DOI:
10.1039/D2MD00373B
(Research Article)
RSC Med. Chem., 2023,
14, 166-172
Design, synthesis and antibacterial evaluation of a novel class of tetrahydrobenzothiophene derivatives†
Received
13th October 2022
, Accepted 9th November 2022
First published on 5th December 2022
Abstract
In this study, a new series of tetrahydrobenzothiophene derivatives have been designed. Newly designed molecules have been synthesized through a medicinal chemistry route, and their characterization was done by using NMR and HR-MS techniques. Biological evaluation of the synthesized compounds has been done on Gram-negative and Gram-positive bacteria. The marketed antibiotics such as ciprofloxacin and gentamicin were used as controls. The in vitro evaluation results have shown that most of the targeted compounds exhibit good potency in inhibiting the growth of bacteria, including E. coli (MIC: 0.64–19.92 μM), P. aeruginosa (MIC: 0.72–45.30 μM), Salmonella (MIC: 0.54–90.58 μM) and S. aureus (MIC: 1.11–99.92 μM). In particular, compound 3b showed excellent activity with an MIC value of 1.11 μM against E. coli, 1.00 μM against P. aeruginosa, 0.54 μM against Salmonella, and 1.11 μM against S. aureus. From the results, a promising lead compound was identified for future development.
1. Introduction
For several hundred years, bacterial infection has become the main threat to human survival.1–6Mycobacterium tuberculosis, Staphylococcus, Salmonella, Escherichia coli, and Bacillus anthracis are known to be the deadliest bacteria.7–11 It is predicted that more than 10 million people will succumb to bacterial infection in the following 30 years if we do not take any measures to deal with antimicrobial resistance (AMR).12 Therefore, it is urgent to find some new broad-spectrum antibacterial agents that can effectively overcome drug-resistant bacterial infections. Despite the efforts for new antibacterial agents,13–20 the shortage of novel antibiotics is still a well-known issue, and developing new antibiotics is a long and slow process. According to the World Health Organization, AMR has become one of the most serious global public health threats in this century, and there is an urgent need to develop promising antibiotics.21
The special outer membranes of Gram-negative bacteria consist of a lipopolysaccharide (LPS) layer and membrane phospholipids, making the penetration of the cell membranes very difficult for most antibiotics.22 The entire LPS biogenesis pathway, including biosynthesis and transport, is highly conserved, and the proteins involved are attractive targets for antibiotic discovery.23,24 One of the emerging targets in Gram-negative bacteria is MsbA, an ATP-dependent flippase that translocates LPS across the inner membrane.25,26
Tetrahydrobenzothiophene derivatives are representative MsbA inhibitors, which demonstrated both selectivity and potency.27 In our paper, we are now reporting a study for the design and development of tetrahydrobenzothiophene derivatives against four types of bacteria. This study can provide useful information for the design of novel antibacterial analogues with higher activity of tetrahydrobenzothiophene.
2. Results and discussion
2.1. Chemistry
The general synthetic pathways for all these tetrahydrobenzothiophene derivatives are demonstrated in Scheme 1. The synthesis began with the preparation of ethyl 2-amino-4,5,6,7-tetrahydrobenzo[b]thiophene-3-carboxylate derivatives 1a–1b,28 which were successfully obtained via a reaction between cyclohexanones and ethyl cyanoacetate. Compounds 1a–1b were then N-acylated with benzoyl chloride to give the intermediates 2a–2f, which were hydrolyzed under alkaline conditions to give the target tetrahydrobenzothiophene derivatives 3a–3r. All these tetrahydrobenzothiophene analogues were purified by silica gel flash column chromatography to obtain the pure compounds.
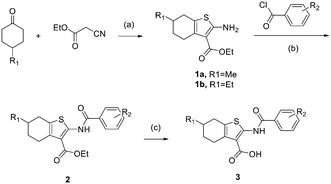 |
| Scheme 1 Synthetic procedures for the target compounds 3a–3r. Reagents and conditions: (a) S8, morpholine, EtOH, 80 °C, 82%; (b) triethylamine, DCM, rt, 62%; (c) NaOH, EtOH, H2O, 80 °C, 95%. | |
The structures of these compounds were characterized by 1H NMR and 13C NMR, as well as electrospray ionisation mass spectrometry (ESI-MS) analysis. The 1H NMR results showed chemical shifts around 13.2 for the hydrogen on the amide, 12.3 for the hydrogen on the carboxyl group, multiple peaks between 7.5–8.0 for the hydrogen on the aromatic ring, multiple peaks between 1.2–3.0 for the hydrogen on the six-membered ring, and around 1.0 for the hydrogen on the methyl group. The 13C NMR results showed resonance peaks for all the carbons consistent with the target molecular structure.
2.2.
