DOI:
10.1039/D2NJ02026B
(Communication)
New J. Chem., 2023,
47, 51-55
Synthesis and antimicrobial activity of novel bis-benzimidazolium salts†
Received
25th April 2022
, Accepted 28th October 2022
First published on 11th November 2022
Abstract
For decades, bacterial resistance has been a significant threat to the efficiency of modern treatments of many serious illnesses requiring the continuous development of new strategies and antibiotics. One of the most common mechanisms used by microorganisms to develop resistance is the formation of biofilms. In this study, we developed new compounds that are able to prevent the formation of biofilms and disrupt the mature formed biofilms. We have synthesized compounds containing benzimidazolium cations, a hydrophobic scaffold, and different apolar side groups. Their antibacterial activity on planktonic microorganisms, ability to prevent the formation of Methicillin-resistant S. aureus (MRSA) biofilms and to disrupt mature MRSA biofilms identify them as promising candidates in the search for new avenues to fight the increase of microbial resistance.
Introduction
Bacterial infections with resistant microorganisms are one of the biggest challenges in hospitals as they are responsible for a significant number of complications during and after hospitalization.1 The development of new antibacterial agents without previous use and fast activity can limit the spread of bacterial infections. Penicillin's discovery by Fleming in 1939 made possible the complete eradication of some bacterial infections but strains resistant to this antibiotic were identified shortly after its extensive commercialization. This scenario was repeated a few years later with methicillin, a penicillin analogue, when Staphylococcus aureus (S. aureus) rapidly developed resistance to this antibiotic.1,2 The increase of bacterial resistance to antibiotics is therefore a major concern that is becoming increasingly topical, which requires the constant development of new antibacterial agents, with old or new modes of action.3 Biofilm formation is one of the most common mechanisms developed by microorganisms to survive in hostile environments and is responsible for many chronic infections.4–6 New compounds able to interfere with the bacterial biofilm formation cycle, inhibiting biofilm formation or dispersing pre-formed biofilm, are constantly needed.
Previously, various organic cationic compounds demonstrated strong antibacterial and antibiofilm properties.7–9 The amphiphilic nature of these compounds (Fig. 1a) is brought by the presence of two benzimidazolium cations substituted with two phenylethynylbenzyl groups. These compounds have shown a higher activity against Methicillin-resistant S. aureus (MRSA) and against biofilms than commercial cationic compounds, which is attributed to their ability to self-assemble in phospholipid membranes and induce membrane permeability. Herein, we designed and studied compounds containing phenylphenylethylene or pyridylphenylethylene scaffolds (Fig. 1b).
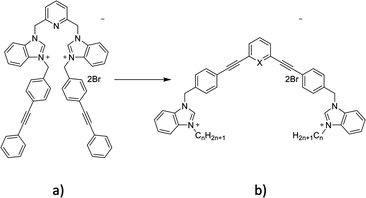 |
| Fig. 1 Structure of the previously reported compounds and those presented in this study. | |
Results and discussion
The new amphiphilic compounds contain two benzimidazolium cations, which are linked through a rigid scaffold and possess different alkyl side chains. The use of a rigid conjugated scaffold between the two benzimidazolium cations may improve their ability to self-assemble through π stacking and enhance their compatibility with the apolar environment of phospholipid bilayers.10,11 Various biological tests were carried out to determine their antibiofilm properties. This was achieved by determining the minimum inhibitory concentration (MIC) of the compounds on planktonic bacteria. We then used this concentration as well as twice that concentration (2xMIC) to determine the lowest concentration at which the compounds can inhibit or destroy biofilms.12
The general procedure for the synthesis of new compounds is shown in Scheme 1.
