DOI:
10.1039/D2FO02788G
(Paper)
Food Funct., 2023,
14, 399-412
Protective effects of Lycium barbarum L. berry extracts against oxidative stress-induced damage of the retina of aging mouse and ARPE-19 cells†
Received
20th September 2022
, Accepted 24th November 2022
First published on 24th November 2022
Abstract
In this study, we investigated the preventive effect of Lycium barbarum L. berry extract on age-related macular degeneration (AMD) and the main components responsible for its antioxidant activity. An AMD mouse model was developed by feeding 18-month-old mice with a 1% hydroquinone diet. Meanwhile, the model mice were treated with water extract (LBW) and alcohol extract (LBE) of L. barbarum berries respectively for 3 months. It was found that the retinal structural abnormalities were improved and the oxidation stress and inflammatory imbalance were both attenuated in model mice treated with the extracts of L. barbarum berries. According to the metabolomics analysis of the serum of model mice, LBW regulated the metabolism of unsaturated fatty acids and sphingolipids, while LBE extracts tended to regulate taurine metabolism. On sodium iodate induced oxidative injury of ARPE-19 cells, water extracts of L. barbarum berries eluted with 95% ethanol (LBW-95E) on AB-8 macroporous resin significantly improved the cell viability and attenuated oxidative stress by increasing the superoxide dismutase (SOD) activity and glutathione (GSH) content, decreasing the reactive oxygen species (ROS) content, promoting the entry of nuclear factor erythroid-derived 2-like 2 (Nrf2) into the nucleus and up-regulating the heme oxygenase-1 (HO-1) expression. Scopoletin, N-trans-feruloyltyramine and perlolyrine were identified as the main components of LBW-95E. These results demonstrated that L. barbarum berry extracts protected the retina of aging AMD model mice from degeneration and LBW-95E was the vital antioxidant activity fraction of LBW. These findings suggest that L. barbarum berry extracts might be an excellent natural source for the development of retinal protection-related drugs or dietary supplements.
1. Introduction
Age-related macular degeneration (AMD) is a disease that causes progressive vision loss in the elderly, primarily in the macular region of the fovea centralis. It is the leading cause of irreversible visual impairment in the elderly worldwide, and it can lead to permanent vision impairment or blindness, which seriously affects patients’ quality of life. Early AMD affects 9.5% while late AMD affects 1.0% of those over 50 in China's industrialized cities.1 With the acceleration of the aging society process, the number of persons affected by AMD is predicted to rise to 288 million by 2040, which imposes a heavy burden on both individuals and society.2 Depending on the disease process, AMD shows two classic clinical phenotypes in the late stages: dry AMD (atrophic) and wet AMD (neovascular). For wet AMD, the therapies targeting anti-vascular endothelial growth factor (VEGF) have been approved and widely used.3 However, some patients are resistant to anti-VEGF therapy.4 Meanwhile, no clinically authorized medications for the treatment of dry AMD have been developed and approved. Therefore, there is an urgent need for preventing the progression of AMD to advanced stages and exploring potential future therapeutic opportunities.
According to the results of epidemiological investigation, aging is the key risk factor for AMD, followed by smoking and poor antioxidant dietary habits.5–7 In addition to susceptibility genes, the dysfunction of retinal pigment epithelial cells caused by oxidative stress and chronic inflammatory response is considered to be the core pathological link of AMD.8,9 Cigarette smoke is high in pro-oxidants and hydroquinone is the main component in it.10 Previous studies have shown that hydroquinone exposure causes oxidative stress and lipid accumulation in the retina of mice and leads to the death of retinal pigment epithelium (RPE) cells.11,12 It was also found that most of the aged mice without feeding the hydroquinone diet had normal retinal structures, whereas abnormal retinal structures were found in aged mice exposed to the hydroquinone diet.13 Considering that aging alone may not be sufficient to induce the typical AMD phenotype, an 18-month-old aging mouse fed with a 1% hydroquinone diet was applied to develop an AMD mouse model.
L. barbarum berry is the dried ripe fruit of the Solanaceae plant L. barbarum L. which is applied for nourishing the liver and kidney and improving eyesight in Chinese medicine. Modern research also proved that L. barbarum berry is a natural preventive agent for improving retinal diseases with prominent antioxidant, anti-inflammatory, and neuroprotective function.14 Human trials support that daily supplements of L. barbarum berry increase macular pigment and delay the progress of AMD in healthy middle-aged persons.15–17In vitro studies indicate that L. barbarum berry protects ARPE-19 cells from oxidative stress-induced apoptosis.18 These findings indicate that L. barbarum berry is beneficial for AMD. We wanted to reveal the material basis and possible mechanism of L. barbarum berry to improve AMD and promote the in-depth development of products related to L. barbarum berry for delaying AMD, so as to serve the needs of vision protection in the aging population. In the current study, we treated the aging mice with hydroquinone to develop an AMD animal model and evaluated the protective effect of L. barbarum berry on retinal injury. Furthermore, sodium iodate-induced oxidative injured ARPE-19 cells were applied for exploring the material basis and elucidating the possible mechanism.
2. Materials and methods
2.1. Lycii Fructus extracts preparation
L. barbarum berries were purchased from Zhongning Co. Ltd, Ningxia and identified as the dried mature fruit of Lycium barbarum L. by Professor Jin-ao Duan of the Nanjing University of Chinese Medicine. Hydroquinone was purchased from the Shanghai Macklin Biochemical Co., Ltd (Shanghai, China).
2 kg of L. barbarum berries were extracted by refluxing with water (1
:
6, w/v) for 2 h. The extracts were filtered with three layers of gauze to separate the supernatant from the residue. Another 2 kg of L. barbarum berry was refluxed with 80% ethanol (1
:
6, w/v) for 2 h and filtered as before. The supernatants were concentrated under reduced pressure and then freeze-dried respectively afterward. The yields of the water extract (LBW) and ethanol extract (LBE) are 36.8% and 5.8%, respectively. The extracts were stored in a refrigerator at −20 °C.
Lycium barbarum polysaccharides (LBP) were prepared by adding ethanol to LBW and left for 24 h at 4 °C. The concentration of ethanol was set at 70% finally. For the preparation of other fractions, LBW was loaded on the AB-8 macroporous resin (Tianjin, China) and eluted with pure water (LBW-W), 30% ethanol (LBW-30E), 70% ethanol (LBW-70E) and 95% ethanol (LBW-95E) sequentially until the eluent was colorless. Those effluents were concentrated under reduced pressure and then freeze-dried respectively.
2.2. UHPLC-QTOF-MS/MS analysis
Chemical analysis of L. barbarum berry extracts was performed on a Waters system (Waters, 2695) consisting of a binary pump, a quadruple time of flight mass detector (QTOF-MS/MS), an auto-sampler, and a thermostated column compartment. Samples were analyzed by using an Acquity ultra high performance liquid chromatography (UHPLC) BEH C18 column (2.1 mm × 100 mm, 1.7 m) with 0.1% formic acid aqueous solution (A)–acetonitrile (B) as the mobile phase. The gradient elution conditions for the analysis of LBW and LBE were set as: 0–8 minutes: 5%–10% B; 8–15 minutes: 10%–15% B; 15–18 minutes: 15%–60% B; 18–20 minutes: 40%–100% B, 20–21 minutes: 100%–5% B; 21–23 minutes: 5% B. The gradient elution conditions for the analysis of LBW-95E were set as: 0–2 minutes: 5%–10% B; 2–10 minutes: 10%–30% B; 10–18 minutes: 30%–35% B; 18–22 minutes: 35%–50% B, 22–28 minutes: 50%–70% B, 28–32 minutes: 70%–85% B, 32–35 minutes: 85%–95% B, 35–40 minutes: 100% B. The column temperature was set as 35 °C. The flow rate was set as 0.4 mL·min−1 and the injection volume was 4 μL. The mass scan range was 100 m/z to 1000 m/z.
2.3. Animals and groups
Male-specific pathogen-free (SPF) grade C57BL/6J mice 12 months old were provided by the animal center of Nanjing University of Chinese Medicine. The animals were raised in an SPF environment of 12 h light/dark cycle, 22–25 °C, and humidity of 40%–70% in the animal center of Nanjing University of Chinese medicine. All procedures for treating animals followed the guide for the care and use of laboratory animals approved by the institutional animal care and use committee. The experimental procedures also conformed to the guidelines of the “Principles of Laboratory Animal Care” (NIH Publication No. 80-23, revised 1996). In particular, all procedures were designed to minimize the number of experimental animals and possible injuries.
