DOI:
10.1039/D2FO02338E
(Paper)
Food Funct., 2023,
14, 319-334
Multispectroscopic, virtual and in vivo insights into the photoaging defense mediated by the natural food colorant bixin
Received
8th August 2022
, Accepted 28th November 2022
First published on 5th December 2022
Abstract
An upsurge in early onset of photoaging due to repeated skin exposure to environmental stressors such as UV radiation is a challenge for pharmaceutical and cosmeceutical divisions. Current reports indicate severe side effects because of chemical or synthetic inhibitors of matrix metalloproteases (MMPs) in anti-skin aging cosmeceuticals. We evaluated the adequacy of bixin, a well-known FDA certified food additive, as a scavenger of free radicals and its inhibitory mechanism of action on MMP1, collagenase, elastase, and hyaluronidase. The anti-skin aging potential of bixin was evaluated by several biotechnological tools in silico, in vitro and in vivo. Molecular docking and simulation dynamics studies gave a virtual insight into the robust binding interaction between bixin and skin aging-related enzymes. Absorbance and fluorescence studies, enzyme inhibition assays, enzyme kinetics and in vitro bioassays of human dermal fibroblast (HDF) cells highlighted bixin's role as a potent antioxidant and inhibitor of skin aging-related enzymes. Furthermore, in vivo protocols were carried out to study the impact of bixin administration on UVA induced photoaging in C57BL/6 mice skin. Here, we uncover the UVA shielding effect of bixin and its efficacy as a novel anti-photoaging agent. Furthermore, the findings of this study provide a strong foundation to explore the pharmaceutical applications of bixin in several other biochemical pathways linked to MMP1, collagenase, elastase, and hyaluronidase.
1. Introduction
The skin is a protective barrier of multiple layers, shielding the body from mechanical stress and environmental stimuli. However, with repeated exposure to UV radiation, the rate of skin aging is amplified, resulting in the loss of skin moisture and elasticity, and wrinkle formation.1 In comparison with the other types of UV radiation (UVB and UVC), 95% of UV rays of the sun is composed of UVA radiation, which penetrates the skin deeply and induces significant changes in the dermal connective tissue. The detrimental effects of UVA at molecular levels and the resultant tissue degradation by the breakdown of biomolecules such as hyaluronic acid, elastin, and collagen mediated by the overexpression of enzymes such as hyaluronidase, elastase, and collagenase2 have been demonstrated in recent years.3,4
Collagen and elastin are essential for skin flexibility and elasticity, making it appear youthful5 while hyaluronic acid is critical for maintaining skin moisture.6 Several chemical compounds are being used in commercial anti-skin aging cosmetics exhibiting rapid results. However, toxicity evaluation of these formulations has reported the use of chemical UV filters such as octyl dimethyl-p-aminobenzoic acid (OD-PABA), butyl methoxydibenzoylmethane (BMDM), ZnO, TiO2 and benzophenone derivatives among several others that are known to cause cytotoxicity, mutagenicity, genotoxicity, estrogenicity and neurotoxicity7,8
As a result, there is an upsurge in identifying plant-based natural compounds as potential therapeutic agents. In this regard, several plant metabolites such as flavonoids, catechins, tannins, ascorbic acid, alkaloids, and polyphenols have been identified as potent inhibitors of collagenase, elastase, and hyaluronidase.2,9–12
Among the plant secondary metabolites, food pigments are the least explored for their anti-skin aging potential despite their well-known antioxidant properties,13 multi-fold bioactivity, and industrial applications.14,15 Therefore, in this study, bixin, a cis-apocarotenoid present in the seeds of Bixa orellana L. commonly used as a food additive and food colorant (FDA approved)16,17 with multiple therapeutic bioactivity and anti-aging properties,18 was selected to screen its potential as an inhibitor of enzymes activated due to photoaging. Moreover, bixin has been found to possess an oxidation potential of 0.94 V, which is the highest among the naturally occurring carotenoids19 owing to its structure (presence of double bonds), allowing for flexible transfer of electrons20 as displayed in the top left of the spectroscopic plot (Fig. 1A). Recently, bixin has also been identified for its association with NRF2 (nuclear factor erythroid 2-related factor 2) activation linked cellular antioxidant response, thereby protecting against dermal hyperproliferation along with attenuation of PUVA (psoralen + UVA) activity, resulting in delayed greying of hair in an animal model-based study.21 The potent antioxidant properties and therapeutic adequacy of bixin could be tapped for use as a nutraceutical in cosmetics to scavenge the free radicals generated by environmental stressors such as solar UV radiation, which triggers premature photoaging pathways by the activation of matrix metalloprotease (MMP) mediated overexpression of collagenase, elastase, and hyaluronidase.22,23 Also, nanotechnology assisted biotechnological interventions have helped to improve the poor stability of bixin, which is one of the major shortcomings of bixin as revealed by biopharmaceutical assessments. In this regard, bixin-solid lipid nanoparticles (SLN) have been reported to enhance bixin's stability, sustained release, and cellular uptake.24 Also, biocompatible bixin-loaded polycaprolactone (PCL) nanofibre formulations have shown an initial 30–40% release within the first 10 hours and a sustained continuous 100% release until 14 days in in vivo models25 making bixin a promising natural bioactive compound to be screened as a drug target for photoaging.
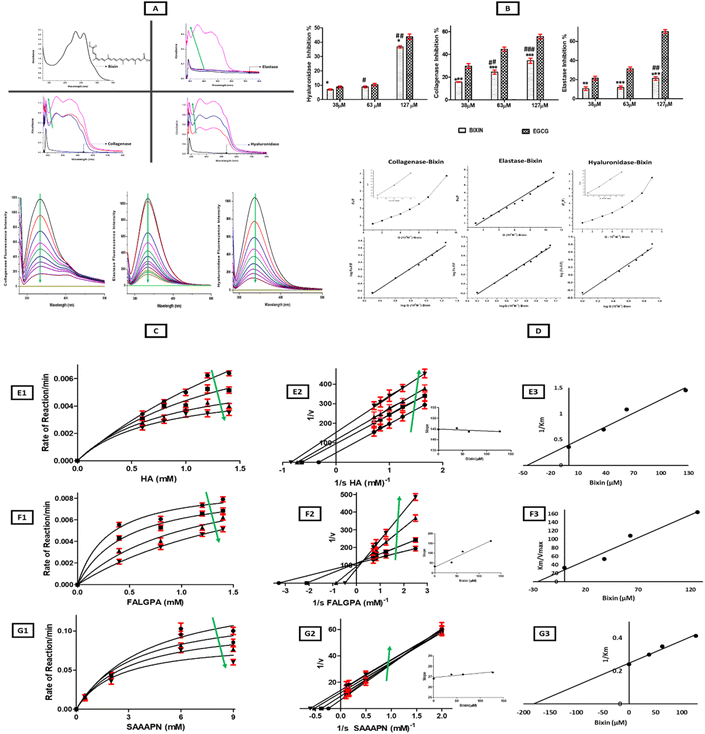 |
| Fig. 1 Spectroscopic assays: binding, interaction, and inhibitory potential of bixin on skin-aging-related enzymes. A: hyperchromic shift in the absorbance intensity of collagenase, elastase, and hyaluronidase on titration with bixin. The chemical structure and UV of bixin alone has also been shown. B: anti-hyaluronidase, anti-collagenase, and anti-elastase activity of bixin in comparison to EGCG (positive control). All values are expressed as mean ± SD (n = 3). *P < 0.05, **P < 0.01, ***P < 0.001 vs. EGCG; #P < 0.05, ##P < 0.01, ###P < 0.001 vs. Bixin dosage. C: quenching of collagenase, elastase, and hyaluronidase fluorescence intensity on titration with increasing concentration of bixin. D: Stern–Volmer plots (top row) and double logarithmic plots (bottom row). Crocin alone did not show any fluorescence as depicted by the straight line above the x-intercept in all plots. E, F and G: Michaelis–Menten (E1, F1 and G1) and Lineweaver–Burk plot (E2, F2 and G2) illustrate the inhibition kinetics of bixin (0 to 127 μM) on hyaluronidase (E1–E3), collagenase (F1–F3), and elastase (G1–G3). Data are expressed as mean ± SD (n = 3). Secondary plots E3, F3 and G3 have been used to determine the dissociation constant Ki. In all assays the increase in the bixin concentration is denoted by the direction of the green arrow. | |
However, the inhibitory effect of bixin on the activity of enzymes such as collagenase, hyaluronidase, and elastase has not yet been studied. Interaction studies between plant-based secondary metabolites such as bixin and skin aging-related enzymes would highlight the mechanism of interaction, binding and inhibition and thus expand their scope as nutraceuticals. Moreover, collagenase, elastase and hyaluronidase are involved in several other biochemical pathways apart from skin aging. Therefore, the findings of this study could pave the way for future research on bixin's therapeutic adequacy on health-related ailments linked to these pathways.
Here, to determine the mechanism of inhibition of bixin on collagenase, elastase and hyaluronidase activity, multiple assays were performed with spectroscopic tools such as absorption spectroscopy, fluorescence spectroscopy, enzyme inhibition assays and enzyme kinetics studies. The impact of bixin on reactive oxygen species (ROS) production and matrix metalloprotease 1 (MMP1) activity in UVA treated human dermal fibroblast (HDF) cells was assessed by flow cytometry and confocal microscopy. To validate the in vitro results, in silico molecular docking and simulation dynamics studies were performed. Furthermore, the inhibitory action of bixin on skin aging was studied on UVA treated dorsal skin of C57BL/6 mice and analysed for changes in skin morphology, collagen, and elastic tissue. Also, studies on bixin mediated changes in the activity of MMP1, collagenase, elastase, hyaluronidase, and oxidative stress markers such as malonaldehyde (MDA) and glutathione peroxidase (GSH-PX) in UVA treated mice skin were carried out. Thus, this work aims to widen the therapeutic and cosmeceutical potential of the natural, plant-derived phytochemical bixin, as a novel anti-skin aging agent.
