DOI:
10.1039/D2FO02775E
(Paper)
Food Funct., 2023,
14, 335-343
L-Theanine alleviates heat stress-induced impairment of immune function by regulating the p38 MAPK signalling pathway in mice†
Received
7th October 2022
, Accepted 22nd November 2022
First published on 22nd November 2022
Abstract
With the current trend of global warming, heat stress-induced impairment could seriously endanger human health. L-Theanine is a non-protein amino acid in tea with various biological activities, including immunoregulatory, anti-anxiety, and anti-oxidation. However, its effect on immune function under heat stress and the underlying mechanism are currently unclear. In this study, male BALB/c mice were used as experimental objects to explore the effect of L-theanine on heat stress-induced changes in immune function and its mechanism. Three doses of L-theanine were used: low (100 mg kg−1 d−1), medium (200 mg kg−1 d−1), and high (400 mg kg−1 d−1). Treatment with L-theanine could attenuate the heat stress-induced reductions in body weight and feed intake in mice, alleviate damage in the liver and jejunum, and inhibit the inflammatory factors IL-6, IL-1β, and TNF-α. Aspartate aminotransferase and alanine transaminase activity levels and the malondialdehyde content decreased, while the IgA, IgM, and IgG contents increased in response to L-theanine. It is possible that L-theanine affects the P38 signalling pathway and inhibits the increase in p-P65/P65 caused by the overexpression of HSP27 and regulation of PPAR-γ and Foxp3 proteins, thereby alleviating immune dysfunction caused by heat stress.
Introduction
Rising temperatures have contributed to major global health problems.1 Many serious and widespread health problems can be attributed to climate change, such as cardiovascular and respiratory diseases as well as malnutrition.2 A high-temperature environment can lead to the generation of heat stress in the body. Heat stress refers to the sum of the non-specific responses to an unfavourable thermal environment. The body shows a stress response when the ambient temperatures exceed a suitable temperature range,3 which can lead to thermal damage,4 with adverse effects on growth, development, and the nervous, endocrine, and immune systems. Continued increases in greenhouse gases and the corresponding increases in the global temperature, including extremely high temperatures in some areas, are expected to cause severe damage to human health and the economy.5
There is increasing evidence that heat stress can affect the immune system, causing inflammatory responses, such as increases in interleukin-1 beta (IL-1β), interleukin-6 (IL-6), and tumour necrosis factor-alpha (TNF-α).6 Stress also promotes immunoglobulin production, and prolonged or intense stimulation will inhibit immunoglobulin production.7,8 Studies of broilers have shown that heat stress reduces the concentrations of immunoglobulin G (IgG) and immunoglobulin M (IgM), decreases the proliferation of lymphocytes, reduces phagocytosis by macrophages, and negatively affects organs, such as the liver, thymus, and gut.9 The gut plays an important role in the digestion and absorption of nutrients and immunity.10 High temperatures cause intestinal damage and have a serious impact on the intestinal microflora. The liver not only has endocrine functions, but also participates in immune regulation at the local or systemic level. In particular, the liver is an important site for the differentiation of T cells, in addition to the thymus.11 Increases in serum aspartate aminotransferase (AST) and alanine aminotransferase (ALT) are indicators of liver damage.12 The impairment of immune function is highly correlated with the production of T cells. PPAR-γ is a ligand-dependent nuclear transcription factor involved in immune responses. PPAR-γ deficiency results in fewer CD4+ and CD25+ T cells, suggesting that PPAR-γ plays an important role in T cell development.13,14 This PPAR-γ signalling cooperates with Foxp3 on the transcriptional signature of T cells, and PPAR-γ can also affect the expression of ROS and inflammatory factors by regulating macrophage activation.15 Furthermore, PPAR-γ controls the metabolism of many cells and regulates upstream MAPK family members, while the downstream factors mediate multiple pathways, such as the NF-κB, JAK2/STAT1, and NFAT pathways.16 The mitogen-activated protein kinase (MAPK) pathway is rapidly activated after heat stress.17 The MAPK family consists of various enzymes related to cell proliferation, apoptosis, differentiation, and growth, including apoptotic and anti-apoptotic proteins, which activate the JNK and p38 kinase signalling pathways.18 Environmental stress, such as ultraviolet radiation and hyperosmolarity, and the activation of cytokines, such as inflammatory factors, induce inflammation. There are many substrates that are involved in p38 phosphorylation, including the heat shock protein HSP2719 and the ARE elements upstream of TNF-α and other inflammatory factors.20 NF-κB functions downstream of p38 are closely related to immunity and apoptosis. It can mediate the production of pro-inflammatory cytokines, such as TNF-α, IL-6, and IL-1β, in monocytes and macrophages.21 p38 MAPK activates NF-κB and promotes its translocation into the nucleus, causing an inflammatory cascade, further amplifying the inflammatory immune response and aggravating tissue damage.22,23
L-Theanine, a characteristic amino acid in tea, is a glutamine compound. It is safe, non-toxic, and highly active, with a bioavailability of close to 100%.24 It has many biological activities; for example, it improves specific and non-specific immunity and has antidepressant and anti-fatigue effects.25–27 It can increase the immunoglobulin (IgG) levels in mouse serum and improve immune function by increasing the spleen tissue index and reducing the serum corticosterone levels in rats.28L-Theanine is widely used as a food additive in Japan, developed European countries, and the United States. The National Health and Family Planning Commission of China issued an announcement in 2014 to approve the use of L-theanine as a new raw material for food.29 Borzelleca et al. administered 4000 mg kg−1 d−1L-theanine to rats for consecutive weeks and found no side effects or adverse reactions.30 Dietary supplementation with L-theanine can support beneficial microbes, while reducing harmful microbes and enhancing immunity.31 Additionally, L-theanine can affect intestinal mucosal immunity by regulating SCFA metabolism under dietary fibre feeding.32 In the liver, L-theanine can improve LPS-induced acute liver injury, inflammation, immune imbalance, and other factors via the NF-κB signalling pathway.33 The absorption, transportation, and decomposition of L-theanine in the body are predictable, and absorption and decomposition are unrelated to the source of L-theanine.34
Previous studies have revealed that L-theanine protects against oxidative damage under heat stress;35 however, its effect on heat stress-induced changes in immune function and related mechanisms of action have not yet been clarified. Therefore, in this study, the effects and mechanism of action of L-theanine in mice exposed to heat stress were evaluated to provide a theoretical basis for alleviating the negative immune response in high-temperature environments and promote the production and safe, healthy, and nutritious utilisation of L-theanine.26
Materials and methods
Animals and experimental design
In total, 80 SPF male BALB/c mice (aged 4 weeks; weight = 22–24 g) and their feed were purchased from Hunan SJA Laboratory Animal Co., Ltd (Changsha, China; license number SCXK (Xiang) 2019-0004); the quality of the experimental animals was verified (certificate no. 1107272011006941). The animals were kept in the animal room of Hunan Agricultural University Tea Research Institute. Animal experiments were performed in accordance with the National Institutes of Health Guide for the Care and Use of Laboratory Animals (National Institutes of Health Publication no. 8023, revised 1978). All animal experimental protocols were reviewed and approved by the Animal Health and Ethics Committee of Hunan Agricultural University (registration number 015063506). Mouse rearing conditions were as follows: 25 ± 2 °C, a 12 h light/dark cycle, light intensity 100–200 lux, 55 ± 5% humidity.