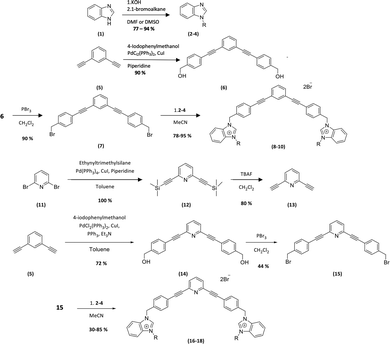 |
| Scheme 1 Synthesis of the bis-benzimidazolium salts. 2, 8, 16 (R = CH3), 3, 9, 17 (R = C4H9), 4, 10, 18 (R = C8H17). | |
The first step of the synthesis is the functionalization of benzimidazole (1) through nucleophilic substitution under basic conditions to obtain alkyl benzimidazole compounds (2–4). A Sonogashira cross coupling is then carried out between 1,3-diethylbenzene and 4-iodobenzylalcohol (5), followed by a bromination reaction, to achieve the dibromo intermediate (7). Finally, compounds 8–10 are obtained after nucleophilic substitution with benzimidazoles (2–4).9
In order to obtain the pyridyl analogs 16–18, diethynylpyridine (13) was prepared by a Sonogashira cross coupling reaction between ethyltrimethylsilane and dibromopyridin (11), followed by a deprotection step. Analogs 16–18 were then prepared using the method described above.13,14
Antimicrobial activity
The ability to inhibit 90% of bacterial growth for compounds 8–10 and 16–18 was studied on Gram-positive Methicillin-resistant S. aureus (ATCC 43300) (MRSA), Vancomycin-resistant E. faecium (BAA-2316) (VRE), Gram-negative E. coli (SK039), and yeasts C. albicans (SC5314).
The results summarized in Table 1 show that all compounds present antimicrobial activities against Gram-positive bacteria (MSRA and VRE) and C. albicans and are more potent than commercial compounds. However, compound 16 is less active against these strains despite its similar hydrophilicity compared to compound 8. In the case of Gram-negative bacteria E. coli (SK039), we observed a stronger antibacterial activity for compounds 9 and 17 with MICs below 10 μg mL−1 (see the Experimental part). Gram-negative bacteria possess an additional external phospholipid bilayer compared to Gram-positive bacteria, which may explain why the other compounds are less able to penetrate and disturb their membranes.15 The lack of activity of compounds 8 and 16 in Gram negative bacteria is probably due to their more hydrophilic character and inability to pass through both external and internal membranes. Compounds 9, 10, 17 and 18 seem to have the right hydrophobicity that permits their insertion into both membranes, which results in membrane perturbation and the ability to kill these microorganisms.
Table 1 Minimum inhibitory concentration (MIC) in μg mL−1 on different microorganisms, haemolytic activity on Human Red Blood cells (HC5) in μg mL−1 and measured log
P*
Antimicrobial |
MRSA |
VRE |
E. Coli
|
C. albicans
|
HC5 |
Log P |
MRSA = Methicillin-resistant S. aureus (ATCC 43300), VRE = vancomycin-resistant E. faecium (BAA-2316), E. coli (SK037), C. albicans (SC5314), HC5: concentration of antimicrobial at which 5% of Human Red Blood cells are lysed, log P: the partition coefficient (octanol/water). |
8
|
2 ± 0.02 |
2 ± 0.02 |
100 ± 0.03 |
10 ± 0.03 |
0.03 |
−0.12 |
9
|
1 ± 0.02 |
0.5 ± 0.006 |
5 ± 0.01 |
5 ± 0.02 |
0.8 |
2.88 |
10
|
2 ± 0.03 |
4 ± 0.01 |
>100 |
10 ± 0.003 |
1 |
3.35 |
16
|
12.5 ± 0.01 |
100 ± 0.03 |
200 ± 0.03 |
50 ± 0.02 |
N. D |
−0.59 |
17
|
2.5 ± 0.006 |
5 ± 0.02 |
10 ± 0.02 |
10 ± 0.03 |
N. D |
2.03 |
18
|
2 ± 0.03 |
2 ± 0.01 |
>100 |
12.5 ± 0.07 |
1 |
3.18 |
Penicillin |
>400 |
>400 |
100 ± 0.02 |
>100 |
N. D |
N. D |
Kanamycin |
>400 |
>400 |
>100 |
>100 |
N. D |
N. D |
Ampicillin |
>400 |
50 ± 0.006 |
<12.5 |
N. D |
N. D |
N. D |
Streptomycin |
>400 |
>400 |
<12.5 |
N. D |
N. D |
N. D |
Trimethoprim |
50 ± 0.02 |
>400 |
>40 |
>100 |
N. D |
N. D |
BAC |
>100 |
N. D |
10 |
50 |
N. D |
N. D |
It should be also mentioned that compounds 8 and 16 may have different aggregation behaviours in the phospholipid bilayers compared to the ones bearing longer alkyl chains that may act like surfactants once inserted in the membrane. Independently of the type of microorganisms and the composition of their cellular membranes, the protonation of the pyridine moiety of compounds 16–18 does not seem to affect their capacity to interact with membranes and increase their antimicrobial activity, compounds 10 and 18 presenting very similar activities in all the studied strains.
