC and EGFR.29 In addition, naringenin also has anticancer effects due to the induction of apoptosis and cell cycle arrest in a variety of cancer cell lines, including MDA-MB-231 breast tumor cells, HepG2 human hepatocellular carcinoma cells, E0771 mammary tumor cells, and PC3 and LNCaP prostate cancer cells.30 Quercetin has an anti-tumor effect on colon cancer cells by suppressing catenin/Tcf signalling.31 Furthermore, because of synergistic interactions, combinations of natural products frequently exhibit additional advantageous properties than pure molecules.32 In conclusion, the major phenolic and flavonoid compounds present in T. peruviana support its cytotoxicity findings.
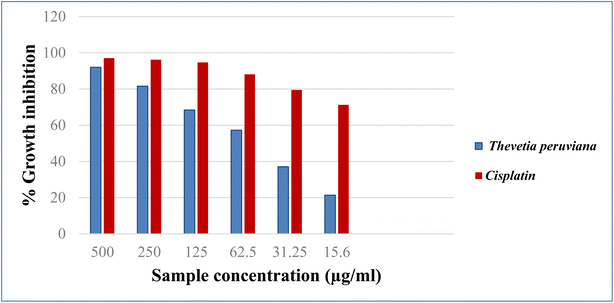 |
| Fig. 2 Growth inhibition percent of the tested extracts against colon carcinoma HCT-116 cell line. | |
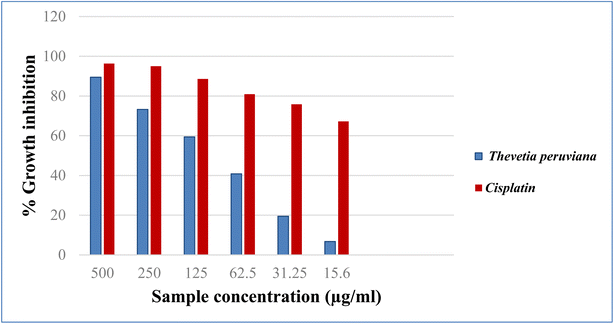 |
| Fig. 3 Growth inhibition percent of the tested extracts against lung carcinoma A-549 cell line. | |
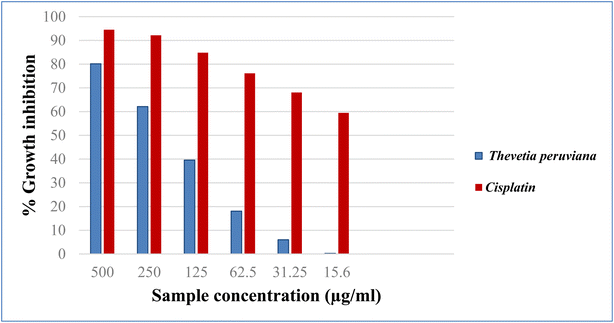 |
| Fig. 4 Growth inhibition percent of the tested extracts against breast carcinoma MCF-7 cell line. | |
4. Conclusion
Our study revealed that methanol extract of T. peruviana leaves has significant anticancer activity against the colon carcinoma HCT-116 cell line compared to the used reference drug cisplatin while exhibiting moderate activity against the lung carcinoma A-549 and breast carcinoma MCF-7 cell lines. Chemical analyses of the extract demonstrated a better evaluation of their biological significance. The anticancer potential of the plant may be attributed to the presence of 14 polyphenolic compounds in the methanol extract, which were identified by HPLC analysis. In addition to the isolation of five phenolic compounds from the EtOAc extract of the plant, T. peruviana leaves have a significant level of phenolic compounds, which should serve as a focus for future research on the mechanisms underlying the extract's cytotoxicity as well as the investigation of other pharmacological activities. Our results imply the merits of T. peruviana as a source of natural compound with anticancer potential.
Author contributions
Collection, drying, and extraction of the plant: S. A. E., identification of the isolated compounds through NMR analysis and mass spectroscopy: H. A. E. and N. A. S., conceptualization, and methodology: H. A. E. and N. A. S., writing, reviewing, revising and editing, all authors.
Conflicts of interest
The authors declare there is no conflict of interest.
References
- H. B. A. Rasool, Pharm. Anal. Acta, 2012, 3(10), 198 Search PubMed.
- E. A. Palombo, Evidence-Based Complementary Altern. Med., 2011, 1–15 CrossRef PubMed.
- G. N. P. Kumar, A. Atreya and T. Kanchan, Wildern. Environ. Med., 2015, 26(4), 590–591 CrossRef PubMed.
- A. Ramos-Silva, F. Tavares-Carreón, M. Figueroa, D. la Torre-Zavala, A. Gastelum-Arellanez, A. Rodríguez-García, L. J. Galán-Wong and H. Avilés-Arnaut, BMC Complementary Altern. Med., 2017, 17(1), 1–11 CrossRef PubMed.
- P. C. Standley and L. O. Williams, Flora of Guatemala. Parts XII. Fieldiana. Botany, Chicago, 1976 Search PubMed.
