DOI:
10.1039/D2FO02364D
(Paper)
Food Funct., 2023,
14, 305-318
Ovotransferrin alleviated acute gastric mucosal injury in BALB/c mice caused by ethanol†
Received
11th August 2022
, Accepted 19th November 2022
First published on 12th December 2022
Abstract
Acute gastric mucosal injury is a common gastrointestinal disorder, which influences patients’ life quality. It was found that ovotransferrin (OVT) reduces the abundance of Helicobacter pylori associated with gastric disease in the intestine of immunosuppressed mice. To clarify its gastric protective function, the present study investigated the effect of OVT on BALB/c mice with ethanol-induced gastric mucosal injury. Results showed that OVT attenuated the ethanol-induced gastric mucosal injury. Furthermore, OVT effectively downregulated the expression of inflammatory markers (tumor necrosis factor-α, interleukin (IL)-1β and IL-6) but enhanced the secretion of IL-4, IL-10 and prostaglandin E2. And OVT pretreatment significantly inhibited the activation of the MAPK/NF-κB pathway. Additionally, OVT improved gastric antioxidant ability by increasing superoxide dismutase and glutathione levels and decreasing malondialdehyde and myeloperoxidase content. Pretreatment with OVT modulated the equilibrium between B-cell lymphoma-2 (Bcl-2) and Bcl-2-associated X. The above results indicated that OVT alleviated inflammatory responses, oxidative stress and apoptosis in gastric mucosal injury mice caused by ethanol.
1. Introduction
The gastric mucosa is a very thin and fragile tissue, which is located in the innermost layer of the gastric wall. It possesses a balanced mechanism of self-repair. At the same time, it protects the stomach and also serves as a natural defense of the tissues against external pathogens and toxic substances. Under normal physiological conditions, the gastric mucosa maintains its integrity by keeping the integrity of the inner mucosal barrier and controlling the secretion of mucus, bicarbonate, prostaglandins, and heat-shock proteins (HSP) as well as growth factors.1,2 Mucosal damage occurs when the invasion of invasive factors such as gastric acid, pepsin, ethanol, Helicobacter pylori, and non-steroidal anti-inflammatory drugs (NSAIDs) exceeds the defense function of the gastric mucosa.3 Disruption of the gastric mucosal barrier causes damage to the epithelial cell and triggers inflammation, then gradually leads to ulcers. Currently, gastric mucosal diseases affect 25.0%–30.0% of the global population.4 And its clinical treatment mainly focuses on the use of antisecretory drugs. Among these are proton pump inhibitors (omeprazole), H2 receptor antagonists (cimetidine), antacids (aluminum hydroxide), prostaglandin analogues, antibiotics (clarithromycin) and mucosal protectors (bismuth).5,6 Although chemical drugs are effective in alleviating gastric mucosal damage, they induce side effects, including drug resistance after long-term use. Therefore, it is necessary to seek effective and natural substances to prevent and treat gastric mucosal injury.
In recent years, food-derived proteins have been found to successfully protect the gastric mucosa and alleviate damage to the gastric mucosa. For instance, wheat peptides protected the gastric mucosa from ethanol-induced lesions in rats via improving the gastric microcirculation and inhibiting inflammation mediated by the nuclear factor kappa-B (NF-κB) signaling transduction pathway.7 Milk and soybean proteins, are considered to protect gastric mucosa by improving the gastric mucosal barrier, preventing oxidative stress, and promoting cellular proliferation.8 In conclusion, food-derived protein has the advantages of wide sources, easy access, safety and non-toxicity, etc. It plays an irreplaceable role in human nutritional health and disease mitigation, and has great development potential and broad application prospects.
Ovotransferrin (OVT) is an iron-binding glycoprotein present in and accounting for about 12.0%–13.0% of egg white.9 OVT displays a ribbon-like structure, with the polypeptide chain folded to form the N-terminal and C-terminal, each of which contains an iron-binding site. And the iron-binding ability of C-terminal is better than that of N-terminal.10 Due to its special structure, OVT is endowed with various biological activities such as anti-inflammatory, anti-oxidant, anti-viral, and immunomodulatory effects, and regulation of intestinal flora.11–15 For example, free radical scavenging domains at the N-terminal of OVT exert the effect of scavenging free radicals and chelating pro-oxidant metal ions to amino acid residues by disrupting its structure, which makes OVT possess antioxidant activity.16,17 OVT inhibits the accumulation of intracellular reactive oxygen species (ROS) and blocks the apoptosis mediated by ROS activated mitochondria.18 In our previous study, OVT was found to decrease the abundance of Helicobacter pylori in the intestine of immunosuppressed mice.10 The bacterium has been reported to be closely associated with the development of gastritis, even stomach cancer.19 Thus, we speculate that OVT may have protective effects on the gastric mucosa.
To verify our hypothesis, a mouse model of ethanol-induced gastric mucosal injury was established, which is widely used to study the preventive or therapeutic effects of drugs or healthy foods on gastric mucosal injury. In this study, the alleviating effect and the possible mechanism of OVT on gastric mucosal injury were investigated. It will provide a theoretical basis for the development of functional foods with OVT as raw material for gastric health.
2. Materials and methods
2.1. Materials and reagents
OVT (purity ≥98) was purchased from Sigma (Sigma-Aldrich, USA). Rabbit anti-mouse B-cell lymphoma-2 (Bcl-2) and rabbit anti-mouse Bcl-2-associated X (Bax) were from ABclonal Biotechnology (Wuhan, China). Rabbit anti-mouse GAPDH, p65, IKK, p-IKK, IκB, p-IκB, p38, p-p38, JNK, p-JNK, ERK, and p-ERK were from Cell Signaling Technology (MA, USA). Tumor necrosis factor-α (TNF-α), interleukin-6 (IL-6), IL-1β, IL-4, IL-10 superoxide dismutase (SOD), malondialdehyde (MDA), glutathione (GSH) and myeloperoxidase (MPO) assay kits were purchased from MEIMIAN (Jiangsu, China). The kits for total RNA extraction, cDNA synthesis, and reverse transcription-polymerase chain reaction (RT-PCR) were bought from TransGen Biotech (Beijing, China). In addition, all chemical reagents used in the experiments were of analytical grade.
2.2. Animals and experimental design
All animal experiment procedures were approved by Jiangxi Agricultural University Ethics Committee (JXAUA01). Female BALB/c mice (17–19 g, 6–8 weeks old) of SPF grade, were provided by Hunan SJA Laboratory Animal Co. (Hunan, China). Standard rodent food and water were freely available to each mouse. Other conditions were room temperature of 24 ± 1 °C, relative humidity of 45.0% ± 5.00%, and a 12 h/12 h light–dark cycle, and the mice were acclimated to feeding for one week.
After one week of adaptive feeding, the 50 mice were randomly divided into 5 groups (n = 10), including the control group (control), gastric mucosal lesion model group (model), positive control group (cimetidine-10 mg kg−1 day−1), and OVT pretreatment groups (OVT-100 mg kg−1 day−1 and OVT-300 mg kg−1 day−1).
To evaluate the effect of OVT on gastric mucosa protection, the acute gastric mucosal damage model of ethanol which has been recommended by China Food and Drug Administration (CFDA Publication No. 107, revised 2012) was used. The animal experimental design was slightly modified according to Mingzhu Guo, et al.20 During the experiment, standard rodent food and water were freely available to each mouse. The animal experimental process is exhibited in Fig. 1. Briefly, cimetidine (10 mg kg−1 day−1) and OVT (OVT-100 mg kg−1 day−1 and OVT-300 mg kg−1 day−1) were administered orally on days 1–14. After the last administration of the drug, the mice were fasted for 24 hours, so that the stomach contents had been completely digested and absorbed. Mice in all groups except the control group were administered 75.0% ethanol (0.1 mL per 20 g), whereas in those in the control group the same amount of sterile water was administered. After 1 hour, blood was collected from the eye sockets and the mice were sacrificed. The gastric tissue was immersed in ice-cold phosphate buffer solution (PBS) and rinsed thoroughly to remove the chyme. To preserve the gastric tissue, it was snap-frozen in liquid nitrogen and subsequently stored at −80 °C.