Animals were raised normally until 18 months of age. The model group mice were then given 1% hydroquinone in drinking water. In the treatment groups, the model mice were treated with the L. barbarum berry ethanol extract group (LBE) at a dosage of 173 mg kg−1 day−1 and the L. barbarum berry water extract group (LBW) at a dosage of 1105 mg kg−1 day−1 for 3 months, respectively. Meanwhile, C57BL/6J mice 3 months old were arranged as the young control group. After the last treatment, the animals were anesthetized with isoflurane (R500IP·RWD, Shenzhen, China), followed by cervical dislocation. Eyeballs and blood samples were collected for the analyses described below.
2.4. Histopathology analysis
The eyeballs of mice were fixed, dehydrated and coated with paraffin, and then cut into 5 μm slices with a microslicer. Retinal slices were dewaxed and stained with hematoxylin–eosin. The central area of the retina was photographed with an optical microscope (Olympus BX43, Japan), and the thickness of the outer nuclear layer (ONL), the inner nuclear layer (INL), the photoreceptor layer (PL) and the whole retina (WR) were measured using NIH Image J software according to the previously published methods.19
2.5. Determination of the oxidative index and pro-inflammation cytokines
The mouse retina was separated under a stereomicroscope and crushed using an ultrasonic cell crusher (Zentz, Ningbo, China) for 90 s. Afterward, the samples were centrifuged at 4 °C for 5 min with a speed of 1000g per min and the supernatant was collected. The expressions of superoxide dismutase (SOD), glutathione (GSH) and malondialdehyde (MDA) were measured using the kits (Jiancheng Institute of Bioengineering, China). The levels of interleukin-6 (IL-6), interleukin-1β (IL-1β) and tumor necrosis factor alpha (TNF-α) were detected by the relevant kit produced by Nanjing Jinyibai Biotechnology Co., Ltd.
ARPE-19 cells were seeded at a density of 5 × 105 cells per well into 6-well plates and incubated for 24 hours. Only DMSO was used to treat the control cells (final concentration of 0.1%). ARPE-19 cells were treated with sodium iodate 6 mM for 24 hours in the sample treatment group. LBW-95E at final concentrations of 1 μg mL−1, 3 μg mL−1, and 10 μg mL−1 was added together with 10 μM DCFH-DA for 8 h. The cells were collected and the intracellular fluorescence intensity was observed under FITC fluorescence microscopy conditions. Other cells were lysed by ultrasonic lysis in an ice-water bath and centrifuged at 4 °C, 4000 rpm for 10 min to remove the insoluble material and debris, and the SOD (Catalogue: A001-3-2), GSH (Catalogue: A006-2-1), and MDA (Catalogue: A003-2-2) levels in plasma were measured using the kits (Jiancheng Institute of Bioengineering, China).
2.6. Identification of biomarkers and construction of metabolic pathways
The metabolomics analysis experimental procedure could be referred from our previously published literature.20 The details of the UHPLC apparatus were the same as mentioned above. The analysis was performed on an Acquity UHPLC BEH C18 column (2.1 mm × 100 mm, 1.7 μm) with the mobile phase composed of 0.1% formic acid aqueous solution (A)–acetonitrile (B) and the elution gradient was set as: 0–3 minutes: 5%–70% B; 3–12 minutes: 70%–82% B; 12–13 minutes: 82%–5% B; 13–14 minutes: 5%–5% B, column temperature 35 °C, flow rate 0.4 mL min−1, and the injection volume was 2 μL.
For chemical and metabolomic analysis, the electrospray ion (ESI) source was employed and the mass scan range was 100 m/z to 1000 m/z both in positive and negative ionization modes; the capillary voltage was 3.0 kV; the cone hole voltage was 40 V; the extraction voltage was 4.0 V; the ion source temperature was 120 °C; the dissolvent temperature was 400 °C and the ion source temperature was 120 °C; the cone hole gas flow rate was 50 L per hour; the dissolvent gas flow rate was 800 L per hour; the collision energy was 630 eV and the ion energy was 6 volts. The leucine–enkephalin solution was employed for the adjustment of the ion mass.
The data were processed by Waters MassLynx 4.2 software (Waters Co.) and analyzed by MetaboAnalyst 5.0 platform (https://www.metaboanaly.ca/). After the Pareto normalization of data, the model group and the blank group were then subjected to independent sample t-tests and partial least-squares discrimination analysis (PLS-DA). Potential differential variables were screened with P < 0.05 and variable importance projection (VIP) >1 as conditions. Differential metabolic fragments were input into HMDB (Human Metabolome Database, https://www.hmdb.ca/) and Pubchem (https://www.pubchem.ncbi.nlm.nih.gov) databases to obtain differential metabolites. MetaboAnalyst 5.0 and KEGG databases (https://www.kegg.jp/) were used to identify metabolic pathways.
2.7. Determination of cell viability
ARPE-19 cells were seeded at a density of 5000 cells per well into 96-well plates and incubated for 24 hours. Only dimethyl sulfoxide was used to treat the control cells (final concentration of 0.1%). The model group cells were treated with sodium iodate (6 mM). In the treatment groups, ARPE-19 cells were co-treated with sodium iodate (6 mM) and different elution fractions (LBW, LBE, LBP, LBW-W, LBW-30E, LBW-70E and LBW-95E) for 24 hours. For each treatment, three dosages (1 μg mL−1, 3 μg mL−1, and 10 μg mL−1) of extracts were applied. The cell viability of ARPE-19 cells was determined by treating cells with 3-(4,5-dimethylthiazol-2-yl)-2,5-diphenyltetrazolium bromide (0.5 mg mL−1) for 3 hours and the residues were dissolved in dimethyl sulfoxide. The absorbance of the solvent was detected at 570 nm.
2.8. Western blotting analysis
APRE-19 cells were scraped from the bottom of the culture plate and the nuclear and cytoplasmic components of the cells were prepared using the nuclear extraction kit (BeyotimeBiotech, China) according to the manufacturer's instructions. The protein concentration of the samples was determined by the BCA method. 10 μg of protein from cell lysates were separated on a 10% SDS-PAGE gel and transferred electrophoretically to the polyvinylidene fluoride membrane for 1 hour. The membranes were blocked with 5 percent (w/v) non-fat dry milk in TBST (20 mM Tris–HCl, 150 mM NaCl, and 0.05% Tween 20; pH 7.5) buffer for 1 hour (25 °C) and then incubated overnight (4 °C) with primary antibodies of anti-Nrf2 (1
:
1000, catalogue: 12721T, CST, USA), anti-HO-1 (1
:
1000, catalogue: 00101747, Proteintech, China), anti-GAPDH (1
:
1000, catalogue: I0008047, Proteintech, China), anti-Lamin B1 (1
:
1000, catalogue: 13435S, CST, USA), anti-α-tubulin (1
:
1000, catalogue: 5568S, CST, USA), and then co-incubated with HRP-labeled secondary antibodies (catalogue: BA1054, Boster, China). The relative band density of each sample was determined with the enhanced chemiluminescence (ECL) western blotting substrate kit (Tanon, China) on the Bio-Rad Imaging System (Hercules, California). The semi-quantification of the expression of each target protein was performed using Image lab 4.0 software (Bio-Rad, America).
2.9. Statistical analysis
All data were statistically analysed by one-way ANOVA (version 13.0, SPSS, IBM Corp., Armonk, NY), and then post-Bonferroni analysis was performed under appropriate conditions. The normal distribution test was carried out before the one-way ANOVA analysis. The results were expressed by the mean ± standard deviation of the mean value, where n = 3–8. A P-value represented a statistically significant change. Specifically, P < 0.05 was statistically significant, and P < 0.01 was highly statistically significant.
3. Results
3.1. Phytochemical composition of L. barbarum extracts
The main chemical composition of L. barbarum extracts was analyzed by UHPLC-Q-TOF-MS/MS (Fig. 1). 18 compounds were identified and are listed in Table 1. These compounds were classified as follows: (1) dicaffeoylspermine derivatives,21,22 including the Glu-kukoamine isomer, Kukoamine B isomer, Lycibarbarspermidine F isomer, Lycibarbarspermidine J isomer, N1–N10 dihydrocaffeoyl spermidine, and N1-caffeoyl, N3-dihydrocaffeoyl spermidine; (2) amide alkaloids,23,24 including Lyciumamide M, N-trans-feruloyltyramine; (3) coumarin,22 including scopoletin; (4) phenolic acids,21 including ethyl isoferulate; (5) flavonoids, including rutin. An integrated peak area was used for the relative quantification of each chemical. The results showed that the amounts of compounds in LBW extracts were relatively abundant containing a large number of dicaffeylspermidine derivatives, while the LBE extracts were mainly composed of flavonoids, amide alkaloids and phenolic amides. LBW-95E extracts mainly contained scopoletin, N-trans-feruloyltyramine and perlolyrine.