In vitro assays of antibacterial activity for tetrahydrobenzothiophene derivatives
The tetrahydrobenzothiophene derivatives 3a–3r were evaluated for bacterial growth inhibition in a standard broth MIC determination, as shown in Table 1. The testing stains were E. coli ATCC 25922, P. aeruginosa ATCC 27853, Salmonella ATCC 12022 and S. aureus ATCC 25922. The marketed antibiotics, such as ciprofloxacin and gentamicin, were used as controls in the present study. In general, most of the new tetrahydrobenzothiophene compounds in Table 1 exhibited moderate to good inhibitory activities. Compounds 3b and 3f showed superior antibacterial activity against E. coli, and the MIC values of six compounds ranged from 0.64 μM to 1.11 μM against E. coli. Compounds 3b, 3c, 3j and 3k showed the highest antibacterial activity compared with other synthesized compounds and ciprofloxacin against P. aeruginosa with MIC values ranging from 0.61 to 1.00 μM. Compounds 3b and 3k displayed strong antibacterial activity against Salmonella with MIC values ranging from 0.54 to 0.73 μM. Compound 3b showed a more potent antibacterial activity than the other synthesized compounds against all the bacteria.
Table 1 Antibacterial activity of the synthesized tetrahydrobenzothiophene derivatives
Compounds |
R
1
|
R
2
|
MIC (μM) |
E. coli
|
P. aeruginosa
|
Salmonella
|
S. aureus
|
3a
|
Me |
H |
1.56 |
1.56 |
1.56 |
99.21 |
3b
|
Me |
2-I |
1.11 |
1.00 |
0.54 |
1.11 |
3c
|
Me |
4-F |
1.47 |
0.72 |
1.47 |
1.47 |
3d
|
Me |
4-OCH3 |
1.42 |
45.30 |
90.58 |
1.42 |
3e
|
Me |
2-F, 6-F |
11.11 |
1.40 |
1.40 |
1.40 |
3f
|
Me |
2-CH3, 3-NO2 |
0.64 |
1.31 |
1.31 |
83.56 |
3g
|
Me |
2-CH3, 4-CH3 |
5.69 |
1.43 |
1.43 |
1.43 |
3h
|
Me |
2-F, 4-F |
22.25 |
11.11 |
11.11 |
1.40 |
3i
|
Me |
2-F, 5-Cl |
1.33 |
1.00 |
1.33 |
2.66 |
3j
|
Et |
2-F, 3-NO2 |
19.92 |
0.61 |
19.92 |
1.25 |
3k
|
Et |
H |
1.49 |
0.73 |
0.73 |
11.85 |
3l
|
Et |
2-Cl |
1.72 |
1.72 |
1.72 |
1.72 |
3m
|
Et |
4-Cl |
3.44 |
3.44 |
1.72 |
3.44 |
3n
|
Et |
4-F |
1.8 |
7.19 |
7.19 |
1.80 |
3o
|
Et |
4-NO2 |
6.68 |
1.67 |
1.67 |
6.68 |
3p
|
Et |
2-F, 4-F |
1.71 |
1.71 |
1.71 |
3.42 |
3q
|
Et |
3-NO2, 5-NO2 |
1.49 |
1.49 |
1.49 |
2.98 |
3r
|
Et |
2-CH3, 4-CH3 |
3.5 |
1.75 |
1.75 |
3.50 |
Lead compound |
H |
4-Cl |
1.46 |
1.46 |
1.46 |
1.46 |
|
|
Ciprofloxacin |
1.27 |
10.1 |
2.54 |
|
|
|
Gentamicin |
|
|
|
1.27 |
SAR studies revealed that compounds with one electron-absorbing group on the benzene ring (compounds 3b, 3c and 3f) exhibited high inhibitory efficacy. If too many electron-absorbing substituents were attached to the benzene ring, the antibacterial potencies of the compounds would be lower (compounds 3h and 3j). And the antibacterial potencies of the compounds were enhanced when R1 was methyl (compounds 3c and 3n). Meanwhile, the antimicrobial activity decreased if the substituent R2 on the benzene ring was an electron donating group (compound 3d).
Compound 3b displayed promising antibacterial activities and therefore was further evaluated for its in vitro time–kill assays. The time–kill curves of 3b with different concentrations against P. aeruginosa and Salmonella show the concentration-dependent bacteriostatic effects, as shown in Fig. 1. Although the 0.5 × MIC of compound 3b showed bacterial propagation, the higher concentrations (1 ×, 2 × and 4 × MIC) showed relatively bacteriostatic kinetics. After treating with derivatives, the living cell counts of bacteria were reduced at 1 ×, 2 ×, and 4 × MIC nearly within 2 h.