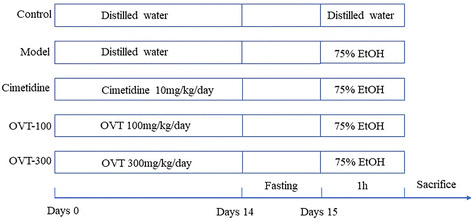 |
| Fig. 1 Experimental design. | |
2.3. Ulcer injury index and injury inhibition rate of gastric tissue analysis
The stomach was obtained by cutting open the abdomen. The contents were washed with pre-cooled saline and then the gastric tissue was unfolded for fixation and photographed for the record. The damaged area of gastric mucosa was calculated by the ImageJ imaging software, and the gastric mucosal ulcer injury index and injury inhibition rate were calculated according to the formula.21
2.4. Histological analysis
The gastric tissues were fixed with 4.00% paraformaldehyde for 24 h. Then, the fixed gastric tissue was dehydrated and embedded in paraffin and cut into 4 μm thick sections. After staining with hematoxylin–eosin (HE), it was observed under a light microscope in the section. The previous literature was referred to for the gastric mucosal damage scoring criteria22 and they are shown in Table 1.
Table 1 Gastric mucosal damage scoring method
Score |
Mucosal edema |
Hemorrhage |
Inflammatory cell infiltration |
Epithelial cell loss |
0 |
Normal |
Normal |
Normal |
Normal |
1 |
Mild edema |
Individual bleeding points |
Individual inflammatory cell infiltration |
Epithelial cells fall off |
2 |
Edema in local area |
Massive bleeding in local area |
Few inflammatory cell infiltrates |
Massive epithelial cells fall off |
3 |
Massive edema |
Large bleeding bands and lots of bleeding spots |
Massive inflammatory cell infiltration |
Massive epithelial cell loss |
2.5. Biochemical analysis
The gastric tissues were homogenized and the supernatants were harvested. The levels of SOD, GSH, MDA and MPO in gastric tissues were detected by biochemical kits according to the instruction of the manufacturers.
2.6. Enzyme-linked immunosorbent assay (ELISA) analysis
Serum was obtained from blood and centrifuged at 4 °C for 15 minutes (10
000 rpm).20 The levels of IL-1β, IL-6 and TNF-α in serum were measured by ELISA kits following the manufacturer's instructions.
The gastric tissues were homogenized and the supernatants were collected. The levels of TNF-α, IL-6, IL-4, IL-10 and prostaglandin E2 (PGE2) in gastric tissues were detected by ELISA kits according to the instruction of the manufacturers.
2.7. RT-PCR analysis
The gastric tissues were washed with pre-cooled PBS and were then ground with TransZol. Total RNA was extracted according to the manufacturer's instructions, and the value of A260/A280 nm was used to evaluate the concentration and purity of RNA. With RNA as the template, reverse transcription was performed to obtain cDNA. As part of the reaction, RT-PCR was carried out using start TM Green qPCR Super Mix (TransGen Biotech, Beijing, China), including denaturation at 94 °C for 5 s, annealing at 60 °C for 15 s, and extension at 72 °C for 10 s. The entire reaction was kept for 40 amplification cycles. The expression levels of the target genes were normalized to GAPDH and the 2−ΔΔCt method was used for calculating the relative expression levels of target genes,23 as shown in Table 2.
Table 2 Sequence of Mouse primers in qRT-PCR
Gene |
Froward primer (5′–3′) |
Reverse primer (5′–3′) |
GAPDH |
GGGTCCCAGCTTAGGTTCAT |
CCAATACGGCCAAATCCGTT |
IL-17 |
CCAGGGAGAGCTTCATCTGT |
AGGAAGTCCTTGGCCTCAGT |
IL-6 |
GACTGATGCTGGTGACAACC |
AGACAGGTCTGTTGGGAGTG |
IL-1β |
ACTCATTGTGGCTGTGGAGA |
AGCCTGTAGTGCAGTTGTCT |
TNF-α |
CTCATGCACCACCATCAAGG |
ACCTGACCACTCTCCCTTTG |
IFN-γ |
CTGCTGA TGGGAGGAGATG |
TTTGTCATTCGGGTGTAGTCA |
IL-10 |
ACCTGGTAGAAGTGATGCC |
ACCTGGTAGAAGTGATGCC |
Bcl-2 |
TTTCCATGGACGCGTTTGAA |
TTCCTCCGCAATGCTGAAAG |
Bax |
ACCATCTTTGTGGCTGGAGT |
AAGACACAGTCCAAGGCAGT |
2.8. Western blot analysis
An appropriate amount of gastric tissue were lysed with RIPA lysis buffer containing 1.00% protease inhibitor and phosphatase inhibitor for 15 min, then centrifuged at 12
000 rpm for 15 min, and the supernatant was collected. Measured protein concentrations using the bicinchoninic acid (BCA) protein assay kit (Solarbio, Beijing, China) and each sample protein concentration were then adjusted to the same level. The protein was transferred to nitrocellulose membranes after it was separated by SDS-PAGE (10.0%). It was then incubated with 5.00% non-fat milk powder for 1.5 hours, then washed 4 times with TBST buffer. Next, nitrocellulose membranes incubated with primary antibody (1
:
1000) overnight at 4 °C. Incubation for 1 hour with horseradish peroxidase-conjugated anti-rabbit IgG secondary antibody at a dilution of 1
:
10
000 was performed after four washes with TBST buffer. Finally, the strips were visualized with SuperSignal West Pico chemiluminescent substrate (Triple Eagle) and photographed with a chemiluminescence imaging system, and the protein expression levels were calculated by the Image J software. During the experiment, the expression of each target protein was detected, and the expression of the target protein was normalized by the internal reference GAPDH.
2.9. Statistical analysis
The data were expressed as the mean ± SEM of at least three biological replicates for each experiment. The differences between groups were evaluated by one-way analysis of variance (ANOVA) and Dunnet post hoc analysis with p < 0.05 for significance (GraphPad Prism 8.0 for Windows).
3. Results
3.1. Effect of OVT on body weight in the mice
As shown in Fig. 2, the body weights of mice in the OVT and cimetidine groups showed the same trend as that of the control group, and there were no significant differences in animal body mass among the groups. In addition, during the experimental period, no abnormalities in the appearance, physical signs, fecal traits, or food intake of the mice were noticed in each group, and no signs of toxicity or death were observed. The above results indicated that OVT and cimetidine had no notable effect on the normal growth of mice.
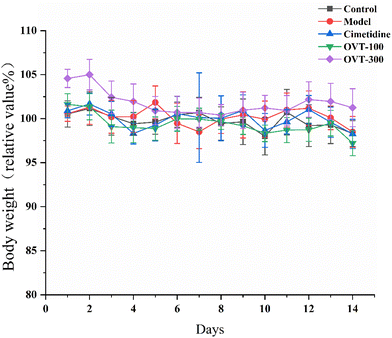 |
| Fig. 2 Effect of OVT on body weight in the mice. Data are presented as the mean ± SEM (n = 10). | |
3.2. Effect of OVT on macroscopic and microscopic histopathology of gastric mucosal injury mice
High concentrations of ethanol can cause acute gastric mucosal injury in mice.24 As shown in Fig. 3A, in the control group, the mucosa was intact, smooth, and regular in appearance, and there were no visible lesions on the stomach of the mice. Four groups of mice that were gavaged with 75.0% ethanol showed varying degrees of hemorrhagic gastric lesions, and the incidence rate of injury was 100%, indicating that ethanol caused gastric mucosal damage (Fig. 3A b-e). Mice in the model group showed the most severe gastric mucosal damage, with the widest range of lesions appearing as extended hemorrhagic bands, while the OVT groups and cimetidine group revealed milder damage compared with the model group. Pretreatment with OVT and cimetidine both alleviated gastric mucosal injury in mice resulting in the reduction of the area of injury, bleeding bands and bleeding points, which implied that OVT effectively repressed the development of gastric injury in the mice.
