DOI:
10.1039/D3QO00591G
(Research Article)
Org. Chem. Front., 2023,
10, 3193-3200
Galvinoxyl-inspired dinitronyl nitroxide: structural, magnetic, and theoretical studies†
Received
21st April 2023
, Accepted 12th May 2023
First published on 16th May 2023
Abstract
A novel galvinoxyl-inspired dinitronyl nitroxide (1) has been synthesized. Structural analysis revealed that 1 exhibited a resonance structure, resulting from p-benzoquinonediimine N,N′-dioxide and N-phenyl nitroxide moieties. The magnetic study revealed an intramolecular exchange-coupling constant J/kB of −761(3) K in H = −J(S1·S2 + S2·S3), indicating a ground doublet state. Theoretical calculations suggested not only the strong intramolecular antiferromagnetic coupling but also a unique electron configuration owing to a remarkable spin polarization effect.
Introduction
Organic radicals have many uses in the fields of spin trapping,1,2 spin labeling,3,4 dynamic nuclear polarization,5,6 organic catalysts,7,8 photochromic materials,9,10 and molecular magnetic materials.11–16 An understanding of not only the physical properties but also the chemical stability of these unique radical-based materials is required. There are two approaches to stabilize radical species: (i) delocalization of the radical spin on the π-conjugated system and (ii) steric protection around the radical centre with bulky substituents.17
Galvinoxyl reported by Coppinger is well known as a stable radical (Fig. 1a).18 The unpaired electron is delocalized throughout the molecule, and the tert-butyl groups play a role in steric protection. These factors impart both thermodynamic and kinetic stabilities to the molecule. As shown in Fig. 1a, galvinoxyl can be illustrated as a triradical, but it behaves as a monoradical species due to strong intramolecular antiferromagnetic coupling. Such triradicals with a doublet ground state (S = 1/2) have been reported several times.19–22 Interestingly, galvinoxyl exhibits a difference in the spin polarization of the α and β spins owing to the strong intramolecular antiferromagnetic coupling; namely, the β-HOMO level is elevated to the α-SOMO level, where the SOMO and HOMO represent singly and doubly highest occupied molecular orbitals, respectively.23,24 This electron configuration is known as a partial SOMO–HOMO inversion (SHI),24 as shown in Fig. 1b. Owing to the thermal stability and the unique electron structure, several galvinoxyl analogues have been reported where the chemical modification is mainly at the methine position.25–36
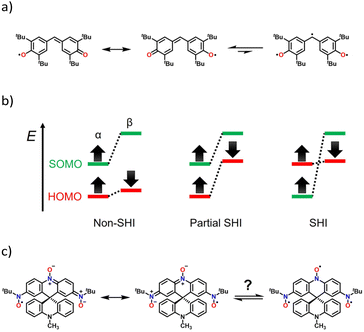 |
| Fig. 1 Resonance and equilibrium schemes of (a) galvinoxyl and (c) dinitronyl nitroxide 1. (b) Illustration for three electronic configurations, non-SHI, partial SHI, and SHI. | |
In this study, we have synthesized a galvinoxyl-inspired dinitronyl nitroxide, 2,7-bis(N-tert-butyl-N-oxylamino)-10′-methyl-9,9′(10H,10′H)spirobiacridin-10-oxyl (1). The N-oxy-acridine side of 1 is an isoelectronic structure with galvinoxyl (Fig. 1c); namely, two oxygen and methine sites in galvinoxyl are replaced by nitroxides/nitrones. The NO site is more thermodynamically stable than phenoxy (>C–O˙) due to a local resonance structure of the >N–O˙ and >N˙+–O− forms. This allows the removal of the tert-butyl substituents used in galvinoxyl, and a six-membered ring can be created in the central moiety. The latter improves the planarity of the whole molecule, resulting in strong intramolecular antiferromagnetic coupling. For 1, the formation of the spiro structure with N-methyl-9(10H)acridine not only forms the central six-membered ring but also introduces steric protection. According to Iwamura's work, the 1,4-phenylene bisnitroxide moiety could be stabilized as a quinoid form, p-benzoquinonediimine N,N′-dioxide.37 Therefore, 1 should favour the dinitronyl nitroxide monoradical structure (the left side in Fig. 1c). In fact, magnetic and theoretical studies of 1 exhibited the ground doublet state (S = 1/2). The MO diagram exhibited the partial SHI state as well as galvinoxyl, owing to the remarkable spin polarization effect.
Results and discussion
Synthesis and characterization
Compound 1 was synthesized by following the procedure shown in Scheme S1.† Black platelet polycrystals of 1 were obtained by recrystallization from CH2Cl2 and n-hexane. Product 1 was characterized through spectroscopic and X-ray crystallographic analyses. The decomposition temperature of 1 was 168 °C, where the thermal stability of 1 is similar to that of galvinoxyl (153 °C).18
The X-band electron spin resonance (ESR) spectrum of 1 in a toluene solution at room temperature (rt) is shown as a red line in Fig. 2. The spectrum was a major triplet splitting with several minor ones, indicating the hyperfine structures for one 14N and three 1H atoms. This pattern indicates that 1 in solution exhibits dinitronyl nitroxide as shown in Fig. 1c. It was also found that the radical spin could be localized in one benzenoid moiety. This finding is also reported in the study of galvinoxyl.26,38,39 When bulky substituents such as tert-butyl or 1-adamantyl are introduced at the methine site of galvinoxyl, two phenyl rings are twisted, resulting in the unpaired electron being confined to one phenoxy ring. By means of simulation using the EasySpin software,40 the hyperfine constants, g values, and the linewidth of peak-to-peak (lwpp) were determined as aN = 1.17 mT, aH1 = 0.252 mT, aH2 = 0.182 mT, aH3 = 0.087 mT, gxx = 2.0037, gyy = 2.0069, gzz = 2.0096, and lwpp = 0.164 mT. The simulation spectrum (a black line in Fig. 2), derived from the above parameters, well reproduced the experimental one. For 1, two N–O sites could be twisted along a single bond relative to the acridine ring, leading to a situation similar to that of the galvinoxyl derivative with bulky substituents.
