DOI:
10.1039/D2FO03006C
(Paper)
Food Funct., 2023,
14, 457-470
Eucommia ulmoides male flower as a remarkable edible floral resource exerts lifespan/healthspan-promoting effects on Caenorhabditis elegans†
Received
7th October 2022
, Accepted 6th December 2022
First published on 7th December 2022
Abstract
Natural products, especially phytochemicals, can effectively improve the health of various model organisms and ultimately prolong their lifespan. As an emerging resource of plant-based food, edible flowers have potential anti-aging effects. Here, we showed that twelve out of 30 drug-food homologous flowers’ extracts significantly extended the lifespan of C. elegans, and the Eucommia ulmoides male flower was screened out by comparing centrally. The lifespan of C. elegans increased by 18.61% under the treatment of 100 μg mL−1 floral extract (EUFE). Interestingly, this effect was attenuated when EUFE was administered late or at higher concentrations. Significantly, EUFE improved health indicators that decline with aging including pharyngeal pumping, mobility, muscle morphology, and lipofuscin accumulation. EUFE also enhanced the resistance of C. elegans to oxidative/heat stress. The longevity-extending effect of EUFE was dependent on transcription factor DAF-16 and mitochondrial function. Moreover, EUFE triggered the nuclear translocation of DAF-16 and promoted downstream LGG-1 and SOD3 protein expression. In body-wall muscles, EUFE stimulated mitochondrial fission and mitophagy to mitigate age-related mitochondrial impairments. The transcriptional checkpoints of daf-16, drp-1, eat-3, lgg-1, and dct-1 further showed that EUFE regulated DAF-16 signaling and mitochondrial homeostasis. Finally, the interpretation of the EUFE components by correlation analysis, UHPLC-QE-MS, and verification experiments showed that aucubin, geniposide, and asperuloside are the main active compounds. We revealed the excellent lifespan/healthspan-promoting efficacy of EUFE and highlighted that edible flowers are worthy of further investigation as anti-aging dietary resources. Meanwhile, related mechanisms enriched the hypothesis that mitochondria might be involved in the healthspan modulation of longevity pathways.
Introduction
Aging is a primary risk factor for chronic diseases and it dramatically increases intractable public health and socioeconomic problems. Encouragingly, theories of aging have gradually evolved from a single ‘free radical theory’ to aging being a systemic decline with diverse hallmarks.1 Besides, increasing numbers of genetic players that may control these hallmarks have been assessed, such as the forkhead box family of transcription factors. Interestingly, regardless of how theories have changed, the impact of mitochondria in the aging process is highly prominent. The maintenance of healthy mitochondria is crucial for long-lasting health,2 which requires a normal network of mitochondrial quality control (MQC). Modulation of MQC to counteract aging is currently being actively investigated.3,4
Natural products like extracts or monomeric compounds with anti-aging activities attract great attention because they may be safer for consumption and more quickly approved for clinical use. Foods, especially vegetables and fruits, are the best choice for preparing or isolating novel natural products to slow aging, such as grape extracts, or sulforaphane from cruciferous vegetables.5 In addition, more dietary resources with anti-aging activities including wolfberry, tree nuts, royal jelly, etc. have also been discovered.6,7 As harmless and healthful parts of plants, edible flowers are habitually consumed as food components or herbal medicines. And they have emerged as a new trend for human nutrition owing to their unique flavor, color, rich nutrients and phytochemicals. As per our previous review, edible flowers can prevent or ameliorate skin aging, immunosenescence, neurodegeneration, and even extend lifespan.8 However, enough attention has not been given to them compared to other anti-aging foods. The reasons for the disparity include, first, systematic in vivo studies on anti-aging activities are still lacking despite the rigorous in vitro investigations on the antioxidant properties of various floral species.9 Second, limited in vivo experimental data, particularly inquiry of mechanisms, are available on flowers with activities against aging.
At present, the model organism C. elegans is ideally suited for in vivo anti-aging assays because of its short life cycle, evolutionarily conserved aging pathways, and genetically tractable traits.10 Therefore, we used this model to centrally compare the lifespan-prolonging effects of 30 common edible flowers. Moreover, we delved into the lifespan/healthspan-promoting effects and mechanisms of E. ulmoides male flower extract (EUFE) and its main active compounds. We highlighted the potential of edible flowers as a valuable dietary resource for healthy aging. And we reported the in vivo lifespan/healthspan-promoting activity of E. ulmoides male flower for the first time and supported the importance of mitochondria in the longevity pathways.2
Materials and methods
Chemical reagents and sample preparation
Paraquat dichloride hydrate (98%), 5-fluoro-2′-deoxyuridine (FUDR), and carbonyl cyanide m-chlorophenylhydrazone (CCCP) were purchased from Macklin Inc. (Shanghai, China). Standards (≥98%), agarose, levamisole hydrochloride, high-performance liquid chromatography (HPLC)-grade methanol, and acetonitrile were purchased from Solarbio Inc. (Beijing, China). Other conventional chemicals of analytical reagent grade or above were from National Pharmaceutical Group Corporation (Shanghai, China). All thirty edible flowers used in this study were authenticated by the suppliers and the flower morphology matched the criteria as provided in eTable 1.† The specific preparation process of floral ethanolic extracts and EUFE was according to eMethod 1.†C. elegans strains used are given in eTable 2.† The strains were cultured at 20 °C on the standard nematode growth medium (NGM) seeded with Escherichia coli OP50, unless specified.
Lifespan and in vitro antioxidant assays
A lifespan assay on solid media was conducted as previously described.11 Synchronized L4 stage worms were picked and placed on new plates containing vehicle or sample solution. The day was recorded as day 0 of adulthood. Without using FUDR, worms were counted every day and considered dead if they failed to respond to stimuli from the picker. Worms that missed and internally hatched were excluded. General procedures for synchronization and administration were as indicated in eMethod 2.†In vitro antioxidant assays were performed according to the procedures described in our previous study.9,12 And we used the antioxidant potency composite index (APCI) for a more consistent comparison,13 see eMethod 3† for details of the related calculation.
Reproduction and diet preference assays
Synchronized L1-larval stage worms were individually picked and placed on the new plates containing vehicle or 100 μg mL−1 EUFE daily until the end of the spawning period. The used plates remained for 36 h to allow eggs to hatch and develop and then the offspring were counted. For the specific procedure of the diet preference assay, see eMethod 4 and eFig. 1A.†
Healthspan assays
Body size.
On the 1st, 5th, and 9th day of adulthood, the worms of the vehicle or sample group were picked and placed on blank NGM plates. Photographs of worms were taken using an optical microscope (Nikon Eclipse Ci, Japan) with a Canon EOS 850D camera (Canon Inc., Japan), and were analyzed using ImageJ software (National Institutes of Health, USA) by selecting a region of interest.
Pharyngeal pumping.
We picked worms and placed them on fresh plates at the same time points. Half an hour later, the tracking videos exceeding 1 min were shot using the above camera to record the pharyngeal pumping of worms. To measure related rates, the videos were played in slow forward mode at a quartile of one speed.
Locomotor activity.
The feeding process and time points were the same as above. Before transfer, the motor status of worms was artificially recorded as follows: autonomously move (A), only move after they were touched (C), and the status between A and C (B). Then the worms belonging to A were picked and placed on the blank plates and videos were taken at a fixed position. And the moving average speed was analyzed using WormLab (MBF Bioscience, VT).
Stress resistance assays.