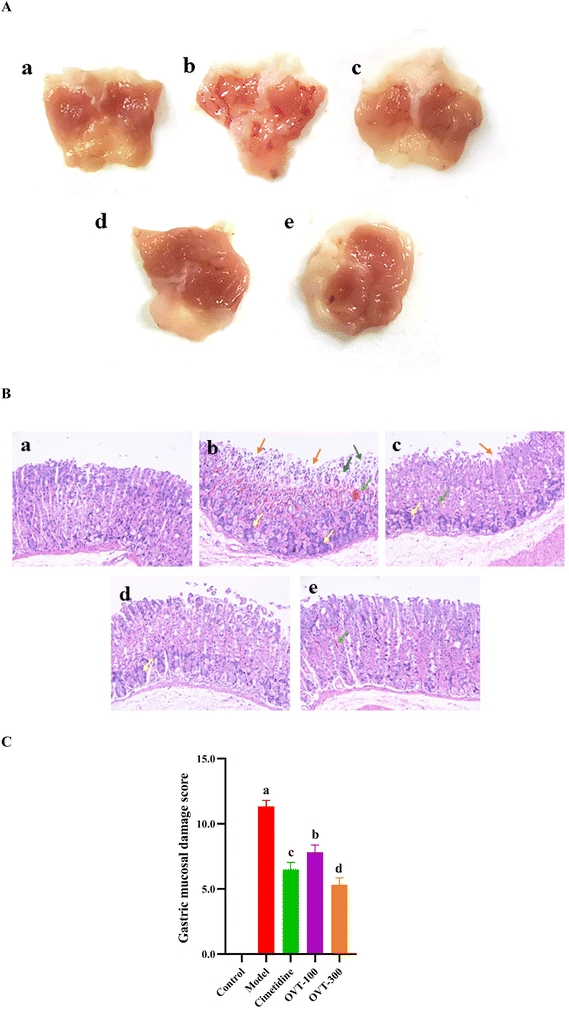 |
| Fig. 3 Effect of OVT on macroscopic and microscopic histopathology of gastric mucosal injury mice. (A) Macroscopic appearance of gastric tissues (n = 10). (B) Representative image of HE staining (magnification of 200×; (n = 10)). a–e: Control group, model group, cimetidine (10 mg kg−1) group, OVT-low (100 mg kg−1) group and OVT-high (300 mg kg−1) group. Orange →: loss of gastric epithelial cells; blue →: edema; green →: vascular congestion; yellow →: inflammatory cells infiltration. (C) Microscopic gastric mucosal damage score. Data are presented as the mean ± SEM (n = 8). Different letters indicate statistically significant differences at the level of p < 0.05. | |
To further investigate the effect of OVT on the pathological alterations in gastric tissues, HE staining was performed (Fig. 3B). In the control group, the gastric mucosa showed an intact structure with a normal epithelial lining. It revealed no signs of a disorganized glandular structure or mucosal edema, and no signs of inflammation or edema (Fig. 3B a). However, ethanol caused inflammation, prominent edema, hemorrhage, and visible diffused hemorrhage in the submucosa of the gastric mucosa (Fig. 3B b). Compared with the model group, the OVT groups showed minor damage to mucosal tissues, including a shallow layer, signs of congestion, and small amounts of exfoliation. The results of the gastric mucosal damage score in Fig. 3C demonstrated that OVT could notably reduce microscopic gastric damage induced by ethanol.
According to the previously mentioned formula, the ulcer injury index and injury inhibition rate were calculated by the software Image J. As shown in Table 3, the index of the gastric mucosal lesion (20.56 ± 2.47%) was obviously increased in the model group, while the index of gastric mucosal damage in the cimetidine, OVT-low, and OVT-high groups was 4.75 ± 1.67%, 6.21 ± 1.06% and 4.03 ± 1.75%, respectively. The inhibition rate of gastric mucosal injury, in the cimetidine, OVT-low, and OVT-high groups was 77.80%, 69.80%, and 80.37%. Compared with the model group, pretreatment of OVT and cimetidine clearly enhanced the inhibition rate of gastric mucosal injury and reduced gastric mucosal injury. These results indicated that both OVT and cimetidine were able to reduce gastric mucosal damage.
Table 3 The ulcer injury index and injury inhibition rate in each group (n = 10)
Groups |
Dosage/mg kg−1 |
Ulcer injury index % |
Injury inhibition rate/% |
Data are presented as the mean ± SEM (n = 10). Different letters indicate statistically significant differences at the level of p < 0.05. |
Control |
— |
0.00 ± 0.00 |
— |
Model |
— |
20.56 ± 2.47a |
0 |
Cimetidine |
10 |
4.56 ± 1.67bc |
77.80 ± 1.45ab |
OVT-100 |
100 |
6.21 ± 1.06b |
69.80 ± 1.74b |
OVT-300 |
300 |
4.03 ± 1.75c |
80.37 ± 1.38a |
3.3. Effect of OVT on antioxidant capacity of gastric tissues of gastric mucosal injury mice
To evaluate the antioxidant activity of OVT on gastric tissues of ethanol-induced damage to mice, the levels of SOD, GSH, MDA and MPO were measured by biochemical kits (Fig. 4). Compared with that of the control group, the activity of SOD and GSH of gastric tissues in the model group was lower, while the levels of MDA and MPO had improved. However, pretreatment with OVT and cimetidine reversed the alteration induced by ethanol. These results suggested that pre-administration of OVT can regulate the balance of oxidative stress and antioxidant activity in the gastric tissues of mice.
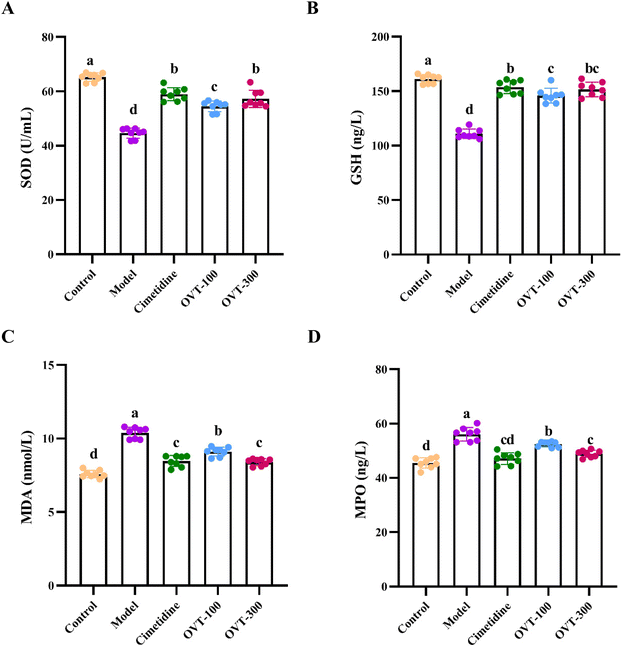 |
| Fig. 4 Effect of OVT on antioxidant capacity of gastric tissues of gastric mucosal injury mice by measuring SOD, GSH, MDA and MPO. (A–D) SOD, GSH, MDA and MPO measurements. Data are presented as the mean ± SEM (n = 8). Different letters indicate statistically significant differences at the level of p < 0.05. | |
3.4. Effect of OVT on inflammation related factor levels in gastric mucosal injury mice
To investigate whether OVT can alleviate the inflammation in the stomach induced by ethanol, the levels of inflammation related factors, such as TNF-α, IL-6, IL-1β, IL-10, IL-4 and PGE2 were measured by ELISA kits.
Multiple pro-inflammatory cytokines, such as IL-1β, IL-6, and TNF-α, are involved in the inflammatory response. In this study, the model group mice showed significantly higher levels of IL-1β, IL-6, and TNF-α in serum (Fig. 5) and gastric tissues (Fig. 6A and B) than those in mice of the control group. However, when compared to the model group, the contents of IL-1β, IL-6, and TNF-α were decreased in OVT and cimetidine groups. OVT could distinctly inhibit the upregulation of the aforementioned pro-inflammatory factors.25 Moreover, there was no difference between the OVT-high group and the cimetidine group.