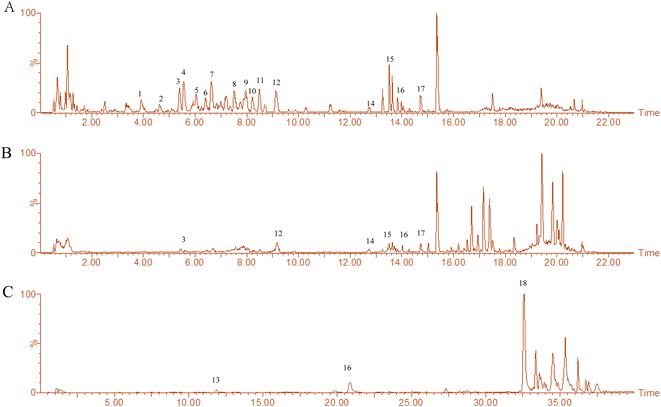 |
| Fig. 1 Chemical fingerprint chromatograms of the extracts of L. barbarum berries. (A) Fingerprint chromatograms of LBW were made by the UHPLC-QTOF-MS/MS method in positive ion mode. (B) Fingerprint chromatograms of LBE were made by the UHPLC-QTOF-MS/MS method in positive ion mode. (C) Fingerprint chromatograms of LBW-95E were made by the UHPLC-QTOF-MS/MS method in negative ion mode. | |
Table 1 Compounds identified from LBW, LBE and LBW-95E of L. barbarum by UPLC-Q-TOF-MS/MS
No. |
RT (min) |
Type |
Compound identity |
Formula |
Molecular weight |
Relative content % |
Comes from |
1 |
3.95 |
[M + H]+ |
Glu-kukoamine isomer |
C34H52O11N4 |
692.79 |
2.09, 0.61 |
LBW |
2 |
4.62 |
[M + H]+ |
Kukoamine B isomer |
C28H42O6N4 |
530.65 |
0.47, 0.27 |
LBW |
3 |
5.40 |
[M + H]+ |
Lycibarbarspermidine F isomer |
C37H53O16N3 |
795.83 |
0.53, 0.32 |
LBW, LBE |
4 |
5.56 |
[M + H]+ |
Lycibarbarspermidine M isomer |
C37H55O16N3 |
797.84 |
0.32 |
LBW |
5 |
6.04 |
[M + H]+ |
Lycibarbarspermidine F isomer |
C37H53O16N3 |
795.83 |
0.31 |
LBW |
6 |
6.41 |
[M + H]+ |
Lycibarbarspermidine B isomer |
C31H43O11N3 |
633.69 |
0.40 |
LBW |
7 |
6.62 |
[M + H]+ |
Lycibarbarspermidine J isomer |
C31H45O11N3 |
635.70 |
0.40, 0.34 |
LBW |
8 |
7.52 |
[M + H]+ |
Lycibarbarspermidine B isomer |
C31H43O11N3 |
633.69 |
0.22 |
LBW |
9 |
7.96 |
[M + H]+ |
N1–N10 dihydrocaffeoyl spermidine |
C25H35O6N3 |
473.56 |
0.55 |
LBW |
10 |
8.22 |
[M + H]+ |
Lycibarbarspermidine B isomer |
C31H43O11N3 |
633.68 |
1.02 |
LBW |
11 |
8.48 |
[M + H]+ |
Lycibarbarspermidine F isomer |
C37H53O16N3 |
795.83 |
0.27 |
LBW |
12 |
9.12 |
[M + H]+ |
N1-caffeoyl, N3-dihydrocaffeoyl spermidine |
C25H33O6N3 |
471.54 |
0.34, 0.36 |
LBW, LBE |
13 |
11.90 |
[M − H]− |
Scopoletin |
C10H8O4 |
192.16 |
2.39 |
LBW-95E |
14 |
12.75 |
[M + H]+ |
Rutin |
C27H30O16 |
610.51 |
0.21, 0.61 |
LBW, LBE |
15 |
13.52 |
[M + H]+ |
Lyciumamide M |
C37H40N2O9 |
656.72 |
0.21, 0.37 |
LBW, LBE |
16 |
14.03 |
[M + H]+ |
N-trans-Feruloyltyramine |
C18H19NO4 |
313.34 |
0.21, 0.38, 2.11 |
LBW, LBE, LBW-95E |
17 |
14.74 |
[M + H]+ |
Ethyl trans-ferulate |
C12H14O4 |
222.23 |
0.02, 0.06 |
LBW, LBE |
18 |
32.56 |
[M − H]− |
Perlolyrine |
C16H12N2O2 |
264.27 |
0.18 |
LBW-95E |
3.2.
L. barbarum berry extracts ameliorate retinal degeneration in aging mice
In the retina of hydroquinone-fed aging model mice, the thickness of the whole retina, outer nuclear layer and inner nuclear layer was significantly thinner than that of the young control group mice. Treatment of the water extracts of L. barbarum berries obviously improved the thickness of the whole retina (P < 0.001), outer nuclear layer (P < 0.01), and inner nuclear layer (P < 0.01) layer, while the alcohol extract of L. barbarum berries only improved the thickness of the inner nuclear layer (P < 0.01) (Fig. 2).
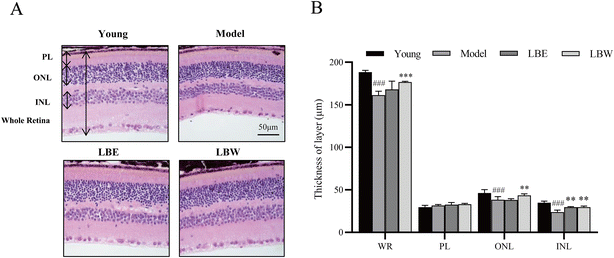 |
| Fig. 2
L. barbarum berry extracts protected retinal degeneration in hydroquinone-fed aging mice. (A) Representative retinal images stained with H&E. Scale bar = 50 μm. (B) The thickness of WR (whole retina), ONL (outer nuclear layer), INL (inner nuclear layer) and PL (plexiform layer) was measured at 5 different locations. The results are shown as mean ± SD (n = 5). ###P < 0.001 vs. the young control group, **P < 0.01; ***P < 0.001 vs. the model group. | |
3.3.
L. barbarum berry extracts exert the antioxidant effect in hydroquinone-fed aging mice
In the serum of hydroquinone-fed aging model mice, the activity of SOD and the content of GSH were significantly decreased (SOD: P < 0.001; GSH: P < 0.05), and the content of MDA was significantly increased compared with those of the control mice (P < 0.05). Treatment with LBE and LBW both significantly increased the SOD activity and GSH content compared with untreated model mice (SOD: P < 0.001; GSH: P < 0.05). No significant difference was found in the MDA content between the model mice treated with LBE and LBW or no drug treatment (Fig. 3).
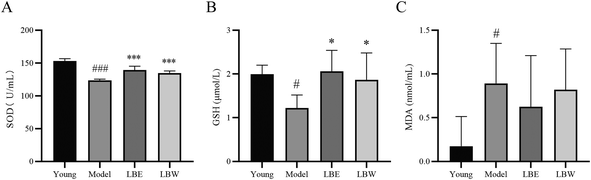 |
| Fig. 3 Effect of L. barbarum berry extracts on the oxidative stress in serum in hydroquinone-fed aging mice. (A) SOD activity in different groups. (B) GSH levels in different groups. (C) MDA levels in different groups. The results are shown as the mean ± SD (n = 8). #P < 0.05, ###P < 0.001 vs. the young control group, *P < 0.05, **P < 0.01; ***P < 0.001 vs. the model group. | |
3.4.
L. barbarum berry extracts decreased the levels of IL-6, IL-1β and TNF-α in the retina and serum of aging mice
As shown in Fig. 4, the levels of IL-6 and IL-1β were significantly upregulated in the serum of aging model mice compared with the young group mice (P < 0.001, P < 0.05). Treatment with LBW and LBE significantly reduced the IL-6 levels in the serum of aging model mice (P < 0.001) while exerting no significant effect on IL-1β levels. LBE treatment also decreased the TNF-α levels in the serum of the aging model mice (P < 0.05), while LBW exerted no obvious effect. Besides, the levels of pro-inflammation cytokines were also determined in the retina of the mice. The expressions of IL-6 and IL-1β were considerably higher compared with the youth mice (P < 0.001) and treatment with LBW or LBE decreased the expressions of these two pro-inflammation cytokines (P < 0.05, P < 0.01). LBE treatment also decreased the expression of TNF-α in the retina of the aging model mice (P < 0.05) (Fig. 4), while LBW treatment showed no obvious effect, which was the same as that in serum.