Worms were pre-treated with vehicle or 100 μg mL−1 EUFE for 1, 5, and 9 d at 20 °C on NGM plates. For oxidative stress,14 worms were collected in centrifuge tubes (2.5 mL) with 0.1 mL of M9 buffer with 200 mM paraquat. Tubes were placed at 20 °C with agitation for 2.5 h. Worms were washed with M9 buffer three times to remove the oxidant and then transferred to fresh NGM plates seeded with OP50. After 5 h, dead worms were counted and the death rate was calculated. For heat stress,15 worms were picked and placed on fresh plates and incubated at 34 °C for 8 h and the death rate was measured after a ‘recovery’ period of 16 h at 20 °C.
Fluorescence microscopy imaging and transmission electron microscopy (TEM)
The RW1596 and SJ4103 strains were used separately for muscle and mitochondrial morphology observation under a green fluorescent protein (GFP) channel.16 The intestinal lipofuscin accumulation level in wild-type nematodes was determined by the study of Vayndorf et al.17 Moreover, we used the TJ356, DA2123, and CF1553 strains to observe the intracellular localization of DAF-16 and compare the expression of LGG-1 and SOD3, respectively.18–20 For mitophagy detection, the IR1511 strain was used, which expressed GFP-tagged DCT-1 and red fluorescent protein (DsRed)-tagged LGG-1 in body-wall muscles. The colocalization of two signals indicates the fusion of mitochondria with the lysosome, that is, the occurrence of mitophagy.21 For more details, including slide preparation, acquisition conditions, EUFE treatments, and so on, see eMethod 5.† Samples for TEM were prepared following eMethod 6† with some modifications.22
Quantitative polymerase chain reaction (qPCR) analysis
∼1000 worms (treated for 1 day) were collected. Differently, the NGM plates with 200 μM FUDR were used to inhibit the reproduction of progeny. Total RNAs were isolated using the TRIzol reagent (Servicebio, China) and cDNAs were synthesized using the Servicebio® RT First Strand cDNA Synthesis Kit (Servicebio, China). The qPCR was performed in an ABI StepOne Plus cycler using StepOne software with the SYBR Green PCR Master Mix (High ROX). The relative expression levels of genes were calculated using the 2−ΔΔCT method and act-1 was set as a reference gene. Related primer sequences are indicated in eTable 3.†
Qualitative and quantitative analyses of EUFE
For qualitative analysis, the extract was analyzed using a Q Exactive orbitrap high-resolution mass spectrometer connected to the UltiMate™ 3000 ultra-high performance liquid chromatography system (Thermo Fisher Scientific, USA) (UHPLC-QE-MS).23 For quantitative analysis, the extract solution was analyzed using the E2695 HPLC system equipped with the 2998 PDA detector (Waters, USA) (for detailed procedures, see eMethod 7†). Determination of total phenolic, total flavonoid, and total terpenoid content was performed by following Xiong et al. and Truong et al.9,24
Statistical analysis
All the lifespan experiments were repeated 2 or more times and at least 60 individuals were scored per treatment per experiment. The Kaplan Meier method was used for survival analyses and significance was calculated using the log-rank (Mantel–Cox) test. The rest of the experiments were repeated 3 times except for TEM. Each group included at least 30 worms for each experiment, unless specified. Differences between two groups were assessed using the unpaired t-test. More than two groups were assessed using the multiple t-test and statistical significance was determined using the Holm–Sidak method, with the significant levels of alpha = 0.05. The GraphPad Prism (San Diego, USA) and Excel (Microsoft, USA) software were used to analyze and plot the experimental data.
Results and discussion
Multiple floral ethanolic extracts extend lifespan in C. elegans
As shown in Fig. 1, eFig. 2 and eTable 4,† ethanol extracts of 12 edible flowers at 50 mg mL−1 significantly prolonged both the mean and median lifespan of nematodes (P < 0.05). Survival curves with different shapes may have a similar mean lifespan,25 the same was true for the comparison of the N. nucifera and E. japonica flower in our data. In addition, the nematodes treated with cherry blossom extract (no. 16) did not differ from the vehicle group in their median lifespan despite having a higher mean lifespan. The combination of survival curves, mean and median lifespan described more accurately the pro-longevity properties of floral extracts. Other studies have reported the same activity in the flowers of H. sabdariffa,26L. japonica,27 and T. erecta,28 which reflects the accuracy of the screening results to some extent. Differing from Zhang et al., the extract of R. rose (no. 13) increased the mean and median lifespan but not significantly (P = 0.1038), which may be related to the different extraction methods.29
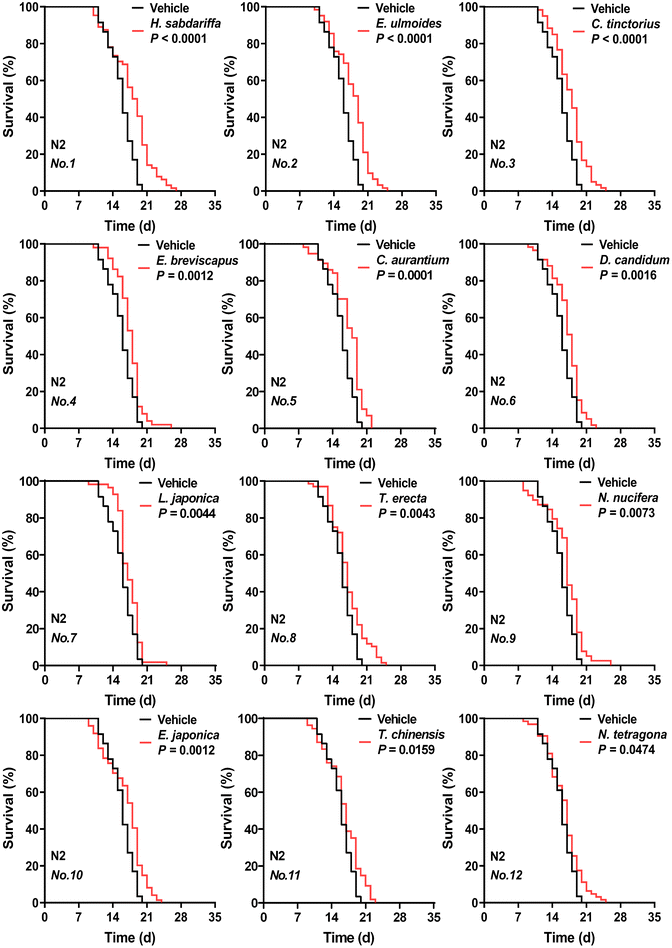 |
| Fig. 1 Effects of ethanolic extracts of 12 edible flowers (at 50 mg mL−1 concentration) on C. elegans lifespan. The respective survival curves of nematodes treated with 12 flowers (significant longevity-promoting effect) or with vehicle (based on sham ethanol extraction). N2 means C. elegans Bristol N2 strain wild-type nematodes. Flowers are abbreviated and numbered according to eTable 1.†P values represent the comparison with the vehicle calculated using the log-rank (Mantel–Cox) test. See eTable 4† for more detailed information. | |
Based on this, the top two flower species with better effects included H. sabdariffa and E. ulmoides. These two floral extracts respectively extended the mean lifespan of nematodes by 12.66% and 12.59% compared to the vehicle group.