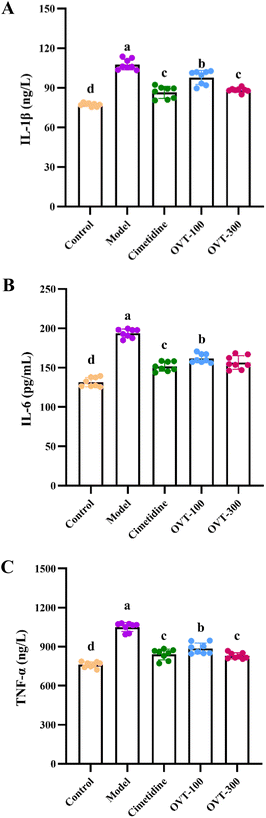 |
| Fig. 5 Effect of OVT on pro-inflammatory cytokines secretion in serum of gastric mucosal injury mice. (A–C) IL-1β, IL-6 and TNF-α concentrations in serum of mice with ethanol-induced gastric injury. Data are presented as the mean ± SEM (n = 8). Different letters indicate statistically significant differences at the level of p < 0.05. | |
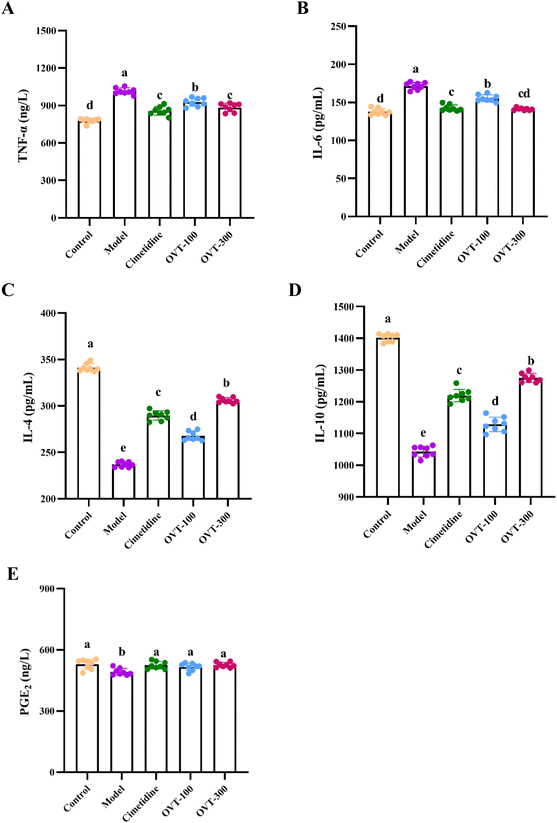 |
| Fig. 6 Effect of OVT on inflammation related factors levels in the gastric tissues of gastric mucosal injury mice. (A–E) TNF-α, IL-6, IL-4, IL-10 and PGE2 contents in the gastric tissues of mice with ethanol-induced gastric injury. Data are presented as the mean ± SEM (n = 8). Different letters indicate statistically significant differences at the level of p < 0.05. | |
PGE2 is now considered a gastrointestinal protective factor and plays a vital role in attenuating acute gastric mucosal injury.26,27 Meanwhile, PGE2, as an inflammatory cytokine, can depress the inflammatory response.28–30 Some cytokines with anti-inflammatory effects such as IL-4 and IL-10 relieve inflammation. As shown in Fig. 6C–E, ethanol stimulation considerably reduced IL-4, IL-10 and PGE2 levels in gastric tissues, while OVT pretreatment enhanced the levels of IL-4 and IL-10, and restored PGE2 to normal levels. These results suggested that OVT protects the gastric mucosa by regulating the contents of inflammation related factors.
mRNA expression levels of cytokines, including TNF-α, IL-17, IL-1β, IL-6, IL-10 and interferon γ (IFN-γ) were analyzed by qRT-PCR. As shown in Fig. 7, mRNA expression of pro-inflammatory factors (TNF-α, IL-17, IL-1β, IL-6 and INF-γ) were increased in the model group, while mRNA expression of the anti-inflammatory factor IL-10 was decreased. In the OVT groups, the different dosages of OVT can obviously downregulate mRNA expression of the above pro-inflammatory factors, while that of IL-10 mRNA was upregulated when compared to those in the model group. These results suggested that OVT can reduce mRNA expression of pro-inflammatory factors but enhance the mRNA level of anti-inflammatory factors, which led to suppression of the development of inflammation. Meanwhile, no significance was found between the OVT-high group and cimetidine group.
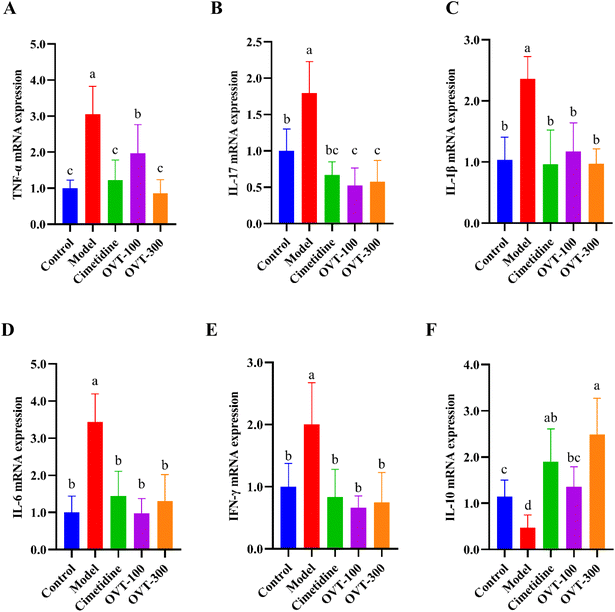 |
| Fig. 7 Effect of OVT on the mRNA expression levels of cytokines in the gastric tissues of gastric mucosal injury mice. (A–F) TNF-α, IL-17, IL-1β, IL-6, INF-γ and IL-10 mRNA measurements. Data are presented as the mean ± SEM (n = 6). Different letters indicate statistically significant differences at the level of p < 0.05. | |
3.5. Effect of OVT on the activation of mitogen-activated protein kinase (MAPK)/NF-κB in the gastric tissues of gastric mucosal injury mice
To determine whether OVT exerted anti-inflammatory effects via the MAPK/NF-κB pathway, we analyzed expression levels of key proteins in the MAPK and NF-κB pathways using western blot (Fig. 8). The western blot analysis showed ethanol induced activation of the NF-κB signaling pathway, resulting in phosphorylation of IKK and IκB. After pretreatment with OVT, the value of p-IKK/IKK, and p-IκB/IκB decreased notably, and p65/GADPH increased significantly, indicating that OVT had an excellent inhibitory effect on the NF-κB pathway (Fig. 8A). MAPK contains three subunits: p38, ERK and JNK, which were phosphorylated under the induction of ethanol. OVT pretreatment suppressed the phosphorylation of MAPK, suggesting that OVT can inhibit the activation of ethanol-induced MAPK in gastric tissues, and thereby prevent the production of pro-inflammatory mediators (Fig. 8B). The above findings suggested that OVT could negate the activation of MAPK/NF-κB pathways.