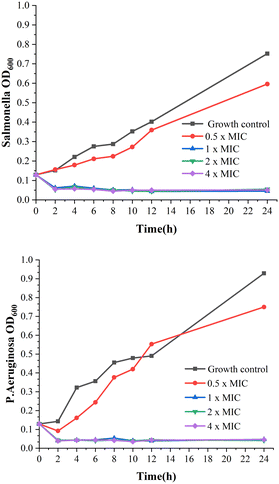 |
| Fig. 1 Time–kill analysis of compound 3b against P. aeruginosa and Salmonella. | |
2.3. Molecular docking study
To test our hypothesis, we performed molecular docking to investigate the potential interactions between 3b and the crystal structure of MsbA from Salmonella typhimurium (PDB ID: 3B60) using a Molecular Operating Environment. The result showed that the thiophene moiety played a crucial role in making the molecule have a more stable conformation, and it closely fitted at the active residue (Tyr-351). Notably, the acetic acid moiety interacted with Ssp-117 through covalent bonding (Fig. 2). The diversity of the binding modes has aroused our interest and encouraged us to do further study on this topic.
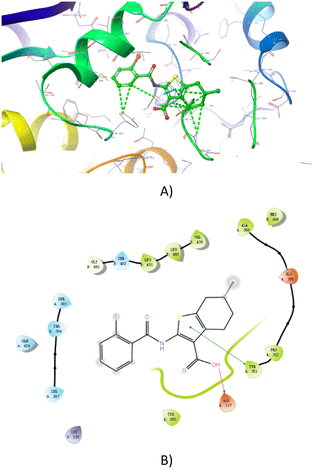 |
| Fig. 2 Best docking results for compound 3b with the crystal structure of MsbA from Salmonella typhimurium (PDB ID: 3B60). A) The 3D docking model and B) the planar docking model. | |
3. Experimental section
All starting materials and reagents were commercially available and were used without further purification. Merck silica gel GF254 plates were used for analytical TLC. 1H and 13C NMR spectra were obtained on a Varian INOVA400 (400 MHz for 1H and 101 MHz for 13C) in CDCl3 or DMSO-d6. Chemical shifts are expressed in δ (ppm) and coupling constants (J) in Hz. The high-resolution mass (HRMS) spectra were recorded on a Bruker maxis impact Q-TOF instrument (Bruker, Billerica, USA) coupled with a Dionex Ultimate 3000 spectrometer (Dionex, Sunnyvale, USA).
General procedure for the synthesis of compounds 1a–1b
A solution of 0.1 mol of cyclohexanone derivatives in 100 ml of ethanol was mixed with ethyl cyanoacetate (0.12 mol), morpholine (0.15 mol) and sulfur (0.12 mol). The mixture was reacted at 80 °C for 1 h. The mixture was washed with brine, followed by drying with Na2SO4 and rotary evaporation to dryness to afford the crude product. The crude product was purified by silica gel column chromatography (hexane/ethyl acetate = 20/1) to give pure compounds 1a–1b.20
General procedure for the synthesis of compounds 2a–2r
To a solution of compounds 1a–1b (1 g, 4.45 mmol) in 10 ml dry DCM, triethylamine (0.68 g, 6.67 mmol) was added and stirred at 0 °C. Then, benzoyl chloride derivative (6.67 mmol) was added slowly, and the reaction was stirred at room temperature for 2 h. After confirming the completion of the reaction by TLC, the mixture was washed with 10% hydrochloric acid, 10% sodium bicarbonate solution, and brine, followed by drying with Na2SO4 and rotary evaporation to dryness to afford compounds 2a–2r, which were used for the next step without purification.
General procedure for the synthesis of compounds 3a–3r
To a solution of compounds 2a–2r (1 g) in ethanol (10 ml), 1 M NaOH (20 ml) was added. The solution was stirred at 80 °C for 2 h. After confirming the completion of the reaction by TLC, the mixture was acidified with 1 M HCl to adjust the pH to 2–3. Ethyl acetate was used to extract the product. The combined ethyl acetate solution was washed with brine, dried over Na2SO4, and filtered. After the solution was removed using a rotavapor, the residue was purified by column chromatography (hexane/ethyl acetate = 10/1) to afford 3a–3r.
2-Benzamido-6-methyl-4,5,6,7-tetrahydrobenzo[b]thiophene-3-carboxylic acid
(3a) yielded 65.1% as a white solid; m.p. 170–172 °C; 1H NMR (400 MHz, DMSO-d6) δ 13.26 (s, 1H), 12.38 (s, 1H), 7.99–7.85 (m, 2H), 7.72–7.62 (m, 2H), 7.59 (d, 1H), 2.91 (m, 1H), 2.64 (m, 2H), 2.20 (m, 1H), 1.88–1.74 (m, 2H), 1.39–1.20 (m, 1H), 1.00 (d, 3H).13C NMR (101 MHz, DMSO-d6) δ 168.00, 162.96, 147.10, 133.09, 132.74, 131.36, 129.61, 128.83, 128.09, 127.42, 126.19, 112.98, 31.36, 31.00, 29.93, 29.22, 26.05. HRMS (ESI) calcd for C17H17NO3S ([M + H]+), 317.0977; found: 317.0980.