- K. K. Samal, H. K. Sahu and P. Gopalakrishnakone, J. Wilderness Med., 1992, 3(4), 382–386 CrossRef.
- T. Ahmad, A. Hamid, A. Sharma and U. Bhardwaj, Int. J. Adv. Res., 2017, 5(8), 486–493 CrossRef PubMed.
- N. Rahman, H. Rahman, M. Haris and R. Mahmood, J. Tradit. Complementary Med., 2017, 7(4), 519–525 CrossRef PubMed.
- K. B. Pandey and S. I. Rizvi, Oxid. Med. Cell. Longevity, 2009, 2(5), 270–278 CrossRef PubMed.
- F. Bray, J. Ferlay, I. Soerjomataram, R. L. Siegel, L. A. Torre and A. Jemal, Ca-Cancer J. Clin., 2018, 68(6), 394–424 CrossRef PubMed.
- C. Yao, J. Zhang, J. Li, W. Wei, S. Wu and D. Guo, Nat. Prod. Rep., 2021, 38(9), 1618–1633 RSC.
- W. Liu, B. Yang, L. Yang, J. Kaur, C. Jessop, R. Fadhil, D. Good, G. Ni, X. Liu, T. Mosaiab, Z. Yi and M. Wei, J. Evidence-Based Complementary Altern. Med., 2019, 2019, 6057837 Search PubMed.
- T. Mosmann, J. Immunol. Methods, 1983, 65(1–2), 55–63 CrossRef CAS PubMed.
- S. M. Riyadh, S. M. Gomha, E. A. Mahmmoud and M. M. Elaasser, ChemInform, 2015, 46(38), 38–150 CrossRef.
- G. Meshram, B. Patil, S. Yadav and D. Shined, Int. J. Res. Ayurveda Pharm., 2011, 2, 559–562 CAS.
- U. H. Jin, J. Y. Lee, S. K. Kang, J. K. Kim, W. H. Park, S. K. Moon and J. G. Kim, Life Sci., 2005, 77(22), 2760–2769 CrossRef CAS PubMed.
- S. Al-Madhagy, N. M. Mostafa, F. S. Youssef, G. Awad, O. A. Eldahshan and A. N. B. Singab, Egypt. J. Chem., 2019, 62(10), 1869–1877 Search PubMed.
- V. S. Saraswathi, D. Saravanan and K. Santhakumar, J. Photochem. Photobiol., B, 2017, 171, 20–26 CrossRef PubMed.
- S. Georgeta, P. Pana, H. Tunde and B. Sanda, An. Univ. Oradea Fasc. Ecotoxicol. Zooteh. Tehnol. Ind. Aliment, 2016, 109–113 Search PubMed.
- E. Attard, Cent. Eur. J. Biol., 2013, 8(1), 48–53 CAS.
- M. Kiranmai, C. B. M. Kumar and I. Mohammed, Res. J. Pharm., Biol. Chem. Sci., 2011, 2(3), 254–261 CAS.
- P. G. Pietta, J. Nat. Prod., 2000, 63(7), 1035–1042 CrossRef CAS PubMed.
- F. Bray, J. Ferlay, I. Soerjomataram, R. L. Siegel, L. A. Torre and A. Jemal, Ca-Cancer J. Clin., 2018, 68(6), 394–424 CrossRef PubMed.
- A. Itharat, P. J. Houghton, E. Eno-Amooquaye, P. J. Burke, J. H. Sampson and A. Raman, J. Ethnopharmacol., 2004, 90(1), 33–38 CrossRef PubMed.
- Y. Gavamukulya, F. Abou-Elella, F. Wamunyokoli and H. AEl-Shemy, Asian Pac. J. Trop. Med., 2014, 7, S355–S363 CrossRef CAS PubMed.
- J. Dai and R. J. Mumper, Molecules, 2010, 15(10), 7313–7352 CrossRef CAS PubMed.
- N. Hou, N. Liu, J. Han, Y. Yan and J. Li, Anti-Cancer Drugs, 2017, 28(1), 59–65 CrossRef CAS PubMed.
- L. Wang, H. Du and P. Chen, Biomed. Pharmacother., 2020, 131, 110673 CrossRef CAS PubMed.
- X. Lin, G. Wang, P. Liu, L. Han, T. Wang, K. Chen and Y. Gao, Exp. Ther. Med., 2021, 21(6), 1–11 Search PubMed.
- J. Choi, D. Lee, H. Jang, S. Park and J. Seol, Int. J. Med. Sci., 2020, 17(18), 3049–3057, DOI:10.7150/ijms.44804.
- C. H. Park, J. Y. Chang, E. R. Hahm, S. Park, H. K. Kim and C. H. Yang, Biochem. Biophys. Res. Commun., 2005, 328(1), 227–234 CrossRef CAS PubMed.
- L. K. Caesar and N. B. Cech, Nat. Prod. Rep., 2019, 36(6), 869–888 RSC.
|
This journal is © The Royal Society of Chemistry 2023 |
Click here to see how this site uses Cookies. View our privacy policy here.