Lifespan-extending activity of floral extracts hardly correlated with the antioxidant activity in vitro
Given the possible causal relationship between the lifespan-prolonging and antioxidant activities,30 we investigated the in vitro antioxidant properties of 30 edible floral extracts. As shown in eTable 5,† the extracts of P. suffruticosa, N. tetragona, and T. erecta flowers were ranked in the top three. Unexpectedly, the P. suffruticosa flower extract with the highest APCI value had no lifespan-extension effects. Furthermore, we analyzed the correlation between the edible flowers’ APCI values and the effects on the nematodes’ mean or median lifespan. The absolute value of correlation coefficients (|r|) was less than 0.5 (eTable 6†), suggesting that some of the flowers with low antioxidant activity but high lifespan-extending activity may have mechanisms other than antioxidant activity. Dietary resource or ingredient screening from the perspective of antioxidant activity followed by activity studies is one of the strategies to seek anti-aging agents.31 However, our data supported that this strategy may have some limitations. We pointed out that only relying on antioxidant activity to screen for anti-aging agents may result in omission.
Considering the strength of the lifespan-prolonging effects and novelty of the research, we finally chose E. ulmoides male flower as subsequent studies’ object. As a member of the Eucommiaceae family, E. ulmoides Oliver was first recorded in the Shennong Ben Cao Jing, and its bark, leaves, seeds, and male flowers are commonly used as medicine. Traditionally, the comprehensive utilization of E. ulmoides remains low, and other parts except bark are not fully utilized. As a new food resource approved by China's Ministry of Health, the male flower of E. ulmoides exhibits physiological activities such as hypolipidemic, anti-obesity, antioxidant, and anti-fatigue.32 Interestingly, although extracts from E. ulmoides bark had shown to extend nematode longevity and improve its stress resistance,33 it is still unknown whether the male flower parts exhibit these activities.
Lifespan-extending activity of EUFE was influenced by the concentration and period of administration
To gain further insight into the lifespan-extending activity, we first examined the effects of different concentrations of EUFE. Since the survival rate of nematodes treated with 1 mg mL−1 EUFE for 7 days was less than 90% (eFig. 3†), the concentrations of EUFE were fixed at 0, 25, 50, 100, 200, and 500 μg mL−1. We observed that nematodes treated with 100 μg mL−1 EUFE had the longest lifespans, with a mean lifespan extension of 18.61% compared to the vehicle group (Fig. 2A and eTable 7†). It should be noted that the effect of EUFE treatment at 500 μg mL−1 on nematode longevity was not significant as found by the log-rank test (P > 0.05). Therefore, 100 μg mL−1 EUFE was used for subsequent experiments.
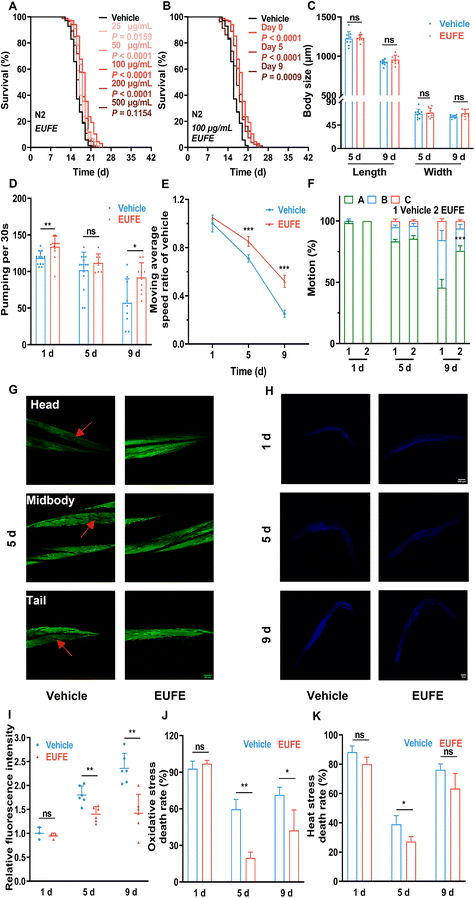 |
| Fig. 2 EUFE extended lifespan and improved fitness in C. elegans. (A) The survival curves of nematodes treated with EUFE at various concentrations (25, 50, 100, 200, and 500 μg mL−1) or with the vehicle (sterile water). (B) The survival curves of nematodes treated with EUFE at different life stages (0, 5, 9 days). For A and B, N2 means C. elegans Bristol N2 strain wild-type nematodes. P values represent the comparison with the vehicle calculated using the log-rank (Mantel–Cox) test and the ESI is summarized in eTable 7.† Each experiment was repeated 3 times. (C) Effects of EUFE on the body length and width of animals on days 5 and 9. Pharyngeal pumping (D) and motility (reflected in the movement rate (E) and the motor status (F)) at days 1, 5 and 9 of adulthood in nematodes treated with 100 μg mL−1 EUFE or vehicle. (G) Representative images of muscle morphology at day 5 of adulthood of pmyo-3MYO-3::GFP nematodes treated with EUFE or vehicle. Scale bar, 20 μm. The captured muscles were located at the head, mid-body, and tail of nematodes. (H) Representative images of lipofuscin accumulation in wild-type nematodes treated with EUFE or vehicle for 1, 5 and 9 days. Scale bar, 100 μm. (I) Quantification of relative lipofuscin fluorescence density. The survival rate of nematodes fed with EUFE (100 μg mL−1) or vehicle under oxidative (J) and heat (K) stress conditions. For C and I, related data were quantified using ImageJ software. For C, D, E, F, I, J, and K, values are mean ± SD. ns, not significant; *P < 0.05, **P < 0.01, ***P < 0.001 by the multiple t-test. Data are representative of three independent experiments. | |
The effectiveness of some nematode lifespan-prolonging active ingredients may be affected by the period of supplementation.34 For this, we treated nematodes with 100 μg mL−1 EUFE at different life stages (0, 5, and 9 days) until their death. It was shown that the mean lifespan of nematodes treated with EUFE at any of the above stages was significantly prolonged versus the vehicle group. And the intervention from day 0 had the best lifespan-extension effect (Fig. 2B and eTable 7†).
Studies have indicated that nematode lifespan can be extended by intervening in development, fertility, and dietary intake.16 Since EUFE had no direct role in the metabolism or growth of E. coli OP50 according to the experimental scheme, we only considered whether nematodes were starved due to the avoidance of the bacteria treated with EUFE. The results showed that EUFE treatment did not affect nematode development (no changes in body shape), reproductive capacity, and diet preference compared with the vehicle group, as shown in eFig. 4, 5, and 1B.†
EUFE improved fitness, especially motility, in C. elegans
Next, we assessed whether the increased lifespan of nematodes treated with EUFE was accompanied by the improvement in fitness through a series of age-related representations. The adult nematodes exhibited a nearly linear growth phase at the beginning, which was characterized by an increase in body size until they entered a growth plateau. In addition, there was a fluctuating decrease in nematode size with age.35 Combining eFig. 4† and Fig. 2C, our data also reflected the same trend. In addition, the mean body length and width of nematodes after 9 days of EUFE treatment were higher than those in the vehicle group, although not significantly.
Nematodes take in bacterial food through the contraction and relaxation of pharyngeal muscles. And the age-related decline in this capacity was positively correlated with lifespan.36 EUFE treatment for 1 day resulted in an increased rate of pharyngeal pumping in nematodes compared to the vehicle group (P < 0.01) (Fig. 2D). More importantly, on day 9, the nematode pumping rate decreased in the vehicle group, while EUFE treatment significantly maintained the pharyngeal muscle function.
