DOI:
10.1039/D2FO02877H
(Paper)
Food Funct., 2023,
14, 516-524
Diosgenin relieves oxaliplatin-induced pain by affecting TLR4/NF-κB inflammatory signaling and the gut microbiota
Received
27th September 2022
, Accepted 5th December 2022
First published on 9th December 2022
Abstract
Diosgenin extracted from fenugreek, yam and other foods exhibits a wide range of pharmacological activities, especially for the treatment of pain and other nervous system diseases. However, its role in oxaliplatin-induced peripheral neuropathy (OIPN) is unclear. To explore its detailed mechanism on the pain caused by chemotherapy, we carried out this experiment. In this study, the effects of diosgenin on injured PC12 cells and OIPN mice were examined. The results showed that diosgenin not only protected PC12 from injury, but also reduced the mechanical withdrawal threshold and cold hyperalgesia in OIPN mice. Diosgenin inhibited oxidative stress, the cell glial fibrillary acidic protein, and the pro-inflammatory cytokines such as tumor necrosis factor-α, toll-like receptor 4 and nuclear factor-κB in the brain. Furthermore, the fecal microbiota transplantation experiment indicated that diosgenin improved OIPN through regulation of the gut microbiota. All in all, diosgenin ameliorates peripheral neuropathy and is worthy of further study in the treatment of neuropathic pain.
Introduction
Chemotherapy-induced peripheral neuropathy (CIPN) occurs with a prevalence from 19% to over 85% in patients receiving antineoplastic agents.1 More than 30% of CIPN patients have peripheral neuropathic pain (NP), which continues to deteriorate for months or even years.2 Oxaliplatin (OXA)-induced peripheral neuropathy (OIPN) is a common adverse reaction for cancer patients receiving chemotherapy.3 To date, there is no drug to thoroughly treat neuralgia caused by chemotherapy.4 Peripheral nerve injury elicits an inflammatory response, involving the activation of microglia, the secretion of pro-inflammatory mediators, and alterations of pain-related signaling molecules.5 Currently, the microbiota–gut–brain axis is drawing increasing attention.6 Recent evidence suggests that gut microbiota may also play a key role in many other types of chronic pain.2
Diosgenin is the main active ingredient in foods such as fenugreek and yam. Due to its wide range of pharmacological activities and medicinal properties, it has been used in the prevention and treatment of neurological diseases,7 such as Alzheimer's disease,8 spinal cord injury,9 cerebral ischemia reperfusion10 and neuropathic pain.11 It has been reported that oral diosgenin can be retained in the intestine for a long time and can be detected in the brain,12 suggesting that diosgenin can penetrate the blood–brain barrier and may regulate the gut microbiota. However, the effect of diosgenin on neuropathic pain caused by OXA and whether it is involved in the regulation of gut microbiota are unclear. Therefore, we studied the potential molecular mechanism of diosgenin in inhibiting neuropathic pain caused by chemotherapy.
Materials and methods
Drugs
Diosgenin was obtained from Nanjing Herbaceous Source Biotechnology Co., Ltd (Nanjing, China). OXA was obtained from Heowns Biochem Technologies LLC (Tianjin, China).
Animals
Male C57BL/6 mice (6 weeks old) were purchased from Beijing HFK Bioscience Co., Ltd (license no. SCXK (Jing) 2019-0008, Beijing, China). They were housed individually in a clean and open room maintained at a stable temperature (22 °C) on a 12/12 h light/dark cycle, and were given access to food and water ad libitum. The mice were grouped according to the experiment after 1 week of adaption. All the experiments were approved by the national legislation of China and local guidelines (Tianjin University of Science & Technology, 20200331).
Evaluation of the analgesic diosgenin
After habituation to the test environment and baseline measurements of pain sensitivity, the normal group was intraperitoneally injected with 5% glucose (10 mL kg−1). The others received an intraperitoneal injection with 3 mg per 10 mL per kg of OXA (dissolved in 5% glucose solution) for 5 days, followed by 5 days of rest, and another 5-day injection of OXA, which made the cumulative dose of OXA reach 30 mg kg−1 (OXA group). In addition, diosgenin-treated mice were also orally administered 30 mg per 10 mL per kg of diosgenin daily for 2 weeks (diosgenin group) (Fig. 1A).
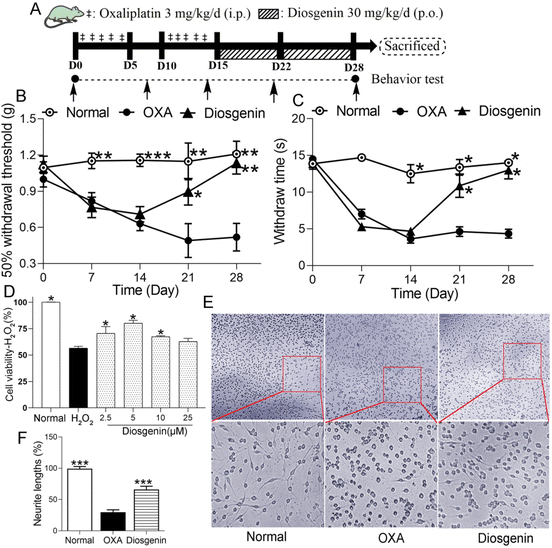 |
| Fig. 1 The effect of diosgenin on OXA-induced peripheral neuropathy was studied in vivo and in vitro. (A) The scheme of the OIPN model. The MWT (B) and cold allodynia tests (C) evaluated the effect of diosgenin on OIPN. (D) The protective effect of diosgenin on H2O2-induced PC12 injury. (E) The morphological changes of PC12 cells treated with OXA or diosgenin. (F) The length of the neurite. *p < 0.05, **p < 0.01 and ***p < 0.001 compared with the OXA group. | |
The fecal material was collected and isolated as previously reported.13 The normal group treated with diosgenin or the normal group was used as a donor to collect the gut microbiota. The fecal pellets were collected under SPF conditions and 100 mg was resuspended in 1 mL of sterile saline. The solution was vigorously mixed for 10 s using a vortexer, before centrifugation at 800g for 3 min. The supernatant was collected and used as the transplant material. The fecal microbiota transplant (FMT) was freshly prepared on the day of transplantation within 30 min before gavage to prevent changes in the bacterial composition.