Adaptive rearing was carried out for 1 week before the experiment, during which mice were allowed to eat and drink freely and were maintained at room temperature (25 ± 2 °C); the experiment began 1 week later. Mice were randomly categorised into 8 groups (n = 10 per group). The groups were as follows (as shown in Fig. 1): control group (CK), model group (MOD), short-term intervention L-theanine low-dose group (S-LLT), short-term intervention L-theanine medium-dose group (S-MLT), short-term intervention L-theanine high-dose group (S-HLT), long-term intervention L-theanine low-dose group (L-LLT), long-term intervention L-theanine medium-dose group (L-MLT), and long-term intervention L-theanine high-dose group (L-HLT). L-Theanine (purity ≥ 98%) was purchased from Sigma-Aldrich (Sunfull Bio-Tech Co., Ltd, Hunan, China). Animals were fed a low dose (100 mg kg−1 d−1), medium dose (200 mg kg−1 d−1), or high dose (400 mg kg−1 d−1) at the same time every day, with a feeding amount of 0.3 mL g−1 and the same amount of potable water. From days 1 to 28, all groups were raised at room temperature. The L-LLT, L-MLT, and L-HLT groups were fed with a low, medium, or high dose of L-theanine, with a feeding volume of 0.3 mL each, and the CK, MOD, S-LLT, S-MLT, and S-HLT groups were fed an equal volume of potable water. After 28 days, the CK and MOD groups were fed with potable water, the S-LLT and L-LLT groups were fed with low-dose L-theanine, the S-MLT and L-MLT groups were fed with medium-dose L-theanine, and the S-HLT and L-HLT groups were fed with high-dose L-theanine. Except for the CK group, mice were subjected to heat stress in a constant temperature and humidity incubator. The treatment conditions were: 19:00–21:00 every day, 40 ± 1 °C, 55 ± 5% humidity. The water intake, food intake, body weight, and survival rate of the mice were monitored daily.
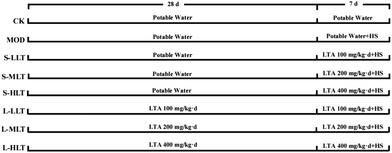 |
| Fig. 1 Animal experimental groups. | |
Sample collection
On the 35th day, the mice were fasted after the last gavage and were anaesthetised immediately after heat stress. After removing the eyeballs to collect the blood, they were sacrificed by dislocating the cervical spine. The blood was left to stand at room temperature (25 ± 2 °C) for 2 h and centrifuged at 3000g for 10 min. The supernatant was collected and stored at −80 °C to be used in the future. After removing the adipose tissue around the liver, thymus, spleen, and jejunum of each mouse, bloodstains were washed with 0.9% normal saline, the surface water was blotted with filter paper, and the samples were weighed. Parts of the liver and jejunum were fixed in 4% polyformaldehyde for H&E staining, and the remaining parts were packaged in tin foil and stored at −80 °C.
Body weight and feed intake determination
Mice in each group were provided with 400 ± 2 g of maintenance feed every week, and the remaining feed was recovered and weighed at a fixed time every week. The body weight of each mouse was determined every week.
Organ index measurement
The collected liver, spleen, and thymus were washed with normal saline to remove the bloodstains on the surface, and the surface water was absorbed with filter paper. The samples were then weighed to obtain the wet weight. We then calculated the organ index according to the following formula: organ index (%) = organ wet weight (g)/mouse body weight (g) × 100.
Serum AST and ALT enzyme activity determination
The mouse serum was obtained to measure the enzymatic activity levels of alanine aminotransferase (ALT, no. C009-2-1) and aspartate aminotransferase (AST, no. C010-2-1) according to the instructions provided with the kit obtained from the Nanjing Jiancheng Bioengineering Institute (Nanjing, China).
Determination of TNF-α, IL-6, and IL-1β
The mouse serum samples were used to detect TNF-α (no. CSB-E11987r), IL-6 (no. CSB-E04640r), and IL-1β (no. CSB-E08054) according to the instructions provided with the ELISA (enzyme-linked immunosorbent assay) kits (Wuhan Huamei Bioengineering Co. Ltd, Wuhan, China).