In addition to pharyngeal muscles, nematode whole body-wall muscle cells also gradually lose vitality during aging, leading to a decrease in motility.16 As indicated in Fig. 2E and F, more nematode populations (A status) were able to move autonomously after 9 days of EUFE treatment compared to the vehicle group, and they had significantly faster average moving rates. Interestingly, when nematodes were treated for 5 days, although there was no clear difference in the motor status between the vehicle and EUFE groups, significant variability in the moving rate had developed in the nematode population with status A among them.
To observe the changes more visually in nematode muscles, we used the pmyo-3MYO-3::GFP strain (RW1596). The muscle fiber organization of the head, middle, or tail body wall of the nematode was complete and arranged in order at day 1 in both groups (eFig. 6†). However, by day 5, as marked by the red arrows, the nematodes in the vehicle group showed obvious breakage, absence, or disordered arrangement, both at the head, middle, and tail (Fig. 2G and eFig. 7†). In contrast, the muscles of EUFE-treated nematodes remained in a relatively good condition.
Lipofuscin, as an auto-fluorescent ‘age pigment’, is one of the widely studied age-dependent biomarkers in C. elegans.37 EUFE treatment for 5 or 9 days significantly reduced lipofuscin accumulation in nematodes versus the control (P < 0.01) (Fig. 2H and I).
The resistance of nematodes to external stimuli decreases dramatically with aging. As also indicated by our data (Fig. 2J and K), nematodes on day 9 had a higher lethality rate than on day 5 under either oxidative or heat stress. Interestingly, the nematode lethality was higher on day 1 of treatment for both vehicle and EUFE, which may be related to the fact that the stress resistance systems were not fully established yet. EUFE treatment for both 5 and 9 days was effective at decreasing mortality under paraquat stimulation. In particular, the lethality rate was lower than 30% in the EUFE-treated group after 5 days of treatment. The enhancement of nematode heat resistance by EUFE did not seem to be as strong and even the mortality in the group treated with EUFE for 9 days was not significantly different from that in the vehicle group.
EUFE required DAF-16 and mitochondrial function to promote lifespan
In fact, with the development of aging theory, more and more longevity pathways including the insulin/insulin-like growth factor (IIS) pathway, caloric restriction, etc. have been identified.2 DAF-16 (mammalian Forkhead box protein O1 (FoxO1) orthologue) serves as the key transcription factor that integrates different signals from multiple longevity pathways, including the IIS pathway, germline signaling pathway, etc. to regulate aging, longevity, and resistance via shuttling between the nucleus and the cytoplasm.38 Interestingly, the improvement of lifespan by EUFE was entirely inhibited in daf-16 mutants (Fig. 3A). This suggested that the effect of EUFE was dependent on DAF-16. Similarly, the lifespan-extending effect of EUFE required mitochondrial function, according to the related experiments in mev-1 mutants (Fig. 3B). The gene mev-1 encodes a subunit of succinate-Q oxidoreductase in the mitochondrial electron transport chain (ETC) and the mutants exhibit phenotypes such as shortened lifespan.39 In contrast, EUFE significantly prolonged the lifespan of eat-2 and skn-1 mutants, indicating that the lifespan-extending activity of EUFE did not involve the diet-restricted pathway and was not dependent on the SKN-1/Nrf2 transcription factor (Fig. 3C and D). Furthermore, we analyzed whether the IIS pathway was involved in the longevity-promoting effect of EUFE. The propensity to form dauers, adult lifespan, and stress resistance were increased when this pathway was disrupted by mutations like the insulin-like receptor or the class I phosphatidylinositol 3-kinase reduction-of-function mutant (daf-2 and age-1 strains).40 It should be mentioned that the age-1(hx546) mutation appeared to affect the nematodes only during starvation and in aging adults, with no effect on the localization of DAF-16 under normal culture conditions.41 EUFE extended longevity of the age-1 mutant suggested that normal DAF-16 was more critical for the activity, not the overall IIS pathway (Fig. 3E). In terms of mitochondria, there are some nematode strains that disrupt the function of ETC but are long-lived, such as the clk-1 mutant.42 However, the mitochondrial function of clk-1 mutants fed on the standard E. coli OP50 was only slightly reduced compared to the wild types.43 Accordingly, we still observed that EUFE extended the longevity of clk-1 mutants (Fig. 3F). This result similarly highlighted the requirement for the mitochondrial function for EUFE activity.
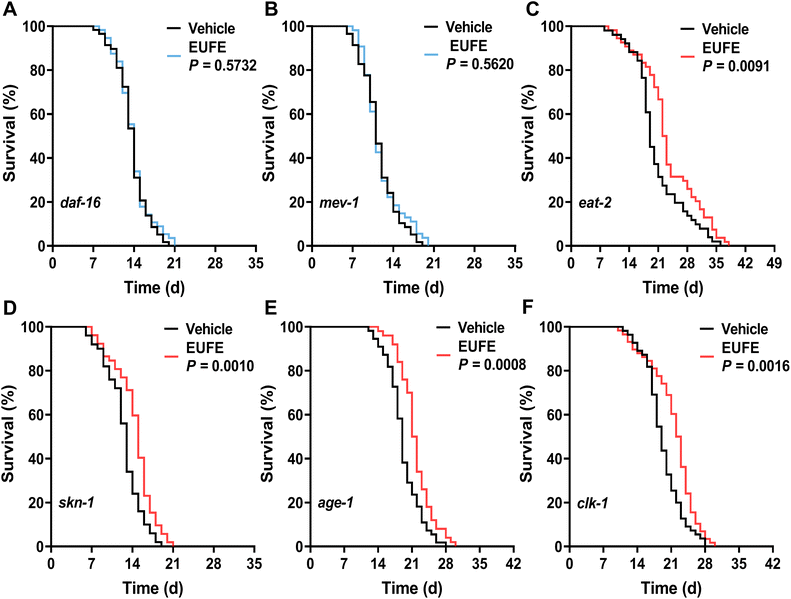 |
| Fig. 3 Lifespan-extending effect of EUFE was dependent on daf-16 and mitochondria. The survival curves of daf-16(mu86) (A), mev-1(kn1) (B), eat-2(ad465) (C), skn-1(mg570) (D), age-1(hx546) (E), and clk-1(qm30) (F) mutants treated with EUFE at 100 μg mL−1 concentration or with vehicle. P values represent the comparison with the vehicle calculated using the log-rank (Mantel–Cox) test and the ESI is summarized in eTable 7.† Each experiment was repeated at least 2 times. | |
EUFE promoted DAF-16 translocation and expression activities of downstream LGG-1 and SOD3
As the transcription factor, DAF-16 needs to enter the nucleus and bind to DNA for activating or repressing downstream target genes.38 We investigated the effect of EUFE treatment on the subcellular localization of DAF-16 in nematode whole body tissues using the TJ356 strain. The results showed that 100 μg mL−1 EUFE treatment for either 1 or 3 days significantly increased DAF-16 nuclear translocation versus the vehicle group, as reflected by the fraction of worms that were increased with the nuclear localization of DAF-16 (P < 0.05) (Fig. 4A and B).