2. Results and discussion
2.1. Absorbance studies: hyperchromic shift in skin aging-related enzymes on interaction with bixin
Absorption spectroscopy has been utilized as an efficient tool to perform a preliminary investigation to observe the interaction of target compounds with bioactive plant metabolites.26 The absorption spectra of collagenase, elastase, and hyaluronidase showed an absorption band centred at 270–280 nm, indicating the presence of amino acids tryptophan and tyrosine while bixin demonstrated a strong absorption band above 400 nm due to the presence of conjugated double bonds in its chemical structure (Fig. 1A). In a collisional encounter, the absorbance spectra will remain unaltered;27 however, a hyperchromic shift was observed in the spectra of enzymes on interaction with bixin as there was an increase in the absorbance intensity of enzymes due to probable alterations in the tertiary structure of the protein and complex formation between enzymes and bixin. In hyaluronidase, the increase in the absorbance intensity was dose (bixin) dependent. However, for collagenase and elastase, the hyperchromic shift was more prominent at higher concentrations of bixin. Therefore, bixin showed interaction and complex formation with all three enzymes under study (Fig. 1A). Also, on interaction with collagenase, elastase, and hyaluronidase, broadening of bixin's peak was observed which could be a result of intermolecular hydrogen bonding between the ligand molecule (bixin) and the binding site (enzymes) residues.28 The absorbance spectra of bixin have also been reported to be highly dependent on the solvent.29 The structure of bixin (Fig. 1A) has 25 carbon atoms and end groups of acid and methyl ester, enhancing its polarity compared to other carotenoids. Therefore, it has been reported to have a higher absorption coefficient in aprotic polar solvents such as DMSO, dichloromethane, acetone, chloroform, ethyl acetate, and dimethyl carbonate.29 Here, we titrated bixin with a fixed concentration of enzymes in their respective buffers (Tricine buffer for collagenase, Tris-HCL for elastase and Enzyme diluent for hyaluronidase (the details have been mentioned in the Experimental section; Enzyme inhibition assays (3.4)) which are predominantly aqueous, resulting in a blue shift of bixin's absorption spectra.30–32 Earlier, bixin has been reported to form aggregates in the presence of Ringer buffer due to low aqueous solubility resulting in hypochromism and a blue shift.33
2.2. Fluorescence studies: static quenching of skin aging-related enzyme fluorescence on interaction with bixin
Fluorescence quenching studies have been used to evaluate protein and drug interactions, providing abundant binding parameters.34 Fluorescence quenching is the reduction in the quantum yield or lifetime of a fluorophore on interaction with a molecule (quencher). It can be further classified as dynamic or static quenching. In dynamic quenching, a collisional encounter between the quencher and fluorophore takes place in the excited state; thereby it depopulates the excited state and influences the rate of fluorescence decay. However, static quenching results in complex (non-fluorescent) formation between the quencher and the fluorophore with increasing quencher concentration, thereby affecting the fluorescence yield in the steady state but has no impact on fluorescence decay rates of leftover non-complexed molecules.35
The fluorescence spectra of all three enzymes (collagenase, elastase, and hyaluronidase) at a concentration of 2 × 10−4 M was titrated with increasing bixin dosage (2 μl increment of bixin from a stock of 1 × 10−6 M) as depicted in Fig. 1C. The excitation wavelength (λex = 280 nm) for collagenase, elastase, and hyaluronidase was determined based on prior previously reported work.36 The maximum emission intensity of collagenase (λem = 334 nm), elastase (λem = 333 nm), and hyaluronidase (λem = 337 nm) decreased gradually on interaction with bixin. These findings are in line with earlier reports on bixin forming caroteno-protein complexes with proteins such as HSA33 and β-lactoglobulin,26 resulting in fluorescence quenching of these proteins. HSA and β-lactoglobulin are both transporter proteins and a high binding affinity of bixin with these proteins would assist in plasma transportation of bixin; however, the critical gap of probable off-target binding toxicity of bixin needs to be evaluated and bridged by integration of virtual, in vitro, and in vivo advanced screening, prediction, and identification strategies37
The Stern–Volmer quenching constants (KSV) were derived from eqn (2) and linear regression of F0/F plots against bixin (Fig. 1D). Elastase exhibited a linear F0/F plot against bixin, confirming static quenching; however, hyaluronidase and collagenase showed an upward concave curvature at higher concentrations of bixin indicating a combination of static and dynamic quenching (Fig. 1D) as described by eqn (1).26
| F0/F = (1 + KD[Q]) (1 + KS[Q]) | (1) |
where
KD and
KS are dynamic and static quenching constants. However, for lower bixin concentrations (<5 × 10
6 M
−1), the Stern–Volmer curves were linear (
Fig. 1D), and the data were analysed using
eqn (2). Also, for dynamic quenching, the maximal collisional quenching rate constant of quenchers has been documented as 2.0 × 10
10 M
−1 S
−1,
38 but
Kq for bixin was observed to be much greater than the
Kq for the scattering mechanism. This signifies that quenching for bixin–hyaluronidase and bixin–collagenase complexes is due to static quenching by complex formation and not dynamic collision quenching. This analysis is further supported by absorbance studies which are not characteristic of collision. Earlier, similar findings were observed for the interaction of bixin with β-lactoglobulin.
26 Also, on interaction with tyrosinase, bixin showed only static quenching by demolishing the hydrogen bonding network of tyrosinase, leading to enzyme rearrangement and conformational changes.
39,40 Similar interactions (electrostatic forces, hydrophobic interactions, hydrogen bonds) have been reported for other plant metabolites such as interactions of flavonoids with hyaluronidase.
36,41 The
Ksv values for six flavonoids analysed for their binding with hyaluronidase ranged from 1.8 × 10
4 M
−1 to 4.8 × 10
4 M
−1 (ref.
36) and ellagic acid showed a
Ksv value of 1.0 × 10
4 M
−1 on binding with elastase,
42 while the
Ksv values for binding of bixin with all three enzymes under study was much higher. The bigger
KSV and
Kq for the bixin–hyaluronidase complex (
KSV: 0.81 × 10
6 M
−1;
Kq: 8.1 × 10
13 M
−1 S
−1) indicates stronger binding between bixin–hyaluronidase in comparison to bixin-collagenase (
KSV: 0.651 × 10
6 M
−1;
Kq: 6.5 × 10
13 M
−1 S
−1), followed by the bixin–elastase complex (
KSV: 0.631 × 10
6 M
−1;
Kq: 6.31 × 10
13 M
−1 S
−1).
The binding constant (K) and the number of binding sites (n) were derived from Scatchard plot analysis (Fig. 1D and Table 1). Bixin exhibited a higher K (3.0 × 105 M−1) and n (1.4) value on interaction with hyaluronidase in comparison to collagenase (K: 2.39 × 105 M−1; n: 1.34) and elastase (K: 1.92 × 105 M−1; n: 1.27). The strong binding of bixin to these enzymes can also be explained by the anchoring of aromatic side chains of carotenoids to the hydrophobic regions of the protein28 as confirmed by the results of computational analysis discussed below in section 3.8.
Table 1 Binding parameters for skin aging related enzymes and bixin complexes
Complex |
K
SV (M−1) |
K
q (M−1 S−1) |
K (M−1) |
n
|
K
sv – Stern–Volmer quenching constant, Kq – biomolecular quenching rate constant, K – binding constant, n – number of binding sites |
Hyaluronidase–bixin |
0.81 × 106 |
8.1 × 1013 |
3.0 × 105 |
1.4 |
Collagenase–bixin |
0.651 × 106 |
6.51 × 1013 |
2.39 × 105 |
1.34 |
Elastase–bixin |
0.631 × 106 |
6.31 × 1013 |
1.92 × 105 |
1.27 |
2.3. Enzyme inhibition assays: bixin inhibited collagenase, hyaluronidase, and elastase activity in a dose-dependent manner
The absorbance and fluorescence studies confirmed a strong binding of bixin to skin aging-related enzymes. Therefore, to investigate its role as a potential anti-skin aging agent, anti-elastase, anti-collagenase, and anti-hyaluronidase assays were carried out and EGCG was selected as the positive control for all three enzymes owing to its well-documented activity as a plant-based inhibitor of skin aging-related enzymes.43 As depicted in Fig. 1B, bixin inhibited collagenase, elastase, and hyaluronidase, and this effect was more prominent at higher concentrations of bixin. Among the enzymes, bixin showed a higher inhibition % for hylauronidase followed by collagenase and elastase (Fig. 1B) which is in line with the binding affinity of bixin with these enzymes as observed in fluorescence studies. With an increase in bixin/EGCG concentration from 0–127 μM the inhibition percentage for hyaluronidase increased from 6.9% to 36.7% (bixin) and 8.8% to 43.4% (EGCG), while for collagenase the inhibition escalated from 15.7% to 34.3% (bixin) and 29.7% to 55.6% (EGCG), and for elastase the inhibition increased from 10.5% to 21.3% (bixin) and 21.5% to 70.4% (EGCG) respectively. Statistical analysis revealed a significant difference in the inhibition % mediated by bixin in comparison to positive control EGCG for all three enzymes under study. Also, a significant difference in the inhibition % of enzymes was observed with increasing bixin dosage for hyaluronidase, collagenase, and elastase (Fig. 1B).
To achieve a greater extent of activity as EGCG, a higher dosage of bixin will be required. The absorbance of bixin (red pigment) at higher concentrations was observed to mask the absorbance of the reaction rate, making it difficult to evaluate the inhibitory action of bixin on the enzymes. Similar findings have been reported for tyrosinase inhibition by bixin.40 Here, we observed substantial inhibitory potential of bixin even at lower concentrations which could be more profound if used in higher concentrations. Moreover, no toxic side effects of bixin have been reported even at high concentrations44 while EGCG has been reported to cause hepatotoxicity at higher dosage and other detrimental effects in several trials.45,46 Earlier, we reported a dose-dependent effect of bixin on tyrosinase inhibition resulting in the loss of tyrosinase's helical structure, polypeptide unfolding and exposure of the hydrophobic region.40 Thus, to study the probable binding site of bixin for collagenase, elastase, and hyaluronidase. Molecular docking and simulation dynamics studies were carried out to study the interaction and stability of bixin-enzyme complexes and the results have been discussed in section 3.8. Also, the mode of inhibition of bixin was analysed by enzyme kinetics studies as discussed below.