Determination of the malondialdehyde (MDA) content in the liver homogenate
The cryopreserved liver tissue was supplemented with pre-cooled normal saline at a mass
:
volume ratio of 1
:
9, ground at a low temperature in an automatic tissue grinder and centrifuged to obtain a 10% liver tissue homogenate. The content of MDA (no. A003-1-2) was detected according to the instructions provided with the MDA kit (Nanjing Jiancheng Bioengineering Institute).
Determination of the immunoglobulin levels
Using the cryopreserved liver tissues, the IgA (no. ZC-38501-96), IgM (no. ZC- 38484-96), and IgG (no. ZC-38497-96) contents were evaluated (Changsha Boyi Biotechnology Co. Ltd, Changsha, China).
Histopathological examination of the jejunum and liver
Identical sections of the liver and jejunum were randomly selected, fixed with 4% paraformaldehyde, embedded in paraffin, and stained with haematoxylin and eosin (H&E). The pathological changes in the liver and jejunum tissue sections were observed using light microscopy.
Western blotting
The mouse liver tissues stored at −80 °C were used for protein extraction according to the instructions provided with the protein extraction kit (no. C510003-0050), luminescence kit (no. C506668-0100), and BCA kit (no. C503021-0500) obtained from Sangon Biotech. The primary antibodies were obtained from Proteintech (Rosemont, IL, USA): rabbit anti-ASK1 polyclonal antibody (no. 28201-1-AP), rabbit anti-MEK3 polyclonal antibody (no. 13898-1AP), rabbit anti-MEK6 polyclonal antibody (no. 12745-1-AP), rabbit anti-P38 MAPK polyclonal antibody (no. 14064-1-AP), rabbit anti-MAPKAPK2 polyclonal antibody (no. 13949-1-AP), and rabbit anti-HSP27 polyclonal antibody (no. 18284-1-AP). The following primary antibodies were obtained from CST (Danvers, MA, USA): rabbit anti-NF-κB P65 monoclonal antibody (no. 8242) and rabbit anti-p-p38MAPK monoclonal antibody (no. 4511). The following primary antibodies were obtained from Abcam (Cambridge, UK): rabbit anti-NF-κB P65 (phospho S536) monoclonal antibody (no. ab76302) and rabbit anti-MSK1 monoclonal antibody (no. ab81294). The following primary antibodies were purchased from Wuhan Sanying (Wuhan, China): rabbit polyclonal antibody PPAR-γ (no. 16643-1-AP) and rabbit polyclonal antibody FOXP3 (no. 22173-1-AP). The following primary antibodies, used as controls, were obtained from Sangon Biotech: mouse anti-β-actin monoclonal antibody (no. D191047), mouse anti-β-tubulin monoclonal antibody (no. D191046), and mouse anti-GAPDH monoclonal antibody (no. D190090). The following primary antibody was purchased from Goodhere (Hangzhou, China): rabbit polyclonal antibody GAPDH (no. AB-P-R001). The following secondary antibody was purchased from Proteintech: horseradish peroxidase (HRP)-conjugated goat anti-rabbit antibody (no. SA00001-2, 1
:
6000). The following secondary antibody was purchased from Beyotime Biotechnology: HRP-labelled goat anti-rabbit secondary antibody (no. A0208).
Statistical analysis
After the data were sorted using Excel 2016, IBM Statistics SPSS 22.0 was used for the analysis of variance and the Waller–Duncan tests. The experimental results are expressed as means ± standard deviation (mean ± SD), the plots were generated using R, and the tissue sections were observed and analysed using CaseViewer.
Results
L-Theanine increases the immune organ index and immunoglobulin content in heat-stressed mice
In order to verify the effect of L-theanine on immunity, the organ index and immunoglobulin levels of mice were analysed. As shown in Fig. 2, compared with the values in the CK group, the organ indexes were lower in the MOD group (Fig. 2A–C), and the thymus and spleen indexes were significantly reduced (P < 0.05, Fig. 2A and B), indicating that heat stress caused damage to the immune organs. The treatment of mice with L-theanine relieved organ damage caused by heat stress, with better effects in the long-term group compared to the short-term group. Immunoglobulins enhance immunity and have important protective effects. The levels of the immunoglobulins IgA, IgM, and IgG were significantly lower in the mice under heat stress (MOD) than in those in the CK group (P < 0.05, Fig. 2D–F). These results showed that intervention with L-theanine could increase the immunoglobulin levels, with the strongest effects in the L-MLT group.
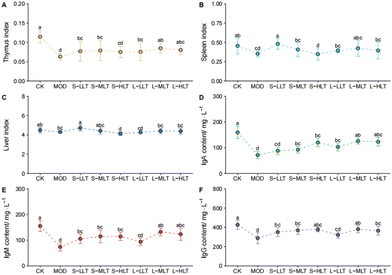 |
| Fig. 2 The levels of the (A) thymus index, (B) spleen index and (C) liver index and the levels of (D) IgA, (E) IgM, and (F) IgG in the mouse liver tissues. The values are reported as means ± SD (n = 10). The lowercase letters above each column within the same group indicate significant differences, as determined by one-way ANOVA (P < 0.05). | |
L-Theanine effectively decreases jejunal tissue damage in heat-stressed mice
A large number of beneficial bacteria in the gut can promote the production of antibodies and enhance immunity. The analyses of the mouse jejunum tissue sections are shown in Fig. 3. Compared with those in the CK group (Fig. 3a(A)), the jejunal villi of the MOD group (Fig. 3a(B)) were shortened, thickened, and broken. However, the jejunal villi of mice treated with L-theanine were more neatly arranged and less fractured under heat stress (Fig. 3a(C–H)), indicating that L-theanine can effectively attenuate the damage caused by heat stress in the mouse jejunum. The villus height was smaller and crypt depth was larger in the heat stress group than those in the CK group, while the L-theanine treatment group showed a significantly increased (P < 0.05) villus height and villus/crypt (V/C) ratio as well as lower crypt depth under heat stress (Fig. 3b).