Antibiofilm activity
The ability of these new compounds to inhibit the formation and disrupt mature S. aureus biofilms was studied using commercially available fluorescent markers (Baclight Live/Dead).16 The results obtained are summarized in Fig. 2 and 3 for the inhibition and in Fig. 4 for the destruction studies.
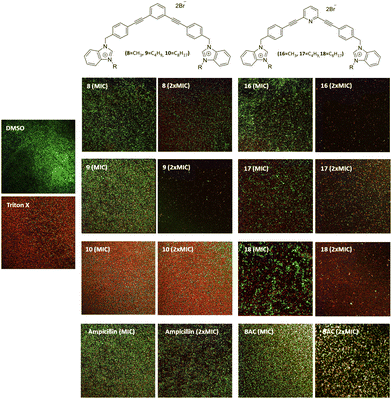 |
| Fig. 2 Representative images inhibition of MRSA biofilms labeled with Live/Dead stains after 48 h incubation at the MIC and 2xMIC of the antimicrobials. DMSO 10% (negative control); Triton X (positive control); compound 8 (MIC: 2 μg mL−1, 2xMIC: 4 μg mL−1); compound 9 (MIC: 1 μg mL−1, 2xMIC: 2 μg mL−1); compound 10 (MIC: 2 μg mL−1, 2xMIC: 4 μg mL−1); compound 16 (MIC: 12.5 μg mL−1, 2xMIC: 25 μg mL−1); compound 17 (MIC: 2.5 μg mL−1, 2xMIC: 5 μg mL−1); compound 18 (MIC: 2 μg mL−1, 2xMIC: 4 μg mL−1); ampicillin (1 : 200 μg mL−1; 2 : 400 μg mL−1); BAC (1 : 100 μg mL−1, 2 : 200 μg mL−1). | |
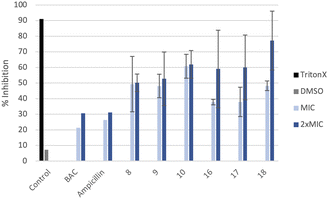 |
| Fig. 3 Inhibition of MRSA biofilms labeled with Live/Dead stains after 48 h incubation at the MIC and 2xMIC of the antimicrobials calculated using the ImageJ software (average of three images). Negative control (DMSO 10%) and positive control (Triton X). | |
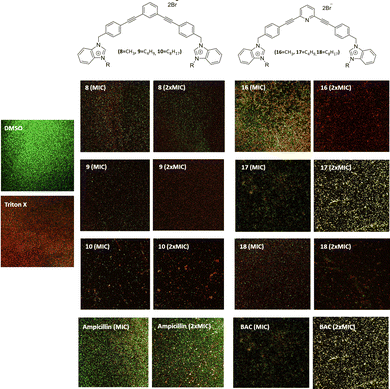 |
| Fig. 4 Representative images of the destruction of mature MRSA biofilms labeled with Live/Dead stains after 48 h incubation at the MIC and 2xMIC of the antimicrobials. DMSO 10% (negative control); Triton X (positive control); compound 8 (MIC: 2 μg mL−1, 2xMIC: 4 μg mL−1); compound 9 (MIC: 1 μg mL−1, 2xMIC: 2 μg mL−1); compound 10 (MIC: 2 μg mL−1, 2xMIC: 4 μg mL−1); compound 16 (MIC: 12.5 μg mL−1, 2xMIC: 25 μg mL−1); compound 17 (MIC: 2.5 μg mL−1, 2xMIC: 5 μg mL−1); compound 18 (MIC: 2 μg mL−1, 2xMIC: 4 μg mL−1); ampicillin (MIC: 200 μg mL−1; 2xMIC: 400 μg mL−1); BAC (MIC: 100 μg mL−1, 2xMIC: 200 μg mL−1). | |
Ability to inhibit the formation of MRSA biofilms
Confocal imaging of untreated MRSA biofilms (Fig. 2) shows the formation of living and homogeneous bacterial biofilms. Once treated with compounds 8 and 9 at their MICs or 2xMIC, an inhibition of 50% of the biofilm can be observed. When compound 9 is used at its 2xMIC, the formation of a thinner biofilm can be observed, suggesting a higher capacity of inhibition for this compound at this concentration. Compound 10 induces 60% inhibition of the biofilm formation when used at its MIC and 2xMIC. Compounds 16 and 17 show a similar ability to inhibit the formation of the biofilm at their MICs (38%) and greater activity at their 2xMICs (60%). When the biofilm is treated with compound 18 at 2xMIC, a significant inhibition (77%) can be observed.