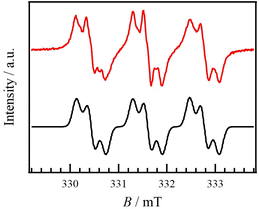 |
| Fig. 2 X-Band ESR spectrum of 1 in a degassed toluene solution at 298 K. The top and bottom spectra represent the experimental and simulation, respectively. For the optimized parameters in the simulation, see the text. | |
Time-dependent ESR measurements were performed under ambient conditions to assess the stability of 1 in solution (Fig. S1a†). The time dependence of the maximum intensities in the central peak is shown in Fig. S1b.† Fitting of the data shows an exponential decay of the ESR intensity, but the intensities did not reach zero for the measurement times. The hyperfine structure (aH) gradually disappeared, resulting in a simplified triplet splitting pattern. This finding can be attributed to the infiltration of O2 into the solution during storage.
Single-crystal X-ray crystallography
The crystal structure of 1 was evaluated at 93 K, and it crystallized in a monoclinic P
space group (Table S1†). There were two crystallographically independent molecules in a unit cell (Fig. 3a). Two independent molecules (1a and 1b) are represented in Fig. 3b and c, respectively. There were disordered CH2Cl2 crystal solvents, which were accounted for in the SQUEEZE/PLATON program, and the electron count was 72 electrons per formula unit. This value is close to the 1.7 CH2Cl2 solvents (71 electrons). The N1–O1, N2–O2, and N3–O3 bond lengths of 1a are 1.285(2), 1.295(2), and 1.281(2) Å, respectively, and the N5–O4, N6–O5, and N7–O6 bond lengths of 1b are 1.285(3), 1.287(3), and 1.284(2) Å, respectively. These bond lengths are shorter than the average of about 1.45 Å for hydroxylamine compounds,41–47 indicating successful oxidation. Three N–O sites in 1 show either nitroxides or nitrones, as shown in Fig. 1b. However, since both nitrones and nitroxides have an N–O length of about 1.3 Å,16,19,37,48–85 the bond length between the N and neighbouring sp2 carbon atoms is important to determine the electron state of the N–O site. In fact, there is a difference in the N–Csp2 length; the average lengths for nitrones are 1.31 Å (N–O: 1.29 Å),48–53 those for aryl tert-butyl nitroxides are 1.43 Å (N–O: 1.29 Å),16,19,37,45,54–71 and those for 10-oxy-9(10H)acridines are 1.41 Å (N–O: 1.29 Å).72–75 The compound 4,4,5,5-tetramethylimidazoline-3-oxide-1-oxyl, known as nitronyl nitroxide, has both nitrone and nitroxide moieties and thus an N–Csp2 length of 1.34 Å (N–O: 1.28 Å),41,54,55,76–85 which is intermediate between those of nitrone and nitroxide species. For 1a and 1b, the N–Csp2 lengths are 1.380(3)–1.387(3) and 1.373(3)–1.396(4) Å, respectively. These values are close to those of nitronyl nitroxides, indicating a dinitronyl nitroxide structure (the left-side structures in Fig. 1b).
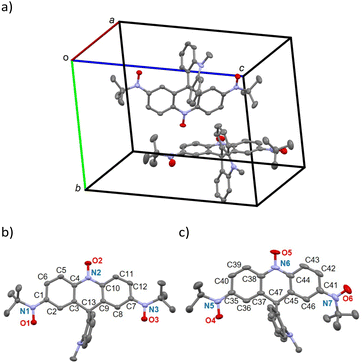 |
| Fig. 3 Crystal structures of (a) asymmetry units in the unit cell and molecular structures of (b) 1a and (c) 1b. Thermal ellipsoids for non-hydrogen atoms are drawn at the 50% probability level. The H atoms and solvent molecules are omitted for clarity. | |
Dinitronyl nitroxide 1 possesses benzenoid and quinoid substructures, and the quinoid one shows bond alternation and reduction of aromaticity. In fact, 1,4-phenylene bis(tert-butyl nitroxide) (2) shows alternating C–C bond lengths (Table 1).37 On the other hand, chemical modifications shifted the equilibrium in favour of the benzenoid form due to (i) linker elongation and (ii) steric hindrance. For example, the former are 4,4′-biphenyl- and 4,4′′-1,1′:4′,1′′-terphenylene bis(tert-butyl nitroxide) compounds (3 and 4, respectively),86 and the latter is 2,3,5,6-tetramethoxy-1,4-phenylene bis(tert-butyl nitroxide) (5).37 Selected bond lengths and torsion angles of 1a and 1b are summarized in Table 1. For comparison purpose, the data of 2–5 are also listed. A harmonic oscillator model for aromaticity (HOMA) value is useful for clarifying comparisons of bond lengths.87,88 Four phenyl rings, C1–C6 (A) and C7–C12 (B) for 1a and C35–C40 (C) and C41–C46 (D) for 1b, show HOMA values of 0.844, 0.853, 0.806, and 0.922, respectively. For 1a, the values are intermediate between those of the quinoid form 2 and the benzenoid forms 3–5, indicating the delocalized radical spin throughout the acridine moiety. On the other hand, the HOMA values of the C and D rings in 1b are smaller and larger, respectively, compared to 1a. This finding implies that the C and D rings favour the quinoid and benzenoid forms, respectively. This situation is also supported by the large torsion angle between the D ring and the N–O site.
Table 1 Selected structural parameters and HOMA values for 1a, 1b, and 2–5
The nearest intermolecular NO⋯NO distance is 3.943(2) Å of O2⋯O2a. This value is larger than the sum of the van der Waals radii of O/O of 3.04 Å,89 indicating no direct radical–radical contact. On the other hand, three O atoms, O2, O5b, and O6c, face each other around the C5 atom, as shown in Fig. S2.† The O2⋯O5, O5⋯O6, and O6⋯O2 distances of 4.007(3), 4.055(3), and 4.330(2) Å, respectively, are larger than the sum of vdW radii (O/O; 3.04 Å), while the O2⋯C5, O5⋯C5, and O6⋯C5 distances of 2.700(2), 3.058(3), and 3.237(2) Å, respectively, are smaller than it (C/O; 3.22 Å). These contacts between three O atoms through an H atom on the C5 atom could induce magnetic interaction.