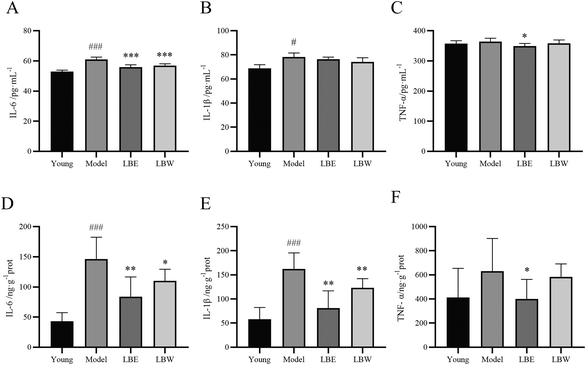 |
| Fig. 4 Effect of L. barbarum berry extracts on inflammatory factors in the serum and retina in hydroquinone-fed aging mice. (A) IL-6 levels in serum. (B) IL-1β levels in serum. (C) TNF-α levels in serum. (D) IL-6 levels in the retina. (E) IL-1β levels in the retina. (F) TNF-α levels in the retina. The results are shown as the mean ± SD (n = 8). #P < 0.05, ###P < 0.001 vs. the young control group, *P < 0.05, **P < 0.01; ***P < 0.001 vs. the model group. | |
3.5.
L. barbarum berry extracts altered serum metabolite profiles in AMD mice
The peak intensity data generated by UHPLC-QTOF-MS were normalized by Pareto scaling before PLS-DA and OPLS-DA analysis. The PLS-DA score plots showed significant clustering in the plasma samples of the aging model mice and the young mice in both positive and negative ion modes (Fig. 5A and B), indicating a change in serum metabolic profiles in the aging model group. Subsequently, differential metabolites were obtained from the volcano map with the corrected P < 0.05 and VIP > 1 (Fig. 5C and D). The differential metabolites were identified based on the UHPLC-MS/MS data. A total of 13 metabolites were identified between the aging model mice and the young mice, including phosphatidylcholine, phosphatidylethanolamine, docosahexaenoic acid, taurine, sphingosine phosphate, cholesterol sulfate, and other metabolites (Table 2).
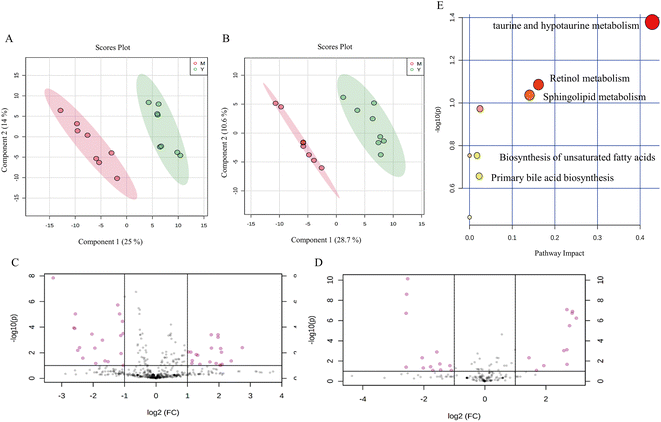 |
| Fig. 5 Characteristic of the serum metabolite profile in hydroquinone-fed aging mice. (A and B) The PLS-DA score plots between the young group and hydroquinone-fed aging mouse group in serum metabolites (A: ESI+; B: ESI−). M represents the model group; Y represents the young group. (C and D) Volcano map of serum metabolites between the young control group and hydroquinone-fed aging mouse group. The red dot means the metabolites’ absolute value of P < 0.05 between the control and model groups (C: ESI+; D: ESI−). (E) Metabolic pathway changes of serum between the young control group and hydroquinone-fed aging mouse group. | |
Table 2 Differential plasma metabolite identification between the model group and the control group
No. |
Metabolite |
RT (time) |
m/z |
Ion |
VIP |
HMDB ID |
M/C |
1 |
LysoPC (20:5(5Z,8Z,11Z,14Z,17Z)) |
3.68 |
542.324 |
+ |
1.74 |
0010397 |
↑ |
2 |
LysoPC (20:4(5Z,8Z,11Z,14Z)) |
4.02 |
544.3396 |
+ |
6.59 |
0010395 |
↑ |
3 |
LysoPC (20:3(8Z,11Z,14Z)) |
4.34 |
546.3556 |
+ |
2.38 |
0010394 |
↑ |
4 |
LysoPC (20:2(11Z,14Z)) |
4.99 |
548.3711 |
+ |
1.28 |
0010392 |
↑ |
5 |
LysoPE (18:0/0:0) |
4.77 |
482.3599 |
+ |
1.25 |
0011130 |
↓ |
6 |
Tetracosahexaenoic acid |
0.65 |
357.0295 |
− |
1.84 |
0000226 |
↓ |
7 |
Taurine |
0.61 |
124.0085 |
− |
1.15 |
0000251 |
↓ |
8 |
Sphingosine 1-phosphate |
3.66 |
378.2397 |
− |
1.22 |
0000277 |
↑ |
9 |
Cholesterol sulfate |
4.01 |
501.2813 |
− |
1.90 |
0000653 |
↑ |
10 |
Docosahexaenoic acid |
7.26 |
327.2299 |
− |
6.12 |
0002183 |
↓ |
11 |
Retinyl ester |
4.49 |
301.2154 |
− |
1.44 |
0003598 |
↑ |
12 |
Cholestane-3,7,12,25-tetrol-3-glucuronide |
4.98 |
593.3651 |
− |
1.11 |
0010355 |
↑ |
13 |
1-Pentadecanecarboxylic acid |
10.18 |
255.2304 |
− |
2.97 |
0000220 |
↓ |
According to the OPLS-DA analysis, plasma samples were randomly divided into four groups: the young control group, the aging model group, the LBW group, and the LBE group. When compared to the aging model group, the serum metabolic profiles of LBW and LBE converge towards the young control group (Fig. 6A and B). According to 100 randomized permutation experiments on the OPLS-DA model, the results showed that R2 = 0.581 and Q2 = −0.556 in positive ion mode and R2 = 0.981 and Q2 = −0.263 in negative ion mode. R2 was larger than 0.5 in both positive and negative ion modes, and Q2 was negative (Fig. 6C and D), which suggests that the established OPLS-DA model was reliable.
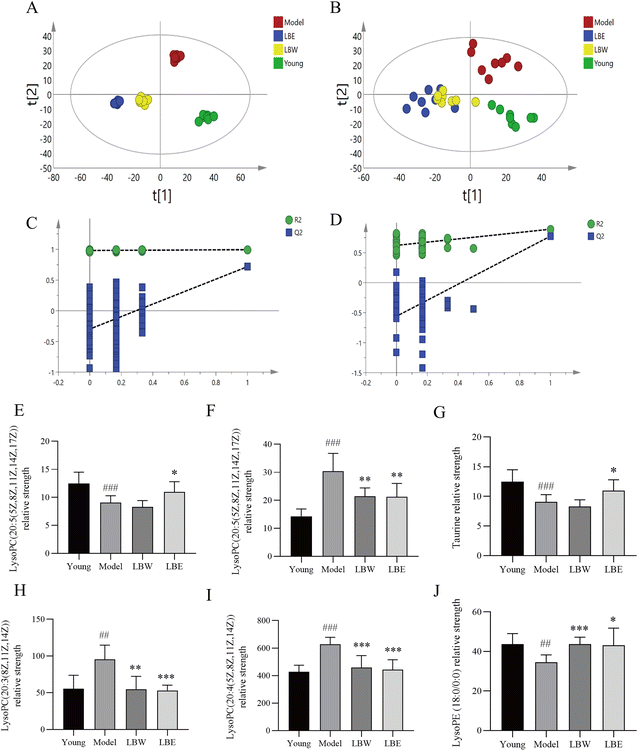 |
| Fig. 6 Effect of L. barbarum berry extracts on the serum metabolite profile in hydroquinone-fed aging mice. (A and B) The OPLS-DA score plots distribution for young, model, LBW and LBE groups in serum samples (A: ESI+; B: ESI−). (C and D) Permutation test charts for the OPLS-DA model (C: ESI+; D: ESI−). (E–J) L. barbarum berry extracts regulated differential metabolites in the serum of hydroquinone-fed aging mice. The results are shown as the mean ± SD (n = 8). #P < 0.05, #P < 0.01, ###P < 0.001 vs. young, *P < 0.05, **P < 0.01; ***P < 0.001 vs. model. | |
To explore the possible pathways influenced by the L. barbarum berry extracts, the metabolites identified in plasma were analyzed using the MetaboAnalyst database to enrich metabolic pathways (Fig. 5E). Compared with the young control group, taurine in taurine and hypotaurine metabolism and docosahexaenoic acid related to the biosynthesis of unsaturated fatty acids in the model group decreased significantly. However, sphingosine 1-phosphate and lysoPC related to sphingolipid metabolism, cholesterol sulfate in primary bile acid biosynthesis and retinyl ester in retinol metabolism increased significantly. Compared with the model group, some metabolites in the LBW group were significantly reversed, including docosahexaenoic acid, lysoPE (18:0) and lysoPE (20:5). Meanwhile, taurine, lysoPE (18:0), and lysoPE (20:5) in the LBE group were significantly reversed (Fig. 6E–J). The enrichment pathways of these differential metabolites mainly included biosynthesis of unsaturated fatty acids, sphingolipid metabolism, and taurine and hypotaurine metabolism, suggesting that L. barbarum berries affect the above pathways and alleviate serum metabolism disorders.