Mechanical withdrawal threshold (MWT) assessment
Von Frey hairs were used to test mechanical allodynia.14 Briefly, each mouse was placed individually in a transparent plastic box and allowed to adapt to the environment for three days (15 min day−1) before the behavior tests. Different strengths of von Frey fibers were used alternately to stimulate the mid-plantar surface of the hind paw. The absolute paw withdrawal threshold (g) was measured as the force that caused the mouse to withdraw its paw. The “up and down” method was used to define the mechanical sensitivity that produced a 50% likelihood of paw withdrawal.
Cold allodynia assessment
Cold allodynia was assessed using the paw immersion test.15 Three day before the baseline assessment, the mice were habituated to the test. The hind paw was immersed in a water bath maintained at 15.0 ± 0.2 °C until paw withdrawal or a sign of struggle was observed. The paw withdrawal latency was measured (cut-off time, 15 s).
Assessment of the oxidative stress markers, IL-1β and LPS
The concentrations of malondialdehyde (MDA), reduced glutathione (GSH) and superoxide dismutase (SOD) were measured in the brain homogenates utilizing kits (Nanjing Jiancheng Bioengineering Institute, Nanjing, China). The concentrations of IL-1β (RX203063M, Rinxin, Ruixinbio, Quanzhou, China) and lipopolysaccharides (LPS) (SBJ-M0941, SenBeiJia Biological Technology Co., Ltd, Nanjing, China) in the serum were determined using ELISA, and the experimental operation was conducted in strict accordance with the kit instructions. The number of measured samples in each group was greater than three.
RT-PCR
The total RNA was extracted from the brain tissues using the TRIzol reagent (Solarbio, Tianjin, China). The subsequent reverse transcription was performed according to the protocol provided with the polymerase chain reaction (PCR) kit (ABM, Canada). The cDNA was amplified with specific primers (Table 1) to investigate the mRNA. The PCR reaction was conducted at 95 °C for 30 s, followed by a thermal cycle of 5 s at 95 °C, 30 s at 60 °C (repeated for 30 cycles), and a final stabilization at 4 °C. The expression ratio of mRNA in the brain was normalized to Gapdh and analyzed.
Table 1 The primer sequences and cycling conditions for PCR
Gene |
Primer forward (5′–3′) |
Primer reverse (5′–3′) |
Gapdh
|
ATTCAACGGCACAGTCAAGG |
GCAGAAGGGGCGGAGATGA |
Tlr4
|
ATGGCATGGCTTACACCACC |
GAGGCCAATTTTGTCTCCACA |
Tnf
|
CTTCTGTCTACTGAACTTCGGG |
CAGGCTTGTCACTCGAATTTTG |
Nfkb1
|
AAATCCTACCCACAGGTCAA |
CGTGCTTCCAGTGTTTCA |
Gfap
|
CGGAGACGCATCACCTCTG |
AGGGAGTGGAGGAGTCATTCG |
Western blot analysis
The total brain tissue from the mice was homogenized in RIPA buffer containing 1 mM phenylmethylsulfonyl fluoride (PMSF) and centrifuged at 12
000 rpm for 10 min at 4 °C. The supernatants were collected. The protein extract was diluted with 1/3 of the 4× loading buffer and heated to 95 °C for 5 min for denaturation. The proteins extracted were separated using SDS-PAGE and transferred to polyvinylidene fluoride (PVDF) membranes. Then they were incubated with primary antibodies overnight at 4 °C after blocking with 5% nonfat milk for 2 h at room temperature. The antibodies used were rabbit anti-GFAP (1
:
1000), mouse anti-TLR4 (1
:
1000), mouse anti-MyD88 (1
:
1000), mouse anti-p-NF-κB p65 (1
:
1000), mouse anti-NF-κβ p65 (1
:
200), mouse anti-IL-1β (1
:
1000) and rabbit anti-IL-6 (1
:
1000). The membranes were incubated with appropriate secondary antibodies.
H2O2-induced oxidative injury in PC12 cells
In order to evaluate whether diosgenin relieves H2O2-induced cell damage, the cell viability was estimated using an MTT assay. Briefly, cells were seeded in 96-well culture plates (1 × 103 cells per well). Prior to exposure to 100 μM H2O2 for 2 h, PC12 cells were pretreated with diosgenin (2.5, 5, 10, or 25 μM) for 24 h. Then 20 μL of the MTT solution was added to each well, and the mixtures were incubated for an additional 4 h at 37 °C. Finally, the cells were dissolved in 100 μL of DMSO, and the absorbance was measured at 570 nm using a microplate reader.