2-(2-Iodobenzamido)-6-methyl-4,5,6,7-tetrahydrobenzo[b]thiophene-3-carboxylic acid
(3b) yielded 35.6% as a white solid; m.p. 196–197 °C; 1H NMR (400 MHz, DMSO-d6) δ 13.24 (s, 1H), 11.65 (s, 1H), 8.01 (m, 1H), 7.66–7.57 (m, 1H), 7.55 (m, 1H), 7.38–7.25 (m, 1H), 3.00–2.85 (m, 1H), 2.75 (m, 1H), 2.70–2.54 (m, 1H), 2.25 (m, 1H), 1.83 (m, 2H), 1.43–1.23 (m, 1H), 1.06 (m, 3H). 13C NMR (101 MHz, DMSO-d6) δ 167.62, 165.47, 146.07, 140.58, 140.41, 132.80, 131.46, 129.08, 129.00, 126.58, 113.38, 93.87, 56.51, 32.29, 30.98, 29.24, 26.11. HRMS (ESI) calcd for C17H16INO3S ([M + H]+), 443.0010; found: 442.9989.
2-(4-Fluorobenzamido)-6-methyl-4,5,6,7-tetrahydrobenzo[b]thiophene-3-carboxylic acid
(3c) yielded 42.9% as a white solid; m.p. 189–191 °C; 1H NMR (400 MHz, DMSO-d6) δ 13.16 (s, 1H), 8.03–7.90 (m, 2H), 7.40 (t, 2H), 2.75–2.53 (m, 2H), 2.21 (m, 2H), 1.81 (m, 2H), 1.39–1.18 (m, 1H), 1.01 (d, 3H). 13C NMR (101 MHz, DMSO-d6) δ 168.69, 166.62, 163.30, 161.62, 131.82, 130.28, 130.16, 129.52, 129.48, 125.60, 116.73, 116.43, 32.39, 31.11, 29.37, 26.22, 21.77. HRMS (ESI) calcd for C17H16FNO3S ([M + H]+), 335.1025; found: 335.1048.
2-(4-Methoxybenzamido)-6-methyl-4,5,6,7-tetrahydrobenzo[b]thiophene-3-carboxylic acid
(3d) yielded 40.6% as a white solid; m.p. 171–173 °C; 1H NMR (400 MHz, DMSO-d6) δ 12.30 (s, 1H), 11.95 (s, 1H), 7.83 (d, 2H), 7.12 (d, 2H), 2.90 (m, 2H), 2.66 (m, 2H), 2.17 (s, 1H), 1.79 (m, 2H), 1.30 (q, 3H), 0.99 (m, 3H). 13C NMR (101 MHz, DMSO-d6) δ 166.31, 163.29, 163.23, 162.50, 162.44, 129.42, 129.37, 126.25, 125.83, 114.93, 112.46, 111.70, 56.02, 32.32, 32.28, 30.98, 29.23, 29.12. HRMS (ESI) calcd for C18H19NO4S ([M + H]+), 347.1156; found: 347.1175.
2-(2,6-Difluorobenzamido)-6-methyl-4,5,6,7-tetrahydrobenzo[b]thiophene-3-carboxylic acid
(3e) yielded 57.8% as a white solid; m.p. 175–176 °C; 1H NMR (400 MHz, DMSO-d6) δ 11.97 (s, 1H), 7.68 (m, 1H), 7.28 (m, 2H), 2.98–2.83 (m, 1H), 2.83–2.53 (m, 2H), 2.52–2.41 (m, 1H), 2.30–2.16 (m, 1H), 1.88–1.67 (m, 1H), 1.41–1.26 (m, 1H), 1.00 (dd, J = 13.8, 6.4 Hz, 3H). 13C NMR (101 MHz, DMSO-d6) δ 167.30, 163.48, 161.66, 161.57, 131.56, 131.39, 126.81, 113.03, 112.99, 112.89, 103.00, 32.52, 32.23, 31.13, 30.94, 29.41, 29.20. HRMS (ESI) calcd for C17H15F2NO3S ([M + H]+), 353.1046; found: 353.1083.
6-Methyl-2-(2-methyl-3-nitrobenzamido)-4,5,6,7-tetrahydrobenzo[b]thiophene-3-carboxylic acid
(3f) yielded 42.7% as a yellow solid m.p. 175–176 °C; 1H NMR (400 MHz, DMSO-d6) δ 7.88 (m, 1H), 7.65 (m, 1H), 7.17 (s, 1H), 2.80–2.69 (m, 1H), 2.45 (m, 2H), 2.03 (m, 3H), 1.74 (m, 1H), 1.22 (m, 3H), 0.97 (m, 3H). 13C NMR (101 MHz, DMSO-d6) δ 165.49, 163.45, 153.47, 150.26, 133.23, 131.37, 130.14, 126.92, 126.29, 126.06, 115.42, 102.93, 32.49, 31.11, 29.39, 26.72, 21.74, 14.83. HRMS (ESI) calcd for C18H18N2O5S ([M + H]+), 376.1058; found: 376.1062.