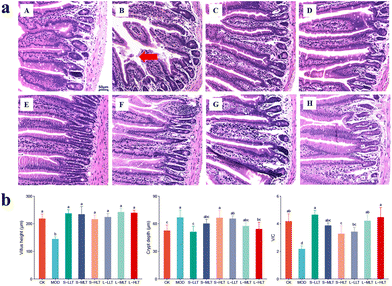 |
| Fig. 3 Effect of L-theanine treatment on the intestinal tissue slices in mice. (a) The representative staining of the jejunum sections (H&E, ×300); the red arrow indicates the jejunum under chronic heat stress. (b) Differences in the villus height and crypt depth among the treatment groups. (A) CK; (B) MOD; (C) S-LLT; (D) S-MLT; (E) S-HLT; (F) L-LLT; (G) L-MLT; and (H) L-HLT treatment groups. | |
L-Theanine effectively decreases liver tissue damage in heat-stressed mice
In addition to its role as the main metabolic organ, the liver plays a major part in immunity as well. The representative liver tissue sections are shown in Fig. 4. Compared with the normal liver cells in the CK group (Fig. 4A), the MOD group (Fig. 4B) had swelling, with substantial clustering of inflammatory cells, and the cytoplasm was translucent. Compared with the MOD group, the L-theanine treatment group showed less heat stress-induced liver tissue damage, with few inflammatory cells gathered around the liver (Fig. 4C–H).
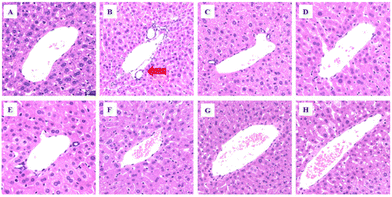 |
| Fig. 4 The representative staining of the liver sections (H&E, ×300); the red arrow indicates liver failure under HS. (A) CK; (B) MOD; (C) S-LLT; (D) S-MLT; (E) S-HLT; (F) L-LLT; (G) L-MLT; and (H) L-HLT treatment groups. | |
L-Theanine reduces the production of the inflammatory factors, MDA content, and AST and ALT enzyme activities in heat-stressed mice
The levels of the inflammatory factors (TNF-α, IL-1β, and IL-6), MDA, and AST and ALT enzyme activities in each treatment group were further verified. The results are shown in Fig. 5. Compared with the levels in the CK group, the enzymatic activity levels of ALT and AST and the MDA content were significantly up-regulated in the mice subjected to heat stress (MOD group) (P < 0.05; Fig. 6A–C), while L-theanine treatment significantly attenuated the injury caused by heat stress, particularly in the long-term group.
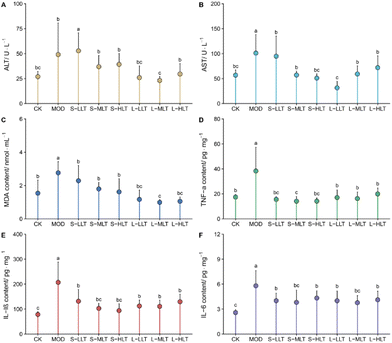 |
| Fig. 5 The levels of (A) ALT, (B) AST, and (C) MDA in the mouse liver tissues and (D) TNT-α, (E) IL-1β, and (F) IL-6 in the mouse serum. The values are reported as means ± SD (n = 10). The lowercase letters above each column within the same group indicate a significant difference, determined by one-way ANOVA (P < 0.05). | |
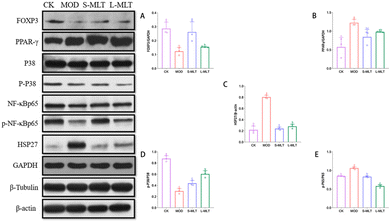 |
| Fig. 6 (A) FOXP3 and (B) PPAR-γ protein expression levels, (C) HSP27 protein expression levels, and (D) p-P38/P38 and (E) NF-κB (p65) phosphorylation in the mouse liver tissues. The values are reported as means ± SD (n = 3). The lowercase letters above each column within the same group indicate a significant difference determined by one-way ANOVA (P < 0.05). | |
The levels of TNF-α, IL-1β, and IL-6 in the MOD group were higher than those in the CK group (P < 0.05; Fig. 5D–F); treatment with L-theanine effectively slowed the production of the inflammatory factors (P < 0.05), consistent with the observations based on the tissue sections, showing that L-theanine can inhibit heat stress-induced inflammatory responses and liver damage. Moreover, the L-MLT group showed a better therapeutic effect.
L-Theanine alleviates the down-regulation of the expression of the Foxp3 protein and the up-regulation of the PPAR-γ protein in the liver tissues of heat-stressed mice
The results of the above-described assays suggested that the effects of L-theanine were optimal at the medium dose (200 mg kg−1 d−1); accordingly, medium-dose L-theanine was used for the subsequent assays.
The damage to immune function is highly related to the production of Tregs. To further explore whether L-theanine has a protective effect on immune function and its related mechanism, we detected the PPAR-γ and FOXP3 proteins. The results showed that compared with the CK group, the MOD group down-regulated Foxp3 (Fig. 6A) and up-regulated the protein expression of PPAR-γ (Fig. 6B). However, the intervention of L-theanine, especially for the S-MLT group, significantly up-regulated the expression of Foxp3 protein and significantly down-regulated the protein expression of PPAR-γ (P < 0.05).