Magnetic properties
Magnetic susceptibility was measured for the polycrystal sample 1 at 2–350 K (Fig. 4a). Note that the molecular weight is calculated excluding the crystalline solvent because the exact amount of solvent cannot be calculated due to the gradual desorption of the solvent during storage. Upon cooling from 350 K, the χmT value gradually decreased to a plateau at 100–10 K. This antiferromagnetic behaviour implies a depopulation from the quartet excited state (S = 3/2) to the doublet ground state (S = 1/2). On the other hand, the plateau value of about 0.32 cm3 K mol−1 was smaller than the calculated χmT value of 0.378 cm3 K mol−1 derived from S = 1/2 and g = 2.0067, where the g value is from the ESR study. This difference implies the presence of the residual crystal solvents in the sample. Upon further cooling from 10 K, the χmT value decreased sharply, indicating intermolecular antiferromagnetic coupling. The experimental data of 1 were analysed with eqn (1) based on the linear three S = 1/2 centre model, Ĥ = −J(Ŝ1·Ŝ2 + Ŝ2·Ŝ3).90 |  | (1) |
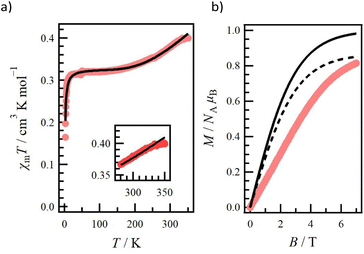 |
| Fig. 4 (a) Temperature dependence of χmT for 1, measured at B = 0.5 T. A solid line shows the fitting curve. The inset shows an enlarged view of the 280 to 350 K region. (b) M vs. B at 2.0 K. The solid line shows the Brillouin function with S = 1/2 and g = 2.0067. The dashed line is multiplied by 0.8668. | |
A Weiss mean-field parameter θ and a parameter f are introduced in order to estimate the intermolecular interaction in the low-temperature region and the amount of the residual CH2Cl2 as a crystal solvent, respectively. The g value was set at 2.0067. The best-fit curve was achieved with J/kB = −761(3) K, θ = −1.22(3) K, and f = 0.8668(9) (a black line in Fig. 4a). The J value was negative, indicating the doublet ground state arising from the through-bond antiferromagnetic interaction, while the magnitude of J is discussed in the theoretical calculations below. The θ value was negative and small due to indirect contacts (Fig. S4†). From the f value of 0.8668(9), we estimated the amount of the CH2Cl2 crystal solvent required for the calculated molecular weight, resulting in 0.991 molecules. This value is less than the 1.7 molecules observed in the above structural study, suggesting desorption of the solvent during storage. Above 330 K, the experimental χmT deviated from the fitting line (the inset of Fig. 4a), and this may be triggered by the desorption of the residual crystal solvents.
The field-dependence of the magnetization curve at 2 K is shown in Fig. 4b. The M value was not saturated and reached 0.814μB at 7 T. Compared to the black solid line in Fig. 4b, which is derived from the Brillouin function with S = 1/2 and g = 2.0067, there is a difference in the scale of the experimental and simulation curves. Therefore, the black dashed line in Fig. 4b is drawn, considering the purity factor f = 0.8668. The magnitude of M in the simulation is well in agreement with the experimental curve, whereas the experimental curve is within the simulation. This result indicates an antiferromagnetic interaction, supporting the negative θ constant.
Theoretical studies
The density functional theory (DFT) calculations on 1a and 1b were performed using the atomic coordination determined from the crystallographic study. For reducing calculation cost, the N-methyl acridine ring and tert-butyl groups were replaced with H atoms and methyl groups, respectively. The calculated spin densities of the doublet (d) and quartet (q) states for 1a and 1b have been mapped onto the molecular skeleton shown in Fig. 5. There is a difference in the maps of the doublet state in 1a and 1b. For 1a, the red and blue lobes, which represent positive and negative spin densities, appeared alternately along the π-conjugation system owing to the spin polarization (Fig. 5a). On the other hand, for 1b, two-coloured lobes appeared alternately only on the left-hand six-membered ring, while on the right-hand one, the spin density is strongly localized at the N–O site. The quartet and doublet energy states for 1a were Eq = −969.95114196 au with 〈S2〉q = 3.7501 and Ed = −969.97229443 au with 〈S2〉d = 0.8284, respectively. Those parameters for 1b were Eq = −969.94969131 au with 〈S2〉q = 3.7501, and the Ed energy was −969.96185149 au with 〈S2〉d = 0.8112. The ΔEq–d values of 1a and 1b were determined to be +0.5756 and +0.3309 eV, respectively. The exchange-coupling constant J/kB values for 1a and 1b are also −1589 and −908 K, respectively, calculated by the approximate spin-projection method,91J = (Ed − Eq)/(〈S2〉q − 〈S2〉d). These findings indicate that both 1a and 1b show the ground doublet state.
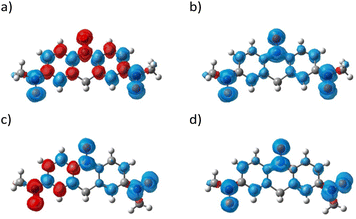 |
| Fig. 5 Spin-density maps illustrating the DFT results for the (a) doublet and (b) quartet states of 1a and the (c) doublet and (d) quartet states of 1b. Those atomic positions were taken from the crystallographic study. Blue and red lobes indicate positive and negative spin densities, respectively, with the isocontour of 0.002 e Å−3. | |
The molecular orbitals (MOs) of the doublet state for 1a and 1b are shown in the left and right columns of Fig. 6, respectively. The α- and β-MO1–3 levels in 1a and 1b are obviously separated from the other MO levels, and thus these MOs play the key role in determining the spin state. For 1a, α-MO2 and β-MO2 (−5.53 and −2.94 eV), α-MO1 and β-MO3 (−2.79 and −5.42 eV), and α-MO3 and β-MO1 (−5.77 and −2.42 eV) are paired, respectively, according to the comparison of those MO maps shown in Fig. 6. The orbital lobes of β-MO3 are positioned to avoid those of α-MO2, reducing electron repulsion; namely, there is a remarkable effect of spin polarization. This situation implies intramolecular antiferromagnetic coupling between the terminal and central N–O sites, providing a partial SHI state like galvinoxyl. On the other hand, there is a difference in the MO diagram of 1b and 1a. According to the structural study, the unpaired electron of 1b is localized on the N7–O6 site with the C42–C47 (D) ring. Those MO maps shown in Fig. 6 imply that α-MO1 and β-MO3 (−2.73 and −5.61 eV), α-MO2 and β-MO1 (−5.03 and −2.19 eV), and α-MO3 and β-MO2 (−6.10 and −3.24 eV) are paired. For the occupied MOs, α-MO2, α-MO1, and β-MO3, the arrangement of the molecular lobes on the left-side ring is affected by spin polarization, which is supported by the quinoid form observed in the structural study. However, only the α spin exists on the right-side ring. These results suggest that the radical spin is localized at the N–O site on the right-side ring. The differences in spin densities and MOs of 1a and 1b were caused by the degree of tilt of the N–O site to the acridine ring.