2-(2,4-Dimethylbenzamido)-6-methyl-4,5,6,7-tetrahydrobenzo[b]thiophene-3-carboxylic acid
(3g) yielded 57.6% as a white solid; m.p. 169–171 °C; 1H NMR (400 MHz, DMSO-d6) δ 11.88 (s, 1H), 7.52 (s, 1H), 7.19 (s, 2H), 2.92 (d, 2H), 2.70 (s, 2H), 2.45 (s, 3H), 2.34 (s, 3H), 1.84 (s, 1H), 1.31 (s, 2H), 1.04 (s, 3H). 13C NMR (101 MHz, DMSO-d6) δ 165.95, 165.34, 141.73, 137.61, 132.77, 131.31, 130.79, 127.68, 127.32, 126.38, 112.03, 32.28, 30.99, 29.15, 26.00, 21.49, 21.27, 20.34, 14.50. HRMS (ESI) calcd for C19H21NO3S ([M + H]+), 345.1196; found: 345.1280.
2-(2,4-Difluorobenzamido)-6-methyl-4,5,6,7-tetrahydrobenzo[b]thiophene-3-carboxylic acid
(3h) yielded 61.3% as a white solid; m.p. 193–194 °C; 1H NMR (400 MHz, DMSO-d6) δ 12.67 (s, 1H), 8.14–7.90 (m, 1H), 7.50–7.12 (m, 1H), 7.02–6.86 (m, 1H), 2.96–2.84 (m, 1H), 2.64 (m, 2H), 2.17 (dd, 1H), 1.84–1.72 (m, 1H), 1.47–1.17 (m, 2H), 0.99 (d, 3H). 13C NMR (101 MHz, DMSO-d6) δ 166.75, 164.09, 163.93, 161.07, 159.26, 131.52, 131.38, 126.73, 126.33, 113.02, 112.45, 102.91, 102.55, 32.26, 31.01, 29.17, 26.04. HRMS (ESI) calcd for C17H15F2NO3S ([M + H]+), 353.0684; found: 353.0775.
2-(5-Chloro-2-fluorobenzamido)-6-methyl-4,5,6,7-tetrahydrobenzo[b]thiophene-3-carboxylic acid
(3i) yielded 57.8% as a white solid; m.p. 182–183 °C; 1H NMR (400 MHz, DMSO-d6) δ 7.94 (m, 1H), 7.86–7.66 (m, 1H), 7.56–7.31 (m, 1H), 2.97–2.74 (m, 1H), 2.69 (m, 1H), 2.51–2.40 (m, 1H), 2.20 (m, 1H), 1.77 (m, 1H), 1.27 (m, 2H), 0.99 (m, 3H). 13C NMR (101 MHz, DMSO-d6) δ 163.46, 160.66, 158.004, 157.35, 131.13, 130.97, 129.77, 129.73, 127.36, 126.98, 119.54, 102.97, 32.51, 32.20, 32.12, 30.96, 29.40. HRMS (ESI) calcd for C17H15ClFNO3S ([M + H]+), 370.0388; found: 370.0420.
6-Ethyl-2-(2-fluoro-3-nitrobenzamido)-4,5,6,7-tetrahydrobenzo[b]thiophene-3-carboxylic acid
(3j) yielded 56.7% as a yellow solid; m.p. 178–180 °C; 1H NMR (400 MHz, DMSO-d6) δ 12.57 (s, 1H), δ 8.27–8.09 (m, 2H), 7.99 (m, 1H), 3.00–2.84 (m, 2H), 2.81–2.50 (m, 2H), 1.93–1.81 (m, 1H), 1.56 (m, 2H), 1.32 (m, 2H), 0.92 (m, 3H).13C NMR (101 MHz, DMSO-d6) δ 164.64, 162.84, 162.31, 160.35, 159.76, 137.18, 137.06, 131.77, 131.15, 130.40, 130.27, 127.03, 126.40, 35.95, 35.90, 28.93, 28.69, 15.24. HRMS (ESI) calcd for C18H17FN2O5S ([M + H]+), 394.0828; found: 394.1012.
2-Benzamido-6-ethyl-4,5,6,7-tetrahydrobenzo[b]thiophene-3-carboxylic acid
(3k) yielded 60.0% as a white solid; m.p. 190–192 °C; 1H NMR (400 MHz, DMSO-d6) δ 13.24(s, 1H), 12.37 (s, 1H), 7.95–7.85 (m, 2H), 7.72–7.63 (m, 1H), 7.60 (dd, 2H), 2.92 (m, 1H), 2.71 (dd, 1H), 2.59 (m, 1H), 2.28–2.12 (m, 1H), 1.92–1.79 (m, 1H), 1.63–1.51 (m, 1H), 1.43–1.17 (m, 3H), 0.91 (t, 3H). 13C NMR (101 MHz, DMSO-d6) δ 168.00, 162.94, 147.15, 133.08, 132.74, 131.61, 129.60, 127.41, 126.30, 112.90, 35.99, 30.19, 28.75, 28.53, 26.08, 11.69. HRMS (ESI) calcd for C18H19NO3S ([M + H]+), 331.1180; found: 331.1209.