2.4. Enzyme kinetics studies: bixin, an uncompetitive inhibitor of hyaluronidase and elastase and a competitive inhibitor of collagenase
The kinetic studies showed the changes in the reaction rate of enzyme–substrate (ES) complexes under study in the presence of bixin. In our previous work, kinetic studies on the interaction of bixin with tyrosinase revealed the inhibitory mechanism as a mixed-type inhibition.40 Here, in the case of hyaluronidase and elastase, the Km and Vmax values decreased in the presence of bixin as shown in Michaelis–Menten (MM) plots, while the Lineweaver Burk (LB) plots yielded parallel lines to the enzyme–substrate plot, with a higher Y-intercept (lower Vmax) with augmented bixin concentration confirming uncompetitive inhibition of hyaluronidase and elastase activity by bixin (Fig. 1E1–E3 and G1–G3). In the case of hyaluronidase, the Vmax and Km values were 0.01946 μmol M−1 and 2.817 mM in the absence of bixin, which reduced to 0.00478 μmol M−1 and 0.6905 mM with an increase in bixin dosage (0–127 μM). Elastase showed a significant difference in Vmax (P < 0.05) and Km (P < 0.05) with increasing bixin concentrations (0–127 μM) (Table 2). The slope of the lines in the LB plot of bixin–elastase and bixin–hyaluronidase complexes showed no significant difference even at increasing concentrations of bixin, thereby reaffirming the inhibitory role of bixin as an uncompetitive inhibitor.47 As an uncompetitive inhibitor, bixin probably reduced the total enzyme–substrate (ES) complex with the formation of the ES–bixin complex, which reduced Vmax and Km.48 This type of inhibition is common in reactions involving two or more substrates/products49 as here, when hyaluronidase acts on HA, it is broken down into mono and disaccharides and small HA fragments. On the other hand, bixin showed competitive inhibition of collagenase activity as indicated by a significant difference (P < 0.05) and increase in Km from 0.3043 mM to 2.064 mM and almost unaltered and no significant difference in Vmax values (Table 2) as depicted by intersecting lines at the Y-intercept with cumulative bixin dosage (Fig. 1F1–F3). The slope of the LB plot of bixin-collagenase interaction showed a significant difference (P < 0.05) and increased steadily with augmented bixin dosage (Table 2) confirming the binding of bixin to the free enzyme.50 The dissociation constant Ki was observed to be lowest for collagenase (28.4 μM), followed by hyaluronidase (48.6 μM), and elastase (180.2 μM), respectively. Since lower the Ki, the tighter the binding of the inhibitor to the enzyme, therefore, these results again confirm an enhanced binding of bixin to collagenase and hyaluronidase in comparison to elastase.
Table 2 Enzyme kinetics studies: Bixin's mode of inhibition is highlighted based on Vmax and Km derived from the Michaelis–Menten (MM) plot, slope (Km/Vmax) of the Lineweaver–Burk (LB) plot, and Ki for hyaluronidase, collagenase, and elastase in the presence of bixin
ES complex |
MM plot Vmax (μmol M−1) |
p-Value |
MM Plot Km (mM) |
p-Value |
LB Plot slope (Km /Vmax) |
p-Value |
Mode of inhibition |
HYALURONIDASE-BIXIN |
No Inhibitor |
0.01946 |
0.1 |
2.817 |
0.1 |
144.7 |
0.6 |
Uncompetitive inhibition |
Bixin 38 μM |
0.0099 |
1.446 |
146.0 |
Bixin 63 μM |
0.00645 |
0.9273 |
143.7 |
Bixin 127 μM |
0.004780 |
0.6905 |
144.4 |
COLLAGENASE-BIXIN |
No Inhibitor |
0.00925 |
0.06 |
0.3043 |
0.02 |
32.8 |
0.02 |
Competitive inhibition |
Bixin 38 μM |
0.00899 |
0.4744 |
52.7 |
Bixin 63 μM |
0.01078 |
1.166 |
108.1 |
Bixin 127 μM |
0.01260 |
2.064 |
163.8 |
ELASTASE-BIXIN |
No Inhibitor |
0.1536 |
0.02 |
4.177 |
0.04 |
27.19 |
0.5 |
Uncompetitive inhibition |
Bixin 38 μM |
0.12854 |
3.349 |
26.05 |
Bixin 63 μM |
0.1079 |
2.865 |
26.5 |
Bixin 127 μM |
0.08745 |
2.424 |
27.7 |
2.5. Cell culture studies: bixin played a cytoprotective role, suppressed UV induced ROS, and inhibited MMP1 expression in UV-treated HDF cells
The results of the MTT assay (Fig. 2A), showed the cytoprotective role of bixin against UVA treated HDF cells as bixin increased the cellular viability in a dose dependent manner. The HDF cells were divided into five groups to assess the cytoprotective action of bixin. NC (negative control; no treatment group), V (vehicle; treatment with 10% DMSO (bixin vehicle) + UVA), BIX 1 (treatment with bixin (62.5 μM) + UVA), BIX 2 (treatment with bixin (125 μM) + UVA) and BIX 3 (treatment with bixin (250 μM) + UVA). The cell viability in irradiated HDF cells increased from 41.43% in the V group to 95.69% upon treatment with increasing bixin dosage (BIX 1-BIX 2-BIX 3). Earlier, the cytoprotective activity of bixin in keratinocytes and fibroblasts via the activation of the NRF2 pathway (master switch of the cellular antioxidant response) has been reported.51 Here, statistically significant difference in cellular viability was observed between NC vs. V groups (p < 0.001) and V vs. BIX 1, BIX 2, and BIX 3 groups (p < 0.001) emphasizing the cytoprotective role of bixin in UV treated HDF cells (Fig. 2A). The flow cytometry assay showed that bixin suppressed the UV light-induced ROS levels in HDF cells, and the effect was more significant at a higher bixin dosage. (Fig. 2B1–B3). In the V group, 91.73% increase in intracellular ROS in UV-treated HDFs was observed, which reduced to 15.4% on treatment with bixin (BIX 1/BIX 2/BIX 3). Also, a significant difference was observed in ROS levels in NC vs. V, BIX 1, BIX 2, and BIX 3 (p < 0.001) and V vs. BIX 1, BIX 2, and BIX 3 (p < 0.001) groups (Fig. 2B3). Similar results in HaCaT keratinocyte cells were examined by flow cytometry and bixin was found to reduce ROS-generated oxidative stress induced by UV radiation.52 These findings along with the results of the MTT assay confirm the high antioxidant potential of bixin, an essential property of an anti-skin aging agent to combat against environmental stressors resulting in an early onset of photoaging.
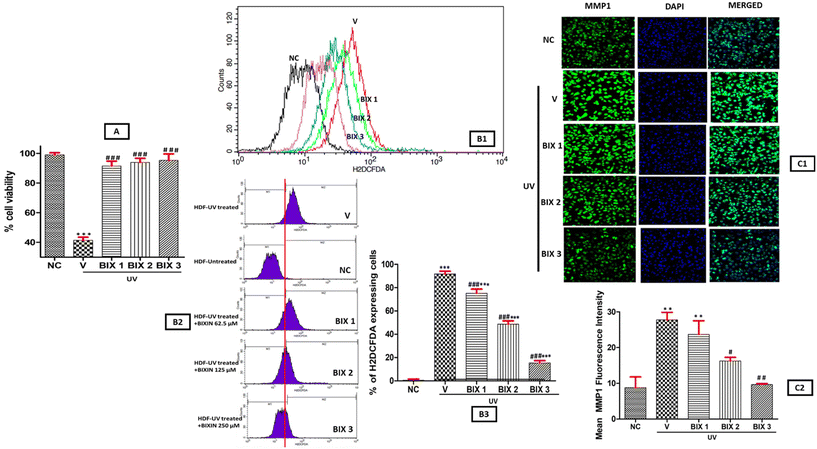 |
| Fig. 2
In vitro assays: A: cell viability % of UV-treated HDF cells in the presence of bixin. B1: an overlay of ROS generation in UV-treated HDFs with increasing bixin dosage. B2: the relative ROS generated in NC, V, BIX 1, BIX 2 and BIX 3. B3: cellular count has been plotted versus the dichlorofluorescein fluorescence detected by FL-2 channel. C1: DAPI and merged confocal images of the cells expressing MMP-1 in UV-treated HDF cell with and without bixin treatment. C2: the bar diagram denotes MMP-1 fluorescence intensity in UV-treated HDFs in the presence of increasing bixin concentrations. All values are expressed as mean ± SD (n = 3). *P < 0.05, **P < 0.01, ***P < 0.001 vs. NC; #P < 0.05, ##P < 0.01, ###P < 0.001 vs. V. | |
UV radiation is one among several other stressors promoting MMP1 expression, a master regulator of photoaging. MMP1 activation results in the degradation of collagen, accelerating skin aging. The elastase enzyme associated with elastin breakdown is also activated by MMP1. Therefore, MMP1 inhibitors possess tremendous potential as anti-aging compounds.22,23 In the spectroscopic assays, bixin inhibited elastase, hyaluronidase, and collagenase. Therefore, to understand the mechanism of action of bixin, we investigated the inhibition of MMP1 activity by bixin in UV-treated HDF cell lines via confocal microscopy. Our findings showed that the inhibitory action of bixin on MMP1 expression was dose-dependent, as depicted in Fig. 2C1 and C2. The fluorescence intensity of MMP1 in the NC group was observed to be 8.8% which increased steadily on UV exposure in the V group to 27.8%. On treatment with increasing bixin, MMP1 expression reduced to 9%. A statistically significant difference in MMP1 expression was observed between NC vs. V and NC vs. BIX 1 (p < 0.01), V vs. BIX 2 (p < 0.05) and V vs. BIX 3 (p < 0.01). Therefore, these results suggest the mechanism of action of bixin as a potential anti-skin aging agent is probably due to its potent antioxidant properties and inhibitory action on the expression of MMP1.
2.6. Virtual insights: molecular docking and dynamics studies concur spectroscopic and cell culture studies
Molecular docking and dynamics studies revealed stable complexes of bixin with collagenase, elastase, and hyaluronidase. The docking results showed better binding energy for bixin than EGCG (positive control) with all three enzymes. The docking posture and binding affinity (kcal mol−1) of all three enzymes complexed with bixin/EGCG has been depicted in Fig. 3-3.1. Table 3 describes the results generated from docking studies, highlighting the types of bonds generated during bixin/EGCG interaction with number of amino acid residues of collagenase, elastase, and hyaluronidase. The RMSD pattern of collagenase complexed with EGCG increased to ∼3 nm until 85 ns and converged, whereas the collagenase–bixin complex increased to ∼1.5 nm until 38 ns and converged (Fig. 3-3.2-A). The RMSD pattern of elastase complexed with bixin increased to ∼0.3 nm until 35 ns and converged, whereas the elastase–EGCG complex increased to ∼0.25 nm at 55 ns and converged (Fig. 3-3.2-B). The RMSD pattern of hyaluronidase complexed with EGCG increased to ∼0.5 nm from 5 ns and maintained its convergence until 100 ns, whereas the hyaluronidase–bixin complex increased to ∼0.4 nm at 25 ns and converged (Fig. 3-3.2-C). The gmx gyrate (Rg) plugin within GROMACS was used to investigate the protein compactness of all docked complexes. The collagenase–EGCG complex showed a huge deviation of ∼5.6 nm at 85 ns, resulting in the least compactness, whereas the collagenase-bixin complex exhibited a slightly less deviation ∼4.8 ns at 15 ns showing increased compactness upon bixin binding (Fig. 3-3.2-D). The case of elastase-bixin/EGCG complexes revealed almost similar Rg patterns throughout the simulation, resulting in significant compactness of the protein upon bixin/EGCG binding (Fig. 3-3.2-E). The hyaluronidase–EGCG complex exhibited a higher deviation of ∼2.35 nm throughout the simulation, whereas the hyaluronidase–bixin complex showed a minimal deviation of ∼2.3 nm, resulting in better compactness of the complex (Fig. 3-3.2-F). The H-bond between all the complexes was analysed with the help of the gmx H-bond from GROMACS. The results from the H-bond investigation showed that all the complexes exhibited similar H-bonds upon bixin/EGCG binding (Fig. 3-3.3 A (collagenase)-B(elastase)-C(hyaluronidase)). In terms of simulation results, all three enzymes bound to bixin showed significant inhibition compared to EGCG binding. However, the elastase–bixin complex showed a slightly higher deviation than the elastase–EGCG complex suggesting that the binding of EGCG has a better structure stabilization with the elastase protein.