OXA-induced PC12 cell injury
The PC12 cells were seeded into collagen-coated 24-well plates at a density of 2.5 × 104 cells per cm2 for 24 h. The old medium was removed, and a medium with 5 μM diosgenin was added to pretreat the samples for 2 h, followed by the addition of 3 μM OXA for another 24 h. Then the cell morphology was observed using an inverted microscope.
Fecal microbiota analysis by 16S rRNA sequencing
The gut microbiota was analyzed using 16S rRNA sequencing. In briefly, fresh feces were obtained from each group after 2-week administration of diosgenin. The genome of the gut microbiota was isolated using a FastDNA Spin Kit (Qbiogene, Carlsbad, CA, USA). The 16S rRNA was amplified by PCR using the forward primer, 5′-GAGAGTTTGATCCTGGCTCAG-3′ and the reverse primer, 5′-GGTTACCTTGTTACGACTT-3′. A heatmap and a taxon relative abundance bar diagram were created using the website of Majorbio.
Data analysis
IBM SPSS Statistics 21.0 was used to analyze the data. Data have been expressed as the means ± SEM. One-way variance analysis and Duncan's multiple range test were used to determine the significantly different groups.
Results
Analgesic activity of diosgenin
To study the protective effect of diosgenin on peripheral neuropathy induced by oxaliplatin, we performed in vivo and in vitro experiments. In the OIPN test (Fig. 1A), as shown in Fig. 1B and C, there were no significant differences in the MWT or cold allodynia tests among the groups before OXA injection (p > 0.05). After 2-week accumulation of OXA, OXA significantly decreased the MWT and paw withdrawal latency compared with the normal group (p < 0.001). Diosgenin treatment alleviated these data compared with the OXA group (p < 0.01). These results suggest that diosgenin should exhibit good analgesic activity.
The protective effect of diosgenin on H2O2- or OXA-induced PC12 cell injury
PC12 pheochromocytoma cells have been used as a model of neurodegeneration due to their neural differentiation. The in vitro cell experiments showed that diosgenin had a good protective effect on PC12 nerve injury. Diosgenin had a significant protective effect against H2O2-induced PC12 injury (Fig. 1D). The survival rate of PC12 cells exposed to H2O2 was significantly reduced, while it was significantly increased after the diosgenin treatment (P < 0.05). As shown in Fig. 1E, nerve damage to the PC12 cells directly affected their morphology. Normally, spindle-shaped and long-and-thick protrusion appeared in PC12 cells, which were regarded as symbols of neurons.16 OXA treatment significantly reduced the neurite length in the PC12 cells. Diosgenin treatment increased the length of the neurites in OXA-treated PC12 cells (Fig. 1F). These data indicated that diosgenin exerts a protective effect on H2O2 or OXA-induced PC12 cell injury.
Diosgenin represses oxidative stress and the TLR4/NF-κB pathway in the brain
To confirm the antioxidant effect of diosgenin, we measured the contents of MDA, SOD and GSH in the brain tissues. The OXA injection decreased the activity of SOD (p < 0.001) and increased the content of MDA (p < 0.05) in the brain tissues compared with the normal mice. Diosgenin treatment significantly reduced the production of MDA and increased the activities of GSH and SOD (Fig. 2A–C) in the OXA group. Furthermore, glial activation and neuroinflammation are considered as important components of the central sensitization mechanism in neuropathic pain.17 To confirm the anti-inflammatory effects of diosgenin, the protein expressions of the glial fibrillary acidic protein (GFAP), TLR4, MyD88, p-NF-κB p65, and IL-1β along with IL-6 were measured in the brain (Fig. 2D1). Compared with the normal group, the OXA injection significantly increased the levels of these proteins (p < 0.001). Similarly, RT-PCR analysis also indicated that OXA injection significantly increased the mRNA expressions of GFAP, TLR4, NF-κB and TNF-α (Fig. 2E1) (p < 0.05), while the diosgenin treatment significantly reduced their expressions (Fig. 2D2–D7 and E2) (p < 0.05). These results suggested that diosgenin counters the neuroinflammatory damage caused by the OXA-induced TLR4/NF-κB axis.