3.6.
L. barbarum berry extracts improve ARPE-19 cell viability
As mentioned above, L. barbarum berry extracts significantly ameliorated the degeneration of the retina of aging mice. It is known that oxidation-induced loss of RPE cells is the core pathology of AMD. Therefore, the ARPE-19 cell was treated with sodium iodate to induce cell injury and the antioxidant effect of the components of L. barbarum berry extracts was evaluated. As shown in Fig. 7A, sodium iodate dramatically reduced the ARPE-19 cell viability, while the treatment with LBW-70E and LBW-95E increased the cell viability compared with the no drug-treated cells (P < 0.05, P < 0.01, P < 0.001). Among these components, LBW-95E exerted the most potent effect in protecting ARPE-19 cells from sodium iodate-induced oxidative stress.
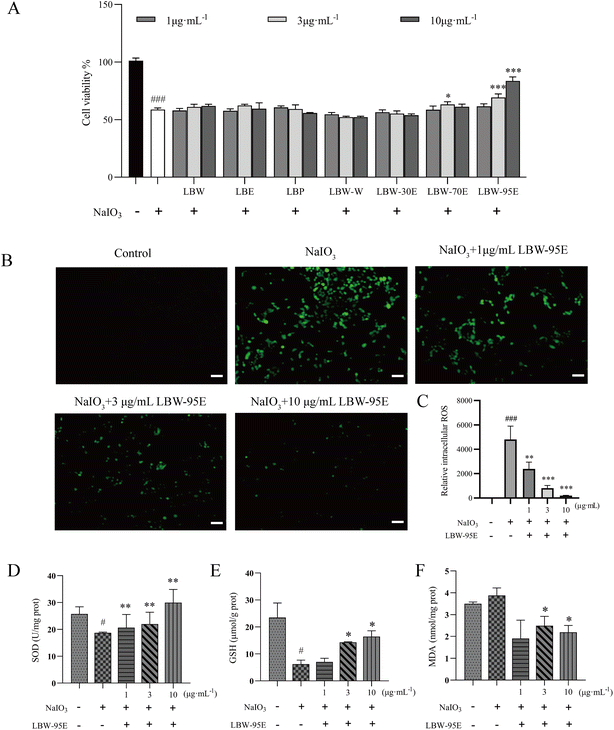 |
| Fig. 7 Protective effect of LBW-95E on sodium iodate induced oxidative stress in ARPE-19 cells. (A) Effect of L. barbarum berry extracts on the viability of ARPE-19 cells induced by sodium iodate. (B) LBW-95E decreased sodium iodate-induced intracellular reactive oxygen species levels in ARPE-19 cells. Scale bar = 200 μm. Effect of LBW-95E on SOD (C), GSH (D) and MDA (E) in ARPE-19 cells induced by sodium iodate. Data are presented as the mean ± SD for each group (n = 3). #P < 0.05, ###P < 0.001 compared with the control group, *P < 0.01, **P < 0.01, ***P < 0.001 compared with the NaIO3 group. | |
3.7. LBW-95E activates the Nrf2/HO-1 pathway and boosts the antioxidant system in ARPE-19 cells
To further elucidate the action mechanism of LBW-95E in protecting APRE-19 cells from sodium iodate-induced damage, the changes in the oxidative stress indexes of LBW-95E treated APRE-19 cells were determined. As displayed in Fig. 7B–F, sodium iodate treatment significantly increased the intracellular reactive oxygen species (ROS) levels of ARPE-19 cells (P < 0.001) while decreasing the SOD activity and GSH levels (P < 0.05). The elevated levels of ROS and reduced GSH levels and SOD activity were reversed by LBE-95E treatment in a dose-dependent manner (P < 0.001, P < 0.01, P < 0.05). As a key transcription factor regulating the antioxidant effect, nuclear factor erythroid-derived 2-like 2 (Nrf2) plays an important role in initiating the antioxidant response. Under normoxic conditions, Nrf2 binds to Kelch-like ECH-associated protein 1 (Keap1) and degrades with ubiquitination in the proteasome. When cells experience oxidative stress, Nrf2 is released from the Keap1 binding site and rapidly transferred to the nucleus, resulting in increased expression of antioxidant genes and enzymes such as heme oxygenase 1 (HO-1), superoxide dismutase (SOD), and others.25 As displayed in Fig. 8, treatment with LBW-95E significantly increased the expression of Nrf2 in the nucleus of sodium iodate-treated APRE-19 cells while exerting no obvious effect on the expression of Nrf2 in the cytoplasm, which indicated that LBW-95E stimulated the Nrf2 translocation. In line with this effect, the up-regulated expression of HO-1 was also observed (P < 0.01, P < 0.05). These data implied that LBW-95E possessed obvious antioxidant capacity and the regulation of the Nrf2/HO-1 signalling pathway might contribute to this effect.
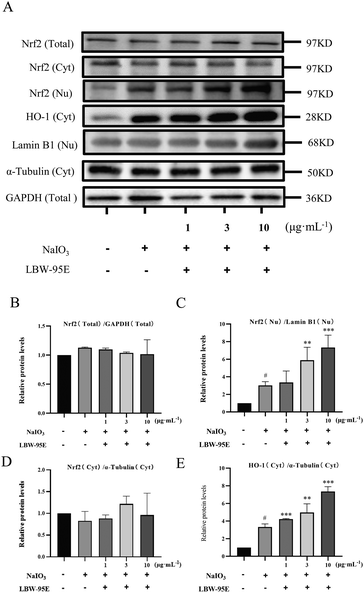 |
| Fig. 8 Effect of LBW-95E on Nrf2 and HO-1 protein expression in ARPE-19 cells induced by sodium iodate. (A) Protein bands. (B) Total Nrf2 expression. (C) Cytoplasmic Nrf2 expression. (D) Nuclear Nrf2 expression. (E) HO-1 expression. The protein levels were quantified by the band density. Data are presented as the mean ± SD for each group (n = 3). #P < 0.05 compared with the control group, **P < 0.01, ***P < 0.001 compared with the NaIO3 group. | |
4. Discussion
Since no satisfactory treatment was developed for advanced AMD patients, the delay of progression of AMD and the preservation of the residual vision have become important problems. L. barbarum berry, a traditional Chinese herb for eyesight improvement, has been validated to possess various health benefits, such as antioxidant,26 anti-aging,27 anti-inflammatory,28 and immune regulation activities.28 Studies have shown that L. barbarum berries have a protective effect on retinal ganglion cell damage in rats induced by chronic intraocular hypertension and oxidative stress-induced retinal ganglion cell damage.29,30 However, there are few reports about the concrete chemical compound of L. barbarum berry on improving AMD. In the current study, the regulatory effects of LBW and LBE on the retina of AMD mice were investigated, and the antioxidant active components in LBW were screened by in vitro cell experiments; at the same time, the phytochemical components of the L. barbarum berry extracts were analyzed by UHPLC-Q/TOF-MS/MS, in order to lay a foundation for the research of its biological mechanism. A total of 18 compounds were identified from the extracts of L. barbarum berries, mainly dicaffeylspermidine derivatives, amide alkaloids, coumarin and flavonoids.