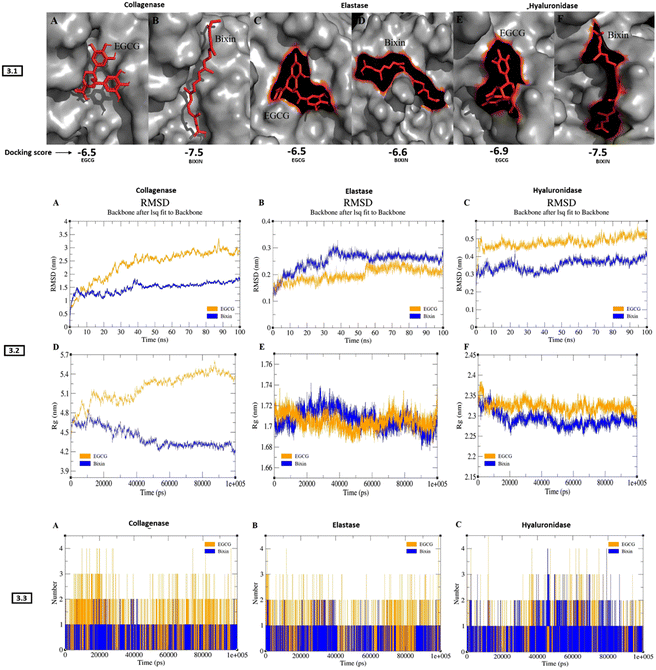 |
| Fig. 3 In silico assays: 3.1: 3D docking poses of bixin/EGCG-enzyme complexes (A) collagenase–EGCG complex; (B) collagenase–bixin complex; (C) elastase–EGCG complex; (D) elastase–bixin; (E) hyaluronidase–EGCG complex; and (F) hyaluronidase–bixin complex. The grey surface model represents the enzyme and bixin/EGCG is represented in the red stick model. The binding affinity (kcal mol−1) has been mentioned below for each of the complex. 3.2: RMSD and Rg plots of bixin/EGCG–enzyme complexes. (A) RMSD of collagenase–bixin/EGCG; (B) RMSD of elastase–bixin/EGCG; (C) RMSD of hyaluronidase–bixin/EGCG; (D) Rg of collagenase–bixin/EGCG; (E) Rg of elastase–bixin/EGCG; (F) Rg of hyaluronidase–bixin/EGCG. The x-axis represents the time in ns/ps; y-axis represents RMSD/Rg values. 3.3: H-bond plots of bixin/EGCG–enzyme complexes. (A) H-bond of the collagenase–bixin/EGCG complex; (B) H-bond of elastase–bixin/EGCG complex; (C) H-bond of the hyaluronidase–bixin/EGCG complex. The time in ps is represented in the x-axis; H-bond numbers between collagenase, elastase, hyaluronidase and bixin are represented in the y-axis. The yellow pattern refers to EGCG while the blue pattern denotes bixin. | |
Table 3 Molecular docking scores and amino acid residues involved in binding of enzyme-bixin/EGCG complexes
Protein |
Ligand |
Docking score (KJ mol−1) |
Amino acid residues bound to bixin/EGCG |
Type of bonds |
Collagenase |
Bixin |
−7.5 |
LYS A:741, PHE A:733, TYR A:618, HIS A:615, LYS A:667, PRO A:572, LEU A:664, LEU A:571, LYS A:734, PRO A:623, TRP A:736, ASP A:737, GLY A:731, MET A:622, LYS A:730, GLU A:619 |
van der Waals, hydrogen bonds, carbon–hydrogen bonds, alkyl bonds, pi–alkyl bonds |
EGCG |
−6.5 |
LYS A:620, VAL A:570, GLU A:619, SER A:568, GLY A:569, ASP A:744, ARG A:451. SER A:740, PHE A:765, TYR A:618, TRP A:736, MET A:622. PHE A :733, LYS A:741, ASP A:737 |
Hyaluronidase |
Bixin |
−7.5 |
TYR A:99, TYR A:100, THR A:158, PHE A:154, TYR A:225, SER A:91, ASP A:114, TYR A:97, ALA A:147, GLU A:146, LYS A :159, ASP A:157, TRP A:156, TYR A:223, ASP A:226, PRO A:237 |
EGCG |
−6.9 |
THR A:158, ASN A:218, ARG A:149, GLU A:146, TYR A:225, ASP A:226, TYR A:223, PRO A:237, LYS A:159, PHE A:154, ASN A:155, TRP A:156, ASP A:157 |
Elastase |
Bixin |
−6.6 |
SER A:170A, TYR A:171, LYS A:224, TRP A:172, SER A:217, ALA A:99A, THR A:175, PHE A:215, ARG A:217A, VAL A:99, VAL A:216, SER A:214, GLN A:192, HIS A: 57, SER A:195, GLY A:193, CYS A:42 |
EGCG |
−6.5 |
GLY A:193, HIS A:57, GLN A:192, THR A:96, TRP A:94, VAL A:99, VAL A:216, SER A:195, SER A:214, PHE A:215, THR A:213, THR A:226, ASP A:194, GLY A:190, CYS A:191, CYS A:220, CYS A:58, THR A:41, ARG A:61 |
2.7.
In vivo outcomes
2.7.1. Bixin showed photoprotective effects on the growth of hair follicles in UV exposed mouse skin.
The hair growth cycle of C57BL/6 mice is a time-synchronized hair growth cycle. The depilated mouse skin is pink in the telogen phase (Fig. 4A1) and turns from grey to black with anagen phase initiation and returns to grey with the catagen phase followed by pink (telogen phase). In this study, the hair follicles for NC, B+, B++ and B+++ groups entered the anagen phase on day 9 until day 18 (skin changes from grey-black-grey), followed by the catagen and telogen phase (day 18–day 25). However, the hair cycle was delayed in the V group in comparison to other four groups as shown in Fig. 4A1. Also, in comparison to other groups, the hair growth in the V group was spot-like, followed by a very small patch of hair growth by day 18, wherein the hair regrowth was seen on the entire depilated dorsal side of the mice for the other four groups. Earlier, similar results have been reported on exposure of depilated C57BL/6 to UVA exposure.53 Also, statistically significant difference (p < 0.01) was observed between NC vs. V groups with respect to mean number of hair follicles/2400 μm (for each skin sample, hair follicle/2400 μm at five random places in the sample was tabulated) (Fig. 4A4). In addition, the number of hair follicle in groups B+, B++ and B+++ showed an increasing trend in the size and number of hair follicles with increasing bixin dosage (Fig. 4). Moreover, significant difference in the number of hair follicles was observed between V vs. B++ and B+++ groups (p < 0.05). Fig. 4 shows a longitudinal (A2) and horizontal slice (A3) of the skin sample of various groups obtained from H&E staining depicting the size and number of hair follicles. The least number of hair follicles were observed for the V group, while the number of hair follicles in the NC group were comparable to B+, B++ and B+++ groups (Fig. 4A2 and A3). UVA has been well reported to cause injury to the hair follicles in the dermis because of its longer wavelength and attenuate hair follicle related stem cells and induce photoaging by miniaturization of hair follicles.53 These results indicate the photoprotective role of bixin against UVA on mice skin with no significant changes in the hair follicle cycle in comparison to the NC group.
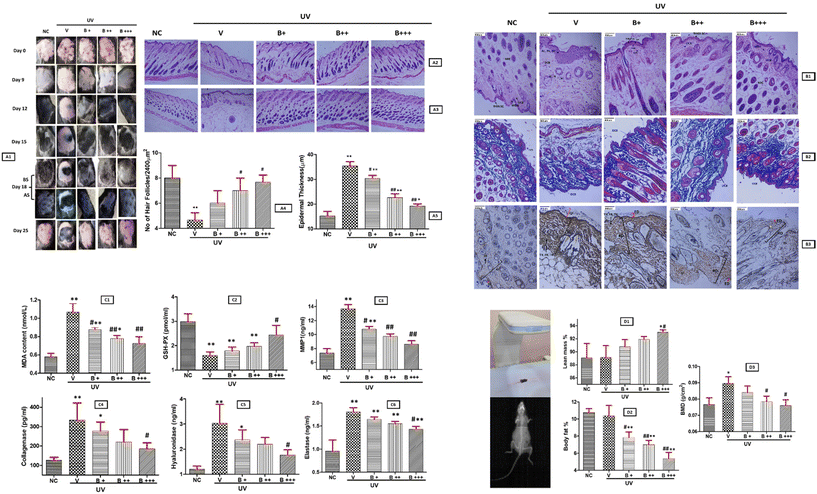 |
| Fig. 4
In vivo assays: A1: effect of bixin on hair growth cycle in mice (BS: before shaving; AS: after shaving). A2 and A3: longitudinal (A2) and horizontal (A3) slice of skin depicting the number and size of hair follicles (scale bar: 280 μm). A4 and A5: graphical representation of the mean number of hair follicles (A4) and mean epidermal thickness (A5). B1: H&E staining of the dorsal skin of mice (NHF: normal hair follicles, OCB: organised collagen bundles, SC: stratum corneum, WDEJ: wavy dermo-epidermal junction, AC: acanthosis, PK: parakeratosis, HP: hyperplasia, DCB: disorganised collagen bundles, IC: inflammatory cells, N: neutrophils, FDEJ: flat dermo-epidermal junction, MDI: mild dermal inflammation, SC: subcutaneous). B2: Masson's Trichrome staining of the dorsal skin of mice. B3: reticulin staining of the dorsal skin of mice (ED: epidermis, RD: reticular dermis, EF: elastic fibres, TN: tangled, FR: fractured, TH: thickened). B1–B3: (scale bar: 800 μm). C1 and C2: effect of bixin on oxidative stress markers MDA (C1) and GSH-PX (C2) in UVA treated mice skin. C3–C6: Inhibitory action of bixin on skin aging-related enzymes in UVA treated mice skin. MMP1(C3), collagenase (C4), hyaluronidase (C5) and elastase (C6). D1–D2–D3: impact of bixin on Dexa scan parameters in UVA treated mice skin. D1: lean mass %, D2: body fat %, D3: BMD. All values are expressed as Mean ± SD (n = 3). *P < 0.05, **P < 0.01, ***P < 0.001 vs. NC; #P < 0.05, ##P < 0.01, ###P < 0.001 vs. V. | |