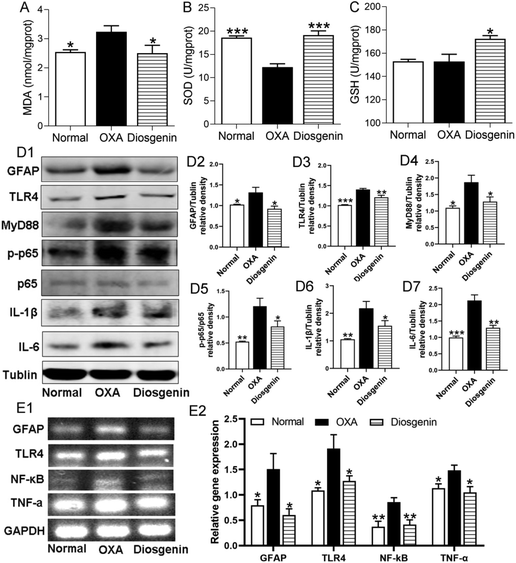 |
| Fig. 2 Effects of diosgenin on oxidative stress and the TLR4/NF-κB signaling pathway in the brain of OXA mice. Effects of diosgenin on the content of MDA (A) and the activities of SOD (B) and GSH (C) in the brain after OIPN. (D1) The representative western blot bands of GFAP, TLR4, MyD88, p-NF-κB p65, NF-κB p65, IL-1β and IL-6. (D2–D7) The relative protein expression levels of GFAP/β-tubulin, TLR4/β-tubulin, MyD88/β-tubulin, p-NF-κB p65/NF-κB p65, IL-1β/β-tubulin and IL-6/β-tubulin. (E1 and E2) RT-PCR analyzing the mRNA expressions of GFAP, TLR4, NF-κB and TNF-α in the brain. *p < 0.05 and ***p < 0.001 compared with the OXA group. | |
Diosgenin regulated the gut microbiota and reduced LPS-induced inflammation
As shown in Fig. 3A–C, the serum concentrations of LPS and IL-1β and the colon mRNA expression of TLR4 were significantly increased in the OXA group compared with those in the normal group (p < 0.05). Diosgenin treatment remarkably decreased their levels compared with those in the OXA group (p < 0.05). As is known, the gut microbiota plays a critical role in OXA mice. In this research, the pan/core species curve and NMDS analysis which reflected the richness and diversity of biological communities were significantly different among the three groups.18 Diosgenin significantly reversed the decrease in bacterial richness and diversity caused by OXA, and adjusted the balance of gut microbiota (Fig. 3D–F). As shown in Fig. 3G–L, the OXA injection reduced the abundances of Firmicutes, Erysipelotrichaceae, Lachnospiraceae and Prevotellaceae and increased the abundance of Bacteroides. Furthermore, OXA reduced the ratio of Firmicutes to Bacteroidetes.
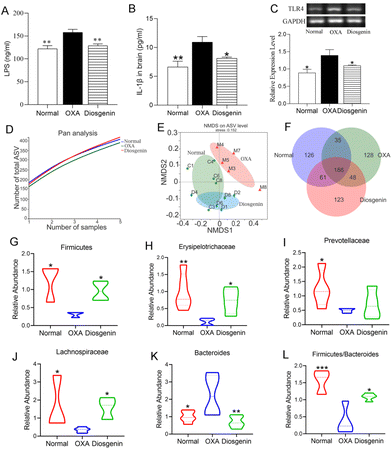 |
| Fig. 3 Diosgenin treatment ameliorated the OXA-induced gut microbiota disorder and LPS-induced inflammation. The contents of LPS (A) and IL-1β (B) in the sera of mice. (C) The mRNA expression of TLR4 in the colons. (D) The bacterial pan/core species curve of the mouse feces. (E) Non-metric multidimensional scaling analysis in the beta diversity analysis. (F) The Wayne cluster diagram for each group. (G–L) The abundance of the microbiota among each group. *p < 0.05 and **p < 0.01 vs. the OXA group. | |
Diosgenin alleviated OIPN depending on the gut microbiota
To verify whether the alleviating effect of diosgenin on OIPN is dependent on the gut microbiota, the feces from the normal and diosgenin-treated mice were used as the FMT. As shown in Fig. 4, the pain threshold of the OXA mice was increased by the FMT treatment. Diosgenin administration inhibited the TLR4/NF-κB pathway. In order to verify the effect of FMT (diosgenin) on this pathway, RT-PCR was used to analyze the brain tissues. As a result, the FMT (diosgenin) also down-regulated the mRNA expressions of GFAP, TLR4, NF-κB and TNF-α. The above results indicate that diosgenin should be dependent on the gut microbiota to exert an analgesic effect.
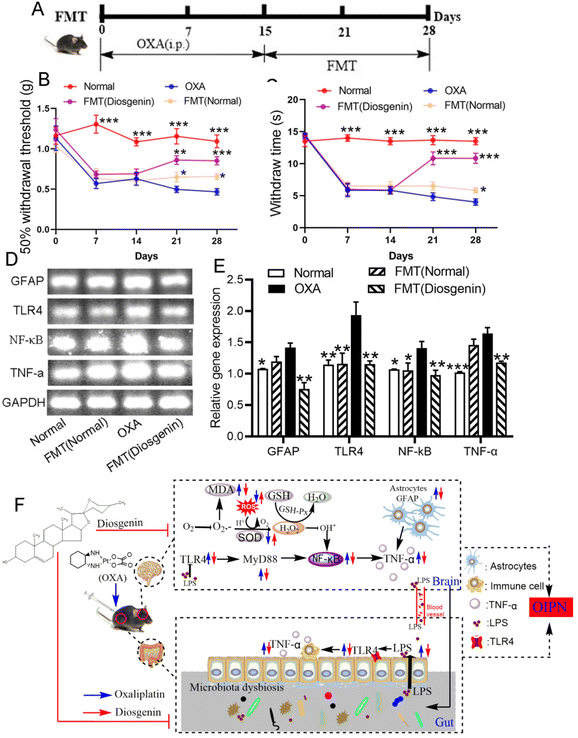 |
| Fig. 4 Diosgenin alleviated OIPN depending on the gut microbiota. (A) The scheme of the FMT treatment for OIPN. The MWT (B) and cold allodynia tests (C) evaluated the effect of FMT on the OXA mice. (D) The mRNA expressions of GFAP, TLR4, NF-κβ and TNF-α. (E) The relative gene expressions of GFAP, TLR4, NF-κB and TNF-α in the brain tissues after the FMT treatment. (F) The protective mechanism of diosgenin against OXA-induced neuropathic pain. *p < 0.05, **p < 0.01 and ***p < 0.001 vs. the OXA group. | |
Discussion
Pain can be divided into nociceptive pain, inflammatory pain, and neuropathic pain.19 It has been reported that diosgenin has therapeutic effects on the neuropathic pain of chronic constriction injury20 and diabetic rats.11 In order to better explore the role of diosgenin in neuralgia, we performed in vivo and in vitro experiments to evaluate the potential molecular mechanism of diosgenin in inhibiting neuropathic pain caused by chemotherapy.