Compounds 10 and 18 bearing octyl chains possess higher inhibition activity at 2xMICs (Fig. 2). These results suggest that most lipophilic compounds possess better inhibition activity for biofilm formation. We have already observed this trend with octyl-functionalized imidazolium or benzimidazolium salts.9 Moreover, when the activity of these salts on the inhibition of MRSA biofilm formation is compared to the commercially and widely used benzalkonium chloride (BAC) or ampicillin, it should be noted that it is significantly higher.
Ability to destroy mature MRSA biofilms
All compounds have a better ability to disrupt mature biofilms when compared to their ability to inhibit biofilm formation. Thereby, it can be seen that all studied compounds possess a good capacity to disrupt mature biofilms at their MIC and very good destruction activity at 2xMIC. However, compounds 9, 16 and 18 are very efficient to disrupt mature biofilms at 2xMIC (Fig. 4) compared to the other compounds. Compound 9 is the best biofilm disruptor by completely destroying mature biofilms at 2xMIC. Moreover, in contrast to the commercial compounds, BAC for example at its 2xMIC (200 μg mL−1), a better capacity to disrupt MRSA mature biofilms can be noted at lower concentrations for all the salts.
In terms of toxicity, the compounds show haemolytic activity, except for compound 8. The toxicity of these new compounds should be evaluated in human fibroblasts in the near future before they could find application in the treatment of topical biofilms.
Experimental
Materials and methods
All starting materials and benzalkonium chloride (BAC) were purchased from Sigma-Aldrich. 1H and 13C NMR spectra were recorded in deuterated solvents at 400 and 100 MHz, respectively, using Bruker spectrometers. The purity of final compounds used in biological assays was determined by ESI/LC-MS analysis (≥95%).
Culture conditions and viability tests for bacteria and yeast=
MICs were determined in 96 well cell culture plates. The tests were carried out in a growing Lauria Broth (LB) medium at 37 °C in triplicate. The optical density of bacterial cells (OD 0.1 to 600 nm) was measured using a Fischer Scientific 40 cell density meter model and UV-vis spectroscopy experiments were performed using a Tecan Infinite M200 microplate reader.
Biofilm inhibition assay
S. aureus were incubated in a 37 °C LB medium for 12 h and re-diluted in the LB medium to be incubated again. After 2 h of incubation, the cells were re-diluted at the final concentration (DO 600 nm = 0.1–0.15). The biofilms of S. aureus were marked with a Live/Dead stain after 48 h incubation with antibiotics in a growing medium (LB) in an 8 well glass plate. DMSO (5% volume) was used as the negative control and 20% Triton X was used as the positive control.