2.7.2. Bixin acted as a UVA screen for epidermis, dermis, collagen, and elastin.
The H&E, Masson's Trichrome and Reticulin staining revealed the UVA shielding effect of bixin on mice skin. The H&E staining highlighted the changes in skin epidermis and dermis in the 5 mice groups under study. In comparison to the NC group, a significant increase in epidermal thickness (for each skin sample, mean of epidermal thickness at five random places in the sample was tabulated) was observed in the V group (p < 0.01) (Fig. 4A5) post UV exposure. However, with an increase in the bixin dosage, the reduction in the epidermal thickness was found to be statistically significant between V vs. B++ and B+++ (p < 0.01) groups (Fig. 4A5). (Fig. 4B1) A wavy dermo-epidermal junction, thin stratum corneum, organised collagen bundles and normal hair follicles were illustrated by H&E staining in the NC group in comparison to the V group wherein the epidermis showed acanthosis, parakeratosis and hyperplasia and the dermis was seen with scattered inflammatory cells (lymphocytes and few neutrophils), reduced number of hair follicles and disordered collagen bundles. Bixin was found to screen the adverse effects of UV and depicted visible changes in H&E staining at high dosage (B+++) as the epidermis showed thickened granular layer alone and no atrophy was observed, while the dermis was presented with ordered collagen bundles along with normal distribution of hair follicles (Fig. 4B1). Similar findings have been reported for quercetin and EGCG as natural antioxidants involved in shielding the skin54,55 from epidermal hyperplasia, inflammatory dermal infiltration, and dysmorphic hair cycles. The Masson's Trichrome staining revealed the effect of UVA on the organization of collagen bundles (Fig. 4B2). UVA completely disorganised the collagen bundles in the V group (blue), however, bixin was found to shield the mice skin as the collagen bundles were observed to be organised at a higher bixin dose (B+++). The reticulin staining demonstrated the elastic fibres (brown) in the reticular dermis. UVA led to tangled, thickened, and fractured elastic fibres in the reticular dermis in comparison to a random arrangement of elastic fibres in NC and B+++ groups (Fig. 4B3). The detrimental changes in the epidermis, dermis and subcutaneous layers of skin, distribution of hair follicles, organization of collagen and elastin were found to be shielded at higher doses of bixin. Thus, these results further confirm the antioxidant properties and probable inhibitory action of bixin on collagenase and elastase enzyme.
2.7.3 Bixin curtailed UVA generated oxidative stress and inhibited the activity of skin aging-related enzymes in mice skin.
The underlying mechanism of ROS generation in skin is due to lipid peroxidation of the cellular membranes in the skin because of UV exposure. Here, we analysed, the level of oxidative stress in mice skin post UVA exposure by an estimation of malonaldehyde (MDA), the end product of lipid peroxidation and glutathione peroxidase (GSH-PX) involved in breaking down lipid hydroperoxides. The UVA exposure of mice skin, increased the (MDA) levels in NC vs. V group (p < 0.01) while a significantly reduced activity of glutathione peroxidase (GSH-PX) was observed between NC vs. V groups (p < 0.01) (Fig. 4C1 and C2). However, with increasing bixin dosage, a significant improvement in the oxidative stress related markers was observed. Bixin was found to significantly reduce MDA levels in comparison to the control; NC (p < 0.01; NC vs. B+, p < 0.05; NC vs. B++) and V (p < 0.05; V vs. B+, p < 0.01; V vs. B++, p < 0.01; V vs. B+++) groups (Fig. 4C1). Also, an improvement in the activity of GSH-PX was observed with increasing bixin dosage compared to the control groups NC (p < 0.01; NC vs. B+, p < 0.01; NC vs. B++) and V (p < 0.05; V vs. B+++) groups (Fig. 4C2). Earlier, topical, and intraperitoneal administration of bixin has also been reported for its NRF-2 dependent (master regulator of antioxidant response) protection against skin photodamage from UV exposure21,52
As detected earlier in HDF cells, UVA exposure activated the skin aging-related enzymes MMP1, collagenase, elastase and hyaluronidase showing a significant upscale in the V group in comparison to NC (p < 0.01) for all the four enzymes. Bixin supplementation inhibited the enzymes significantly in a dose dependent manner. MMP-1 inhibition was observed to be significant between V vs. B++ and V vs. B+++ groups (p < 0.01) (Fig. 4C3). In the case of collagenase and hyaluronidase statistically significant difference between V and bixin treated groups, were observed; NC vs. B+ (p < 0.05) and V vs. B+++ (p < 0.05) (Fig. 4C4 and C5). Elastase inhibition was found to increase with increasing bixin dosage and significant difference between NC vs. B+, B++ and B+++ groups (p < 0.01) was observed, while in comparison to the V group a significant difference was observed for the V vs. B+++ group (p < 0.05) (Fig. 4C6). These results validate our earlier findings obtained via in silico and in vitro studies as discussed above.
2.7.4. DEXA confirmed the protective role of bixin on cardiometabolic health.
UV radiation has been reported to enhance the bone mineral density (BMD)56 as 90% of vitamin D required for human beings is generated from sun exposure. The DEXA scan of the mice revealed a significant increase in BMD post UVA exposure in V, B+, B++ and B+++ groups in comparison to the NC group (Fig. 4D3). The increase in BMD was significant (p < 0.05) in the NC vs. V group, while the reduction in BMD was significant (p < 0.05) between V vs. B++ and B+++ vs. V groups. Thus, bixin significantly protected the impact of UVA on bone mineral density in a dose dependent manner (Fig. 4D3). In addition, increasing bixin supplementation also showed significant reduction in body fat percentage in NC vs. B+, B++, B+++ groups (p < 0.01) and V vs. B++ and V vs. B+++; (p < 0.05) (Fig. 4D2). Also, significant improvement in lean mass (p < 0.05) was observed for B+++ in comparison to control mice groups (NC and V) (Fig. 4D1). Since the control groups, NC and V showed no significant difference in fat and lean mass %, the changes in metabolic parameters revealed by DEXA were attributed to bixin alone. This may have future implications to study the role of bixin in improving the impact of ageing on cardiometabolic health. Earlier, bixin has also been reported to ameliorate cardiac injury in rats,57 antiatherogenic effects in rabbits,58 and improved the metabolic parameters in C57BL/6 male mice.59 The anti-obesity and anti-hyperlipidemic efficiency of bixin has been associated with its antioxidant properties and inhibitory action on fat and carbohydrate digestion enzymes.60 Our results are in concurrence with these studies and since the fat% reduced with increasing bixin dosage, the lean mass % improved with a significant difference between the two control groups NC and V vs. B+++ (p < 0.05) (Fig. 4D1–D3).
3. Experimental
3.1. Materials
Preparation of bixin: bixin was purchased from Sigma-Aldrich and prepared freshly prior to each use by dissolving in 10% DMSO.
The source of enzymes and their substrates were chosen based on the existing literature evaluating the efficacy of skin aging-related agents.2,61 Elastase, porcine pancreatic (E.C-3.4.21.36), N-succinyl-Ala-Ala-Ala-p-nitroanilide (SAAAPN), collagenase, Clostridium histolyticum (E.C-3.4.23.3), N-[3-(2-furyl)acryloyl]-Leu-Gly-Pro-Ala (FALGPA), hyaluronidase, bovine testes (E.C-3.2.1.35), hyaluronic acid sodium salt from rooster comb (HA), EGCG and human dermal fibroblast (HDF) cell line were purchased from Sigma-Aldrich. Human anti-MMP-1 antibody and secondary antibody-FITC were acquired from Novus Biologics. The assays for the detection of MMP1, collagenase, elastase, hyaluronidase, MDA, GSH-PX levels in mice skin were performed using standard ELISA Kits purchased from Abbkine.
3.2. Absorbance spectroscopy
The absorption spectra were recorded on a spectrophotometer (JASCO V-730). The concentration of enzymes collagenase, hyaluronidase and elastase was fixed at 2 × 10−4 M and shifts in the UV spectra of enzymes on titration with 2 μl increment of bixin (1 × 10−6 M) was recorded to ascertain the probable mode of interaction.
3.3. Fluorescence studies
The fluorescence studies were executed as per the experimental design of Zeng et al. (2015) with a few modifications10 and were measured on a spectrofluorometer (FP-8200). The interaction of bixin with the enzymes was studied by tryptophan emission quenching experiments. The fluorescence spectra of collagenase, elastase, and hyaluronidase was measured at an excitation wavelength of 270–280 nm, and emission spectra between 290 and 450 nm was recorded at a fixed concentration of enzymes (2 × 10−4 M) titrated with 2 μl increment of bixin (1 × 10−6 M) and the quenching of enzyme emission signals was noted.36,62
Fluorescence quenching constant KSV was determined by the Stern–Volmer equation:
| F0/F = 1 + Kqτ0[Q] = 1 + KSV[Q] | (2) |
where,
F0 and
F are the fluorescence intensities of collagenase, elastase, and hyaluronidase in the absence and presence of bixin, [Q] is the bixin concentration,
KSV is the Stern–Volmer fluorescence quenching constant,
Kq is the quenching rate constant, and
τ0 is the average lifetime of tryptophan (10
−8 s).
For static fluorescence quenching interactions, the binding constant (K) and the number of binding sites (n) were estimated from the Scatchard equation:
| log(F0 − F/F) = log K + n log[Q] | (3) |
3.4. Enzyme inhibition assays
All enzyme inhibition assays were performed in triplicate, and inhibition % for elastase, hyaluronidase, and collagenase were calculated using the formula: | Enzyme inhibition activity (%) = (1 − B/A) × 100 | (4) |
where A and B refer to the enzyme activity in the absence and presence of the test sample bixin. Water and 10% DMSO were negative controls, while EGCG was the positive control for all enzyme inhibition assays.
3.4.1. Anti-collagenase assay.
The assay was performed as per the protocol of Thring et al. (2009)63 with some modifications. Collagenase was dissolved in 50 mM Tricine buffer (pH 7.5) to obtain 0.8 units per ml of the enzyme. 2 mM stock of substrate N-[3-(2-furyl)acryloyl]-Leu-Gly-Pro-Ala (FALGPA) was prepared in Tricine buffer. After the standardization of the experiment, a fixed quantity of the enzyme and buffer were incubated for 15 minutes with increasing concentrations of bixin ranging from 0–127 μM at 37 °C. With the addition of substrate FALGPA, the reaction was initiated, and after an incubation period of 20 minutes, absorbance at 340 nm was measured.