Since PC12 cells are similar to neurons in structure and function, the PC12 cell injury model is widely used in the study of nerve cell injury models.21 In this research, OXA and H2O2 were used to induce PC12 cell injury. In the H2O2 injury model, 2.5–10 μM diosgenin significantly improved the survival rate of cells. Therefore, we chose 5 μM diosgenin to study the damage of PC12 cells induced by OXA. Normally, long and thick protrusions are regarded as the symbols of neurons.16 When treated with OXA alone, the PC12 cells seemed to be round in shape and contracted, and the neurites became shorter, whereas most cells remained normal after the treatment with diosgenin. These data indicated that diosgenin had a good protective effect on nerve injury models in vitro.
Subsequently, we established a neuralgia model induced by OXA. In this research, diosgenin increased the MWT and paw withdrawal latency in the OIPN model, showing a better analgesic effect. It is reported that the mechanisms of chronic OIPN formation are involved in excessive oxidative stress injury, glial activation, neuroinflammation,22 intestinal flora disorder23,24 and so forth. Inflammation is the major cause of neuropathic pain.25 The pathogenesis of neuropathic pain involves various pain-related molecules, especially for the TLR4/NF-κB signaling pathway, which is one of the most critical cascades in response to the inflammatory process.26 Astrocytes play an important role in the occurrence and maintenance of pain.27 They are activated by inflammatory stress and lead to the secretion of pro-inflammatory factors such as TNF-α,28 IL-1β and IL-6,29 which are involved in the maintenance of chronic pain.27 To verify the effect of diosgenin on OIPN, we detected the TLR4/NF-κB pathway and astrocytes. In our study, the expression of GFAP was significantly increased in the OXA mice, indicating that the OXA injection significantly activated the astrocytes. Subsequently, it increased the expressions of TLR4, MyD88, p-NF-κB, TNF-α, IL-1β and IL-6, which indicated that the OXA injection activated the TLR4/NF-κB signalling pathway. Diosgenin significantly inhibited the astrocyte markers and TLR4/NF-κB signalling pathway, and thereby inhibited the production of inflammatory factors to relieve chronic pain.
Oxidative stress and inflammation are closely related to pathophysiological processes and involved in the pathogenesis of neuropathic pain.30 Oxidative stress stimulates numerous intracellular signaling pathways and causes the production of pro-inflammatory cytokines.31 It is known that the brain is more vulnerable to oxidative stress than any other organ because of its higher oxygen consumption, higher lipid content and weaker antioxidant defense.32 When cells are exposed to oxidative stress, NF-κB can be activated and phosphorylated into the nucleus, and then up-regulate pro-inflammatory cytokines such as TNF-α.33 In this research, we examined the biomarkers of oxidative stress in the brain tissues. As a result, diosgenin significantly reduced the biomarker of lipid damage, MDA, and increased the activities of antioxidants such as SOD and GSH34 in the brain to alleviate pain.
The gut microbiota plays a crucial role in the bidirectional communication between the gut and the brain.35 For example, the gut microbes may shape neural development, modulate neurotransmission, and affect behavior, and can thereby contribute to the progression of neurodevelopmental and neuropsychiatric disorders.35 In our study, we analyzed changes in the related bacteria. It is reported that chemotherapy-induced peripheral neuropathy reduces the abundances of Firmicutes,36 Prevotellaceae, Lachnospiraceae and Erysipelotrichaceae37 and increases the abundance of Bacteroides.36,38 The gut microbiota can be remodeled to suppress the LPS/TLR4/NF-κB axis by increasing the ratio of Firmicutes to Bacteroidetes.39 Diosgenin, taken orally, can remain in the intestine for a long time.40 In order to evaluate the improvement of diosgenin on OIPN and explore its relationship with the changes in the gut microbiota, we measured the data of the microbiota. In our study, diosgenin reversed the changes of the microbiota induced by OXA and increased the richness and diversity of the gut microbiota. It increased the abundances of Firmicutes, Erysipelotrichaceae and Lachnospiraceae and decreased the amount of Bacteroides. Furthermore, it increased the ratio of Firmicutes to Bacteroidetes.
In addition, gut microbiota influences the development of OIPN through the LPS-TLR4 pathway.23 LPS plays an important role in the gut–brain dialogue, which induces blood–brain barrier damage;41 the content of LPS and the expression of TLR4 were tested in the serum and colon. As a result, their levels were significantly increased in the OXA group compared with those in the normal group, while diosgenin treatment significantly reversed these levels. Furthermore, considering the close relationship between neuralgia and intestinal flora, we set up a FMT experiment on OXA mice to verify the dependence of diosgenin exerting analgesic effects on the gut microbiota. The FMT (diosgenin) preserved the species of microbiota and significantly improved the mechanical pain threshold of OIPN. These results suggest that the analgesic effect of diosgenin on OIPN is based on the regulation of the intestinal flora.