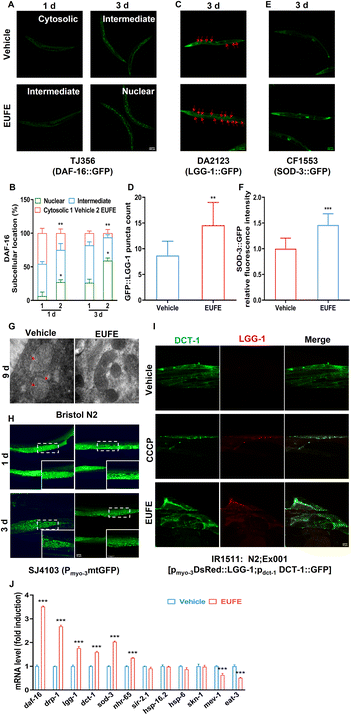 |
| Fig. 4 Related mechanisms underlying the roles of EUFE are revealed. (A) Representative images of DAF-16 localization in the TJ356 strain treated with EUFE or vehicle for 1 and 3 days. (B) Statistical graph summarizing the fraction of worms with either nuclear, intermediate, or cytosolic localization of DAF-16 after EUFE treatment. Representative images of the expression of LGG-1 in the DA2123 strain (C) or SOD3 in the CF1553 strain (E) after treatment for 3 days. Statistical graphs (D) and (F) corresponded to (C) and (E). For B, D, and F, values are mean ± SD and the number of worms was counted over 30. (G) TEM images of mitochondria within C. elegans body wall muscles under treatment for 9 days. (H) Representative images of mitochondrial morphology in SJ4103 strain's body wall muscles under treatment as (A). The white box located in the lower right was for local magnification. The magnification was 3× and the position was the dotted box. (I) Confocal images of the body wall muscle cells of worms (IR1511 strain) expressing fluorescently tagged DCT-1::GFP along with the autophagosomal marker LGG-1 (tagged with DsRed). And the nematodes were fed with EUFE or vehicle for 24 h. (J) Relative mRNA expression of daf-16 signaling, stress resistance, and mitochondrial homeostasis-related genes in worms after treatment for 1 d. act-1 mRNA acts as the loading control. Statistically significant at *P < 0.05, **P < 0.01, ***P < 0.001 by the unpaired t-test. Each experiment was repeated 3 times. The scale bar in A, C, E is 100 μm, in H and I is 10 μm, and in G is 0.2 μm. | |
As the DAF-16 target gene, lgg-1 is the orthologous gene of mammalian microtubule-associated protein light chain 3 (MAP-LC3).44 And LGG-1 is a specific marker protein for the nematode autophagosome.45 As indicated by the red arrows in Fig. 4C, compared to the vehicle group, the number of GFP::LGG-1 puncta in DA2123 was significantly increased in the EUFE-treated group (P < 0.01) (Fig. 4D), which indicated that EUFE treatment increased nematode autophagosome formation and in turn activated autophagy. Similarly, antioxidant enzyme related genes such as sod-3, ctl-1, etc. are also DAF-16 target genes.46 As a mitochondrial enzyme, superoxide dismutase 3 (SOD3) is essential to maintain the homeostasis of reactive oxygen species (ROS). By comparing the relative fluorescence intensity of the CF1553 strain, EUFE treatment for 3 days significantly increased SOD3 expression in nematodes (Fig. 4E and F). This indicated that EUFE improved the ability of mitochondria in nematodes to process ROS.
EUFE maintained mitochondrial health possibly through the early induction of mitochondrial fission and mitophagy
Considering the improved motility and muscle health of nematodes by EUFE, we narrowed our study of mitochondria to body wall muscles. We observed using TEM that after 9 days of growth in the vehicle group of nematodes, their muscle mitochondrial outer membrane appeared defective and mitochondrial ridges appeared reduced and blurred. In contrast, EUFE treatment well maintained the mitochondrial morphology (Fig. 4G).
To reflect the changes in the muscle mitochondrial network more visually, we used the SJ4103 strain. As shown in Fig. 4H, at the structural level, linear mitochondria progressively declined with the age of nematodes in the vehicle group. Conversely, the nematode muscles treated with EUFE for 3 days had more linear mitochondria. Interestingly, we observed that the mitochondrial network in muscles was more fragmented following EUFE treatment for 1 day than vehicle treatment. However, it appeared that the mitochondria of the EUFE group were in an actively divided state, as reflected by the presence of multiple mitochondrial sites of fission.
Mitophagy is a means of MQC for the removal of dysfunctional or damaged mitochondria by binding autophagosomes and subsequently undergoing lysosomal degradation. We used the IR1511 strain to explore whether EUFE treatment affected the occurrence of mitophagy in nematode body wall muscles. DCT-1 (the BNIP3L/NIX homolog) is located in the outer mitochondrial membrane and is a key regulator of mitophagy.21 As shown in Fig. 4I, consistent with the well-known mitophagy-inducer CCCP, EUFE treatment for 1 day increased LGG-1 expression in nematode body wall muscles. More importantly, EUFE increased the co-localization of LGG-1 with DCT-1, suggesting enhanced mitophagy.
EUFE affected the expression of genes involved in DAF-16 signaling and mitochondria homeostasis
As seen in Fig. 4J, 100 μg mL−1 EUFE treatment for 1 day significantly increased the expression of daf-16 and sod-3 (P < 0.001), significantly decreased the expression of mev-1 (P < 0.001), and did not affect the expression of hsp-16.2 (a heat-responsive gene) and skn-1. The protein encoded by the gene sir-2.1 is the orthologue of mammalian sirtuin 1 (SIRT1). The study showed that SIRT1 could deacetylase FoxO1 to enhance nuclear translocation and increase the expression of antioxidant enzymes.47 Regrettably, EUFE treatment did not affect the mRNA expression of the sir-2.1 gene. We selected representative genes involved in MQC, such as nhr-65 (PGC-1α) for mitochondrial biogenesis, drp-1 (DRP-1) for mitochondrial fission, eat-3 (OPA-1) for mitochondrial fusion, lgg-1 and dct-1 for mitophagy, and hsp-6 (HSPA-9) for mitochondrial proteostasis.48 It was shown that EUFE treatment for 1 day significantly increased the expression of nhr-65, drp-1, lgg-1, and dct-1 and significantly decreased the expression of eat-3, but had no effect on the expression of hsp-6. Interestingly, the reciprocal and multi-level interactions between longevity pathways and the importance of mitochondria in aging and health have led to the gradual establishment of the hypothesis that mitochondria might be involved in the healthspan modulation of these pathways. Mitochondrial health appeared to play an important role in the healthspan-prolonging activity of EUFE. Wang et al. showed that DAF-16 played a role in protecting nematode muscle and mitochondrial function during aging.49 But regrettably, due to the lack of time and means, we did not establish a direct relationship between DAF-16 and nematode muscle mitochondrial health. However, it is plausible to speculate the mechanism that EUFE might promote DAF-16 into the nucleus to cause enhanced autophagy and activated mitochondrial fission and mitophagy to ultimately maintain mitochondrial health in nematode muscles during aging. And we plan to explore this possible mechanism in the future on active monomers of EUFE.
Identification of 5 active components in EUFE
First, we determined the total phenolic, flavonoid, and terpenoid contents in 12 edible flowers with lifespan-prolonging activity. The total terpenoid content in E. ulmoides male flower stood out among them with 16.43 ± 0.17 mg linalool equivalents per g DW (eTable 8†). Moreover, the total terpenoid content in flowers was positively correlated (r = 0.64 > 0.5) with the longevity-extending activity of flowers (eTable 9†). These results aroused our interest in the terpenoid components of EUFE. Furthermore, we qualitatively identified a total of 28 compounds present in EUFE using UHPLC-QE-MS (eTable 10†). These compounds were mainly classified into iridoids, phenolic acids, flavonoids, etc. In addition, it has been reported that chlorogenic acid had the ability to prolong the longevity of nematodes.50 We performed the lifespan assays of all iridoid compounds and chlorogenic acid at 100 μM concentration, respectively. As shown in Fig. 5A and eTable 7,† aucubin, geniposide, geniposidic acid, asperuloside, and chlorogenic acid all significantly prolonged nematode longevity (P < 0.05). Among them, asperuloside was the most effective by extending the mean lifespan of nematode by 19.44% compared to the vehicle group.