Biofilm disruption assay
S. aureus were incubated in a 37 °C LB medium for 12 h and re-diluted in the LB medium to be incubated again. After 2 h of incubation, the cells were re-diluted at the final concentration (OD600 = 0.1–0.15). After an incubation of 48 h in 8 well chambers, the growth media was removed and the biofilms were washed two times with PBS solution (137 mM NaCl, 2.7 mM KCl, 10 mM Na2HPO4, 1.8 mM KH2PO4, in 200 μL distilled water) to remove planktonic cells. The S. aureus biofilms were incubated with antibiotics for 24 h in PBS solution. DMSO (final concentration 5% volume) was used as the negative control and 20% Triton was used as the positive control.
Biofilm staining and confocal laser scanning microscopy (LSM) analysis
Biofilms were stained with the FilmTracer™ LIVE/DEAD® Biofilm viability Kit (Molecular Probes, Life Technologies Ltd). A fluorescent marker solution was prepared by adding 1 μL of SYTO® 9 dye and 1 μL of PI dye in 1 mL of filter-sterilized water. One hundred μL of the staining solution was added to each well of an 8 well glass plate containing the mature biofilm. After 30 min at room temperature in the dark, the samples were washed with a saline phosphate buffer (PBS) solution (137 mM NaCl, 2.7 mM KCl, 10 mM Na2HPO4, 1.8 mM KH2PO4, in 200 μL of distilled water). The biofilms were then observed using a confocal laser microscope (model Leica TCS SP5; Leica Microsystems CMS GmbH, Mannheim, Germany) with a 20× lens (HC PL FLUOTAR 20.0 × 0.70 DRY). A 483 nm laser was used to excite SYTO® 9, while fluorescent emission was detected from 500–540 nm. The PI was excited with a laser at 535 nm and its fluorescent emission was detected from 600–695 nm.
Haemolytic assay
Red blood cells (RBCs) in Alserver's solution were diluted in PBS buffer and centrifuged for 10 min. at 2000 rpm. The RBCs were washed two more times with PBS buffer until the surface liquid was crystal clear and were resuspended in PBS at 2% v/v. In a 96 well plate, 195 μL of the red blood cell solution and 5 μL of the compound in DMSO were added. The plate was incubated with light agitation (25 rpm) for 1 h at 37 °C. The plate was then centrifuged for 10 min at 2000 rpm and 100 μL of the supernatant was transferred to another plate to analyse the absorbance (λ = 405 nm) using a Tecan Infinite M200 microplate reader.
Conclusions
The new compounds presented here have a better ability to inhibit the proliferation of planktonic bacteria and yeasts than most commercially available antibiotics. In addition, we determined that all compounds are better at disrupting mature biofilms than inhibiting their formation. Nevertheless, our new compounds are stronger antibiofilm agents than compounds such as BAC and ampicillin. Finally, the most unexpected results are those showing that the presence of a rigid or a flexible scaffold between the two benzimidazolium cations does not affect the antibiofilm properties of these compounds. These results, together with those previously reported, show that the benzimidazolium cations used in combination with different alkyl chains or rigid scaffolds lead to compounds that can insert into bacterial membranes and disturb them significantly to alter their integrity.
Conflicts of interest
There are no conflicts of interest to declare.
Acknowledgements
We gratefully acknowledge the Natural Sciences and Engineering Research Council of Canada (NSERC) and the Université de Montréal for financial support. We thank J. N. Pelletier and K. J. Wilkinson from the Département de Chimie Université de Montréal for access to their laboratories and instruments.
Notes and references
- S. B. Levy and B. Marshall, Antibacterial resistance worldwide: causes, challenges and responses, Nat. Med., 2004, 10(12), S122–129 CrossRef CAS.
- S. R. Palumbi, Humans as the World's Greatest Evolutionary Force, Science, 2001, 293(5536), 1786–1790 CrossRef CAS PubMed.
-
(a) C. Xu, L. Kong, H. Gao, X. Cheng and X. Wang, A review of current bacterial resistance to antibiotics in food animals, Front. Microbiol., 2022, 13 DOI:10.3389/fmicb.2022.822689;
(b) E. Y. Furuya and F. D. Lowy, Antimicrobial-resistant bacteria in the community setting, Nat. Rev. Microbiol., 2006, 4(1), 36–45 CrossRef CAS PubMed.