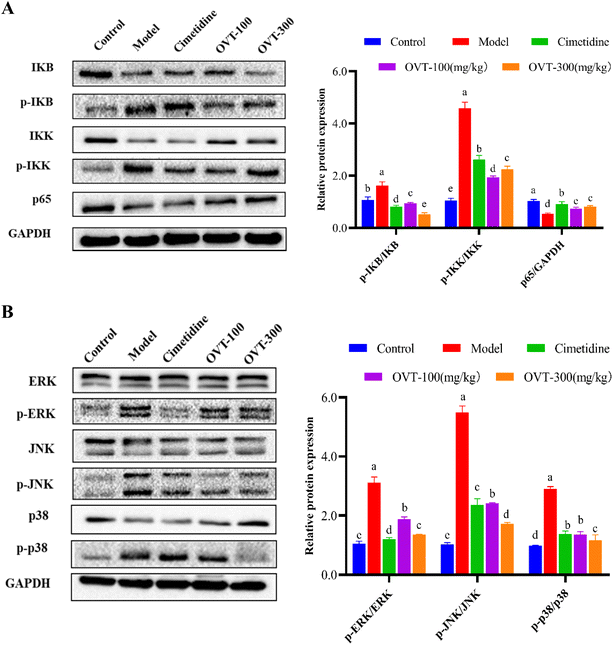 |
| Fig. 8 Effect of OVT on the activation of the MAPK/NF-κB pathway. (A) Western blotting analyses of key protein expressions on the NF-κB pathway in mouse gastric tissues. (B) Western blotting analyses of key protein expressions on MAPK pathway in mouse gastric tissues Data are presented as the mean ± SEM (n = 4). Different letters indicate statistically significant differences at the level of p < 0.05. | |
3.6. Effect of OVT on apoptotic capacity of gastric mucosal injury mice
The ratio of Bcl-2 to Bax reflects the probability of apoptosis. To detect the apoptotic ability of OVT on ethanol-induced damage to mouse gastric tissues, mRNA and protein expression levels of Bax and Bcl-2 in mouse gastric tissues were examined by RT-PCR and western blot analysis, respectively (Fig. 9). Compared with the control group, ethanol treatment markedly increased the expression of Bax and suppressed the expression of Bcl-2, thus decreasing the Bcl-2/Bax ratio. Compared with the levels of ratio in the ethanol-treated group, OVT dramatically upregulated the value of Bcl-2/Bax.
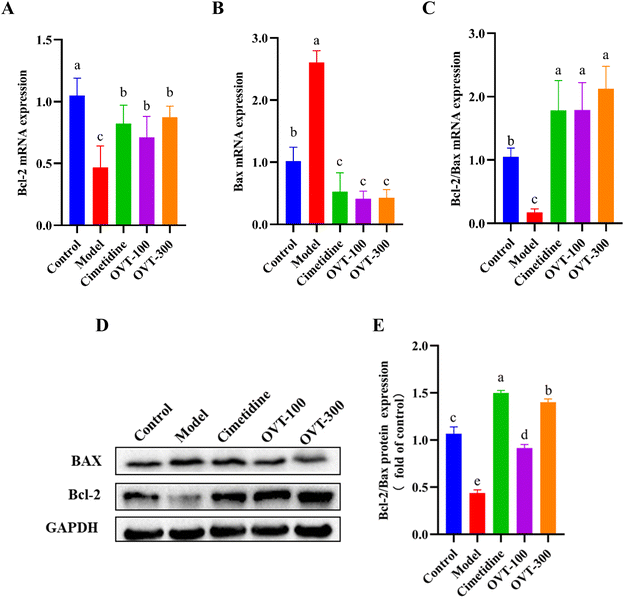 |
| Fig. 9 Effect of OVT on the apoptosis capacity of gastric tissues of gastric mucosal injury mice. (A and B) mRNA expression level of Bcl-2 and Bax. (C) Bcl-2/Bax mRNA ratio. Data are presented as the mean ± SEM (n = 6). (D–E) Western blotting analyses of Bax and Bcl-2 protein expression in mouse gastric tissues. Data are presented as the mean ± SEM (n = 4). Different letters indicate statistically significant differences at the level of p < 0.05. | |
4. Discussion
Nowadays, alcohol-induced gastric mucosal injury is a common upper gastrointestinal tract disease. Either a small amount of long-term or excessive drinking of short-term can cause significant damage to the gastric mucosa. When alcohol, also named ethanol, enters the digestive tract, it damages the mucosal areas of the gastrointestinal tract. In previous studies, it was demonstrated that high concentrations of alcohol destroy the mucus layer on the surface of gastric mucosa by directly eroding the surface of gastrointestinal tissues, leading to oxidative damage to the epithelial cells, and then resulting in inflammation, apoptosis, and ultimately gastric damage.27,31 The main manifestations of gastric injury are mucosal edema, congestion, bleeding, epithelial detachment, and inflammatory cell infiltration. However, gastric bleeding and ulcers are considered to be the most common clinical manifestations.
OVT can be extracted from poultry egg whites at a low cost. It has good biological functions, such as anti-inflammation, antioxidant, immunomodulation, and regulation of intestinal flora.17 In this study, by constructing a mouse model of ethanol-induced gastric mucosal injury at high concentrations, OVT was found to alleviate ethanol-induced gastric mucosal injury through anti-inflammatory and antioxidant effects, and reduction in the apoptotic pathways.
Both macroscopic and microscopic observations were performed to evaluate the gastric mucosal damage in mice.22 From the macroscopic result, OVT pretreatment could reduce the area of ulcers, bleeding points, and bleeding bands. Microscopic results, which were obtained through HE staining, showed that pretreatment of OVT alleviated the pathological changes of gastric tissues to some extent, such as reducing inflammatory cell infiltration and decreasing epithelial cell shedding and cell gaps, resulting in neater cell alignment. These results implied that OVT pretreatment could effectively alleviate ethanol-induced gastric mucosal injury.
When ethanol induces gastric mucosal injury, the body produces a large number of free radicals, which changes the content of antioxidant enzymes, like SOD and GSH, in the body. When the level of free radicals exceeds the scavenging capacity of the antioxidant enzymes, lipid peroxidation reaction will happens. MDA is the final metabolite of lipid peroxidation, indirectly reflecting the degree of gastric injury to some extent. In the ethanol-induced gastric mucosal injury model, the concentration of MDA increased, while SOD and GSH levels declined.32–34 In this study, the model group exhibited the same trend. MPO is a heme proteinase expressed mainly in neutrophils and is closely associated with neutrophil infiltration in response to gastric mucosal injury.35 In acute tissue injury and chronic inflammatory diseases, such as atherosclerosis, cancer, kidney disease, lung injury, and multiple sclerosis, the elevation of MPO is one of the markers of inflammation and oxidative stress.36,37 OVT increased the levels of SOD and GSH and decreased the concentration of MDA and MPO, which implied that OVT could promote the antioxidative ability of gastric tissue.
OVT modulated secretion and mRNA expression of factors associated with inflammation. And the production and secretion of inflammatory cytokines are regulated by various signaling pathways. In recent years, many studies have confirmed that the MAPK/NF-κB pathway plays an important role in ethanol-induced gastric mucosal injury.32,34,38 The MAPK pathway, containing JNK, p38, and ERK kinases, is a transduction pathway for expression of various pro-inflammatory mediators and apoptotic signals. Upon stimulation of the organism, p38, ERK and JNK proteins are phosphorylated and transferred into the nucleus to regulate a variety of transcription factors, further promoting the transcription and translation of inflammatory factors and exacerbating the inflammatory response. Butyrate pretreatment attenuated the phosphorylation of p38 MAPK and ERK in the gastric tissues and may be applied to the prevention and treatment of gastric ulcers.39 Fermented soybean prevents gastric injury by inhibiting the JNK pathway.40 In short, inhibition of either MAPK pathways reduces inflammatory response.41 It has also been reported that the MAPK pathway may suppress the downstream NF-κB pathway.42 NF-κB is a cytoplasmic transcription factor that is phosphorylated in the cytoplasm and then translocated to the nucleus. It then binds to the promoter regions of many genes and activates inflammation regulatory genes (TNF-α, IL-6 and IL-1β, etc.), of which the expression of downstream proteins has increased. IKK is an upstream regulatory protein of IκB. When the organism was stimulated by inflammatory cytokines or other foreign stimuli, IκB kinase was activated, IKB dissociated from NF-κB, and the free NF-κB rapidly entered the nucleus from the cytoplasm and bound to specific sequences of target genes (TNF-α, IL-6 and IL-17, etc.).43 It strongly induces the transcription and release of pro-inflammatory mediators, while the products of NF-κB activation, such as TNF-α and IL-1β, in turn activate NF-κB, thus forming a complex regulatory loop that amplifies and perpetuates the inflammatory response. Blocking NF-κB activation is an effective way to control the downstream inflammatory response.