2-(2-Chlorobenzamido)-6-ethyl-4,5,6,7-tetrahydrobenzo[b]thiophene-3-carboxylic acid
(3l) yielded 58.5% as a white solid; m.p. 189–190 °C; 1H NMR (400 MHz, DMSO-d6) δ 13.24 (s, 1H), 11.93 (s, 1H), 7.79 (m, 1H), 7.68–7.57 (m, 2H), 7.53 (m, 1H), 2.94 (m, 1H), 2.78 (m, 1H), 2.65–2.55 (m, 1H), 2.26 (m, 1H), 1.90 (m, 1H), 1.62 (s, 1H), 1.46–1.20 (m, 3H), 0.94 (m, 3H). 13C NMR (101 MHz, DMSO-d6) δ 167.53, 162.62, 145.89, 133.81, 133.24, 131.73, 130.98, 130.59, 130.55, 128.33, 126.70, 113.43, 35.93, 30.13, 28.75, 28.58, 26.10, 11.81. HRMS (ESI) calcd for C18H18ClNO3S ([M + H]+), 366.1022; found: 366.0784.
2-(4-Chlorobenzamido)-6-ethyl-4,5,6,7-tetrahydrobenzo[b]thiophene-3-carboxylic acid
(3m) yielded 53.4% as a white solid; m.p. 195–196 °C; 1H NMR (400 MHz, DMSO-d6) δ 13.30 (s, 1H), 12.38 (s, 1H), 7.93–7.86 (m, 2H), 7.69 (dd, 2H), 2.94 (m, 1H), 2.75 (m, 1H), 2.51 (m, 1H), 2.23 (m, 1H), 1.89 (d, 1H), 1.60 (s, 1H), 1.44–1.36 (m, 1H), 1.36–1.24 (m, 2H), 0.93 (m, 3H). 13C NMR (101 MHz, DMSO-d6) δ 168.10, 161.83, 146.87, 138.00, 131.60, 131.32, 129.72, 129.30, 126.41, 113.00, 35.93, 30.15, 28.74, 28.65, 26.13, 11.78. HRMS (ESI) calcd for C18H18ClNO3S ([M + H]+), 366.1012; found: 3660668.
6-Ethyl-2-(4-fluorobenzamido)-4,5,6,7-tetrahydrobenzo[b]thiophene-3-carboxylic acid
(3n) yielded 45.6% as a white solid; m.p. 214–215 °C; 1H NMR (400 MHz, DMSO-d6) δ 13.32 (s, 1H), 12.37 (s, 1H), 7.95 (dd, 2H), 7.45 (t, 2H), 2.91 (s, 1H), 2.74 (dd, 1H), 2.59 (m, 1H), 2.23 (dd, 1H), 1.88 (d, 1H), 1.59 (d, 1H), 1.33 (m, 3H), 0.93 (m, 3H). 13C NMR (101 MHz, DMSO-d6) δ 168.13, 166.34, 163.84, 161.87, 131.59, 130.29, 130.20, 129.17, 129.14, 126.28, 116.84, 116.62, 112.93, 35.94, 30.15, 28.76, 28.64, 26.13. HRMS (ESI) calcd for C18H18FNO3S ([M + H]+), 349.1028; found: 349.1032.
6-Ethyl-2-(4-nitrobenzamido)-4,5,6,7-tetrahydrobenzo[b]thiophene-3-carboxylic acid
(3o) yielded 50.2% as a yellow solid; m.p. 206–207 °C; 1H NMR (400 MHz, DMSO-d6) δ 13.41 (s, 1H), 12.50 (s, 1H), 8.44 (d, 2H), 8.14 (d, 2H), 2.79 (dd, 2H), 2.27 (dd, 2H), 1.46–1.15 (m, 4H), 0.92 (m, 3H). 13C NMR (101 MHz, DMSO-d6) δ 163.48, 161.40, 150.17, 146.41, 138.13, 131.84, 129.11, 127.00, 124.80, 113.68, 36.20, 35.94, 30.18, 28.74, 28.60, 26.11. HRMS (ESI) calcd for C18H18N2O5S ([M + H]+), 376.0942; found: 376.1010.
2-(2,4-Difluorobenzamido)-6-ethyl-4,5,6,7-tetrahydrobenzo[b]thiophene-3-carboxylic acid
(3p) yielded 47.6% as a white solid; m.p. 200–201 °C; 1H NMR (400 MHz, DMSO-d6) δ 13.22 (s, 1H), 12.40 (s, 1H), 8.11 (m, 1H), 7.55 (m, 1H), 7.32 (td, 1H), 7.08–6.93 (m, 1H), 2.94 (d, 2H), 2.76 (dd, 2H), 1.89 (d, 1H), 1.60 (s, 1H), 1.46–1.24 (m, 3H), 0.94 (t, J = 7.4 Hz, 3H). 13C NMR (101 MHz, DMSO-d6) δ 161.01, 160.42, 159.73, 159.60, 159.22, 159.18, 158.54, 131.74, 131.60, 126.81, 126.41, 116.88, 116.74, 35.91, 35.88, 30.08, 28.87, 28.76. HRMS (ESI) calcd for C18H17F2NO3S ([M + H]+), 367.0662; found: 367.1031.