L-Theanine alleviates the up-regulation of the HSP27 protein expression, the down-regulation of the p-P38/P38 phosphorylation levels, and the up-regulation of related proteins in the NF-κB signalling pathway in the liver tissues of heat-stressed mice
As a subtype of MAPKs, p38 MAPK can be activated via heat stress and mediate immune functions. Therefore, the NF-κB signalling pathway, which can mediate the production of pro-inflammatory factors in tandem with p38 MAPK, and the phosphorylated heat shock protein HSP27 downstream of p38 were evaluated.
Compared with the levels in the CK group, the NF-κB signalling pathway and the expression of the heat stress protein HSP27 were significantly up-regulated in the MOD group (P < 0.05; Fig. 6E and C) and the phosphorylation level of p-P38/P38 (Fig. 6D) was significantly down-regulated (P < 0.05; Fig. 6E and C). The injury caused by heat stress was significantly improved by theanine supplementation (S-MLT and L-MLT), and the effect was most pronounced in the L-MLT group (P < 0.05).
L-Theanine alleviates the up-regulation of the expressions of the ASK1 and MK2 proteins and the down-regulation of the MEK3, MEK6, and MSK1 proteins in the p38MAPK signalling pathway in the liver tissues of heat-stressed mice
To further explore the mechanism of action of L-theanine, the expression levels of the p38MAPK signalling pathway proteins and heat stress-related proteins were detected. The MOD group showed higher levels of ASK1 (Fig. 7A) and MK2 (Fig. 7D) and lower protein expression levels of MEK3 (Fig. 7B), MEK6 (Fig. 7C), and MSK1 (Fig. 7E) compared to those in the CK group. However, L-theanine treatment could attenuate the up-regulation of ASK1 and MK2 and the down-regulation of MEK3, MEK6, and MSK1; in particular, in the L-MLT group, there was a significant up-regulation of the protein expression of MEK3/6 (P < 0.05), suggesting that long-term treatment (L-MLT) has the greatest effects and should be a focus of comparative analyses.
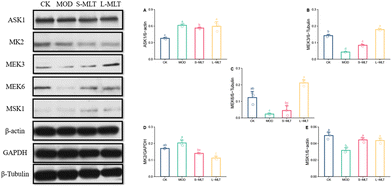 |
| Fig. 7 The protein expression levels of (A) ASK1, (B) MEK3, (C) MEK6, (D) MK2, and (E) MSK1. The values are reported as means ± SD (n = 3). The lowercase letters above each column within the same group indicate significant differences, as determined by one-way ANOVA (P < 0.05). | |
Discussion
To analyze the effects of heat stress, mice were housed in a high-temperature stress environment to stimulate thermal damage, which is one of the most commonly used experimental mouse models33 to investigate the functional components with therapeutic effects against heat stress. We studied the protective effect of L-theanine against the immune function damage induced by heat stress. Specifically, L-theanine intervention was shown to alleviate heat stress-induced liver and intestinal damage, increase the immune organ index and immunoglobulin content, and improve the systemic inflammatory response. Through the p38MAPK signalling pathway, L-theanine can inhibit the production of HSP27 and the activation of NF-κB signalling. These findings suggest that L-theanine is an effective functional food component that can be used as a protectant of the immune function against heat stress-induced impairment.
Strengthening immune function and maintaining health through food nutritional interventions are important directions in nutrition science research.36 As one of the main quality and functional components, L-theanine exerts various biological effects, including immunoregulatory, anti-oxidant, sedative, and anti-anxiety.37 Theanine is absorbed in the intestinal tract, an important digestive organ that provides nutrients to other immune and detoxification systems. Under high-temperature conditions, the gut, as the central organ involved in the stress response, is vulnerable to damage.38 In this study, the jejunal villi of the mice were shortened, thickened, and broken after the high-temperature treatment, and the body weight and feed intake also decreased (Table S1†). The mice treated with L-theanine had intact jejunal villi, arranged neatly, with the attenuation of the decline in body weight and feed intake (Table S2†).
Immune organ indexes are indicators of immune function. The thymus is an important central immune organ and the spleen is an important peripheral immune organ. As an important metabolic organ in humans, the liver is involved in protein and sugar metabolism, detoxification, defense, and the regulation of blood circulation. The liver is also regarded as an immune organ.39 The developmental status of the immune organs can determine the level of immunity, and an increase in the immune organ index can indicate an enhanced immune function in cells.39 In this study, the thymus, spleen, and liver indexes decreased in the mice treated with heat stress and these immune organ indexes all rebounded after the preventive L-theanine treatment. The ALT and AST levels can be used to assess liver damage, which can be induced by heat stress.33 Our findings reveal that heat stress significantly increased the activities of AST and ALT in the serum and the content of MDA in the liver. We also showed that L-theanine supplementation attenuates these heat stress-induced changes and may have beneficial effects on liver injury. The protective effects of PPAR-γ involve a number of mechanisms in vivo and in vitro, including the effects on the transcriptional signatures of T cells and the attenuation of oxidative stress damage.40 In our results, the MOD group showed the up-regulation of PPAR-γ, which was likely caused by the stress response of the mice under heat stress conditions. The L-theanine treatment group showed alleviated heat stress injury, so the PPAR-γ up-regulation was not as high as that in the MOD group. Foxp3 and PPAR-γ co-regulate T cells.41 In this study, we showed that L-theanine can effectively up-regulate the Foxp3 protein expression, thereby improving the immune capacity of mice.