3.4.2. Anti-elastase assay.
A colorimetric assay was carried out based on Tu and Tawata's (2015) method with slight modifications.64 The substrate was dissolved in Tris-HCL buffer (0.1 M, pH 8.0) to obtain a concentration of 1 mM. Bixin concentrations ranging from 0–127 μM were incubated with the substrate for 10 minutes and the reaction was initiated by the addition of elastase (0.03 U ml−1) and incubation for 10 minutes following which the absorbance at 410 nm was measured.
3.4.3. Anti-hyaluronidase assay.
The anti-hyaluronidase activity of bixin was measured by a turbidimetric determination based on the Sigma protocol, with slight modifications.65 The enzyme hyaluronidase (1.50 U), pH 7, was prepared by dissolving it in the enzyme diluent (20 mM sodium phosphate,77 mM sodium chloride, 0.01% of BSA). The enzyme was incubated with bixin at concentrations ranging from 0–127 μM for 10 minutes and hyaluronic acid (HA) (0.3 mg ml−1) in sodium phosphate buffer at pH 5.35 was added to initiate the reaction and incubated for 45 minutes. Undigested HA was measured by mixing 1 ml of acidic albumin solution (pH 3.75) into the reaction mix and after 10 minutes the absorbance was read at 600 nm.
3.5. Enzyme kinetics studies
For enzyme kinetic studies, increasing concentrations of substrates HA, FALGPA (0–1.5 mM) and SAAAPN (0–9 mM) were incubated with their respective enzymes at a fixed concentration, standardised by enzyme inhibition assays earlier. The Vmax and Km obtained were studied in the presence of increasing concentrations of bixin (0–127 μM) and the inhibition type was analysed by plotting the Michaelis–Menten (MM) (5) and Lineweaver Burk (LB) plots (6). | 1/V0 = Km/Vmax × 1/S + 1/Vmax | (6) |
where V0 is initial velocity of the reaction, Vmax is the maximal reaction rate, S is the substrate concentration and Km the Michaelis–Menten constant.
The enzyme inhibition constant (Ki) was determined for uncompetitive and competitive inhibition by eqn (7) and (8).47
| 1/K′m = 1/Km × (1 + I/Ki) | (7) |
where
K′
m is the apparent
Km derived from the
x-intercept of the LB plot, by varying the inhibitor (bixin) concentration (
I).
| V = Vmax/1/Km + S × (1 + I/Ki) | (8) |
3.6. Cell viability and treatment
Human dermal fibroblasts (HDF) cells were cultured in high glucose DMEM, 10% FBS, penicillin (100 IU mL−1), and streptomycin (100 pg mL−1) at 37 °C. After reaching 80% confluency, the cells were detached with trypsin-EDTA and washed with DPBS. Then, 200 μl of the cell suspension was seeded at a cell density of 0.5 × 106 cells per well and allowed to grow for 12 to 16 hours. To set up an UVA irradiation model, after inoculation for 8–12 hours, the cells were irradiated with varying UVA irradiation intensities of 0, 3, 6, 9 and 12 J cm−2 (ref. 3) and based on cellular response a dosage of 9 J cm−2 UVA was fixed for treatment of HDF cells. Bixin cytotoxicity was evaluated by the MTT standard assay to determine the bixin dosage for the treatment of HDF cells.52 The experimental design comprised of five groups; NC (negative control; no treatment group), V (vehicle; treatment with 10% DMSO (bixin vehicle) + UVA), BIX 1 (treatment with bixin (62.5 μM) + UVA), BIX 2 (treatment with bixin (125 μM) + UVA), and BIX 3 (treatment with bixin (250 μM) + UVA).
3.6.1. Assessment of the cytoprotective role of bixin in UVA treated HDF cell lines: MTT assay.
The cellular viability and proliferation of HDFs in the presence of bixin (0–250 μM) was assessed post UVA exposure by the MTT assay. Post-treatment of HDF cells with UV, the spent media was removed and MTT reagent (0.5 mg mL−1) was added. To avoid light exposure, the plate was wrapped with aluminium foil and kept in the incubator for 3 hours, following which the MTT reagent was removed, and 100 μl of DMSO was added. The absorbance was read at 570 nm.
3.6.2. UVA light-induced ROS expression in HDF cell lines in the presence of bixin: flow cytometry.
To evaluate the impact of bixin on intracellular ROS levels post UVA exposure, the HDF cells were incubated with increasing bixin concentration (0–250 μM) at 37 °C prior to irradiation. After 24 hours, the HDF cells were stained at a density of 1 × 106 cells per ml with ROS indicator 2′,7′-dichlorodihydrofluorescein diacetate (H2DCFDA) followed by a light protected incubation period of 30 minutes. These cells were then inspected by flow cytometry at an excitation of 488 nm, and the emission at 535 nm was recorded.66
3.6.3. MMP1 expression in UVA treated HDF cell lines in the presence of bixin: confocal microscopy.
MMP1 expression in UVA-treated HDF cell lines following treatment with bixin was analysed by confocal microscopy. HDF cells were cultured and treated with bixin (0–250 μM) followed by exposure to UVA and the cells were fixed with 70% methanol at 4 °C for 20 minutes. The cells were washed twice with PBS and primary anti-MMP-1 antibody was added and incubated in the dark for 1 h. The unbound antibodies were washed with PBS, and goat anti-mouse IgG (H + L) secondary antibody-FITC was added and incubated for 30 minutes in the dark. The unbound antibodies were washed with PBS and DAPI was added. The cells were imaged under a confocal microscope, and the mean fluorescence intensity of MMP1 was measured using Image J software.
3.7 Molecular docking and simulation studies
The elastase, hyaluronidase, and collagenase protein sequences were downloaded from the UniProt database. The protein 3D structure for elastase was downloaded from the RCSB database (PDB ID: 1B0E). Since the crystal structures of hyaluronidase and collagenase were not available, we used I-Tasser to model the hyaluronidase and collagenase structures with protein sequences as an input.67 The AutoDock platform version 4.2 was used for the docking of bixin and EGCG (positive control), individually with elastase, hyaluronidase, and collagenase. Residues GLN448, ARG451, VAL452, SER568, GLY569, VAL570, TYR618, GLU619, MET622, ILE626, PHE733, TRP736, ASP737, SER740, LYS741, and ASP744 were the predicted binding sites for collagenase, whereas the residues HIS57, TRP172, THR175, CYS191, GLN192, GLY193, ASP194, SER195, THR213, SER214, PHE215, VAL216, and SER217 were the predicted active sites for elastase. For hyaluronidase, we predicted TYR90, ASP144, TYR217, TYR225, SER260, TYR262, ALA265, ASP307, and TRP336 residues as active sites. To investigate the structural changes and dynamic behaviour between elastase, hyaluronidase, and collagenase complexed with bixin and EGCG structures, molecular dynamic simulation was analysed for about 100 ns. Further graphical representation was plotted using Graphing, advanced computation, and exploration (GRACE) programme for the obtained molecular dynamics trajectories. The root mean square deviation (RMSD), hydrogen bond (H-bond), and radius of gyration (Rg) for all the structures were determined using the GROMACS package's gmx rms, gmx H-bond, and gmx gyrate tools after the MD trajectory files were completed and the protocol followed from our previous studies.68,69
3.8.
In vivo studies: experimental design and treatment
All experiments involving animals were approved by the Institutional Animal Ethics Committee (IAEC), VIT, Vellore; VIT/IAEC/20/DEC2021/07, and the experimental procedures were designed and carried out in accordance with the animal ethical guidelines. Fifteen, five-week-old female C57BL/6 mice were approved and purchased from the animal house, VIT, Vellore. The animals were housed in the animal facility at 50% humidity, 24 ± 2 °C and allowed to acclimatize to the animal house environment for a week before the commencement of the experiment. The bixin dosage in vivo was determined based on prior data reported on bixin administration and toxicity studies in mice.52,70–72 The mice were divided randomly into five groups. Group1 was the negative control (NC) and unexposed to UV and bixin treatment. The other four groups of mice were treatment groups. Group 2 (V) was treated with UVA and 10% DMSO (vehicle for bixin), group 3 (B+) was treated with UVA and bixin (100 mg kg−1), group 4 (B++) was treated with UVA and bixin (250 mg kg−1), and group 5 (B+++) was treated with UVA and bixin (500 mg kg−1). The hair on the dorsal skin (3 × 3 cm2) of all the mice was shaved and trimmed every 3 days. The treatment groups were exposed to a narrow spectrum UVA wavelength of 340–370 nm, with a peak at 365 nm, using a TL-D/18 W Philips lamp. Mice were placed in a closed box where they could move around freely and the distance between the UV lamp and the bottom of the box was 25 cm with exposure to UVA for a duration of 20 minutes (1 minimal erythemal dose, MED) five times in the first week and 2 MED three times per week during the following 3 weeks.53 After every UVA exposure the mice in the treatment group (V) were injected with 0.5 ml of 10% DMSO, while group 3, group 4 and group 5 mice were injected with increasing dosage of bixin via the intraperitoneal route. The visible changes in the mice skin and hair cycle were recorded. A day after the last UVA exposure the mice were sacrificed, and their skin samples were collected and divided into two parts. For all five groups, one part of the skin section was stored in 10% buffered formalin for Haematoxylin Eosin (H&E), Masson's Trichrome and Reticulin staining while the other part of the skin section was homogenised (4 °C, 3000 rpm for 10 minutes) in saline and stored at −20 °C, for the estimation of oxidative stress indicators malonaldehyde (MDA) and glutathione peroxidase (GSH-PX) and the enzymes under study; MMP-1, collagenase, elastase, and hyaluronidase using ELISA kits. Also, the impact of bixin was studied on the body composition and bone mineral density (BMD) of UVA treated mice using the gold standard for BMD assessment – DXA (dual energy X-Ray absorptiometry) Scanner – Hologic Discovery A series. Ketamine was administered as an anaesthetic agent to avoid the movement of mice during the scan.
3.9 Statistical analysis
Graphical representation and analysis were performed using the GraphPad Prism Software Version 5 and Origin Pro 8.5. The results have been presented as the mean ± SD of three independent experiments, each performed in triplicate. The difference between groups were considered significant at p < 0.05 by employing one-way analysis of variance (ANOVA) with Tukey's post hoc test.