In conclusion, diosgenin relieved the pain induced by chemotherapy caused by OXA. On the one hand, diosgenin distributed in the intestine regulated the gut microbiota, reduced the release of LPS and inhibited the expression of TLR4, which could inhibit inflammatory pathways in the intestine. On the other hand, brain-infused diosgenin attenuated OXA-induced neuroinflammation by inhibiting oxidative stress and the TLR4/MyD88/NF-κB pathway. These results suggest that diosgenin can exhibit good analgesic activity in OIPN patients.
Abbreviations
CIPN | Chemotherapy-induced peripheral neuropathy |
FMT | Fecal microbiota transplantation |
GFAP | Glial fibrillary acidic protein |
GSH | Glutathione |
MDA | Malondialdehyde |
MWT | Mechanical withdrawal threshold |
NF-κB | Nuclear factor kappa B |
NP | Neuropathic pain |
OIPN | Oxaliplatin-induced peripheral neuropathy |
OXA | Oxaliplatin |
SOD | Superoxide dismutase |
TLR4 | Toll-like receptor 4 |
TNF-α | Tumor necrosis factor-α |
Conflicts of interest
All authors declare no conflict of interest.
Acknowledgements
This work was supported by the grant YJSKC2021S23 from the Tianjin University of Science & Technology and the grant 82074069 from the National Natural Science Foundation of China.
References
- R. Zajaczkowska, M. Kocot-Kepska, W. Leppert, A. Wrzosek, J. Mika and J. Wordliczek, Mechanisms of chemotherapy-induced peripheral neuropathy, Int. J. Mol. Sci., 2019, 20(6), 1451 CrossRef CAS PubMed.
- R. Guo, L. H. Chen, C. Xing and T. Liu, Pain regulation by gut microbiota: Molecular mechanisms and therapeutic potential, Br. J. Anaesth., 2019, 123(5), 637–654 CrossRef CAS PubMed.
- Y. Li, M. B. Lustberg and S. Hu, Emerging pharmacological and non-pharmacological therapeutics for prevention and treatment of chemotherapy-induced peripheral neuropathy, Cancers, 2021, 13(4), 766 CrossRef CAS PubMed.
- S. Rottenberg, C. Disler and P. Perego, The rediscovery of platinum-based cancer therapy, Nat. Rev. Cancer, 2021, 21(1), 37–50 CrossRef CAS PubMed.
- X. Y. Wang, H. J. Ma, M. Xue, Y. L. Sun, A. Ren, M. Q. Li, Z. H. Huang and C. Huang, Anti-nociceptive effects of Sedum lineare Thunb. on spared nerve injury-induced neuropathic pain by inhibiting TLR4/NF-kappaB signaling in the spinal cord in rats, Biomed. Pharmacother., 2021, 135, 111215 CrossRef CAS PubMed.
- B. Lin, Y. Wang, P. Zhang, Y. Yuan, Y. Zhang and G. Chen, Gut microbiota regulates neuropathic pain: Potential mechanisms and therapeutic strategy, J. Headache Pain, 2020, 21(1), 103 CrossRef PubMed.
- B. Cai, Y. Zhang, Z. Wang, D. Xu, Y. Jia, Y. Guan, A. Liao, G. Liu, C. Chun and J. Li, Therapeutic potential of diosgenin and its major derivatives against neurological diseases: Recent advances, Oxid. Med. Cell. Longevity, 2020, 2020, 3153082 Search PubMed.
- C. Tohda, X. Yang, M. Matsui, Y. Inada, E. Kadomoto, S. Nakada, H. Watari and N. Shibahara, Diosgenin-rich yam extract enhances cognitive function: A placebo-controlled, randomized, double-blind, crossover study of healthy adults, Nutrients, 2017, 9(10), 1160 CrossRef PubMed.
- X. B. Chen, Z. L. Wang, Q. Y. Yang, F. Y. Zhao, X. L. Qin, X. E. Tang, J. L. Du, Z. H. Chen, K. Zhang and F. J. Huang, Diosgenin glucoside protects against spinal cord injury by regulating autophagy and alleviating apoptosis, Int. J. Mol. Sci., 2018, 19(8), 2274 CrossRef PubMed.
- X. Zhang, X. Wang, Z. Xue, G. Zhan, Y. Ito and Z. Guo, Prevention properties on cerebral ischemia reperfusion of medicine food homologous Dioscorea yam-derived diosgenin based on mediation of potential targets, Food Chem., 2021, 345, 128672 CrossRef CAS PubMed.
- Z. Kiasalari, T. Rahmani, N. Mahmoudi, T. Baluchnejadmojarad and M. Roghani, Diosgenin ameliorates development of neuropathic pain in diabetic rats: Involvement of oxidative stress and inflammation, Biomed. Pharmacother., 2017, 86, 654–661 CrossRef CAS PubMed.
- G. Wang, R. Hao, Y. Liu, Y. Wang, S. Man and W. Gao, Tissue distribution, metabolism and absorption of Rhizoma Paridis Saponins in the rats, J. Ethnopharmacol., 2021, 273, 114038 CrossRef CAS PubMed.
- Y. Jing, Y. Yu, F. Bai, L. Wang, D. Yang, C. Zhang, C. Qin, M. Yang, D. Zhang, Y. Zhu, J. Li and Z. Chen, Effect of fecal microbiota transplantation on neurological restoration in a spinal cord injury mouse model: Involvement of brain-gut axis, Microbiome, 2021, 9(1), 59 CrossRef CAS PubMed.