- D. Sharma, L. Misba and A. U. Khan, Antibiotics versus biofilm: an emerging battleground in microbial communities, Antimicrob. Resist. Infect. Control, 2019, 8, 76–86 CrossRef PubMed.
- H. C. Flemming, J. Wingender, U. Szewzyk, P. Steinberg, S. A. Rice and S. Kjelleberg, Biofilms: an emergent form of bacterial life, Nat. Rev. Microbiol., 2016, 14(9), 563–575 CrossRef CAS.
- R. M. Donlan, Biofilm formation: a clinically relevant microbiological process, Clin. Infect. Dis., 2001, 33(8), 1387–1392 CrossRef CAS PubMed.
-
(a) C. R. Elie, G. David and A. R. Schmitzer, Strong antibacterial properties of anion transporters, J. Med. Chem., 2015, 58(5), 2358–2366 CrossRef CAS PubMed;
(b) J. Tessier, M. Lecluse, J. Gravel and A. R. Schmitzer, Antimicrobial and antibiofilm activity of disubstituted bis-benzimidazolium salts, ChemMedChem, 2018, 13(23), 2567–2572 CrossRef CAS PubMed.
- J. Tessier, A. R. Schmitzer and A. R. Schmitzer, Benzimidazolium salts prevent and disrupt methicillin-resistant Staphylococcus aureus biofilms, RSC Adv., 2020, 10(16), 9420–9430 RSC.
- A. Janas, P. Pecyna, M. Gajecka, F. Bartl and P. Przybylski, Synthesis and antibacterial activity of new N-alkylammonium and carbonate-triazole derivatives within Desosamine of 14- and 15-membered lactone macrolides, ChemMedChem, 2020, 15(16), 1529–1551 CrossRef CAS PubMed.
- A. Hebert, M. Parisotto, M. C. Rowell, A. Dore, A. Fernandez Ruiz, G. Lefrancois, P. Kalegari, G. Ferbeyre and A. R. Schmitzer, Phenylethynylbenzyl-modified biguanides inhibit pancreatic cancer tumor growth, Sci. Rep., 2021, 11(1), 1–11 CrossRef.
- C. R. Elie, A. Hebert, M. Charbonneau, A. Haiun and A. R. Schmitzer, Benzimidazolium-based synthetic chloride and calcium transporters in bacterial membranes, Org. Biomol. Chem., 2013, 11(6), 923–928 RSC.
- M. Yasir, D. Dutta and M. D. P. Willcox, Enhancement of antibiofilm activity of ciprofloxacin against Staphylococcus aureus by administration of antimicrobial peptides, Antibiotics, 2021, 10(10), 1–17 CrossRef.
- N. K. Allampally, C. G. Daniliuc, C. A. Strassert and L. De Cola, Tuning the structural and photophysical properties of cationic Pt(II) complexes bearing neutral bis(triazolyl)pyridine ligands, Inorg. Chem., 2015, 54(4), 1588–1596 CrossRef CAS.
- Q. Li, F. Huang, Y. Fan, Y. Wang, J. Li, Y. He and H. Jiang, Ag(I)-directed triple stranded helicates with meta-ethynylpyridine, Eur. J. Inorg. Chem., 2014, 3235–3244 CrossRef CAS.
- A. Zamyatina, Aminosugar-based immunomodulator lipid A: synthetic approaches, Beilstein J. Org. Chem., 2018, 14, 25–53 CrossRef CAS PubMed.
- ThermoFisher, LIVE/DEAD™ BacLight™ Bacterial Viability Kit, for microscopy & quantitative assays.
Footnote |
† Electronic supplementary information (ESI) available: Details of all the synthesized compounds and their characterization and biological results. See DOI: https://doi.org/10.1039/d2nj02026b |
|
This journal is © The Royal Society of Chemistry and the Centre National de la Recherche Scientifique 2023 |
Click here to see how this site uses Cookies. View our privacy policy here.