The retina is one of the highest oxygen-consumption tissues in the body.31 High levels of oxygen exposure, constant light exposure, and high levels of polyunsaturated fatty acids in photoreceptor cells increase oxidative stress in retina.32 Oxygen radicals attack sugars, lipids, and proteins in cells, leading to the accumulation of lipid oxidation products such as 7-ketocholesterol,33 lipid oxidation modification products such as carboxyethylpyrrole oxidative protein modifications34 and advanced glycation end products35 in the retina. The accumulation of these metabolites induces retinal microglia activation and releases IL-6, IL-1β, TNF-α, and IL-8. A long-term chronic inflammatory reaction leads to the degeneration of RPE cells and photoreceptor cells, which aggravates retinal metabolic abnormalities. Clinical research also showed that patients with late AMD showed an increase in the pro-inflammatory cytokine IL-6, TNF receptor 2, and C-reactive protein (CRP) compared to healthy controls.36 For oxidation regulation systems, SOD and GSH are well-known enzymes protecting RPE cells from oxidative damage.37 In contrast, MDA is a lipid peroxide marker that reflects the degree of oxidative damage in RPE cells.38 Studies have shown that Prunella vulgaris var. L extract increases the activity of SOD, decreases the contents of ROS and MDA, and improves the light-induced retinal damage in the ARPE-19 cells of AMD mice.39 Supplementation with antioxidant vitamins C and E, beta carotene, and zinc has been shown to reduce the risk of progression to advanced AMD in a randomized controlled clinical trial.40 Therefore, alleviation of oxidative stress and inflammation of retina and RPE cells have been accepted as effective strategies for ameliorating early AMD.
The results showed that LBW and LBE have a significant protective effect on retinal pathological damage in AMD mice, which may be related to the increase of the activities of SOD and GSH in serum and the decrease of inflammatory factors such as IL-6 and IL-1β in serum and retina.
Metabonomics has been widely used to explore the action mechanism of traditional Chinese medicine. A series of evidence reveal that AMD patients have altered plasma metabolomic profiles compared with normal people.41 Lains discovered that 87 metabolites in the plasma of AMD patients were altered, primarily involving glycerol phospholipid metabolism, purine metabolism, and taurine and subtaurine metabolism.42 Modern research studies indicate that taurine supplementation can prevent retinal diseases by increasing the level of GSH/oxidized glutathione (GSSH), reducing endoplasmic reticulum stress and autophagy response in retinal pigment epithelium cells.43,44 Docosahexaenoic acid (DHA) is the most abundant unsaturated fatty acid in the outer segment of the retinal photoreceptor. Therefore, DHA supplementation could both increase the capacity of endogenous antioxidants and mobilize selective autophagy of misfolded proteins.45 Meanwhile, increased dietary intake of DHA has been demonstrated to improve the macular pigment density and halt the progression of AMD.46
Based on the metabonomics analysis in our study, we found that specific metabolites in serum, such as phosphatidylcholine, phosphatidylethanolamine, docosahexaenoic acid, taurine, etc. are closely related to the occurrence of AMD and may be the potential biomarkers of AMD. However, the specific metabolites were regulated by different extracts. LBW extracts tended to regulate the metabolism of unsaturated fatty acids, while LBE extracts tended to regulate taurine metabolism, which indicated that various chemical components in wolfberries regulated different pathological processes of AMD. Dicaffeylspermidine derivatives, mostly found in LBW extract, might be the representative chemicals in L. barbarum in improving AMD.
Previous studies have confirmed that Lycium barbarum polysaccharide can effectively ameliorate the damage to retinal pigment epithelial cells caused by oxidative stress and play a positive role in the prevention and treatment of AMD. However, there are few reports on the efficacy of other chemical components in L. barbarum berries. Based on the fact that retinal oxidative stress is an important pathological mechanism of AMD, we constructed a cell model to screen the active components of L. barbarum berries in protecting retinal pigment epithelial cells from oxidative damage. Nrf2 plays an important role in initiating the antioxidant response. Nrf2 deficiency decreases nicotinamide adenine dinucleotide phosphate (NADPH) and magnifies oxidative stress via an impaired pentose phosphate pathway and finally causes increased RPE cell death.47 Another study reported similar results: knockout of Nrf2 with specific siRNA successfully inhibits the transcription of HO-1 and eliminates the protective effect of farrerol on RPE cells.48 Natural product Centella Asiatica extract suppresses RPE cell apoptosis and protects the retina from degeneration by up-regulating the Nrf2/HO-1 signalling pathway.49 The current in vitro cell experiments showed that the LBW-95E could significantly improve the survival rate of ARPE-19 cells from oxidative stress which was the main active fraction of LBW. Its mechanism might be related to promoting the translocation of Nrf2 into the nucleus, increasing the levels of intracellular HO-1, SOD and GSH and reducing the level of intracellular ROS.
In the current study, we found that LBW-95E exhibited better antioxidant activity than other fractions. Through the identification of components by UHPLC-Q/TOF-MS/MS, we found that scopoletin, N-feruloyltyramine and perlolyrine were the main components in the LBW-95E fraction. For these compounds, several studies have been reported for its beneficial effect on alleviating oxidative stress and inflammation. Scopoletin increases the accumulation of GSH and improves the survival of neurons.50N-trans-Feruloyltyramine resists H2O2-induced oxidative damage in HepG2 and LO2 cells51 and inhibits the production of nitric oxide (NO) and prostaglandin E2 (PGE2) in lipopolysaccharide-stimulated RAW 264.7 cells.52 Perlolyrine can reduce triglyceride levels in 3T3-L1 cells.53 LBW-30E and LBW-70E mainly contain dicaffeoylspermine derivatives, amide alkaloids and flavonoids according to our identification results for LBW. Dicaffeoylspermidine derivatives in L. barbarum berries have been reported to reverse Alzheimer's symptoms in flies.21 Amide alkaloids in wolfberries had immunomodulatory54 and antioxidant activities.55 Rutin exerts anti-diabetic, anti-inflammation, antioxidation and neuroprotection effects.56 In conclusion, different types of compounds might exhibit different types of pharmacological activities. The better effect of the LBW-95E fraction on alleviating oxidation stress might be due to its unique components.
5. Conclusions
This study demonstrated that L. barbarum berry extracts inhibited retinal degeneration by restoring the thicknesses of the retinal layers in aging mice, which could be related to an increase in antioxidant capacity, as well as a reduction in systemic and retinal inflammation. From metabolomic studies, L. barbarum berries might exert a retinal protection effect by regulating the metabolism of taurine, unsaturated fatty acids and sphingolipids. We also demonstrated that LBW-95E might be the vital fraction of LBW for exerting antioxidant capacity by regulating the Nrf2/HO-1 pathway. Based on these data, we found that L. barbarum berry extracts could alleviate retinal damage and delay the progression of AMD. The chemicals with low polarity in LBW had a positive effect on reducing oxidative damage to the retina, which might be helpful for the development of drugs or therapies using L. barbarum berries for the prevention or treatment of AMD.
Author contributions
Zheng HL: investigation, visualization, and writing – original draft. Li MT: investigation. Zhou T: investigation. Wang YY: methodology. Shang Er-Xin: data curation. Hua YQ: writing – review & editing. Duan JA: conceptualization, funding acquisition, supervision, and writing – review & editing. Zhu Y: project administration, supervision, and writing – review & editing. All authors have read and approved the submitted manuscript.
Conflicts of interest
There are no conflicts to declare.
Acknowledgements
This research was supported by the Key Projects of Regional Joint Fund of National Natural Science Foundation of China (U21A20408) to Duan JA; the Key R&D Program of Ningxia Hui Autonomous Region (East-West Science and Technology Cooperation) (2021BEF02012, 2021BEF01003, 2021BEF02009, 2021BEF02010) to Duan JA and Zhu Y; Ningxia Science and Technology Infrastructure Construction Project (2021DPC05005) to Duan JA. The Key R&D Program of Jiangsu Province (BE2021907) to Duan JA; Major Programs of Natural Science Research in Universities of Jiangsu Province (22KJA360008) and Open Project Program of Jiangsu Key Laboratory for High Technology Research of TCM Formulae and Jiangsu Collaborative Innovation Centre of Chinese Medicinal Resources Industrialization (ZDXM-2020-03) to Zhu Y.
References
- H. Ye, Q. Zhang and X. Liu,
et al., Prevalence of age-related macular degeneration in an elderly urban chinese population in China: the Jiangning Eye Study, Invest. Ophthalmol. Visual Sci., 2014, 55, 6374–6380 CrossRef PubMed
.