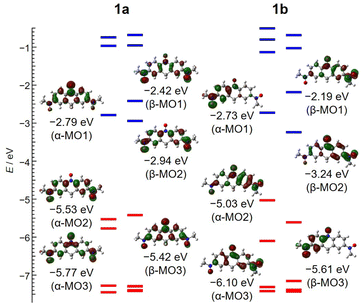 |
| Fig. 6 Molecular-orbital (MO) energy-level diagram for α and β spins in the doublet state of (left) 1a and (right) 1b (isovalue = 0.03). Blue and red colours represent unoccupied and occupied orbitals, respectively. Selected MO images are superposed. | |
Conclusions
We have prepared the novel galvinoxyl-inspired dinitronyl nitroxide 1, which shows a ground doublet state (S = 1/2) as confirmed by magnetic measurements. Compound 1a shows high coplanarity between the acridine and N–O sites. The unpaired electron is delocalized throughout the acridine ring, resulting in thermodynamic stability similar to that of galvinoxyl. DFT calculations reveal strong intramolecular antiferromagnetic coupling and a remarkable spin polarization effect. On the other hand, in 1b, one N–O site is twisted relative to the acridine ring, and the radical spin is localized to one phenyl ring. According to the ESR study, in solution, the radical spin localizes either on the phenyl rings due to the configuration of the N–O sites or the N-methyl acridine sites. Therefore, these studies on 1 reveal that the SOMO and HOMO electronic configurations are highly responsive to the conformation of the N–O sites. This highly responsive electronic structure makes it possible to produce a switching material that is triggered by the external environment.
Conflicts of interest
There are no conflicts to declare.
Acknowledgements
This study was financially supported by KAKENHI (JSPS, 20K22538). Prof. Takayuki Ishida (The University of Electro-Communications) kindly lent HPLC (Japan Analytical Industry, 1H + 1H GPC columns; a flow rate of 3.5 mL min−1) for the isolation of 1. The crystallography study for 1 was supported by “Advanced Research Infrastructure for Materials and Nanotechnology in Japan (ARIM)” of the Ministry of Education, Culture, Sports, Science and Technology (MEXT), Grant Number A-22-UT-0080.
References
- E. G. Janzen, Spin trapping, Acc. Chem. Res., 1971, 4, 31–40 CrossRef CAS.
- M. J. Davies, Detection and characterisation of radicals using electron paramagnetic resonance (EPR) spin trapping and related methods, Methods, 2016, 109, 21–30 CrossRef CAS PubMed.
- J. F. W. Keana, Newer aspects of the synthesis and chemistry of nitroxide spin labels, Chem. Rev., 1978, 78, 37–64 CrossRef CAS.
- A. Bonucci, O. Ouari, B. Guigliarelli, V. Belle and E. Mileo, In-Cell EPR: Progress towards Structural Studies Inside Cells, ChemBioChem, 2020, 21, 451–460 CrossRef CAS PubMed.
- O. Haze, B. Corzilius, A. A. Smith, R. G. Griffin and T. M. Swager, Water-Soluble Narrow-Line Radicals for Dynamic Nuclear Polarization, J. Am. Chem. Soc., 2012, 134, 14287–14290 CrossRef CAS PubMed.
- D. Wisser, G. Karthikeyan, A. Lund, G. Casano, H. Karoui, M. Yulikov, G. Menzildjian, A. C. Pinon, A. Purea, F. Engelke, S. R. Chaudhari, D. Kubicki, A. J. Rossini, I. B. Moroz, D. Gajan, C. Copéret, G. Jeschke, M. Lelli, L. Emsley, A. Lesage and O. Ouari, J. Am. Chem. Soc., 2018, 140, 13340 CrossRef CAS PubMed.
- L. Li, R. Matsuda, I. Tanaka, H. Sato, P. Kanoo, H. J. Jeon, M. L. Foo, A. Wakamiya, Y. Murata and S. Kitagawa, A Crystalline Porous Coordination Polymer Decorated with Nitroxyl Radicals Catalyzes Aerobic Oxidation of Alcohols, J. Am. Chem. Soc., 2014, 136, 7543–7546 CrossRef CAS PubMed.
- P. Qu, M. Kuepfert, S. Jockusch and M. Weck, Compartmentalized Nanoreactors for One-Pot Redox-Driven Transformations, ACS Catal., 2019, 9, 2701–2706 CrossRef CAS.
-
R. Dessauer, Photochemistry, History, and Commercial Applications of Hexaarylbiimidazoles, Elsevier, 2006 Search PubMed.
- A. Tokunaga, L. M. Uriarte, K. Mutoh, E. Fron, J. Hofkens, M. Sliwa and J. Abe, Photochromic Reaction by Red Light via Triplet Fusion Upconversion, J. Am. Chem. Soc., 2019, 141, 17744–17753 CrossRef CAS PubMed.
- I. Ratera and J. Veciana, Playing with organic radicals as building blocks for functional molecular materials, Chem. Soc. Rev., 2012, 41, 303–349 RSC.