Under the influence of ethanol, the MAPK/NF-κB signaling pathway was activated and numerous inflammatory factors (TNF-α, IL-6, and IL-1β etc.) are produced, leading to neutrophil infiltration and intensified gastric mucosal damage.39,41,46,47 Persistent inflammation is a predisposing factor for gastric mucosal damage. Meanwhile, TNF-α can promote the secretion of IL-1β, IL-6 and other cytokines, triggering the inflammatory response.48 The secretion of IL-1β promotes the recruitment of TNF-α and other cytokines, creating a vicious circle.49 IL-6 secretion activates neutrophils and macrophages at inflammatory sites to trigger oxidative stress, resulting in gastric mucosal damage. The results showed that OVT downregulated the expression of those cytokines in serum and the stomach of gastric mucosal injury mice, which suppressed inflammation. Inflammatory response was by the strict coordination of pro-inflammatory and anti-inflammatory mediators. IL-4 and IL-10 are cytokines with anti-inflammatory properties.50 PGE2 is a defense factor in gastric tissue that stimulates gastric mucus and bicarbonate secretion, thereby strengthening the gastric mucosal barrier, and enhancing mucosal blood flow, so that tissue repair and ulcer healing can be promoted.51,52 In addition, PGE2 is an anti-inflammatory factor that depresses the inflammatory response.53,54 In the present study, OVT pretreatment remarkably enhanced IL-4, IL-10 and PGE2 levels, which alleviated inflammation and promoted gastric repair by promoting the secretion of anti-inflammatory factors (IL-10, IL-4) and PGE2.
Results of western blot showed that OVT with anti-inflammatory activity markedly restrained the phosphorylation of IKK and IκB in the NF-κB pathway as well as the phosphorylation of ERK, p38, and JNK in the MAPK pathway, suggesting that pretreatment of OVT has a mitigating effect on ethanol-induced gastric injury through inhibiting the MAPK/NF-κB pathway. The same pathway has been confirmed in many bioactive substances. For example, it was found that blueberry anthocyanin extracts (BE) prevent gastric cancer by inhibiting the MAPK signaling pathway and NF-κB downstream signaling.44 Fermented soybean attenuated gastric injury induced by ethanol/HCl by inhibiting MAPK and NF-κB signals.40 Astragalus polysaccharides (APS) have anti-inflammatory activity and reduce intestinal inflammation by inhibiting the activation of MAPK and NF-κB inflammatory pathways and suppressing the production of inflammatory factors and chemokines.45
OVT has been shown to reduce oxidative stress in ethanol-induced stomach tissues in mice, and oxidative stress initiates the disruption of mitochondria, which is controlled by the Bcl-2 family genes.34,55 In the initiation of apoptosis, anti-apoptotic members (Bcl-2) and the pro-apoptotic factors (Bax) are the most representative genes controlling apoptosis. Bax can induce apoptosis by upregulating the release of pro-apoptotic factors when stimulated by cell death signal, while Bcl-2 inhibits apoptosis in this process. An apoptotic stimulus activates Bax, which accelerates cell death. The Bcl-2 protein helps cells resist classical apoptotic stimuli and is considered to be an apoptosis suppressor. Bcl-2/Bax is a key index for evaluating cell apoptosis. Studies have shown that the degree of cell damage can be reduced by regulating the expression balance of Bcl-2 and Bax.56 The results of this study showed that gavage of OVT could prevent gastric mucosal damage, which could remarkably enhance mRNA and protein expression of Bcl-2 and significantly decrease mRNA and protein expression of Bax, and OVT could regulate the balance of both and inhibit the occurrence of apoptosis. In conclusion, OVT may protect gastric mucosa by regulating apoptosis.
The above findings suggest that OVT has a protective effect on the gastric mucosa. Based on the dose in the study mice, the human dose was explored through body surface area conversion. According to S. Reagan-Shaw et al.,57 the low dose (100 mg kg−1) in mice is converted to the human dose of 8.10 mg kg−1, which is equivalent to the daily intake of 486 mg for a 60 kg adult. We suggest that OVT should be supplemented orally daily to best achieve its gastric protective effect. Currently, the use scope and dosage of OVT have not been specified in the standards for the use of food nutritional fortifiers. However, OVT and lactoferrin (LF) are both single chain glycoproteins in the transferrin family, with similar amino acid sequences and spatial structures, resulting in a variety of similar biological activities, such as antibacterial, immunomodulatory and anti-inflammatory activities.58 LF has been allowed to be used as a nutritional fortifier for infant formula food, flavored fermented milk and milk containing beverages, with the maximum content of 1 g kg−1.59 Similar to lactoferrin, in the future, we expect promotion of the application of OVT in milk powder, blending it into a functional gastric milk beverage, and meeting the nutritional needs of people with gastric mucosal injury by adjusting the composition and proportion of natural nutrients in the beverage. The biggest advantage of consuming OVT and using drugs is that it is not for the purpose of pretreatment, but to play the role of protecting the gastric mucosa by regulating the body's own physical function, that is, to “maintain” rather than “treat”.
In conclusion, OVT can attenuate ethanol-induced acute gastric mucosal injury in mice. OVT suppresses the release of inflammatory factors by inhibiting activation of the MAPK/NF-κB pathway, thereby decreasing the inflammatory response. At the same time, OVT alleviated oxidative stress and enhanced the antioxidant capacity of the gastric mucosa. In addition, OVT reduced apoptosis by regulating the value of Bcl-2 and Bax for both gene and protein expression, and then accelerated the healing of damaged ulcers. This study has demonstrated for the first time that OVT has anti-inflammatory, antioxidant, and apoptosis reduction effects on ethanol-induced gastric mucosal injury. These results suggest that OVT is a promising foodborne alternative for treating ethanol induced gastric injury.
Conflicts of interest
The authors declare no conflicts of interest.
Acknowledgements
We gratefully acknowledge the financial support provided by Egg Nutrition and Deep Processing Innovation Team of Jiangxi Agricultural University (JXAUCXTD011).
References
- S. Ren, Y. Wei, R. Wang, S. Wei, J. Wen, T. Yang, X. Chen, S. Wu and M. Jing, Rutaecarpine ameliorates ethanol-induced gastric mucosal injury in mice by modulating genes related to inflammation, oxidative stress and apoptosis, Front. Pharmacol., 2020, 11, 1892 Search PubMed.
- R. Wang, Y. Wu, Y. Zhu, S. Yao and Y. Zhu, ANKRD22 is a novel therapeutic target for gastric mucosal injury, Biomed. Pharmacother., 2022, 147, 112649 CrossRef CAS.
- P. Dejban, F. Eslami, N. Rahimi, N. Takzare, M. Jahansouz and A. R. Dehpour, Involvement of nitric oxide pathway in the anti-inflammatory effect of modafinil on indomethacin-, stress-, and ethanol-induced gastric mucosal injury in rat, Eur. J. Pharmacol., 2020, 887, 173579 CrossRef CAS PubMed.
- F. Shen, C.-S. Zhao, M.-F. Shen, Z. Wang and G. Chen, The role of hydrogen sulfide in gastric mucosal damage, Med. Gas Res., 2019, 9, 88 CAS.
- O. Sulaieva and J. Wallace, Trends in development of safe anti-inflammatory drugs, Klin. Med., 2017, 95, 222–227 CrossRef CAS.
- M. J. Song, S. Kim, D. Boo, C. Park, S. Yoo, H. I. Yoon and Y.-J. Cho, Comparison of proton pump inhibitors and histamine 2 receptor antagonists for stress ulcer prophylaxis in the intensive care unit, Sci. Rep., 2021, 11, 1–7 CrossRef.