- W. L. Wong, X. Su, X. Li, C. M. Cheung, R. Klein, C. Y. Cheng and T. Y. Wong, Global prevalence of age-related macular degeneration and disease burden projection for 2020 and 2040: a systematic review and meta-analysis, Lancet Global Health, 2014, 2, e106–e116 CrossRef PubMed
.
- R. R. Bourne, G. A. Stevens and R. A. White,
et al., Causes of vision loss worldwide, 1990–2010: a systematic analysis, Lancet Global Health, 2013, 1, e339–e349 CrossRef PubMed
.
- P. S. Mettu, M. J. Allingham and S. W. Cousins, Incomplete response to Anti-VEGF therapy in neovascular AMD: Exploring disease mechanisms and therapeutic opportunities, Prog. Retinal Eye Res., 2021, 82, 100906 CrossRef CAS PubMed
.
- T. H. Rim, R. Kawasaki and Y. C. Tham,
et al., Prevalence and Pattern of Geographic Atrophy in Asia: The Asian Eye Epidemiology Consortium, Ophthalmology, 2020, 127, 1371–1381 CrossRef PubMed
.
- V. Kuan, A. Warwick, A. Hingorani, A. Tufail, V. Cipriani, S. Burgess and R. Sofat, Association of Smoking, Alcohol Consumption, Blood Pressure, Body Mass Index, and Glycemic Risk Factors With Age-Related Macular Degeneration: A Mendelian Randomization Study, JAMA Ophthalmol., 2021, 139, 1299–1306 CrossRef PubMed
.
- S. G. Francisco, K. M. Smith and G. Aragones,
et al., Dietary Patterns, Carbohydrates, and Age-Related Eye Diseases, Nutrients, 2020, 12, 2862 CrossRef CAS PubMed
.
- J. T. Handa, M. Cano, L. Wang, S. Datta and T. Liu, Lipids, oxidized lipids, oxidation-specific epitopes, and Age-related Macular Degeneration, Biochim. Biophys. Acta, Mol. Cell Biol. Lipids, 2017, 1862, 430–440 CrossRef CAS PubMed
.
- S. Datta, M. Cano, K. Ebrahimi, L. Wang and J. T. Handa, The impact of oxidative stress and inflammation on RPE degeneration in non-neovascular AMD, Prog. Retinal Eye Res., 2017, 60, 201–218 CrossRef CAS PubMed
.
- J. K. Kibet, L. Khachatryan and B. Dellinger, Phenols from pyrolysis and co-pyrolysis of tobacco biomass components, Chemosphere, 2015, 138, 259–265 CrossRef CAS PubMed
.
- K. Kunchithapautham, C. Atkinson and B. Rohrer, Smoke exposure causes endoplasmic reticulum stress and lipid accumulation in retinal pigment epithelium through oxidative stress and complement activation, J. Biol. Chem., 2014, 289, 14534–14546 CrossRef CAS PubMed
.
- M. Pons and M. E. Marin-Castano, Cigarette smoke-related hydroquinone dysregulates MCP-1, VEGF and PEDF expression in retinal pigment epithelium in vitro and in vivo, PLoS One, 2011, 6, e16722 CrossRef CAS PubMed
.
- D. G. Espinosa-Heidmann, I. J. Suner, P. Catanuto, E. P. Hernandez, M. E. Marin-Castano and S. W. Cousins, Cigarette smoke-related oxidants and the development of sub-RPE deposits in an experimental animal model of dry AMD, Invest. Ophthalmol. Visual Sci., 2006, 47, 729–737 CrossRef PubMed
.
- Y. Gao, Y. Wei, Y. Wang, F. Gao and Z. Chen, Lycium Barbarum: A Traditional Chinese Herb and A Promising Anti-Aging Agent, Aging Dis., 2017, 8, 778–791 CrossRef PubMed
.
- K. Neelam, S. Dey, R. Sim, J. Lee and E. K. Au, Fructus lycii: A Natural Dietary Supplement for Amelioration of Retinal Diseases, Nutrients, 2021, 13, 246 CrossRef CAS PubMed
.
- X. Li, R. R. Holt, C. L. Keen, L. S. Morse, G. Yiu and R. M. Hackman, Goji Berry Intake Increases Macular Pigment Optical Density in Healthy Adults: A Randomized Pilot Trial, Nutrients, 2021, 13, 4409 CrossRef CAS PubMed
.
- S. Li, N. Liu, L. Lin, E. D. Sun, J. D. Li and P. K. Li, Macular pigment and serum zeaxanthin levels with Goji berry supplement in early age-related macular degeneration, Int. J. Ophthalmol., 2018, 11, 970–975 Search PubMed
.
- L. Liu, W. Lao, Q. Ji, Z. Yang, G. Yu and J. Zhong, Lycium barbarum polysaccharides protected human retinal pigment epithelial cells against oxidative stress-induced apoptosis, Int. J. Ophthalmol., 2015, 8, 11–16 CrossRef PubMed
.
- H. M. Cho, Y. D. Jo and S. Y. Choung, Protective Effects of Spirulina maxima against Blue Light-Induced Retinal Damages in A2E-Laden ARPE-19 Cells and Balb/c Mice, Nutrients, 2022, 14, 401 CrossRef CAS PubMed
.
- Y. Hua, S. Guo and H. Xie,
et al., Ziziphus jujuba Mill. var. spinosa (Bunge) Hu ex H. F. Chou Seed Ameliorates Insomnia in Rats by Regulating Metabolomics and Intestinal Flora Composition, Front. Pharmacol., 2021, 12, 653767 CrossRef CAS PubMed
.
- Z. Q. Zhou, H. X. Fan and R. R. He,
et al., Lycibarbarspermidines A-O, New Dicaffeoylspermidine Derivatives from Wolfberry, with Activities against Alzheimer's Disease and Oxidation, J. Agric. Food Chem., 2016, 64, 2223–2237 CrossRef CAS PubMed
.
- X. Xiao, W. Ren, N. Zhang, T. Bing, X. Liu, Z. Zhao and D. Shangguan, Comparative Study of the Chemical Constituents and Bioactivities of the Extracts from Fruits, Leaves and Root Barks of Lycium barbarum, Molecules, 2019, 24, 1585 CrossRef CAS PubMed
.
- P. F. Zhu, Y. L. Zhao and Z. Dai,
et al., Phenolic Amides with Immunomodulatory Activity from the Nonpolysaccharide Fraction of Lycium barbarum Fruits, J. Agric. Food Chem., 2020, 68, 3079–3087 CrossRef CAS PubMed
.
- J. Zhang, S. Guan and J. Sun,
et al., Characterization and profiling of phenolic amides from Cortex Lycii by ultra-high performance liquid chromatography coupled with LTQ-Orbitrap mass spectrometry, Anal. Bioanal. Chem., 2015, 407, 581–595 CrossRef CAS PubMed
.
- M. Catanzaro, C. Lanni, F. Basagni, M. Rosini, S. Govoni and M. Amadio, Eye-Light on Age-Related Macular Degeneration: Targeting Nrf2-Pathway as a Novel Therapeutic Strategy for Retinal Pigment Epithelium, Front. Pharmacol., 2020, 11, 844 CrossRef CAS PubMed
.
- L. Xiong, N. Deng, B. Zheng, T. Li and R. H. Liu, Goji berry (Lycium spp.) extracts exhibit antiproliferative activity via modulating cell cycle arrest, cell apoptosis, and the p53 signaling pathway, Food Funct., 2021, 12, 6513–6525 RSC
.
- L. Xiong, N. Deng, B. Zheng, T. Li and R. H. Liu, HSF-1 and SIR-2.1 linked insulin-like signaling is involved in goji berry (Lycium spp.) extracts promoting lifespan extension of Caenorhabditis elegans, Food Funct., 2021, 12, 7851–7866 RSC
.
- Y. Ding, Y. Yan and D. Chen,
et al., Modulating effects of polysaccharides from the fruits of Lycium barbarum on the immune response and gut microbiota in cyclophosphamide-treated mice, Food Funct., 2019, 10, 3671–3683 RSC
.
- Q. Luo, J. Li, X. Cui, J. Yan, Q. Zhao and C. Xiang, The effect of Lycium barbarum polysaccharides on the male rats reproductive system and spermatogenic cell apoptosis exposed to low-dose ionizing irradiation, J. Ethnopharmacol., 2014, 154, 249–258 CrossRef CAS PubMed
.