- N. M. Gallagher, A. Olankitwanit and A. Rajca, High-Spin Organic Molecules, J. Org. Chem., 2015, 80, 1291–1298 CrossRef CAS PubMed.
- E. Jin, M. Asada, Q. Xu, S. Dalapati, M. A. Addicoat, M. A. Brady, H. Xu, T. Nakamura, T. Heine, Q. Chen and D. Jiang, Two-dimensional sp2 carbon–conjugated covalent organic frameworks, Science, 2017, 357, 673–676 CrossRef CAS PubMed.
- N. Nakamura, K. Inoue, H. Iwamura, T. Fujioka and Y. Sawaki, Synthesis and characterization of a branched-chain hexacarbene in a tridecet ground state. An approach to superparamagnetic polycarbenes, J. Am. Chem. Soc., 1992, 114, 1484–1485 CrossRef CAS.
- A. Rajca and S. Utamapanya, Toward organic synthesis of a magnetic particle: dendritic polyradicals with 15 and 31 centers for unpaired electrons, J. Am. Chem. Soc., 1993, 115, 10688–10694 CrossRef CAS.
- J. Fujita, Y. Matsuoka, K. Matsuo, M. Tanaka, T. Akita, N. Koga and H. Iwamura, Molecular structure and magnetic properties of N,N-bis{4-methoxy-3,5-bis(N-tert-butyl-N-oxyamino)phenyl)}aminoxyl. An approach to a stable and high-spin pentaradical, Chem. Commun., 1997, 2393–2394 RSC.
- B. Tang, J. Zhao, J.-F. Xu and X. Zhang, Tuning the stability of organic radicals: from covalent approaches to non-covalent
approaches, Chem. Sci., 2020, 11, 1192–1204 RSC.
- G. M. Coppinger, A stable phenoxy radical inert to oxygen, J. Am. Chem. Soc., 1957, 79, 501–502 CrossRef CAS.
- S. Suzuki, A. Nagata, M. Kuratsu, M. Kozaki, R. Tanaka, D. Shiomi, K. Sugisaki, K. Toyota, K. Sato, T. Takui and K. Okada, Trinitroxide-Trioxytriphenylamine: Spin-State Conversion from Triradical Doublet to Diradical Cation Triplet by Oxidative Modulation of a π-Conjugated System, Angew. Chem., Int. Ed., 2012, 51, 3193–3197 CrossRef CAS PubMed.
- X. Lu, S. Lee, Y. Hong, H. Phan, T. Y. Gopalakrishna, T. S. Herng, T. Tanaka, M. E. Sandoval-Salinas, W. Zeng, J. Ding, D. Casanova, A. Osuka, D. Kim and J. Wu, Fluorenyl Based Macrocyclic Polyradicaloids, J. Am. Chem. Soc., 2017, 139, 13173–13183 CrossRef CAS PubMed.
- T. Kodama, M. Aoba, Y. Hirao, S. M. Rivero, J. Casado and T. Kubo, Molecular and Spin Structures of a Through-Space Conjugated Triradical System, Angew. Chem., Int. Ed., 2022, 61, e20220688 CrossRef PubMed.
- S. Tang, H. Ruan, Z. Hu, Y. Zhao, Y. Song and X. Wang, A cationic sulfur-hydrocarbon triradical with an excited quartet state, Chem. Commun., 2022, 58, 1986–1989 RSC.
- K. Awaga, T. Sugano and M. Kinoshita, Ferromagnetic intermolecular interaction in the galvinoxyl radical: Cooperation of spin polarization and charge-transfer interaction, Chem. Phys. Lett., 1987, 141, 540–544 CrossRef CAS.
- S. Kasemthaveechok, L. Abella, J. Crassous, J. Autschbach and L. Favereau, Organic radicals with inversion of SOMO and HOMO energies and potential applications in optoelectronics, Chem. Sci., 2022, 13, 9833–9847 RSC.
- N. C. Yang and A. J. Castro, Synthesis of a stable biradical, J. Am. Chem. Soc., 1960, 82, 6208 CrossRef CAS.
- K. Hinrichs, B. kirste, H. Kurreck and J. Reusch, Über galvinole und galvinoxyle—IV: Untersuchung dynamischer effekte an galvinoxyl-dublett-radikalen mit endor-in-lösung, Tetrahedron, 1977, 33, 151–154 CrossRef CAS.
- B. Kirste, M. Grimm and H. Kurreck, EPR, proton and carbon-13 ENDOR studies of a quintet state 13C-labeled galvinoxyl-type tetraradical, J. Am. Chem. Soc., 1989, 111, 108–114 CrossRef CAS.
- D. A. Shultz and A. K. Boal, Nitroxyl-Galvinoxyl – A New Biradical, Mol. Cryst. Liq. Cryst., 1995, 272, 75–79 CrossRef.
- D. A. Shultz and Q. Zhao, Preparation and characterization of a bis-galvinoxyl-disulfide, Tetrahedron Lett., 1996, 37, 8837–8840 CrossRef.
- K. K. Anderson, D. A. Shultz and D. A. Dougherty, Attempted Synthesis of a Stable, Quintet, Tetraphenoxyl Tetraradical: Facile Rearrangement of a Substituted Bicyclobutane, J. Org. Chem., 1997, 62, 7575–7584 CrossRef CAS.
- M. Miyashita, T. Yamazaki and H. Nishide, Poly(3-phenylgalvinoxylthiophene). A New Conjugated Polyradical with High Spin Concentration, Polym. J., 2001, 33, 849–856 CrossRef.
- P. Wautelet, P. Turek and J. Le Moigne, Synthesis of Phenyl Ethynylene Coupled Biradicals and Polyradicals Based on Galvinoxyl, Synthesis, 2002, 1286–1292 CAS.
- E. Fukuzaki, N. Takahashi, S. Imai, H. Nishide and A. Rajca, Synthesis of Dendritic, Non-Kekulé-, and Nondisjoint-type Triphenylmethanes Terminated with Galvinoxyl Radicals, Polym. J., 2005, 37, 284–293 CrossRef CAS.