- S. B. Zhang, S. Y. Lin, M. Liu, C. C. Liu, H. H. Ding, Y. Sun, C. Ma, R. X. Guo, Y. Y. Lv, S. L. Wu, T. Xu and W. J. Xin, CircAnks1a in the spinal cord regulates hypersensitivity in a rodent model of neuropathic pain, Nat. Commun., 2019, 10(1), 4119 CrossRef PubMed.
- P. Y. Martin, S. Doly, A. M. Hamieh, E. Chapuy, V. Canale, M. Drop, S. Chaumont-Dubel, X. Bantreil, F. Lamaty, A. J. Bojarski, P. Zajdel, A. Eschalier, P. Marin and C. Courteix, mTOR activation by constitutively active serotonin6 receptors as new paradigm in neuropathic pain and its treatment, Prog. Neurobiol., 2020, 193, 101846 CrossRef CAS PubMed.
- L. Zhang, X. Chen, L. Wu, Y. Li, L. Wang, X. Zhao, T. Zhao, L. Zhang, Z. Yan and G. Wei, Ameliorative effects of escin on neuropathic pain induced by chronic constriction injury of sciatic nerve, J. Ethnopharmacol., 2021, 267, 113503 CrossRef CAS PubMed.
- E. A. Old, A. K. Clark and M. Malcangio, The role of glia in the spinal cord in neuropathic and inflammatory pain, Handb. Exp. Pharmacol., 2015, 227, 145–170 Search PubMed.
- L. Chen, W. Xu, A. Lee, J. He, B. Huang, W. Zheng, T. Su, S. Lai, Y. Long, H. Chu, Y. Chen, L. Wang, K. Wang, J. Si and S. Chen, The impact of Helicobacter pylori infection, eradication therapy and probiotic supplementation on gut microenvironment homeostasis: An open-label, randomized clinical trial, EBioMedicine, 2018, 35, 87–96 CrossRef CAS PubMed.
- M. Costigan, J. Scholz and C. J. Woolf, Neuropathic pain: A maladaptive response of the nervous system to damage, Annu. Rev. Neurosci., 2009, 32, 1–32 CrossRef CAS PubMed.
- W. X. Zhao, P. F. Wang, H. G. Song and N. Sun, Diosgenin attenuates neuropathic pain in a rat model of chronic constriction injury, Mol. Med. Rep., 2017, 16(2), 1559–1564 CrossRef CAS PubMed.
- L. Wang, Z. Yin, F. Wang, Z. Han, Y. Wang, S. Huang, T. Hu, M. Guo and P. Lei, Hydrogen exerts neuroprotection by activation of the miR-21/PI3K/AKT/GSK-3beta pathway in an in vitro model of traumatic brain injury, J. Cell. Mol. Med., 2020, 24(7), 4061–4071 CrossRef CAS PubMed.
- L. Kang, Y. Tian, S. Xu and H. Chen, Oxaliplatin-induced peripheral neuropathy: Clinical features, mechanisms, prevention and treatment, J. Neurol., 2021, 268(9), 3269–3282 CrossRef CAS PubMed.
- S. Shen, G. Lim, Z. You, W. Ding, P. Huang, C. Ran, J. Doheny, P. Caravan, S. Tate, K. Hu, H. Kim, M. McCabe, B. Huang, Z. Xie, D. Kwon, L. Chen and J. Mao, Gut microbiota is critical for the induction of chemotherapy-induced pain, Nat. Neurosci., 2017, 20(9), 1213–1216 CrossRef CAS PubMed.
- J. L. Alexander, I. D. Wilson, J. Teare, J. R. Marchesi, J. K. Nicholson and J. M. Kinross, Gut microbiota modulation of chemotherapy efficacy and toxicity, Nat. Rev. Gastroenterol. Hepatol., 2017, 14(6), 356–365 CrossRef CAS PubMed.
- C. Sommer, M. Leinders and N. Uceyler, Inflammation in the pathophysiology of neuropathic pain, Pain, 2018, 159(3), 595–602 CrossRef CAS PubMed.
- L. Xu, Y. Liu, Y. Sun, H. Li, W. Mi and Y. Jiang, Analgesic effects of TLR4/NF-kappaB signaling pathway inhibition on chronic neuropathic pain in rats following chronic constriction injury of the sciatic nerve, Biomed. Pharmacother., 2018, 107, 526–533 CrossRef CAS PubMed.
- R. R. Ji, C. R. Donnelly and M. Nedergaard, Astrocytes in chronic pain and itch, Nat. Rev. Neurosci., 2019, 20(11), 667–685 CrossRef CAS PubMed.
- F. Lopes, F. A. Vicentini, N. L. Cluny, A. J. Mathews, B. H. Lee, W. A. Almishri, L. Griffin, W. Goncalves, V. Pinho, D. M. McKay, S. A. Hirota, M. G. Swain, Q. J. Pittman and K. A. Sharkey, Brain TNF drives post-inflammation depression-like behavior and persistent pain in experimental arthritis, Brain, Behav., Immun., 2020, 89, 224–232 CrossRef CAS PubMed.
- C. R. Donnelly, A. S. Andriessen, G. Chen, K. Wang, C. Jiang, W. Maixner and R. R. Ji, Central nervous system targets: Glial cell mechanisms in chronic pain, Neurotherapeutics, 2020, 17(3), 846–860 CrossRef PubMed.