4. Conclusion
This report highlights the pharmaceutical and cosmeceutical potential of bixin, an FDA approved food colourant, as a natural anti-photoaging agent. This is a therapeutically important finding as the results of this study taken together with our previous work on bixin confirmed its anti-tyrosinase activity, strong binding affinity with BSA and antiaging properties of apocarotenoids,18,39,40 indicating the potential usage of bixin as an anti-skin aging cosmeceutical. The molecular docking and dynamics simulation studies gave a virtual insight into the networking of bixin with skin aging-related enzymes indicating a strong binding of bixin to collagenase, elastase, and hyaluronidase, comparable to the positive control EGCG. The in vitro, spectroscopic and HDF cell culture studies strongly support the inhibitory potential of bixin on ROS production and skin aging. Furthermore, in vivo studies confirmed the potent antioxidant properties and UVA screening effect of bixin on skin. The in vivo findings also established the novel therapeutic role of bixin as a potent inhibitor of MMP1, collagenase, elastase, and hyaluronidase. These findings are a steppingstone towards future research prospects targeting bixin's mechanism of action and pharmaceutical potential on several other pathways linked to MMP-1, collagenase, hyaluronidase, and elastase. Moreover, this study provides a platform to explore other natural food-based phytochemicals with probable anti-skin aging potential.
Data availability
All the data described are contained within the manuscript.
Author contributions
Conceptualization: Siva Ramamoorthy and Leepica Kapoor; methodology: Leepica Kapoor, S. Udhaya Kumar, Sourav De, Sujithra Vijayakumar, and Nitin Kapoor; supervision: Ashok Kumar S. K., George Priya Doss C., and Siva Ramamoorthy; resources: Nitin Kapoor, Ashok Kumar S. K., and George Priya Doss C.; writing − original draft: Leepica Kapoor, S. Udhaya Kumar, George Priya Doss C., and Siva Ramamoorthy; writing – review, and editing: Siva Ramamoorthy.
Conflicts of interest
The authors declare that they have no conflicts of interest with the contents of this article.
Acknowledgements
This research did not receive any specific grant from funding agencies in the public, commercial, or not-for-profit sectors. The authors are thankful to the VIT management for their support and encouragement.
References
- J. C. Fussell and F. J. Kelly, Oxidative contribution of air pollution to extrinsic skin ageing, Free Radicals Biol. Med., 2020, 151, 111 CrossRef.
- R. Boran, Investigations of anti-aging potential of Hypericum origanifolium Willd. for skincare formulations, Ind. Crops Prod., 2018, 118, 290–295 CrossRef.
- Q. Sun, P. Liu, S. You, D. Zhao, C. Wang, J. Zhang, D. Wang and M. Li, Protective effects of LPL-EPS-02 on human dermal fibroblasts damaged by UVA radiation, J. Funct. Foods, 2021, 83, 104544 CrossRef.
- C. Battie, S. Jitsukawa, F. Bernerd, S. Del Bino, C. Marionnet and M. Verschoore, New insights in photoaging, UVA induced damage and skin types, Exp. Dermatol., 2014, 23, 7–12 CrossRef.
- C. T. Horng, H. C. Wu, N. N. Chiang, C. F. Lee, Y. S. Huang, H. Y. Wang, J. S. Yang and F. A. Chen, Inhibitory effect of burdock leaves on elastase and tyrosinase activity, Exp. Ther. Med., 2017, 14, 3247–3252 CrossRef PubMed.
- A. Huynh and R. Priefer, Hyaluronic acid applications in ophthalmology, rheumatology, and dermatology, Carbohydr. Res., 2020, 489, 107950 CrossRef CAS PubMed.
- M. Bilal and H. M. Iqbal, An insight into toxicity and human-health-related adverse consequences of cosmeceuticals—a review, Sci. Total Environ., 2019, 670, 555–568 CrossRef CAS PubMed.
- M. Bilal, S. Mehmood and H. M. N. Iqbal, The Beast of Beauty: Environmental and Health Concerns of Toxic Components in Cosmetics, Cosmetics, 2020, 7, 13 CrossRef CAS.
- L. Scotti, R. K. Singla, H. M. Ishiki, F. J. Mendonca, M. S. da Silva, J. M. Barbosa Filho and M. T. Scotti, Recent Advancement in Natural Hyaluronidase Inhibitors, Curr. Top. Med. Chem., 2016, 16, 2525–2531 CrossRef CAS PubMed.
- H.-j. Zeng, J. Ma, R. Yang, Y. Jing and L.-b. Qu, Molecular interactions of flavonoids to hyaluronidase: insights from spectroscopic and molecular modeling studies, J. Fluoresc., 2015, 25, 941–959 CrossRef CAS PubMed.
- C. Jiratchayamaethasakul, Y. Ding, O. Hwang, S.-T. Im, Y. Jang, S.-W. Myung, J. M. Lee, H.-S. Kim, S.-C. Ko and S.-H. Lee, In vitro screening of elastase, collagenase, hyaluronidase, and tyrosinase inhibitory and antioxidant activities of 22 halophyte plant extracts for novel cosmeceuticals, Fish. Aquat. Sci., 2020, 23, 1–9 CrossRef.
- K. Madan and S. Nanda,
In vitro evaluation of antioxidant, anti-elastase, anti-collagenase, anti-hyaluronidase activities of safranal and determination of its sun protection factor in skin photoaging, Bioorg. Chem., 2018, 77, 159–167 CrossRef CAS PubMed.
- A. J. Simkin, L. Kapoor, C. Doss, T. A. Hofmann, T. Lawson and S. Ramamoorthy, The role of photosynthesis related pigments in light harvesting, photoprotection and enhancement of photosynthetic yield in planta, Photosynth. Respir., 2022, 1–20 Search PubMed.
- I. N. Gahlawat, Emerging new insights into significance and applications of Plant Pigments, J. Integr. Sci. Technol., 2019, 7, 29–34 CrossRef.
- L. Kapoor, A. J. Simkin, C. George Priya Doss and R. Siva, Fruit ripening: dynamics and integrated analysis of carotenoids and anthocyanins, BMC Plant Biol., 2022, 22, 27 CrossRef PubMed.
- A. J. Meléndez-Martínez, An Overview of Carotenoids, Apocarotenoids and Vitamin A in Agro–Food, Nutrition, Health and Disease, Mol. Nutr. Food Res., 2019, 1801045 CrossRef PubMed.
- L. Kapoor and S. Ramamoorthy, Strategies to meet the global demand for natural food colorant bixin: A multidisciplinary approach, J. Biotechnol., 2021, 338, 40–51 CrossRef.
-
L. Kapoor and S. Ramamoorthy, in Biology, Chemistry, and Applications of Apocarotenoids, CRC Press, 2020, pp. 73–87 Search PubMed.
- S. Tay-Agbozo, S. Street and L. Kispert, The carotenoid Bixin found to exhibit the highest measured carotenoid oxidation potential to date consistent with its practical
protective use in cosmetics, drugs and food, J. Photochem. Photobiol., B, 2018, 186, 1–8 CrossRef PubMed.
- R. Rivera-Madrid, M. Aguilar-Espinosa, Y. Cárdenas-Conejo and L. E. Garza-Caligaris, Carotenoid derivates in achiote (Bixa orellana) seeds: synthesis and health promoting properties, Front. Plant Sci., 2016, 7, 1406 Search PubMed.
- M. Rojo de la Vega, D. D. Zhang and G. T. Wondrak, Topical bixin confers NRF2-dependent protection against photodamage and hair graying in mouse skin, Front. Pharmacol., 2018, 9, 287 CrossRef PubMed.
- S. Wan, Y. Liu, J. Shi, D. Fan and B. Li, Anti-Photoaging and Anti-Inflammatory Effects of Ginsenoside Rk3 During Exposure to UV Irradiation, Front. Pharmacol., 2021, 12, 716248–716248 CrossRef.
- R. I. Amer, S. M. Ezzat, N. M. Aborehab, M. F. Ragab, D. Mohamed, A. Hashad, D. Attia, M. M. Salama and M. H. El Bishbishy, Downregulation of MMP1 expression mediates the anti-aging activity of Citrus sinensis peel extract nanoformulation in UV induced photoaging in mice, Biomed. Pharmacother., 2021, 138, 111537 CrossRef CAS.
- M. P. Rao, K. Manjunath, S. T. Bhagawati and B. S. Thippeswamy, Bixin loaded solid lipid nanoparticles for enhanced hepatoprotection – Preparation, characterisation and in vivo evaluation, Int. J. Pharm., 2014, 473, 485–492 CrossRef CAS PubMed.
- A. D. Pinzón-García, P. Cassini-Vieira, C. C. Ribeiro, C. E. de Matos Jensen, L. S. Barcelos, M. E. Cortes and R. D. Sinisterra, Efficient cutaneous wound healing using bixin-loaded PCL nanofibers in diabetic mice, J. Biomed. Mater. Res., 2017, 105, 1938–1949 CrossRef.
- Y. Zhang, E. Wright and Q. Zhong, Effects of pH on the molecular binding between β-lactoglobulin and bixin, J. Agric. Food Chem., 2013, 61, 947–954 CrossRef.
- F. Rasoulzadeh, D. Asgari, A. Naseri and M. R. Rashidi, Spectroscopic studies on the interaction between erlotinib hydrochloride and bovine serum albumin, Daru, J. Pharm. Sci., 2010, 18, 179 Search PubMed.
- F. Zsila, P. Molnár, J. Deli and S. F. Lockwood, Circular dichroism and absorption spectroscopic data reveal binding of the natural cis-carotenoid bixin to human α1-acid glycoprotein, Bioorg. Chem., 2005, 33, 298–309 CrossRef PubMed.
- W. Rahmalia, J.-F. Fabre, T. Usman and Z. Mouloungui, Aprotic solvents effect on the UV–visible absorption spectra of bixin, Spectrochimica Acta, Part A: Molecular and Biomolecular, 2014, 131, 455–460 CrossRef PubMed.
- G. Silva, F. Gamarra, A. d. Oliveira and F. Cabral, Extraction of bixin from annatto seeds using supercritical carbon dioxide, Braz. J. Chem. Eng., 2008, 25, 419–426 CrossRef.
- R. C. Chisté, F. Yamashita, F. C. Gozzo and A. Z. Mercadante, Simultaneous extraction and analysis by high performance liquid chromatography coupled to diode array and mass spectrometric detectors of bixin and phenolic compounds from annatto seeds, J. Chromatogr. A, 2011, 1218, 57–63 CrossRef PubMed.
- S. Tay-Agbozo, S. Street and L. D. Kispert, The carotenoid bixin: Optical studies of aggregation in polar/water solvents, J. Photochem. Photobiol., 2018, 362, 31–39 CrossRef CAS.
- F. Zsila, P. Molnár and J. Deli, Analysis of binding interaction between the natural apocarotenoid bixin and human serum albumin by circular dichroism and fluorescence spectroscopy, Chem. Biodivers., 2005, 2, 758–772 CrossRef CAS.
- H.-j. Zeng, J. You, R. Yang and L.-b. Qu, Molecular interaction of silybin with hyaluronidase: a spectroscopic and molecular docking study, Spectrosc. Lett., 2017, 50, 515–521 CrossRef CAS.