- T. Kaneko, H. Karagiri, Y. Umeda, T. Namikoshi, E. Marwanta, M. Teraguchi and T. Aoki, Optically active helical structure and magnetic interaction of poly(phenylacetylene)-based polyradicals, Polyhedron, 2009, 28, 1927–1929 CrossRef CAS.
- T. Jähnert, B. Häupler, T. Janoschka, M. D. Hager and U. S. Schubert, Synthesis and Charge–Discharge Studies of Poly(ethynylphenyl)galvinoxyles and Their Use in Organic Radical Batteries with Aqueous Electrolytes, Macromol. Chem. Phys., 2013, 214, 2616–2623 CrossRef.
- T. Chi, S. Akkiraju, Z. Liang, Y. Tan, H. J. Kim, X. Zhao, B. M. Savoie and B. W. Boudouris, Design of an n-type low glass transition temperature radical polymer, Polym. Chem., 2021, 12, 1448–1457 RSC.
- S. Nakazono, S. Karasawa, N. Koga and H. Iwamura, Stabilization of p-Phenylenebis(N-tert-butylaminoxyl) Relative to p-Benzoquinonediimine N,N′-Dioxide, Angew. Chem., Int. Ed., 1998, 37, 1550–1552 CrossRef CAS PubMed.
- B. Kirste, W. Harrer, H. Kurreck, S. H. Bauer and W. Gierke, Hydrogen-1 and carbon-13 ENDOR investigations of sterically hindered galvinoxyl radicals, J. Am. Chem. Soc., 1981, 103, 6280–6286 CrossRef CAS.
- J. von Gersdorff, B. Kirste, D. Niethammer, W. Harrer and H. Kurreck, EPR and ENDOR investigations of dynamic processes in sterically overcrowded phenoxyl-type galvinoxyl radicals, Magn. Reson. Chem., 1988, 26, 416–424 CrossRef CAS.
- S. Stoll and A. Schweiger, EasySpin, a comprehensive software package for spectral simulation and analysis in EPR, J. Magn. Reson., 2006, 178, 42–55 CrossRef CAS PubMed.
- C. Hirel, K. E. Vostrikova, J. Pécaut, V. I. Ovcharenko and P. Rey, Nitronyl and Imino Nitroxides: Improvement of Ullman's Procedure and Report on a New Efficient Synthetic Route, Chem. – Eur. J., 2001, 7, 2007–2014 CrossRef CAS PubMed.
- J. K. Bjernemose and A. D. Bond, N,N-Bis[2-(2-pyridyl)ethyl]hydroxylamine, Acta Crystallogr., Sect. E: Struct. Rep. Online, 2004, 60, o1143 CrossRef CAS.
- J. R. Malpass, P. S. Skerry and S. L. Rimmington, Meisenheimer and Other Rearrangements of N-Oxides Derived from 2-Azabicyclo[2.2.1]hept-5-enes; the Influence of Reaction Conditions and Stereochemistry at Nitrogen, Heterocycles, 2004, 62, 679–691 CrossRef CAS PubMed.
- C. Berini, F. Minassian, N. Pelloux-Léon, J.-N. Denis, Y. Vallée and C. Philouze, Efficient stereoselective nucleophilic addition of pyrroles to chiral nitrones, Org. Biomol. Chem., 2008, 6, 2574–2586 RSC.
- A. Okazawa, Y. Nagaichi, T. Nogami and T. Ishida, Magneto-Structure Relationship in Copper(II) and Nickel(II) Complexes Chelated with Stable tert-Butyl 5-Phenyl-2-pyridyl Nitroxide and Related Radicals, Inorg. Chem., 2008, 47, 8859–8868 CrossRef CAS PubMed.
- P. A. Tziouris, C. G. Tsiafoulis, M. Vlasiou, H. N. Miras, M. P. Sigalas, A. D. Keramidas and T. A. Kabanos, Interaction of Chromium(III) with a N,N′-Disubstituted Hydroxylamine-(diamido) Ligand: A Combined Experimental and Theoretical Study, Inorg. Chem., 2014, 53, 11404–11414 CrossRef CAS PubMed.
- I. Golomolzina, S. Tolstikov, G. Letyagin, G. Romanenko, A. S. Bogomyakov, A. Y. Akyeva, M. A. Syroeshkin, M. P. Egorov, V. Morozov and V. Ovcharenko, Cu(hfac)2 Complexes with Acyclic Nitroxide Prone to Single-Crystal to Single-Crystal Transformation and Showing Mechanical Activity, Cryst. Growth Des., 2022, 22, 6148–6167 CrossRef CAS.
- A. M. Lobo, S. Prabhakar, H. S. Rzepa, A. C. Skapski, M. R. Tavares and D. A. Widdowson, C-substitution reactions of c,n-diaryl nitrones, Tetrahedron, 1983, 39, 3833–3841 CrossRef CAS.
- J.-G. Kang, J.-P. Hong and I.-H. Suh, N,-(4-Cyanophenyl)-α-(4-methoxyphenyl)nitrone, Acta Crystallogr., Sect. C: Cryst. Struct. Commun., 2000, 56, 231–232 CrossRef PubMed.
- R. Herrera, A. Nagarajan, M. A. Morales, F. Méndez, H. A. Jiménez-Vázquez, L. G. Zepeda and J. Tamariz, Regio- and Stereoselectivity of Captodative Olefins in 1,3-Dipolar Cycloadditions. A DFT/HSAB Theory
Rationale for the Observed Regiochemistry of Nitrones, J. Org. Chem., 2001, 66, 1252–1263 CrossRef CAS PubMed.
- H. Hopf, A. A. Aly, V. N. Swaminathan, L. Ernst, I. Dix and P. G. Jones, A Simple Route to a Pyridinyl[2.2]paracyclophane, Eur. J. Org. Chem., 2005, 68–71 CrossRef CAS.
- C. Riplinger, J. P. Y. Kao, G. M. Rosen, V. Kathirvelu, G. R. Eaton, S. S. Eaton, A. Kutateladze and F. Neese, Interaction of Radical Pairs Through-Bond and Through-Space: Scope and Limitations of the Point–Dipole Approximation in Electron Paramagnetic Resonance Spectroscopy, J. Am. Chem. Soc., 2009, 131, 10092–10106 CrossRef CAS PubMed.