- V. Singh and S. Ubaid, Role of silent information regulator 1 (SIRT1) in regulating oxidative stress and inflammation, Inflammation, 2020, 43(5), 1589–1598 CrossRef CAS PubMed.
- W. Y. Abdelzaher, H. H. Mohammed, N. N. Welson, G. E. Batiha, R. S. Baty and A. M. Abdel-Aziz, Rivaroxaban modulates TLR4/Myd88/NF-Kbeta signaling pathway in a dose-dependent manner with suppression of oxidative stress and inflammation in an experimental model of depression, Front. Pharmacol., 2021, 12, 715354 CrossRef CAS PubMed.
- B. Clavo, G. Martinez-Sanchez, F. Rodriguez-Esparragon, D. Rodriguez-Abreu, S. Galvan, D. Aguiar-Bujanda, J. A. Diaz-Garrido, S. Canas, L. B. Torres-Mata, H. Fabelo, T. Tellez, N. Santana-Rodriguez, L. Fernandez-Perez and G. Marrero-Callico, Modulation by ozone therapy of oxidative stress in chemotherapy-induced peripheral neuropathy: The background for a randomized clinical trial, Int. J. Mol. Sci., 2021, 22(6), 2802 CrossRef CAS PubMed.
- R. Yehia, S. Saleh, H. El Abhar, A. S. Saad and M. Schaalan,
L-Carnosine protects against oxaliplatin-induced peripheral neuropathy in colorectal cancer patients: A perspective on targeting Nrf-2 and NF-kappaB pathways, Toxicol. Appl. Pharmacol., 2019, 365, 41–50 CrossRef CAS PubMed.
- H. Celik, S. Kucukler, S. Ozdemir, S. Comakli, C. Gur, F. M. Kandemir and A. Yardim, Lycopene protects against central and peripheral neuropathy by inhibiting oxaliplatin-induced ATF-6 pathway, apoptosis, inflammation and oxidative stress in brains and sciatic tissues of rats, Neurotoxicology, 2020, 80, 29–40 CrossRef CAS PubMed.
- K. Socala, U. Doboszewska, A. Szopa, A. Serefko, M. Wlodarczyk, A. Zielinska, E. Poleszak, J. Fichna and P. Wlaz, The role of microbiota–gut–brain axis in neuropsychiatric and neurological disorders, Pharmacol. Res., 2021, 172, 105840 CrossRef CAS PubMed.
- E. Montassier, T. Gastinne, P. Vangay, G. A. Al-Ghalith, S. Bruley des Varannes, S. Massart, P. Moreau, G. Potel, M. F. de La Cochetiere, E. Batard and D. Knights, Chemotherapy-driven dysbiosis in the intestinal microbiome, Aliment. Pharmacol. Ther., 2015, 42(5), 515–528 CrossRef CAS PubMed.
- M. P. Roberti, S. Yonekura, C. P. M. Duong, M. Picard, G. Ferrere, M. Tidjani Alou, C. Rauber, V. Iebba, C. H. K. Lehmann, L. Amon, D. Dudziak, L. Derosa, B. Routy, C. Flament, C. Richard, R. Daillere, A. Fluckiger, I. Van Seuningen, M. Chamaillard, A. Vincent, S. Kourula, P. Opolon, P. Ly, E. Pizzato, S. Becharef, J. Paillet, C. Klein, F. Marliot, F. Pietrantonio, S. Benoist, J. Y. Scoazec, P. Dartigues, A. Hollebecque, D. Malka, F. Pages, J. Galon, I. Gomperts Boneca, P. Lepage, B. Ryffel, D. Raoult, A. Eggermont, T. Vanden Berghe, F. Ghiringhelli, P. Vandenabeele, G. Kroemer and L. Zitvogel, Chemotherapy-induced ileal crypt apoptosis and the ileal microbiome shape immunosurveillance and prognosis of proximal colon cancer, Nat. Med., 2020, 26(6), 919–931 CrossRef CAS PubMed.
- N. Lian, M. Shen, K. Zhang, J. Pan, Y. Jiang, Y. Yu and Y. Yu, Drinking hydrogen-rich water alleviates chemotherapy-induced neuropathic pain through the regulation of gut microbiota, J. Pain Res, 2021, 14, 681–691 CrossRef PubMed.
- Y. Qu, X. Li, F. Xu, S. Zhao, X. Wu, Y. Wang and J. Xie, Kaempferol alleviates murine experimental colitis by restoring gut microbiota and inhibiting the LPS-TLR4-NF-kappaB axis, Front. Immunol., 2021, 12, 679897 CrossRef CAS PubMed.
- K. Li, Y. Tang, J. P. Fawcett, J. Gu and D. Zhong, Characterization of the pharmacokinetics of dioscin in rat, Steroids, 2005, 70(8), 525–530 CrossRef CAS PubMed.
- X. Wang, J. Y. Yu, Y. Sun, H. Wang, H. Shan and S. Wang, Baicalin protects LPS-induced blood–brain barrier damage and activates Nrf2-mediated antioxidant stress pathway, Int. Immunopharmacol., 2021, 96, 107725 CrossRef CAS PubMed.
|
This journal is © The Royal Society of Chemistry 2023 |
Click here to see how this site uses Cookies. View our privacy policy here.