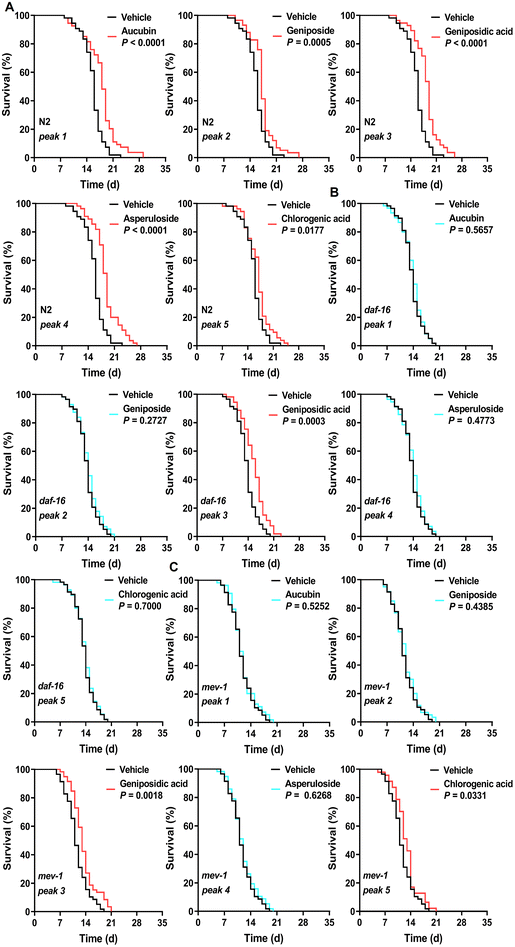 |
| Fig. 5 Effects of five identified active components in EUFE on the lifespan of different C. elegans strains. The respective survival curves of N2 (A), daf-16(mu86) (B), and mev-1(kn1) (C) nematodes treated with 5 active components at 100 μM or with vehicle. For A, N2 means C. elegans Bristol N2 strain wild-type nematodes. P values represent the comparison with the vehicle calculated using the log-rank (Mantel–Cox) test and the ESI is summarized in eTable 7.† Each experiment was repeated 3 times. | |
By a separate intervention in the daf-16 mutants and targeted daf-16 gene expression analyses in wild-type nematodes, we found that the rest of the above compounds depended on DAF-16 for their lifespan-extension activity and effectively activated the daf-16 gene expression, except geniposidic acid (Fig. 5B, eTable 7 and eFig. 8†). More interestingly, the lifespan assays of the mev-1 mutants showed that only the lifespan extension activities of aucubin, geniposide, and asperuloside were dependent on mitochondrial function (Fig. 5C and eTable 7†). We highlighted the mechanism of the lifespan-prolonging activity of geniposidic acid and chlorogenic acid different from that of EUFE, where the results of chlorogenic acid were consistent with Zheng et al.50 The chemical structures and secondary mass spectra of these five compounds are shown in Fig. 6A–F, respectively.
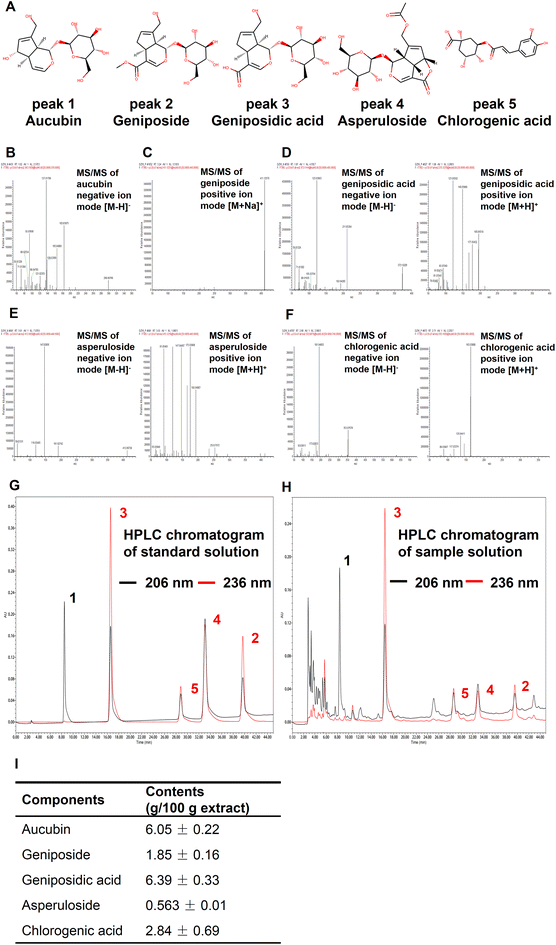 |
| Fig. 6 Qualitative and quantitative analyses of 5 active components in EUFE. (A) Chemical structures of 5 functional ingredients, including aucubin, geniposide, geniposidic acid, asperuloside, and chlorogenic acid. (B) The characteristic secondary mass spectrum of aucubin in negative ion mode. (C) The characteristic secondary mass spectrum of geniposide in positive ion mode. Characteristic secondary mass spectra of (D) geniposidic acid, (E) asperuloside, and (F) chlorogenic acid in both positive and negative ion modes. For B, C, D, E, and F, the mass spectra were obtained by UHPLC-QE-MS and components were identified qualitatively by matching the mass spectrometry database of Compound Discoverer™ or by retrieving the literature. Representative HPLC chromatograms of the (G) standard solution and (H) the sample solution at 206 nm and 236 nm for quantitative analysis. For G and H, components corresponding to the number of chromatographic peaks were consistent with (A). (I) The contents of five compounds in EUFE according to the HPLC. | |
Finally, we quantified the five components by the HPLC method. The HPLC chromatograms of the standard and sample solution are shown in Fig. 6G and H, respectively. It should be emphasized that aucubin (peak 1) had almost no absorption at 236 nm, while 206 nm was the suitable UV absorption setting condition for its quantification. The results showed that among these five compounds, geniposidic acid had the highest content of 6.39 ± 0.33% and asperuloside had the lowest content of 0.56 ± 0.01% (Fig. 6I). Compared to 15.30% of the major active components in L. japonica extracts,27 the components including iridoids and phenylpropanoids such as chlorogenic acid that we identified in EUFE were up to 17.69%. Our study showed the healthspan-enhancing activity of the flower extract and identified 5 active components, which is important for the development and utilization of this resource.
Collectively, we used the in vivo model of C. elegans to screen out EUFE with activities of lifespan and healthspan extension. And we revealed the possible mechanism of action of EUFE related to DAF-16 and mitochondrial function. Aucubin, geniposide, and asperuloside are the main active components in EUFE, from the same mechanism point of view. We believe that further animal experiments in mammals are needed to corroborate the healthspan-prolonging effects of EUFE and its main active compounds.