To further explore the mechanism by which L-theanine regulates immune function during heat stress, we evaluated p38 MAPK, the NF-κB signalling pathway, and HSP27. Studies have shown that under heat stress, the p38 MAPK signalling pathway is activated, and the inhibition of p38 MAPK may be crucial for the treatment of human autoimmune diseases.42 Dorion et al.43 found that transient heat stress (44 °C, 10 min) or oxidative stress can activate the p38 MAPK signalling pathway by the continuous induction of kinases, such as ASK1 (a MAP3K). In this study, heat stress led to a significant increase in the protein expression of ASK1 in the mouse liver, while long-term L-theanine treatment significantly attenuated heat-stress induced increases in ASK1 in the liver. The activation of the p38 MAPK signalling pathway is the result of the joint action of multiple kinases, including MAPK, MAPK kinase (MAP2Ks), and MAP2Ks kinase (MAP3Ks). The key MAP2Ks are mainly MEK3 and MEK6, which depend on signalling by the upstream MAP3K family, resulting in a cascade reaction, which transmits the signal downstream of p38 and regulates the activation of p38.44 The first p38 substrate discovered was MK2. There are many substrates for MK2 phosphorylation, including the heat shock protein HSP27 and the ARE elements upstream of inflammatory factors, such as TNF-α,45 which induce the expression of inflammatory factors. Studies in rat cardiomyocytes have revealed that HSP27 can be induced in vivo and in vitro by acute heat stress at the gene and protein levels46 and that HSP27 can enhance the cellular thermotolerance (i.e., improve the survival rate of cells under heat stress).47,48 Furthermore, several studies have shown that HSP27 is up-regulated with increased inflammation.49 In our study, the levels of inflammatory factors TNF-α, IL-6, and IL-1β increased after heat stress and these increases were attenuated by L-theanine, consistent with the changes in HSP27, suggesting that HSP27 may have some anti-inflammatory or immunomodulatory effects on leukocytes.50 In this study, heat stress led to the overexpression of ASK1 in the mouse liver, decreases in MEK3 and MEK6 by MAP3K family proteins, decreases in the expression of p-P38/P38 via the decreases in MEK3/6 and negative feedback involving stress-induced MAPs. The MK2 expression increased, the downstream HSP27 expression increased, the MSK1 expression decreased, and the downstream p-P65/P65 expression increased because the over-activation of HSP27 also led to an increase in p-P65/P65. Therefore, we believe that the regulation of the p38MAPK signalling pathway by L-theanine may be a potential target for relieving heat stress by modulating the immune function. Although we evaluated the p38 MAPK signalling pathway and the mechanism by which L-theanine improves immune function under heat stress, further studies are needed to determine how L-theanine regulates protein synthesis. In future research, we will study the key genes and transcription factors that regulate pathway proteins and further clarify the relevant mechanisms.
Conclusion
Our findings indicate that L-theanine can prevent heat stress-induced inflammation and ameliorate heat stress-induced damage to the body's immune function. The effects of L-theanine may be mediated by the p38 MAPK signalling pathway, the regulation of HSP27, PPAR-γ, and Foxp3 proteins, and interactions with the NF-κB signalling pathway. Further research is needed to determine how L-theanine regulates proteins in these pathways by altering the expression of key genes under heat stress.
Data availability statement
The datasets supporting this article have been uploaded as part of the ESI.†
Author contributions
Yuan Hu: data curation, methodology, software, validation, writing – reviewing and editing, and writing – original draft; Ling Lin: data curation, software and validation; Kehong Liu: editing; Enshuo Liu: visualisation; Shumin Han: investigation; Zhihua Gong: supervision; and Wenjun Xiao: conceptualisation, methodology, funding acquisition, validation, supervision, project administration, reviewing and editing.
Conflicts of interest
The authors declare that there are no conflicts of interest.
Acknowledgements
This work was financially supported by the National Key Research and Development Program of China (grant number 2017YFD0400803), the Key R&D Program of Hunan Province, China (grant number 2021NK2016), and the Major Science and Technology Project of Hunan Province (grant number 2021NK2010).
References
- C. Caminade, K. M. McIntyre and A. E. Jones, Impact of recent and future climate change on vector-borne diseases, Ann. N. Y. Acad. Sci., 2019, 1436(1), 157–173 CrossRef PubMed.
-
J. Fadda, Climate change: An overview of potential health impacts associated with climate change environmental driving forces, in Renewable Energy and Sustainable Buildings, ed. A. Sayigh, Springer, Cham, 2020, ch. 8, pp. 77–119 Search PubMed.
- Y. Xiong, X. Y. Ma, C. Zheng, Z. Tian, J. Zhang, W. Chen, Y. Hu and L. Wang, Effects of heat stress on intestinal health, immune system and meat quality of pigs and its mechanisms, Chin. J. Anim. Nutr., 2017, 29(02), 374–381 CAS.
- N. Zhao and G. H. Li, Research progress on the effects of heat stress on the immune system of the body, Ningxia Med. J., 2014, 36(06), 564–566 CAS.
- M. A. Borg, J. J. Xiang, O. Anikeeva, D. Pisaniello, A. Hansen and K. Zander,
et al., Occupational heat stress and economic burden: A review of global evidence, Environ. Res., 2021, 195(5), 110781 CrossRef CAS PubMed.
- P. Li, G. Wang, X. L. Zhang, G. L. He, X. Luo and J. Yang,
et al., MicroRNA-155 promotes heat stress-induced inflammation via targeting liver X receptor α in microglia, Front. Cell. Neurosci., 2019, 13, 12 CrossRef CAS PubMed.
- H. Chen, C. J. Wang, Smujid, C. Zhang, S. T. Wu and P. Xu,
et al., Effects of chronic heat stress on blood biochemical indexes, antioxidant capacity and immune function of grazing beef cattl, J. China Agric. Univ., 2021, 26(02), 61–69 Search PubMed.