- W. Frey, A. Baskakova, A. Menzel and V. Jäger, Crystal structure of 2,3-O-cyclohexylidene-D-glyceraldehyde N-benzylnitrone, C16H21NO3, Z. Kristallogr. - New Cryst. Struct., 2010, 225, 245–246 CAS.
- M. Tanaka, K. Matsuda, T. Itoh and H. Iwamura, Syntheses and Magnetic Properties of Stable Organic Triradicals with Quartet Ground States Consisting of Different Nitroxide Radicals, J. Am. Chem. Soc., 1998, 120, 7168–7173 CrossRef CAS.
- Y. Hosokoshi, K. Katoh, Y. Nakazawa, H. Nakano and K. Inoue, Approach to a Single-Component Ferrimagnetism by Organic Radical Crystals, J. Am. Chem. Soc., 2001, 123, 7921–7922 CrossRef CAS PubMed.
- T. Itoh, K. Matsuda, H. Iwamura and K. Hori, The Ground Spin States of Tris[p-(N-oxyl-N-tert-butylamino)phenyl] amine, -Methyl, and -Borane. Prospects of Further Studies, J. Solid State Commun., 2001, 159, 428–439 CrossRef CAS.
- J. Ohshita, T. Iida, N. Ohta, K. Komaguchi, M. Shiotani and A. Kunai, Synthesis of Phenylnitroxides Bridged by an sp3-Linkage, Org. Lett., 2002, 4, 403–406 CrossRef CAS PubMed.
- D. A. Shultz, R. M. Fico Jr., H. Lee, J. W. Kampf, K. Kirschbaum, A. A. Pinkerton and P. D. Boyle, Mechanisms of Exchange Modulation in Trimethylenemethane-type Biradicals: The Roles of Conformation and Spin Density, J. Am. Chem. Soc., 2003, 125, 15426–15432 CrossRef CAS PubMed.
- G. Kurokawa, T. Ishida and T. Nogami, Remarkably strong intermolecular antiferromagnetic couplings in the crystal of biphenyl-3,5-diyl bis(tert-butyl nitroxide), Chem. Phys. Lett., 2004, 392, 74–79 CrossRef CAS.
- O. B. Borobia, P. Guionneau, H. Heise, F. H. Köhler, L. Ducasse, J. Vidal-Gancedo, J. Veciana, S. Golhen, L. Ouahab and J.-P. Sutter, Discrepancy between the Spin Distribution and the Magnetic Ground State for a Triaminoxyl Substituted Triphenylphosphine Oxide Derivative, Chem. – Eur. J., 2005, 11, 128–139 CrossRef PubMed.
- Z. Delen and P. M. Lahti, Crystallography and Magnetism of Two 1-(4-Nitroxylphenyl)pyrroles, J. Org. Chem., 2006, 71, 9341–9347 CrossRef CAS PubMed.
- H. Nishimaki, S. Mashiyama, M. Yasui, T. Nogami and T. Ishida, Bistable Polymorphs Showing Diamagnetic and Paramagnetic States of an Organic Crystalline Biradical Biphenyl-3,5-diyl Bis(tert-butylnitroxide), Chem. Mater., 2006, 18, 3602–3604 CrossRef CAS.
- N. Roques, P. Gerbier, U. Schatzschneider, J.-P. Sutter, P. Guionneau, J. Vidal-Gancedo, J. Veciana, E. Rentschler and C. Guérin, Experimental and Theoretical Studies of Magnetic Exchange in Silole-Bridged Diradicals, Chem. – Eur. J., 2006, 12, 5547–5562 CrossRef CAS PubMed.
- H. Nishimaki and T. Ishida, Organic Two-Step Spin-Transition-Like Behavior in a Linear S = 1 Array: 3′-Methylbiphenyl-3,5-diyl Bis(tert-butylnitroxide) and Related Compounds, J. Am. Chem. Soc., 2010, 132, 9598–9599 CrossRef CAS PubMed.
- T. Konno, H. Kudo and T. Ishida, Intermediate-paramagnetic phases with a half and a quarter spin entities in fluorinated biphenyl-3,5-diyl bis(tert-butyl nitroxides), J. Mater. Chem. C, 2015, 3, 7813–7918 RSC.
- H. Kawakami, A. Tonegawa and T. Ishida, Pyridine-2,6-diyl dinitroxides as room-temperature triplet ligands, AIP Conf. Proc., 2016, 1709, 020017 CrossRef.
- H. Kawakami, A. Tonegawa and T. Ishida, A designed room-temperature triplet ligand from pyridine-2,6-diyl bis(tert-butyl nitroxide), Dalton Trans., 2016, 45, 1306–1309 RSC.
- T. Yoshitake, H. Kudo and T. Ishida, Thermally Activated Paramagnets from Diamagnetic Polymers of Biphenyl-3,5-diyl Bis(tert-butyl Nitroxides) Carrying Methyl and Fluoro Groups at the 2′- and 5′-Positions, Crystals, 2016, 6, 30 CrossRef.
- N. Koizumi and T. Ishida, Forced proximity of nitroxide groups in pincer compounds with a xanthene spacer, Tetrahedron Lett., 2017, 58, 2804–2808 CrossRef CAS.
- A. Okazawa, Y. Terakado, T. Ishida and N. Kojima, A triplet biradical with double bidentate sites based on tert-butyl pyridyl nitroxide as a candidate for strong ferromagnetic couplers, New J. Chem., 2018, 42, 17874–17878 RSC.
- H. Han, D. Zhang, Z. Zhu, R. Wei, X. Xiao, X. Wang, Y. Liu, Y. Ma and D. Zhao, Aromatic Stacking Mediated Spin–Spin Coupling in Cyclophane-Assembled Diradicals, J. Am. Chem. Soc., 2021, 143, 17690–17700 CrossRef CAS PubMed.
- M. Nakagawa, T. Ishida, M. Suzuki, D. Hashizume, M. Yasui, F. Iwasaki and T. Nogami, Intermolecular ferromagnetic interaction in the crystal of a diphenyl nitroxide derivative. The role of spin-polarized hydrogen atoms located near a neighboring N–O site, Chem. Phys. Lett., 1999, 302, 125–131 CrossRef CAS.