Abbreviations used
EUFE |
Eucommia ulmoides male flower extract |
MQC | Mitochondrial quality control |
FUDR | 5-Fluoro-2′-deoxyuridine |
CCCP | Carbonyl cyanide m-chlorophenylhydrazone |
NGM | Nematode growth medium |
TEM | Transmission electron microscopy |
GFP | Green fluorescent protein |
DsRed | Red fluorescent protein |
qPCR | Quantitative polymerase chain reaction analysis |
UHPLC-QE-MS | Q Exactive orbitrap high-resolution mass spectrometer connected to the UltiMate™ 3000 ultra-high performance liquid chromatography system |
IIS | Insulin/insulin-like growth factor |
FoxO1 | Forkhead box protein O1 |
ETC | Mitochondrial electron transport chain |
SOD3 | Superoxide dismutase 3 |
ROS | Reactive oxygen species |
SIRT1 | Mammalian sirtuin 1 |
HPLC | High performance liquid chromatography |
APCI | Antioxidant potency composite index |
Author contributions
Baiyi Lu: conceptualization, project administration, funding acquisition, and supervision. Qi Chen: conceptualization, investigation, writing – original draft, data curation, and formal analysis. Yang Xuan: supervision and writing – review & editing. Esra Capanoglu: writing – review & editing. Amel Thanina Amrouche, Lipeng Wu, Jingyang Luo and Yuhang Zhu: methodology, investigation, and validation. Yixuan Wang, Xiongtao Jiang, and Dayong Zhang: resources.
Conflicts of interest
There are no conflicts to declare.
Acknowledgements
The authors greatly thank Prof. Tavernarakis at the University of Crete for providing the nematode strains. We are also grateful to SunyBiotech (Fujian, China) and Angela Pasparaki (the technician in Prof. Tavernarakis's Lab) for their timely communication and assistance in nematode mailing, culture, etc. We also thank the Caenorhabditis Genetics Center (University of Minnesota, USA). This work was supported by the Zhejiang Provincial Basic Public Welfare Research [LGN20C200010], the Fundamental Research Funds for the Provincial Universities, Zhejiang Institute of Economics and Trade [19SBYB07], the Zhejiang Provincial Major R & D Program [2019C02070].
References
- C. López-Otín, M. A. Blasco, L. Partridge, M. Serrano and G. Kroemer, The hallmarks of aging, Cell, 2013, 153(6), 1194–1217 CrossRef PubMed.
- J. A. Amorim, G. Coppotelli, A. P. Rolo, C. M. Palmeira, J. M. Ross and D. A. Sinclair, Mitochondrial and metabolic dysfunction in ageing and age-related diseases, Nat. Rev. Endocrinol., 2022, 20(5–6), 1800404 Search PubMed.
- X. Cen, Y. Chen and X. Xu,
et al., Pharmacological targeting of MCL-1 promotes mitophagy and improves disease pathologies in an Alzheimer's disease mouse model, Nat. Commun., 2020, 11(1), 5731 CrossRef CAS PubMed.
- C. Xie, X. Zhuang and Z. Niu,
et al., Amelioration of Alzheimer's disease pathology by mitophagy inducers identified via machine learning and a cross-species workflow, Nat. Biomed. Eng., 2022, 6(1), 76–93 CrossRef CAS PubMed.
- A. Argyropoulou, N. Aligiannis, I. P. Trougakos and A. Skaltsounis, Natural compounds with anti-ageing activity, Nat. Prod. Rep., 2013, 30(11), 1412–1437 RSC.
- Y. Gao, Y. Wei, Y. Wang, F. Gao and Z. Chen,
Lycium Barbarum: A traditional Chinese herb and a promising anti-aging agent, Aging Dis., 2017, 8(6), 778–791 CrossRef PubMed.
- M. E. Rusu, R. Simedrea and A. Gheldiu,
et al., Benefits of tree nut consumption on aging and age-related diseases: Mechanisms of actions, Trends Food Sci. Technol., 2019, 88, 104–120 CrossRef CAS.
- Q. Chen, B. Xu and W. Huang,
et al., Edible flowers as functional raw materials: A review on anti-aging properties, Trends Food Sci. Technol., 2020, 106, 30–47 CrossRef CAS.
- L. Xiong, J. Yang and Y. Jiang,
et al., Phenolic compounds and antioxidant capacities of 10 common edible flowers from China, J. Food Sci., 2014, 4(79), C517–C525 CrossRef PubMed.
- V. H. Liao, Use of Caenorhabditis elegans to study the potential bioactivity of natural compounds, J. Agric. Food Chem., 2018, 66, 1737–1742 CrossRef CAS PubMed.
- G. L. Sutphin and M. Kaeberlein, Measuring Caenorhabditis elegans lifespan on solid media, J. Visualized Exp., 2009, 1152 Search PubMed.
- F. Xiao, T. Xu, B. Lu and R. Liu, Guidelines for antioxidant assays for food components, Food Front., 2020, 1(1), 60–69 CrossRef.
- N. P. Seeram, M. Aviram and Y. Zhang,
et al., Comparison of antioxidant potency of commonly consumed polyphenol-rich beverages in the United States, J. Agric. Food Chem., 2008, 56(4), 1415–1422 CrossRef CAS PubMed.
- I. Gusarov, B. Pani and L. Gautier,
et al., Glycogen controls Caenorhabditis elegans lifespan and resistance to oxidative stress, Nat. Commun., 2017, 8(1), 15868 CrossRef CAS PubMed.
- C. Kumsta, J. T. Chang, J. Schmalz and M. Hansen, Hormetic heat stress and HSF-1 induce autophagy to improve survival and proteostasis in C. elegans, Nat. Commun., 2017, 8(1), 14337 CrossRef CAS PubMed.
- D. Ryu, L. Mouchiroud and P. A. Andreux,
et al., Urolithin A induces mitophagy and prolongs lifespan in C. elegans and increases muscle function in rodents, Nat. Med., 2016, 22(8), 879–888 CrossRef CAS PubMed.
- E. M. Vayndorf, S. S. Lee and R. H. Liu, Whole apple extracts increase lifespan, healthspan and resistance to stress in Caenorhabditis elegans, J. Funct. Foods, 2013, 5(3), 1235–1243 CrossRef PubMed.
- V. Singh and A. Aballay, Regulation of DAF-16-mediated innate immunity in Caenorhabditis elegans, J. Biol. Chem., 2009, 284(51), 35580–35587 CrossRef CAS PubMed.
- H. H. Peng, C. Y. Wu and Y. C. Hsiao,
et al., Ganoderma lucidum stimulates autophagy-dependent longevity pathways in Caenorhabditis elegans and human cells, Aging, 2021, 13(10), 13474–13495 CrossRef CAS PubMed.
- P. Kittimongkolsuk, M. Roxo, H. Li, S. Chuchawankul, M. Wink and T. Tencomnao, Extracts of the tiger milk mushroom (Lignosus rhinocerus) enhance stress resistance and extend lifespan in Caenorhabditis elegans via the DAF-16/FoxO signaling pathway, Pharmaceuticals, 2021, 14(2), 93 CrossRef CAS PubMed.
- K. Palikaras, E. Lionaki and N. Tavernarakis, Coordination of mitophagy and mitochondrial biogenesis during ageing in C. elegans, Nature, 2015, 521(7553), 525–528 CrossRef CAS PubMed.
- Z. Cao, Y. Wu, K. Curry, Z. Wu, Y. Christen and Y. Luo,
Ginkgo biloba extract EGb 761 and wisconsin ginseng delay sarcopenia in Caenorhabditis elegans, J. Gerontol., Ser. A, 2007, 62(12), 1337–1345 CrossRef PubMed.
- J. Liu, Q. Zhang and R. L. Li,
et al., Anti-proliferation and anti-migration effects of an aqueous extract of Cinnamomi ramulus on MH7A rheumatoid arthritis-derived fibroblast-like synoviocytes through induction of apoptosis, cell arrest and suppression of matrix metalloproteinase, Pharm. Biol., 2020, 58(1), 863–877 CrossRef CAS PubMed.