- J. Yu, J. Xue, Y. X. Hu and M. Wang, Effects of acute heat stress on gene expression of heat stress proteins and cytokines in mouse lung tissue, Chin. J. Vet. Med., 2015, 51(10), 20–23 CAS.
- S. P. He, M. A. Arowolo, R. F. Medrano, S. Li, Q. F. Yu and J. Y. Chen,
et al., Impact of heat stress and nutritional interventions on poultry production, World's Poult. Sci. J., 2018, 74(4), 647–664 CrossRef.
- M. Dawood, Nutritional immunity of fish intestines: Important insights for sustainable aquaculture, Rev. Fish. Sci., 2020, 13(1), 642–663 Search PubMed.
- T. Abo, T. Kawamura and H. Watanabe, Physiological responses of extrathymic T cells in the liver, Immunol. Rev., 2000, 174(1), 135–149 CrossRef CAS PubMed.
- H. Q. Cui, Application of serum ALT, AST and GGT detection in the diagnosis of liver diseases, Int. J. Lab. Med., 2017, 38(16), 2293–2295 Search PubMed.
- R. Hontecillas and J. Bassaganyariera, Peroxisome proliferator-activated receptor γ is required for regulatory CD4+ T cell-mediated protection against colitis, J. Immunol., 2007, 178(5), 2940–2949 CrossRef CAS PubMed.
- Y. X. Zhu, Y. Y. Ni, R. Liu, M. Hou, B. Y. Yang and J. W. Song,
et al., PPAR-γ agonist alleviates liver and spleen pathology via inducing Treg cells during Schistosoma japonicum infection, J. Immunol. Res., 2018, 11 Search PubMed.
- A. Viola, F. Munari, R. Sánchez-Rodríguez, R. Scolaro and A. Castegna, The metabolic signature of macrophage responses, Front. Immunol., 2019, 10, 1462 CrossRef CAS PubMed.
- S. Xiang, K. Chen, L. Xu, T. Wang and C. Guo, Bergenin exerts hepatoprotective effects by inhibiting the release of inflammatory factors, apoptosis and autophagy via the PPAR-γ pathway, Drug Des., Dev. Ther., 2020, 14, 129 CrossRef CAS PubMed.
- H. Yuan, X. M. Song, J. F. Jiang, J. T. Guo, F. X. Shi and Y. Q. Jing, Mitogen-activated protein kinase signaling pathway and its relationship with heat stress response, China Anim. Husb. Vet. Med., 2012, 39(01), 114–118 CAS.
- T. Wada and J. M. Penninger, Mitogen-activated protein kinases in apoptosis regulation, Oncogene, 2004, 23(16), 2838–2849 CrossRef CAS PubMed.
- A. Kotlyarov, Y. Yvonne and S. Fritz, Distinct cellular functions of MK2, Mol. Cell. Biol., 2002, 22(13), 4827–4835 CrossRef CAS PubMed.
- A. Neininger, D. Kontoyiannis, R. Winzen, R. Eckert, H. D. Volk and H. Holtmann,
et al., MK2 targets AU-rich elements and regulates biosynthesis of tumor necrosis factor and interleukin-6 independently at different post-transcriptional levels, J. Biol. Chem., 2002, 277(5), 3065–3068 CrossRef CAS PubMed.
- H. Zelová and J. Hoek, TNF-α signalling and inflammation: Interactions between old acquaintances, Inflammation Res., 2013, 62(7), 641–651 CrossRef PubMed.
- Y. Cai, W. C. Li, H. F. Tu, N. M. Chen, Z. P. Zhong and P. C. Yan,
et al., Curcumolide reduces diabetic retinal vascular leukostasis and leakage partly via inhibition of the p38MAPK/NF-κ B signaling, Bioorg. Med. Chem. Lett., 2017, 27(8), 1835–1839 CrossRef CAS PubMed.
- O. S. Zaki, M. M. Safar, A. A. Ain-Shoka, L. A. Rashed and A. Laila, Bone marrow mesenchymal stem cells combat lipopolysaccharide-induced sepsis in rats via amendment of P38-MAPK signaling cascade, Inflammation, 2018, 41(2), 541–554 CrossRef CAS PubMed.
- P. Pijl, L. Chen and T. Mulder, Human disposition of L-theanine in tea or aqueous solution, J. Funct. Foods, 2010, 2(4), 239–244 CrossRef.
- P. J. Nathan, K. Lu, M. Gray and C. Oliver, The neuropharmacology of L-theanine (N-ethyl-L-glutamine), J. Herb. Pharmacother., 2006, 6(2), 21–30 CAS.
-
C. Guo, Study on the anti-heat stress effect of L-theanine and its mechanism, Hunan Agricultural University, 2017 Search PubMed.
- J. F. Bukowski and S. S. Percival,
L-Theanine intervention enhances human γδ T lymphocyte function, Nutr. Rev., 2008, 96–102 CrossRef PubMed , ch. 2.
- W. Zhu, P. G. Feng, J. J. Ma, R. Zhang, S. Zheng and H. M. Yang,
et al., Effects of ambient temperature on harmful gas emissions, environmental microorganisms and serum biochemical indexes of mutton sheep, Chin. J. Anim. Nutr., 2018, 30(11), 10 Search PubMed.
- C. J. Li, L. Luo and C. H. Huang, Research progress on antioxidant effect of L-theanine, J. Food Sci. Technol., 2018, 36(04), 69–75 Search PubMed.
- J. F. Borzelleca, D. Peters and W. Hall, A 13-week dietary toxicity and toxiicokinetic study with L-theanine in rats, Food Chem. Toxicol., 2006, 44(7), 1158–1166 CrossRef CAS PubMed.