- L. Yu, R. Li, W. Liu, Y. Zhou, Y. Li, Y. Qin, Y. Chen and Y. Xu, Protective effects of wheat peptides against ethanol-induced gastric mucosal lesions in rats: vasodilation and anti-inflammation, Nutrients, 2020, 12, 2355 CrossRef CAS.
- S. Fernández-Tomé and B. Hernández-Ledesma, Gastrointestinal digestion of food proteins under the effects of released bioactive peptides on digestive health, Mol. Nutr. Food Res., 2020, 64, 2000401 CrossRef.
- X. Wang, Z. Wei and C. Xue, The past and future of ovotransferrin: Physicochemical properties, assembly and applications, Trends Food Sci. Technol., 2021, 116, 47–62 CrossRef CAS.
- G. Zhu, J. Luo, H. Du, Y. Jiang, Y. Tu, Y. Yao and M. Xu, Ovotransferrin enhances intestinal immune response in cyclophosphamide-induced immunosuppressed mice, Int. J. Biol. Macromol., 2018, 120, 1–9 CrossRef CAS PubMed.
- B. Ma, Y. Guo, X. Fu and Y. Jin, Identification and antimicrobial mechanisms of a novel peptide derived from egg white ovotransferrin hydrolysates, LWT–Food Sci. Technol., 2020, 131, 109720 CrossRef.
- G. Zhu, Y. Jiang, Y. Yao, N. Wu, J. Luo, M. Hu, Y. Tu and M. Xu, Ovotransferrin ameliorates the dysbiosis of immunomodulatory function and intestinal microbiota induced by cyclophosphamide, Food Funct., 2019, 10, 1109–1122 RSC.
- J. K. Mann and T. Ndungu, The potential of lactoferrin, ovotransferrin and lysozyme as antiviral and immune-modulating agents in COVID-19, Future Virol., 2020, 15, 609–624 CrossRef.
- X. Zhang, X. Yue, B. Ma, X. Fu, H. Ren and M. Ma, Ultrasonic pretreatment enhanced the glycation of ovotransferrin and improved its antibacterial activity, Food Chem., 2021, 346, 128905 CrossRef PubMed.
- H. Jiao, Q. Zhang, Y. Lin, Y. Gao and P. Zhang, The ovotransferrin-derived peptide IRW attenuates lipopolysaccharide-induced inflammatory responses, BioMed Res. Int., 2019, 2019, 7 Search PubMed.
- H. R. Ibrahim, M. I. Hoq and T. Aoki, Ovotransferrin possesses SOD-like superoxide anion scavenging activity that is promoted by copper and manganese binding, Int. J. Biol. Macromol., 2007, 41, 631–640 CrossRef CAS PubMed.
- E. C. N. Rathnapala, D. U. Ahn and S. Abeyrathne, Functional properties of ovotransferrin from chicken egg white and its derived peptides: A review, Food Sci. Biotechnol., 2021, 30, 619–630 CrossRef CAS PubMed.
- S. H. Moon, J. H. Lee, D. U. Ahn and H. D. Paik,
In vitro antioxidant and mineral–chelating properties of natural and autocleaved ovotransferrin, J. Sci. Food Agric., 2015, 95, 2065–2070 CrossRef CAS PubMed.
- S. Fujimori, Progress in elucidating the relationship between Helicobacter pylori infection and intestinal diseases, World J. Gastroenterol., 2021, 27, 8040 CrossRef CAS.
- M. Guo, H. Yu, M. Meng and C. Wang, Research on the structural characteristics of a novel Chinese Iron Yam polysaccharide and its gastroprotection mechanism against ethanol-induced gastric mucosal lesion in a BALB/c mouse model, Food Funct., 2020, 11, 6054–6065 RSC.
- Y. Zou, X. Cui, Q. Xiang, M. Guo, Y. Liang, Y. Qu and X. Yang, Protective effect of against ethanol-induced gastric ulcer and its mechanism, Med. Sci., 2021, 50, 561–567 Search PubMed.
- S. Simões, R. Lopes, M. C. D. Campos, M. J. Marruz, M. E. M. da Cruz and L. Corvo, Animal models of acute gastric mucosal injury: Macroscopic and microscopic evaluation, Anim. Models Exp. Med., 2019, 2, 121–126 Search PubMed.
- N. Xiao, Y. Zhao, W. He, Y. Yao, N. Wu, M. Xu, H. Du and Y. Tu, Egg yolk oils exert anti-inflammatory effect via regulating Nrf2/NF-κB pathway, J. Ethnopharmacol., 2021, 274, 114070 CrossRef CAS.
- L. Sun, M. Li, S. Zhang, Z. Bao and S. Lin, Mechanism of Ser-Ala-Gly-Pro-Ala-Phe treatment with a pulsed electric field to improve ethanol-induced gastric mucosa injury in mice, Food Funct., 2022, 13, 6716–6725 RSC.
- Y. Zhang, L. Sun, X. Lai, X. Peng, S. Wen, Z. Zhang, Y. Xie, Q. Li, R. Chen and X. Zheng, Gastroprotective effects of extract of Jasminum grandiflorum L., flower in HCl/EtOH-induced gastric mucosal ulceration mice, Biomed. Pharmacother., 2021, 144, 112268 CrossRef CAS.
- H. Cheng, H. Huang, Z. Guo, Y. Chang and Z. Li, Role of prostaglandin E2 in tissue repair and regeneration, Theranostics, 2021, 11, 8836 CrossRef.
- S. Lu, S. Kong, Y. Wang, Z. Hu, L. Zhang and M. Liao, Gastric acid-response chitosan/alginate/tilapia collagen peptide composite hydrogel: Protection effects on alcohol-induced gastric mucosal injury, Carbohydr. Polym., 2022, 277, 118816 CrossRef.
- Q. Tang, Q. Wang, Z. Sun, S. Kang, Y. Fan and Z. Hao, Bergenin Monohydrate Attenuates Inflammatory Response via MAPK and NF-κB Pathways Against Klebsiella pneumonia Infection, Front. Pharmacol., 2021, 12, 651664 CrossRef.
- L. Ouyang, Y. Dan, Z. Shao, S. Yang, C. Yang, G. Liu, W. Zhou and D. Duan, Effect of umbelliferone on adjuvant-induced arthritis in rats by MAPK/NF-κB pathway, Drug Des., Dev. Ther., 2019, 13, 1163 CrossRef.
- S. Ahn, P. Singh, V. Castro-Aceituno, S. Yesmin Simu, Y.-J. Kim, R. Mathiyalagan and D.-C. Yang, Gold nanoparticles synthesized using Panax ginseng leaves suppress inflammatory-mediators production via blockade of NF-κB activation in macrophages, Artif. Cells, Nanomed., Biotechnol., 2017, 45, 270–276 CrossRef.
- R. Yang, J. Li, X. Xu, K. Xu and J. Shi, Preventive and therapeutic effects of Lactobacillus rhamnosus SHA113 and its culture supernatant on alcoholic gastric ulcers, Food Funct., 2021, 12, 7250–7259 RSC.
- S. Ma, Q. Wu, Z. Zhao, J. Xiong, J. Niu, C. Liu, T. Liu, Y. Chai, X. Qu and Z. Ma, Mechanisms of dendrobium officinale polysaccharides in repairing gastric mucosal injuries based on mitogen-activated protein kinases (MAPK) signaling pathway, Bioengineered, 2022, 13, 71–82 CrossRef CAS.
- X. Chen, Y. Zhao, K. Liu, Z. Li, X. Tan, Y. Wang, N. Gao, C. Liu, X. Fang and Y. Wang, Lycopene aggravates acute gastric injury induced by ethanol, Front. Nutr., 2021, 8, 697879 CrossRef.
- M. Raish, A. Ahmad, M. A. Ansari, K. M. Alkharfy, F. I. Aljenoobi, B. L. Jan, A. M. Al-Mohizea, A. Khan and N. Ali, Momordica charantia polysaccharides ameliorate oxidative stress, inflammation, and apoptosis in ethanol-induced gastritis in mucosa through NF-κB signaling pathway inhibition, Int. J. Biol. Macromol., 2018, 111, 193–199 CrossRef.