2-(3,5-Dinitrobenzamido)-6-ethyl-4,5,6,7-tetrahydrobenzo[b]thiophene-3-carboxylic acid
(3q) yielded 50.8% as a red solid; m.p. 198–199 °C; 1H NMR (400 MHz, DMSO-d6) δ 14.37 (s, 1H), 9.02 (q, 1H), 8.93–8.88 (m, 2H), 2.81–2.71 (m, 1H), 2.56–2.39 (m, 1H), 2.10–1.99 (m, 1H), 1.82 (d, 1H), 1.33 (m, 2H), 1.24 (m, 3H), 0.95–0.87 (m, 3H). 13C NMR (101 MHz, DMSO-d6) δ 165.44, 164.38, 163.45, 148.69, 134.44, 131.63, 129.30, 122.46, 115.53, 102.85, 36.19, 30.36, 28.94, 28.73, 26.77, 14.79, 11.81. HRMS (ESI) calcd for C18H17N3O7S ([M + H]+), 421.1041; found: 421.1012.
2-(2,4-Dimethylbenzamido)-6-ethyl-4,5,6,7-tetrahydrobenzo[b]thiophene-3-carboxylic acid
(3r) yielded 46.3% as a white solid; m.p. 219–220 °C; 1H NMR (400 MHz, DMSO-d6) δ 13.21 (s, 1H), 11.82 (s, 1H), 7.52 (d, 1H), 7.19 (d, 2H), 2.94 (d, 1H), 2.77 (dd, 1H), 2.65–2.55 (m, 1H), 2.45 (s, 3H), 2.34 (s, 3H), 2.25 (dd, 1H), 1.90 (d, 1H), 1.61 (s, 1H), 1.35 (m, 3H), 0.94 (t, 3H). 13C NMR (101 MHz, DMSO-d6) δ 167.89, 165.25, 147.00, 141.67, 137.47, 132.75, 131.46, 131.10, 127.75, 127.36, 125.97, 112.52, 35.97, 30.14, 28.70, 26.13, 21.30, 20.43, 11.81. HRMS (ESI) calcd for C20H23NO3S ([M + H]+), 359.1620; found: 359.1126.
Minimum inhibitory concentration testing
The MIC of the target compounds was conducted on the following four bacteria stains: Staphylococcus aureus (ATCC 25923), Escherichia coli (ATCC 25922), Pseudomonas aeruginosa (ATCC 27853), and Salmonella (ATCC 12022) by microdilution in a 96-well cell culture plate. The 5 μl of suspension was transferred to 5 ml of fresh medium and incubated for a few hours to reach the log phase stage. After that, the bacteria were diluted to reach 2 × 106 colony-forming units per milliliter (CFU ml−1). Each well contained 100 μl of the bacterial cell suspension and various concentrations of the target compounds in 100 μl for a final volume of 200 μl wells. The experiment was repeated three times.
Constant concentration time–kill curves
P. aeruginosa and Salmonella were cultured in an LB liquid medium broth at 37 °C using a shaking incubator at 180 rpm with shaking and then diluted to approximately 6 × 105 CFU ml−1. Test compound 3b with final concentrations of 0.5 × MIC, 1 × MIC, 2 × MIC, and 4 × MIC were inoculated with aliquots of bacteria resuspended in fresh media. Samples (0.1 ml) were taken from each sample 2, 4, 6, 8, 10, 12 and 24 h following inoculation, and the optical density was determined using an enzyme plate analyzer. The experimental results were expressed as OD600, and the experiment was repeated in triplicate.
Molecular docking
Schrodinger was used for the docking study. The crystal structure of MsbA from Salmonella typhimurium (PDB ID: 3B60) was used for molecular docking. The binding affinity between the pleuromutilin analogues and receptor were estimated using Schrodinger.
Conclusions
In summary, a new series of tetrahydrobenzothiophene derivatives have been designed in pursuit of novel antibacterial agents. Newly designed molecules have been synthesized via a medicinal chemistry route, and their characterization was done by using IR, NMR, & HR-MS techniques. Biological evaluation of the synthesized compounds has been done on Gram-negative and Gram-positive. The in vitro evaluation results have shown that most of the targeted compounds exhibited good potency in inhibiting the growth of bacteria. In particular, compound 3b showed excellent activity. From the results, a promising lead compound was identified for future modification and transformation.
Author contributions
Conceptualization, L. L.; methodology, W. S.; software, J. Y.; data curation, L. L.; writing—original draft preparation, S. P.; writing—review and editing, X. C.; visualization, J. C.; supervision, J. C.; project administration, X. S.; funding acquisition, S. P. All authors have read and agreed to the published version of the manuscript.
Conflicts of interest
There are no conflicts to declare.