- M. Saeed, Y. Xu, T. T. Zang, R. Qian and S. Chao, 16S ribosomal RNA sequencing reveals a modulation of intestinal microbiome and immune response by dietary L-theanine supplementation in broiler chickens, Poult. Sci., 2019, 98(2), 842–854 CrossRef CAS PubMed.
- W. Xu, L. Lin, A. Liu, T. Zhang, S. Zhang and Y. Li,
et al., L-Theanine affects intestinal mucosal immunity by regulating short-chain fatty acid metabolism under dietary fiber feeding, Food Funct., 2020, 11, 8369 RSC.
- D. X. Wang, Q. Gao, G. S. Zhao, Z. P. Kan, X. X. Wang and H. S. Wang,
et al., Protective effect and mechanism of theanine on lipopolysaccharide-induced inflammation and acute liver injury in mice, J. Agric. Food Chem., 2018, 66(29), 7674–7683 CrossRef CAS PubMed.
- T. Treashima, J. Takido and H. Yokgoshi, Time-dependent changes of amino acids in the serum, liver, brain and urine of rats administered with theanine, Biosci., Biotechnol., Biochem., 1999, 63(4), 615–618 CrossRef PubMed , ch. 4.
- D. Wang, M. Cai, T. Wang, G. Zhao, J. Huang and H. Wang,
et al., Theanine supplementation prevents liver injury and heat shock response by normalizing hypothalamic-pituitary-adrenal axishyperactivity in mice subjected to whole body heat stress, J. Funct. Foods, 2018, 45, 181–189 CrossRef CAS.
- C. Venter, S. Eyerich, T. Sarin and C. Klatt, Nutrition and the immune system: a complicated tango, Nutrients, 2020, 12(3), 818 CrossRef CAS PubMed.
- K. Liu, E. Liu, L. Lin, Y. Hu, Y. Yuan and X. Xiao,
L-Theanine mediates the p38MAPK signaling pathway to alleviate heat-induced oxidative stress and inflammation in mice, Food Funct., 2022, 13, 2120–2130 RSC.
- G. Zhong, D. Shao, Q. Wang, H. Tong and S. Shi, Effects of dietary supplemented of γ-amino butyric acid on growth performance, blood biochemical indices and intestinal morphology of yellow-feathered broilers exposed to a high temperature environment, Ital. J. Anim. Sci., 2020, 19(1), 431–438 CrossRef CAS.
- V. Benseler and H. J. Schlitt, The liver as an immunological organ, Gastroenterology, 2011, 49(1), 54–62 CAS.
- Y. Jiang and Q. Zhang, Catalpol ameliorates doxorubicin-induced inflammation and oxidative stress in H9C2 cells through PPAR-γ activation, Exp. Ther. Med., 2020, 20(2), 1003–1011 CrossRef CAS PubMed.
- S. Kawamoto, M. Maruya, L. Kato, W. Suda, K. Atarashi and D. Y,
et al., Foxp3(+) t cells regulate immunoglobulin a selection and facilitate diversification of bacterial species responsible for immune homeostasis, Immunity, 2014, 41(1), 152–165 CrossRef CAS PubMed.
- R. Beyaert, A. Cuendal, W. V. Berghe, S. Plaisance, J. C. Lee and G. Haegeman,
et al., The p38/RK mitogen-activated protein kinase pathway regulates interleukin-6 synthesis response to tumor necrosis factor, EMBO J., 1996, 15(8), 1914–1923 CrossRef CAS.
- S. Dorion, H. Lambert and J. Landry, Activation of the p38 Signaling Pathway by Heat Shock Involves the Dissociation of Glutathione S-Transferase Mu from Ask1, J. Biol. Chem., 2002, 277(34), 30792–30797 CrossRef CAS PubMed.
- Y. J. Liang and W. X. Yang, Kinesins in MAPK cascade: how kinesin motors are involved in the MAPK pathway?, Gene, 2018, 684, 1–9 CrossRef PubMed.
- S. Tang, Study on the expression of small heat shock protein and the mechanism of anti-stress injury in rat cardiomyocytes induced by heat stress, J. Nanjing Agric. Univ., 2015, 72–76 Search PubMed.
- E. H. Richards, E. Hickey, L. Weber and J. R. Master, Effect of overexpression of the small heat shock protein HSP27 on the heat and drug sensitivities of human testis tumor cells, Cancer Res., 1996, 56(10), 2446–2451 CAS.
- F. Trautinger, C. Kokesch, I. Herbacek, R. M. Knobler and K. Ingela, Overexpression of the small heat shock protein, hsp27, confers resistance to hyperthermia, but not to oxidative stress and UV-induced cell death, in a stably transfected squamous cell carcinoma cell line, J. Photochem. Photobiol., B, 1997, 39(1), 90–95 CrossRef CAS PubMed.
- A. T. Hastie, K. B. Everts, J. Zangrilli, J. R. Shaver and S. P. Peters, HSP27 elevated in mild allergic inflammation protects airway epithelium from H2SO4 effects, Am. J. Physiol., 1997, 273(2 Pt 1), L401–L409 CAS.
- N. Hashiguchi, H. Ogura, H. Tanka, T. Koh, Y. Nakamori and T. Shizaki,
et al., Enhanced expression of heat shock proteins in activated polymorphonuclear leukocytes in patients with sepsis, J. Trauma, 2001, 51(6), 1104–1109 CAS.
- M. Iddir, A. Brito, G. Dingeo, F. Sosa, H. Samouda and M. L. Frano,
et al., Strengthening the immune system and reducing inflammation and oxidative stress through diet and nutrition: Considerations during the COVID-19 Crisis, Nutrients, 2020, 12(6), 1562 CrossRef CAS PubMed.
|
This journal is © The Royal Society of Chemistry 2023 |