- T. Ishida, M. Ooishi, N. Ishii, H. Mori and T. Nogami, Mono- and dinitroxide radicals from 9,9′(10H,10′H)-spirobiacridine: An approach to a D2d triplet biradical, Polyhedron, 2007, 26, 1793–1799 CrossRef CAS.
- T. Kanetomo, K. Ichihashi, M. Enomoto and T. Ishida, Ground Triplet Spirobiradical: 2,2′,7,7′-Tetra(tert-butyl)-9,9′(10H,10′H)-spirobiacridine-10,10′-dioxyl, Org. Lett., 2019, 21, 3909–3912 CrossRef CAS PubMed.
- K. Ichihashi, T. Kanetomo, M. Enomoto and T. Ishida, 2,7-Di-tert-butyl-9,9′(10H,10′H)-spirobiacridine-10,10′-dioxyl as a ground triplet biradical: The role of tert-butylation, Tetrahedron Lett., 2020, 61, 152428 CrossRef CAS.
- A. Zheludev, V. Berone, M. Bonnet, B. Delley, A. Grand, E. Ressouche, P. Rey, R. Subra and J. Schweizer, Spin density in a nitronyl nitroxide free radical. Polarized neutron diffraction investigation and ab initio calculations, J. Am. Chem. Soc., 1994, 116, 2019–2027 CrossRef CAS.
- M. M. Matsushita, A. Izuoka, T. Sugawara, T. Kobayashi, N. Wada, N. Takeda and M. Ishikawa, Hydrogen-Bonded Organic Ferromagnet, J. Am. Chem. Soc., 1997, 119, 4369–4379 CrossRef CAS.
- R. Akabane, M. Tanaka, K. Matsuo, N. Koga, K. Matsuda and H. Iwamura, Crystal Structures and Magnetic Properties of m-Phenylenebis(imidazole) Derivatives Having Two Nitronyl Nitroxide or Iminyl Nitroxide Radicals. The Two Kinds of Antiferromagnetic Interaction Alternating along One-Dimensional Chains, J. Org. Chem., 1997, 62, 8854–8861 CrossRef CAS.
- N. L. Frank, R. Clérac, J.-P. Sutter, N. Daro, O. Kahn, C. Coulon, M. T. Green, S. Golhen and L. Ouahab, Synthesis, Crystal Structure, Magnetic, and Electron Paramagnetic Resonance Properties of a Spiroconjugated Biradical. Evidence for Spiroconjugation Exchange Pathway, J. Am. Chem. Soc., 2000, 122, 2053–2061 CrossRef CAS.
- J. Nakazaki, I. Chung, M. M. Matsushita, T. Sugawara, R. Watanabe, A. Izuoka and Y. Kawada, Design and preparation of pyrrole-based spin-polarized donors, J. Mater. Chem., 2003, 13, 1011–1022 RSC.
- P. Brough, R. Chiarelli, J. Pécaut, A. Rassat and P. Rey, A versatile
synthesis of new pyrimidinyl nitronyl nitroxides, Chem. Commun., 2003, 2722–2723 RSC.
- Y. Masuda, M. Kuratasu, S. Suzuki, M. Kozaki, D. Shiomi, K. Sato, T. Takui, Y. Hosokoshi, X.-Z. Lan, Y. Miyazaki, A. Inaba and K. Okada, A New Ferrimagnet Based on a Radical-Substituted Radical Cation Salt, J. Am. Chem. Soc., 2009, 131, 4670–4673 CrossRef CAS PubMed.
- K. Kolanji, L. Postulka, B. Wolf, M. Lang, D. Schollmeyer and M. Baumgarten, Planar Benzo[1,2-b:4,5-b’]dithiophene Derivatives Decorated with Nitronyl and Imino Nitroxides, J. Org. Chem., 2019, 84, 140–149 CrossRef CAS PubMed.
- E. V. Tretyakov, P. V. Petunin, S. I. Zhivetyeva, D. E. Gorbunov, N. P. Gritsan, M. V. Fedin, D. V. Stass, R. I. Samoilova, I. Y. Bagryanskaya, I. K. Shundrina, A. S. Bogomyakov, M. S. Kazantsev, P. S. Postnikov, M. E. Trusova and V. I. Ovcharenko, Platform for High-Spin Molecules: A Verdazyl-Nitronyl Nitroxide Triradical with Quartet Ground State, J. Am. Chem. Soc., 2021, 143, 8164–8176 CrossRef CAS PubMed.
- C. Shu, M. Pink, T. Junghoefer, E. Nadler, S. Rajca, M. B. Casu and A. Rajca, Synthesis and Thin Films of Thermally Robust Quartet (S = 3/2) Ground State Triradical, J. Am. Chem. Soc., 2021, 143, 5508–5518 CrossRef CAS PubMed.
- P. Ravat and M. Baumgarten, “Tschitschibabin type biradicals”: benzenoid or quinoid?, Phys. Chem. Chem. Phys., 2015, 17, 983–991 RSC.
- J. Kruszewski and T. M. Krygowski, Definition of aromaticity basing on the harmonic oscillator model, Tetrahedron Lett., 1972, 13, 3839–3842 CrossRef.
- T. M. Krygowski, Crystallographic studies of inter- and intramolecular interactions reflected in aromatic character of .pi.-electron systems, J. Chem. Inf. Comput. Sci., 1993, 33, 70–78 CrossRef CAS.
- A. Bondi, van der Waals Volumes and Radii, J. Phys. Chem., 1964, 68, 441–451 CrossRef CAS.
-
O. Kahn, Molecular Magnetism, VCH-Verlag, Weinheim, New York, 1993 Search PubMed.
- T. Onishi, D. Yamaki, K. Yamaguchi and Y. Takano, Theoretical calculations of effective exchange integrals by spin projected and unprojected broken-symmetry methods. I. Cluster models of K2NiF4-type solids, J. Chem. Phys., 2003, 118, 9747–9761 CrossRef CAS.
|
This journal is © the Partner Organisations 2023 |
Click here to see how this site uses Cookies. View our privacy policy here.