- L. Liu, X. Y. Sha, Y. N. Wu, M. T. Chen and J. X. Zhong, Lycium barbarum polysaccharides protects retinal ganglion cells against oxidative stress injury, Neural Regener. Res., 2020, 15, 1526–1531 CrossRef PubMed
.
- D. Y. Yu and S. J. Cringle, Retinal degeneration and local oxygen metabolism, Exp. Eye Res., 2005, 80, 745–751 CrossRef CAS PubMed
.
- S. Datta, M. Cano, K. Ebrahimi, L. Wang and J. T. Handa, The impact of oxidative stress and inflammation on RPE degeneration in non-neovascular AMD, Prog. Retinal Eye Res., 2017, 60, 201–218 CrossRef CAS PubMed
.
- C. Yang, L. Xie, Q. Gu, Q. Qiu, X. Wu and L. Yin, 7-Ketocholesterol disturbs RPE cells phagocytosis of the outer segment of photoreceptor and induces inflammation through ERK signaling pathway, Exp. Eye Res., 2019, 189, 107849 CrossRef CAS PubMed
.
- Q. Ebrahem, K. Renganathan and J. Sears,
et al., Carboxyethylpyrrole oxidative protein modifications stimulate neovascularization: Implications for age-related macular degeneration, Proc. Natl. Acad. Sci. U. S. A., 2006, 103, 13480–13484 CrossRef CAS PubMed
.
- K. AnandBabu, P. Sen and N. Angayarkanni, Oxidized LDL, homocysteine, homocysteine thiolactone and advanced glycation end products act as pro-oxidant metabolites inducing cytokine release, macrophage infiltration and pro-angiogenic effect in ARPE-19 cells, PLoS One, 2019, 14, e216899 CrossRef PubMed
.
- N. M. Krogh, Y. Subhi, C. R. Molbech, M. K. Falk, M. H. Nissen and T. L. Sorensen, Systemic Levels of Interleukin-6 Correlate With Progression Rate of Geographic Atrophy Secondary to Age-Related Macular Degeneration, Invest. Ophthalmol. Visual Sci., 2019, 60, 202–208 CrossRef PubMed
.
- V. M. Di Mambro, M. F. Borin and M. J. Fonseca, Topical formulations with superoxide dismutase: influence of formulation composition on physical stability and enzymatic activity, J. Pharm. Biomed. Anal., 2003, 32, 97–105 CrossRef CAS PubMed
.
- Y. Yan, Y. Ren, X. Li, X. Zhang, H. Guo, Y. Han and J. Hu, A polysaccharide from green tea (Camellia sinensis L.) protects human retinal endothelial cells against hydrogen peroxide-induced oxidative injury and apoptosis, Int. J. Biol. Macromol., 2018, 115, 600–607 CrossRef CAS PubMed
.
- J. Kim, K. Cho and S. Y. Choung, Protective effect of Prunella vulgaris var. L extract against blue light-induced damages in ARPE-19 cells and mouse retina, Free Radicals Biol. Med., 2020, 152, 622–631 CrossRef CAS PubMed
.
- Age-Related Eye Disease Study 2 Research Group, Lutein + zeaxanthin and omega-3 fatty acids for age-related macular degeneration: the Age-Related Eye Disease Study 2 (AREDS2) randomized clinical trial, J. Am. Med. Assoc., 2013, 309, 2005–2015 CrossRef PubMed
.
- I. Lains, R. S. Kelly and J. B. Miller,
et al., Human Plasma Metabolomics Study across All Stages of Age-Related Macular Degeneration Identifies Potential Lipid Biomarkers, Ophthalmology, 2018, 125, 245–254 CrossRef PubMed
.
- I. Lains, W. Chung and R. S. Kelly,
et al., Human Plasma Metabolomics in Age-Related Macular Degeneration: Meta-Analysis of Two Cohorts, Metabolites, 2019, 9, 127 CrossRef CAS PubMed
.
- K. Homma, E. Toda and H. Osada,
et al., Taurine rescues mitochondria-related metabolic impairments in the patient-derived induced pluripotent stem cells and epithelial-mesenchymal transition in the retinal pigment epithelium, Redox Biol., 2021, 41, 101921 CrossRef CAS PubMed
.
- Y. Zhang, S. Ren, Y. Liu, K. Gao, Z. Liu and Z. Zhang, Inhibition of Starvation-Triggered Endoplasmic Reticulum Stress, Autophagy, and Apoptosis in ARPE-19 Cells by Taurine through Modulating the Expression of Calpain-1 and Calpain-2, Int. J. Mol. Sci., 2017, 18, 2146 CrossRef CAS PubMed
.
- I. Johansson, V. T. Monsen and K. Pettersen,
et al., The marine n-3 PUFA DHA evokes cytoprotection against oxidative stress and protein misfolding by inducing autophagy and NFE2L2 in human retinal pigment epithelial cells, Autophagy, 2015, 11, 1636–1651 CrossRef CAS PubMed
.
- C. Schnebelen-Berthier, N. Acar and E. Simon,
et al., The ALGOVUE Clinical Trial: Effects of the Daily Consumption of Eggs Enriched with Lutein and Docosahexaenoic Acid on Plasma Composition and Macular Pigment Optical Density, Nutrients, 2021, 13, 3347 CrossRef CAS PubMed
.
- M. Cano, S. Datta and L. Wang,
et al., Nrf2 deficiency decreases NADPH from impaired IDH shuttle and pentose phosphate pathway in retinal pigmented epithelial cells to magnify oxidative stress-induced mitochondrial dysfunction, Aging Cell, 2021, 20, e13444 CrossRef CAS PubMed
.
- N. Ma, X. Yang, C. Qi, Q. Yu, C. Zhu and H. Ren, Farrerol Enhances Nrf2-Mediated Defense Mechanisms against Hydrogen Peroxide-Induced Oxidative Damage in Human Retinal Pigment Epithelial Cells by Activating Akt and MAPK, Oxid. Med. Cell. Longevity, 2021, 2021, 8847844 Search PubMed
.
- D. W. Park, Y. G. Lee, Y. J. Jeong, H. Jeon and S. C. Kang, Preventive Effects against Retinal Degeneration by Centella asiatica Extract (CA-HE50) and Asiaticoside through Apoptosis Suppression by the Nrf2/HO-1 Signaling Pathway, Antioxidants, 2021, 10, 613 CrossRef CAS PubMed
.
- P. Pradhan, O. Majhi, A. Biswas, V. K. Joshi and D. Sinha, Enhanced accumulation of reduced glutathione by Scopoletin improves survivability of dopaminergic neurons in Parkinson's model, Cell Death Dis., 2020, 11, 739 CrossRef CAS PubMed
.
- X. Gao, C. Wang and Z. Chen,
et al., Effects of N-trans-feruloyltyramine isolated from laba garlic on antioxidant, cytotoxic activities and H2O2-induced oxidative damage in HepG2 and L02cells, Food Chem. Toxicol., 2019, 130, 130–141 CrossRef CAS PubMed
.
- Y. Jiang, L. Yu and M. H. Wang, N-trans-feruloyltyramine inhibits LPS-induced NO and PGE2 production in RAW 264.7 macrophages: Involvement of AP-1 and MAP kinase signalling pathways, Chem.-Biol. Interact., 2015, 235, 56–62 CrossRef CAS PubMed
.
- P. N. Chen, M. J. Hao, H. J. Li, J. Xu, T. Mahmud and W. J. Lan, Biotransformations of anthranilic acid and phthalimide to potent antihyperlipidemic alkaloids by the marine-derived fungus Scedosporium apiospermum F41-1, Bioorg. Chem., 2021, 116, 105375 CrossRef CAS PubMed
.
- P. F. Zhu, Y. L. Zhao and Z. Dai,
et al., Phenolic Amides with Immunomodulatory Activity from the Nonpolysaccharide Fraction of Lycium barbarum Fruits, J. Agric. Food Chem., 2020, 68, 3079–3087 CrossRef CAS PubMed
.
- K. Gao, D. Ma and Y. Cheng,
et al., Three New Dimers and Two Monomers of Phenolic Amides from the Fruits of Lycium barbarum and Their Antioxidant Activities, J. Agric. Food Chem., 2015, 63, 1067–1075 CrossRef CAS PubMed
.
- L. S. Chua, A review on plant-based rutin extraction methods and its pharmacological activities, J. Ethnopharmacol., 2013, 150, 805–817 CrossRef CAS PubMed
.
|
This journal is © The Royal Society of Chemistry 2023 |
Click here to see how this site uses Cookies. View our privacy policy here.