Acknowledgements
This research was funded by the Scientific Technological Research Program of Chongqing Municipal Education Commission (No. KJQN201901501), the Open Fund of Chongqing Key Laboratory of Industrial Fermentation Microorganism, Chongqing University of Science and Technology (No. GYFJWSW-06), the Postgraduate Innovation Program of Chongqing University of Science and Technology (No. YKJCX2120504) and the Innovation and College Student Innovation Training Program of Chongqing University of Science and Technology (No. s202211551023).
Notes and references
- R. Pitt and H. Fifer, Medicine, 2022, 50, 277–279 CrossRef.
- S. George, F. F. Muhaj and C. D. Nguyen,
et al.
, J. Am. Acad. Dermatol., 2022, 86, 1189–1204 CrossRef CAS.
- A. W. Fujita, K. Werner and J. T. Jacob,
et al.
, Int. J. Infect. Dis., 2022, 119, 120–129 CrossRef.
- S. Falkow, Cell, 2006, 124, 699–702 CrossRef CAS.
- N. Kumar and S. Ghogale, Ann. Med. Surg., 2022, 78, 103949 CrossRef.
- E. de Souza Cândido, E. Barros, M. H. Cardoso and O. L. Franco, Curr. Opin. Pharmacol., 2019, 48, 76–81 CrossRef.
- M. Hamad, F. Al-Marzooq, G. Orive and T. H. Al-Tel, Drug Discovery Today, 2019, 24, 2225–2228 CrossRef PubMed.
- S. Rao, L. Linke, R. Magnuson, L. Jauch and D. R. Hyatt, One Health, 2022, 15, 100407 CrossRef CAS PubMed.
- A. Chahouri, N. Radouane, B. Yacoubia, A. Moukrim and A. Banaouia, Mar. Pollut. Bull., 2022, 180, 113824 CrossRef CAS.
- M. Giufrè, E. Mazzolini, M. Cerquetti and S. Brusaferro, Int. J. Antimicrob. Agents, 2021, 58, 106433 CrossRef.
- C. Park, J. Lee, D. Lee and J. Jang, Sens. Actuators, B, 2022, 335, 131321 CrossRef.
-
Review on Antimicrobial Resistance, Antimicrobial Resistance: Tackling a Crisis for the Health and Wealth of Nations, 2014 Search PubMed.
- G. A. Salman, D. S. Zinad and A. Mahal,
et al.
, ChemistrySelect, 2022, 7(4), e202103901 Search PubMed.
- D. S. Zinad, A. Mahal and G. A. Salman, Org. Prep. Proced. Int., 2021, 53(6), 578–584 CrossRef CAS.
- G. A. Salman, D. S. Zinad and A. Mahal, Monatsh. Chem., 2020, 151, 1621–1628 CrossRef CAS.
- A. Mahal, S. D'Errico and N. Borbone,
et al.
, Beilstein J. Org. Chem., 2015, 11, 2689–2695 CrossRef CAS.
- L. Yang, A. Mahal and Y. Liu,
et al.
, Phytochem. Lett., 2017, 20, 89–92 CrossRef CAS.
- N. M. El-Barasi, M. M. Miloud and M. M. El-ajaily,
et al.
, J. Saudi Chem. Soc., 2020, 24(6), 492–503 CrossRef CAS.
- M. Duan, A. Mahal and B. Mohammed,
et al.
, Nat. Prod. Res., 2022, 36(20), 5268–5276 CrossRef CAS PubMed.
- D. S. Zinad, A. Mahal and R. K. Mohapatra,
et al.
, Chem. Biol. Drug Des., 2019, 95(1), 16–47 CrossRef.
-
WHO, Antibacterial Resistance Global Report on surveillance, Geneva, 2014 Search PubMed.
- M. F. Richter, B. S. Drown, A. P. Riley, A. Garcia, T. Shirai, R. L. Svec and P. J. Hergenrother, Nature, 2017, 545, 299–304 CrossRef CAS.
- R. L. Guest, S. T. Rutherford and T. J. Silhavy, Trends Microbiol., 2021, 29, 334–345 CrossRef CAS PubMed.
- N. Paracini, E. Schneck, A. Imberty and S. Micciulla, Adv. Colloid Interface Sci., 2022, 301, 102603 CrossRef CAS PubMed.
- T. Wu, A. C. McCandlish, L. S. Gronenberg, S. S. Chng, T. J. Silhavy and D. Kahne, Proc. Natl. Acad. Sci. U. S. A., 2006, 103, 11754–11759 CrossRef CAS.
- M. Vaara, Antimicrob. Agents Chemother., 1993, 37, 2255–2260 CrossRef CAS PubMed.
- G. Zhang, V. Baidin, K. S. Pahil and E. Moison,
et al.
, Proc. Natl. Acad. Sci. U. S. A., 2018, 115, 6834–6839 CrossRef CAS.
- M. M. Mojtahedi, Synth. Commun., 2010, 40, 2067–2074 CrossRef CAS.
|
This journal is © The Royal Society of Chemistry 2023 |