- U. Alexiev and D. L. Farrens, Fluorescence spectroscopy of rhodopsins: Insights and approaches, Biochim. Biophys. Acta, Bioenerg., 2014, 1837, 694–709 CrossRef CAS.
- X. Li, R. Xu, Z. Cheng, Z. Song, Z. Wang, H. Duan, X. Wu and T. Ni, Comparative study on the interaction between flavonoids with different core structures
and hyaluronidase, Spectrochim. Acta, Part A, 2021, 262, 120079 CrossRef CAS.
- T. R. Van Vleet, M. J. Liguori, J. J. Lynch III, M. Rao and S. Warder, Screening Strategies and Methods for Better Off-Target Liability Prediction and Identification of Small-Molecule Pharmaceuticals, SLAS Discovery, 2019, 24, 1–24 CrossRef CAS.
- Z. Chi, R. Liu and H. Zhang, Noncovalent Interaction of Oxytetracycline with the Enzyme Trypsin, Biomacromolecules, 2010, 11, 2454–2459 CrossRef CAS.
- S. Mohan, H. Hemachandran, P. Sneha, C. George Priya Doss, J. Godwin Christopher, G. Jayaraman and S. Ramamoorthy, Structural insights into the binding mode and conformational changes of BSA induced by bixin and crocin, J. Biomol. Struct. Dyn., 2018, 36, 2085–2098 CrossRef CAS PubMed.
- A. Anantharaman, H. Hemachandran, R. R. Priya, M. Sankari, M. Gopalakrishnan, N. Palanisami and R. Siva, Inhibitory effect of apocarotenoids on the activity of tyrosinase: multi-spectroscopic and docking studies, J. Biosci. Bioeng., 2016, 121, 13–20 CrossRef CAS PubMed.
- H.-J. Zeng, R. Yang, J. You, L.-B. Qu and Y.-J. Sun, Spectroscopic and Docking Studies on the Binding of Liquiritigenin with Hyaluronidase for Antiallergic Mechanism, Scientifica, 2016, 2016, 9178097–9178097 CrossRef.
- X. Xing, X. Yang and Y. Cao, Study of ellagic acid as a natural elastase inhibitor by spectroscopic methods, J. Appl. Spectrosc., 2016, 83, 149–155 CrossRef.
- K. Eun Lee, S. Bharadwaj, U. Yadava and S. Gu Kang, Evaluation of caffeine as inhibitor against collagenase, elastase and tyrosinase using in silico and in vitro approach, J. Enzyme Inhib. Med. Chem., 2019, 34, 927–936 CrossRef.
- A. R. Bautista, E. L. Moreira, M. S. Batista, M. S. Miranda and I. C. Gomes, Subacute toxicity assessment of annatto in rat, Food Chem. Toxicol., 2004, 42, 625–629 CrossRef.
- K. D. James, M. J. Kennett and J. D. Lambert, Potential role of the mitochondria as a target for the hepatotoxic effects of (-)-epigallocatechin-3-gallate in mice, Food Chem. Toxicol., 2018, 111, 302–309 CrossRef PubMed.
- Z. Shi, J.-x. Zhu, Y.-m. Guo, M. Niu, L. Zhang, C. Tu, Y. Huang, P.-y. Li, X. Zhao and Z.-t. Zhang, Epigallocatechin Gallate During Dietary Restriction—Potential Mechanisms of Enhanced Liver Injury, Front. Pharmacol., 2021, 2467 Search PubMed.
-
T. Palmer and P. L. Bonner, in Enzymes (Second Edition), ed. T. Palmer and P. L. Bonner, Woodhead Publishing, 2011, pp. 126–152, DOI:10.1533/9780857099921.2.126.
-
I. G. Dougall and J. Unitt, in The Practice of Medicinal Chemistry (Fourth Edition), ed. C. G. Wermuth, D. Aldous, P. Raboisson and D. Rognan, Academic Press, San Diego, 2015, pp. 15–43, DOI:10.1016/B978-0-12-417205-0.00002-X.
-
V. Leskovac, Comprehensive enzyme kinetics, Springer Science & Business Media, 2003 Search PubMed.
- G. L. Waldrop, A qualitative approach to enzyme inhibition, Biochem. Mol. Biol. Educ., 2009, 37, 11–15 CrossRef CAS.
- C. J. Schmidlin, M. Rojo de la Vega, J. Perer, D. D. Zhang and G. T. Wondrak, Activation of NRF2 by topical apocarotenoid treatment mitigates radiation-induced dermatitis, Redox Biol., 2020, 37, 101714 CrossRef.
- S. Tao, S. L. Park, M. Rojo de la Vega, D. D. Zhang and G. T. Wondrak, Systemic administration of the apocarotenoid bixin protects skin against solar UV-induced damage through activation of NRF2, Free Radicals Biol. Med., 2015, 89, 690–700 CrossRef PubMed.
- X. Zhai, M. Gong, Y. Peng and D. Yang, Effects of UV Induced-Photoaging on the Hair Follicle Cycle of C57BL6/J Mice, Clin., Cosmet. Invest. Dermatol., 2021, 14, 527–539 CrossRef.
- A. Kahraman and M. E. Inal, Protective effects of quercetin on ultraviolet A light–induced
oxidative stress in the blood of rat, J. Appl. Toxicol., 2002, 22, 303–309 CrossRef PubMed.
- S. Shin, L.-X. Wang, X.-Q. Zheng, L.-P. Xiang and Y.-R. Liang, Protective effect of (-)-epigallocatechin gallate against photo-damage induced by ultraviolet a in human skin fibroblasts, Trop. J. Pharm. Res., 2014, 13, 1079–1084 CrossRef.
- J. Cui, Y. Fu, Z. Yi, C. Dong and H. Liu, The beneficial effects of ultraviolet light supplementation on bone density are associated with the intestinal flora in rats, Appl. Microbiol. Biotechnol., 2021, 105, 3705–3715 CrossRef CAS PubMed.
- Z. Xu and X.-Q. Kong, Bixin ameliorates high fat diet-induced cardiac injury in mice through inflammation and oxidative stress suppression, Biomed. Pharmacother., 2017, 89, 991–1004 CrossRef PubMed.
- S. Somacal, C. G. Figueiredo, A. Quatrin, A. R. Ruviaro, L. Conte, P. R. Augusti, M. Roehrs, I. T. Denardin, J. Kasten and M. L. Da Veiga, The antiatherogenic effect of bixin in hypercholesterolemic rabbits is associated to the improvement of lipid profile and to its antioxidant and anti-inflammatory effects, Mol. Cell. Biochem., 2015, 403, 243–253 CrossRef PubMed.
- A. D. Pinzón-García, L. A. A. Orellano, M. G. T. de Lazari, P. P. Campos, M. E. Cortes and R. D. Sinisterra, Evidence of hypoglycemic, lipid-lowering and hepatoprotective effects of the Bixin and Bixin: β-CD inclusion compound in high-fat-fed obese mice, Biomed. Pharmacother., 2018, 106, 363–372 CrossRef PubMed.
- R. M. Perez Gutierrez and R. Valadez Romero, Effects of bixin in high-fat diet-fed-induced fatty liver in C57BL/6J mice, Asian Pac. J. Trop. Biomed., 2016, 6, 1015–1021 CrossRef.
- Ö. B. Acıkara, M. Ilhan, E. Kurtul, K. Šmejkal and E. K. Akkol, Inhibitory activity of Podospermum canum and its active components on collagenase, elastase, and hyaluronidase enzymes, Bioorg. Chem., 2019, 93, 103330 CrossRef PubMed.
- J. Y. Kim, Y. Wang, Z. Uddin, Y. H. Song, Z. P. Li, J. Jenis and K. H. Park, Competitive neutrophil elastase inhibitory isoflavones from the roots of Flemingia philippinensis, Bioorg. Chem., 2018, 78, 249–257 CrossRef.
- T. S. Thring, P. Hili and D. P. Naughton, Anti-collagenase, anti-elastase, and antioxidant activities of extracts from 21 plants, BMC Complementary Altern. Med., 2009, 9, 27 CrossRef.
- P. T. B. Tu and S. Tawata, Antioxidant, anti-aging, and anti-melanogenic properties of the essential oils from two varieties of Alpinia zerumbet, Molecules, 2015, 20, 16723–16740 CrossRef.
- Y. A. Yahaya and M. M. Don, Evaluation of Trametes lactinea extracts on the inhibition of hyaluronidase, lipoxygenase, and xanthine oxidase activities in vitro, J. Physiol. Sci., 2012, 23, 1–15 Search PubMed.
- Y. Liu, E. Hwang, H. T. T. Ngo, H. Perumalsamy, Y. J. Kim, L. Li and T.-H. Yi, Protective Effects of Euphrasia officinalis Extract against Ultraviolet B-Induced Photoaging in Normal Human Dermal Fibroblasts, Int. J. Mol. Sci., 2018, 19, 3327 CrossRef.
- C. Zhang, S. M. Mortuza, B. He, Y. Wang and Y. Zhang, Template-based and free modeling of I-TASSER and QUARK pipelines using predicted contact maps in CASP12, Proteins, 2018, 86(Suppl 1), 136–151 CrossRef.
- S. Uk, D. Tk, C. Doss and H. Zayed, Mutational landscape of K-Ras substitutions at 12th position-a systematic molecular dynamics approach, J. Biomol. Struct. Dyn., 2020, 1–16 Search PubMed.
- S. U. Kumar, D. T. Kumar, P. D. Mandal, S. Sankar, R. Haldar, B. Kamaraj, C. E. J. Walter, R. Siva, C. G. P. Doss and H. Zayed, Comprehensive in silico screening and molecular dynamics studies of missense mutations in Sjogren-Larsson syndrome associated with the ALDH3A2 gene, Adv. Protein Chem. Struct. Biol., 2020, 120, 349–377 Search PubMed.
- S. Tao, M. Rojo de la Vega, H. Quijada, G. T. Wondrak, T. Wang, J. G. N. Garcia and D. D. Zhang, Bixin protects mice against ventilation-induced lung injury in an NRF2-dependent manner, Sci. Rep., 2016, 6, 18760 CrossRef PubMed.
- Y. Yu, D.-M. Wu, J. Li, S.-H. Deng, T. Liu, T. Zhang, M. He, Y.-Y. Zhao and Y. Xu, Bixin attenuates experimental autoimmune encephalomyelitis by suppressing TXNIP/NLRP3 inflammasome activity and activating NRF2 signaling, Front. Immunol., 2020, 11, 593368 CrossRef.
- A. R. P. L. Bautista, E. Moreira, M. S. Batista, M. Miranda and I. Gomes, Subacute toxicity assessment of annatto in rat, Food Chem. Toxicol., 2004, 42, 625–629 CrossRef.
|
This journal is © The Royal Society of Chemistry 2023 |