- D. H. Truong, N. T. A. Ta and T. V. Pham,
et al., Effects of solvent-solvent fractionation on the total terpenoid content and in vitro anti-inflammatory activity of Serevenia buxifolia bark extract, Food Sci. Nutr., 2021, 9(3), 1720–1735 CrossRef CAS PubMed.
- J. Yang, H. Nam and M. Seo,
et al., OASIS: online application for the survival analysis of lifespan assays performed in aging research, PLoS One, 2011, 6(8), e23525 CrossRef CAS PubMed.
- K. Koch, N. Weldle, S. Baier, C. Büchter and W. Wätjen,
Hibiscus sabdariffa L. extract prolongs lifespan and protects against amyloid-β toxicity in Caenorhabditis elegans: involvement of the FoxO and Nrf2 orthologues DAF-16 and SKN-1, Eur. J. Nutr., 2020, 59(1), 137–150 CrossRef CAS PubMed.
- Z. Yang, Y. Yu, H. Lin, D. Liao, X. Cui and H. Wang,
Lonicera japonica extends lifespan and healthspan in Caenorhabditis elegans, Free Radical Biol. Med., 2018, 129, 310–322 CrossRef CAS PubMed.
- C. Moliner, L. Barros and M. I. Dias,
et al., Edible flowers of Tagetes erecta L. as functional ingredients: Phenolic composition, antioxidant and protective effects on Caenorhabditis elegans, Nutrients, 2018, 10(12), 2002 CrossRef PubMed.
- J. Zhang, Y. Xiao and Y. Guan,
et al., An aqueous polyphenol extract from Rosa rugosa tea has antiaging effects on Caenorhabditis elegans, J. Food Biochem., 2019, 43(4), e12796 CrossRef PubMed.
- D. Chattopadhyay and K. Thirumurugan, Longevity promoting efficacies of different plant extracts in lower model organisms, Mech. Ageing Dev., 2018, 171, 47–57 CrossRef PubMed.
- D. Fusco, G. Colloca, M. R. L. Monaco and M. Cesari, Effects of antioxidant supplementation on the aging process, Clin. Interventions Aging, 2007, 2(3), 377–387 CAS.
- Y. Xing, D. He and Y. Wang,
et al., Chemical constituents, biological functions and pharmacological effects for comprehensive utilization of Eucommia ulmoides Oliver, Food Sci. Hum. Wellness, 2019, 8(2), 177–188 CrossRef.
- S. M. A. Sayed, K. Siems, C. Schmitz-Linneweber, W. Luyten and N. Saul, Enhanced healthspan in Caenorhabditis elegans treated with extracts from the traditional Chinese medicine plants Cuscuta chinensis Lam. and Eucommia ulmoides Oliv, Front. Pharmacol., 2021, 12, 604435 CrossRef CAS PubMed.
- L. Xiong, Y. Chen and J. Tong,
et al., Epigallocatechin-3-gallate promotes healthy lifespan through mitohormesis during early-to-mid adulthood in Caenorhabditis elegans, Redox Biol., 2018, 14, 305–315 CrossRef CAS PubMed.
- S. E. Hulme, S. S. Shevkoplyas, A. P. McGuigan, J. Apfeld, W. Fontana and G. M. Whitesides, Lifespan-on-a-chip: microfluidic chambers for performing lifelong observation of C. elegans, Lab Chip, 2010, 10(5), 589–597 RSC.
- C. Huang, C. Xiong and K. Kornfeld, Measurements of age-related changes of physiological processes that predict lifespan of Caenorhabditis elegans, Proc. Natl. Acad. Sci. U. S. A., 2004, 101(21), 8084–8089 CrossRef CAS PubMed.
- H. G. Son, O. Altintas, E. J. E. Kim, S. Kwon and S. J. V. Lee, Age-dependent changes and biomarkers of aging in Caenorhabditis elegans, Aging Cell, 2019, 18(2), e12853 CrossRef PubMed.
- X. Sun, W. Chen and Y. Wang, DAF-16/FOXO transcription factor in aging and longevity, Front. Pharmacol., 2017, 8, 548 CrossRef PubMed.
- N. Senoo-Matsuda, P. S. Hartman, A. Akatsuka, S. Yoshimura and N. Ishii, A complex II defect affects mitochondrial structure, leading to ced-3- and ced-4-dependent apoptosis and aging, J. Biol. Chem., 2003, 278(24), 22031–22036 CrossRef CAS PubMed.
- E. Ziv and D. Hu, Genetic variation in insulin/IGF-1 signaling pathways and longevity, Ageing Res. Rev., 2011, 10(2), 201–204 CrossRef CAS PubMed.
- D. Weinkove, J. R. Halstead, D. Gems and N. Divecha, Long-term starvation and ageing induce AGE-1/PI3-kinase-dependent translocation of DAF-16/FOXO to the cytoplasm, BMC Biol., 2006, 4(1), 1 CrossRef PubMed.
- J. A. Butler, N. Ventura, T. E. Johnson and S. L. Rea, Long-lived mitochondrial (Mit) mutants of Caenorhabditis elegans utilize a novel metabolism, FASEB J., 2010, 24(12), 4977–4988 CAS.
- T. Jonassen, B. N. Marbois, K. F. Faull, C. F. Clarke and P. L. Larsen, Development and fertility in Caenorhabditis elegans clk-1 mutants depend upon transport of dietary coenzyme Q8 to mitochondria, J. Biol. Chem., 2002, 277(47), 45020–45027 CrossRef CAS PubMed.
- L. Yang, Q. Ye and X. Zhang,
et al., Pyrroloquinoline quinone extends Caenorhabditis elegans’ longevity through the insulin/IGF1 signaling pathway-mediated activation of autophagy, Food Funct., 2021, 12(22), 11319–11330 RSC.
- A. Melendez, Z. Talloczy, M. Seaman, E. L. Eskelinen, D. H. Hall and B. Levine, Autophagy genes are essential for dauer development and life-span extension in C. elegans, Science, 2003, 301(5638), 1387–1391 CrossRef CAS PubMed.
- H. Wang, S. Zhang and L. Zhai,
et al., Ginsenoside extract from ginseng extends lifespan and health span in Caenorhabditis elegans, Food Funct., 2021, 12(15), 6793–6808 RSC.
- L. Mouchiroud, R. H. Houtkooper and N. Moullan,
et al., The NAD+/Sirtuin pathway modulates longevity through activation of mitochondrial UPR and FOXO signaling, Cell, 2013, 154(2), 430–441 CrossRef CAS PubMed.
- A. Picca, R. Calvani and C. Leeuwenburgh,
et al., Targeting mitochondrial quality control for treating sarcopenia: lessons from physical exercise, Expert Opin. Ther. Targets, 2019, 23(2), 153–160 CrossRef PubMed.
- H. Wang, P. Webster, L. Chen and A. L. Fisher, Cell-autonomous and non-autonomous roles of daf-16 in muscle function and mitochondrial capacity in aging C. elegans, Aging, 2019, 11, 2295–2311 CrossRef CAS PubMed.
- S. Zheng, X. Huang, T. Xing, A. Ding, G. Wu and H. Luo, Chlorogenic acid extends the lifespan of Caenorhabditis elegans via Insulin/IGF-1 signaling pathway, J. Gerontol., Ser. A, 2017, 72(4), 464–472 CAS.
|
This journal is © The Royal Society of Chemistry 2023 |
Click here to see how this site uses Cookies. View our privacy policy here.