- Y. Aratani, Myeloperoxidase: its role for host defense, inflammation, and neutrophil function, Arch. Biochem. Biophys., 2018, 640, 47–52 CrossRef PubMed.
- G. Ndrepepa, Myeloperoxidase–A bridge linking inflammation and oxidative stress with cardiovascular disease, Clin. Chim. Acta, 2019, 493, 36–51 CrossRef PubMed.
- Y. Kargapolova, S. Geißen, R. Zheng, S. Baldus, H. Winkels and M. Adam, The enzymatic and non-enzymatic function of myeloperoxidase (MPO) in inflammatory communication, Antioxidants, 2021, 10, 562 CrossRef PubMed.
- F. Heidari, T. Komeili-Movahhed, Z. Hamidizad and A. Moslehi, The protective effects of rosmarinic acid on ethanol-induced gastritis in male rats: antioxidant defense enhancement, Res. Pharm. Sci., 2021, 16, 305 CrossRef.
- J. Zhou, G. Wang, R. Han, R. Wang, Y. Kong, R. Zhang, L. Hou and M. Meng, Glycopeptides from Paecilomyces sinensis ameliorate ethanol-induced gastric ulcers via anti-inflammation and the miR-9–5p-MEK/ERK signaling pathway, Food Funct., 2021, 12, 7664–7675 RSC.
- M. Lee, D. Kim, H. Kim, S. Jo, O.-K. Kim and J. Lee, Gastro-protective effect of fermented soybean (Glycine max (L.) Merr.) in a rat model of ethanol/HCl-induced gastric injury, Nutrients, 2022, 14, 2079 CrossRef PubMed.
- J. Zhang, S. Sayakoummane, S. A. Kim, J. S. Lee, E. S. Choung, E. S. Kim, S.-G. Lee, J. Yum, B.-H. Lee and S. Lee, Hymenocallis littoralis ameliorates inflammatory responses in LPS-stimulated RAW264. 7 cells and HCl/EtOH-induced gastric mucosal injury via targeting the MAPK pathway, J. Ethnopharmacol., 2022, 295, 115400 CrossRef PubMed.
- N. Zhou, Y. Yao, N. Wu, H. Du, M. Xu, Y. Zhao and Y. Tu, VF–4 and DR–8 derived from salted egg white inhibit inflammatory activity via NF–κB/PI3K–Akt/MAPK signal transduction pathways in HT–29 cells induced by TNF–α, Mol. Nutr. Food Res., 2022, 66, 2100682 CrossRef PubMed.
- M. A. Khan and M. J. Khan, Nano-gold displayed anti-inflammatory property via NF-κB pathways by suppressing COX-2 activity, Artif. Cells, Nanomed., Biotechnol., 2018, 46, 1149–1158 CrossRef.
- C. Shu, J. Tian, X. Si, X. Xie, B. Li and D. Li, Blueberry anthocyanin extracts protect against Helicobacter pylori-induced peptic epithelium injuries both in vitro and in vivo: the key role of MAPK/NF-κB pathway, Eur. J. Nutr., 2022, 1–11 Search PubMed.
- N. Dong, X. Li, C. Xue, L. Zhang, C. Wang, X. Xu and A. Shan, Astragalus polysaccharides alleviates LPS–induced inflammation via the NF–κB/MAPK signaling pathway, J. Cell. Physiol., 2020, 235, 5525–5540 CrossRef PubMed.
- K. Ding, F. Zhang, G. Qi, M. L. Lin, M. Chen, Y. Chen, J. Zheng and F. Zhou, ZFP36L1 Promotes gastric cancer progression via regulating JNK and p38 MAPK signaling pathways, Recent Pat. Anti-Cancer Drug Discovery, 2022, 18, 80–91 Search PubMed.
- F. T. Crews, J. Zou and L. G. Coleman Jr., Extracellular microvesicles promote microglia–mediated pro–inflammatory responses to ethanol, J. Neurosci. Res., 2021, 99, 1940–1956 CrossRef PubMed.
- M. Li, R. Lv, X. Xu, Q. Ge and S. Lin, Tricholoma matsutake-Derived Peptides Show Gastroprotective Effects against Ethanol-Induced Acute Gastric Injury, J. Agric. Food Chem., 2021, 69, 14985–14994 CrossRef.
- Y. Sun, N. Ma, J. Yi, L. Zhou and S. Cai, Gastroprotective effect and mechanisms of Chinese sumac fruits (Rhus chinensis Mill.) on ethanol-induced gastric ulcers in mice, Food Funct., 2021, 12, 12565–12579 RSC.
- M. Norouzi, M. Pirestani, E. Arefian, A. Dalimi, J. Sadraei and H. Mirjalali, Exosomes secreted by Blastocystis subtypes affect the expression of proinflammatory and anti-inflammatory cytokines (TNFα, IL-6, IL-10, IL-4), Front. Med., 2022, 9, 1–11 Search PubMed.
- F. Heidari, T. Komeili-Movahhed, Z. Hamidizad and A. Moslehi, The protective effects of rosmarinic acid on ethanol-induced gastritis in male rats: antioxidant defense enhancement, Res. Pharm. Sci., 2021, 16, 305 CrossRef PubMed.
- J. Zhou, G. Wang, R. Han, R. Wang, Y. Kong, R. Zhang, L. Hou and M. Meng, Glycopeptides from Paecilomyces sinensis ameliorate ethanol-induced gastric ulcers via anti-inflammation and the miR-9–5p-MEK/ERK signaling pathway, Food Funct., 2021, 12, 7664–7675 RSC.
- H. Yang, J. Sun, Y. Li, W.-M. Duan, J. Bi and T. Qu, Human umbilical cord-derived mesenchymal stem cells suppress proliferation of PHA-activated lymphocytes in vitro by inducing CD4+ CD25highCD45RA+ regulatory T cell production and modulating cytokine secretion, Cell. Immunol., 2016, 302, 26–31 CrossRef.
- R. Kim, M. Emi and K. Tanabe, Cancer cell immune escape and tumor progression by exploitation of anti-inflammatory and pro-inflammatory responses, Cancer Biol. Ther., 2005, 4, 924–933 CrossRef PubMed.
- M. S. Lokman, D. Zaafar, H. A. Althagafi, M. M. Abdel Daim, A. Theyab, A. Hasan Mufti, M. Algahtani, O. A. Habotta, A. A. Alghamdi and K. F. Alsharif, Antiulcer activity of proanthocyanidins is mediated via suppression of oxidative, inflammatory, and apoptotic machineries, J. Food Biochem., 2022, 46, e14070 CrossRef PubMed.
- İ. İlhan, H. Aşçi, N. Hasseyid, H. K. Doğan, Ş. Ağirca, M. Altintaş and M. Y. Tepebasi, Irbesartan decreased mitochondrial stress related apoptosis in cisplatin induced acute kidney injury via regulating Bcl-2/Bax signaling, Mol. Biol. Rep., 2022, 49, 6125–6133 CrossRef.
- S. Reagan-Shaw, M. Nihal and N. Ahmad, Dose translation from animal to human studies revisited, FASEB J., 2008, 22, 659–661 CrossRef.
- R. Galla, P. Grisenti, M. Farghali, L. Saccuman, P. Ferraboschi and F. Uberti, Ovotransferrin Supplementation Improves the Iron Absorption: An In Vitro Gastro-Intestinal Model, Biomedicines, 2021, 9, 1543 CrossRef.
- B. Niaz, F. Saeed, A. Ahmed, M. Imran, A. A. Maan, M. K. I. Khan, T. Tufail, F. M. Anjum, S. Hussain and H. A. R. Suleria, Lactoferrin (LF): A natural antimicrobial protein, Int. J. Food Prop., 2019, 22, 1626–1641 CrossRef.
|
This journal is © The Royal Society of Chemistry 2023 |
Click here to see how this site uses Cookies. View our privacy policy here.