Development of sodium alginate–aloe vera hydrogel films enriched with organic fibers: study of the physical, mechanical, and barrier properties for food-packaging applications
Received
2nd August 2023
, Accepted 15th October 2023
First published on 30th October 2023
Abstract
Hydrogels from a botanical origin can be beneficially utilized in the development of edible and biodegradable food-packaging films. Polymer hydrogels are biodegradable and non-toxic in nature and have the potential to replace conventional plastics. In the present study, composite films based on alginate and aloe vera were reinforced with two hydrogel-forming polymers, namely gum tragacanth (GT) and hydroxypropyl methylcellulose (HPMC). A comparative study to assess their reinforcing effect in improving the physical properties as well as mechanical properties of the films was carried out. It was observed that both GT and HPMC significantly improved the tensile strength (TS) of the composite films whereby the TS increased to a maximum value of 68.25 MPa at a 12% concentration of GT and 52.19 MPa at a 4% concentration of HPMC. The flexibility of the films was also improved by incorporating the two hydrogel-forming polymers that caused an increase in the thickness, moisture content, swelling index, and water vapor permeability (WVP) of the composite films. However, the oxygen permeability (OP) was reduced by the addition of GT and the value decreased from 1.46 × 10−15 to 0.45 × 10−15 g m−1 s−1 Pa−1 at the maximum concentration of GT. The solubility of the films also decreased as a result of adding GT and was reduced to 23.12% at a 14% concentration of GT.
Sustainability spotlight
Due to their biodegradable nature, alginate–aloe vera composite films reinforced with gum tragacanth and HPMC have the potential to replace pollution-causing synthetic polymer-based films in food-packaging applications. Furthermore, the water-soluble HPMC-reinforced film can be utilized as an edible food-packaging material as its better solubility will improve digestibility.
|
1. Introduction
While bio-based hydrogels have been utilized in food packaging, superabsorbent biopolymer-based hydrogels still find limited use in the food industry. The recent trends show an increasing interest in innovative technologies that utilize bio-based hydrogels to reduce the use of fossil fuel-derived nonbiodegradable synthetic plastic materials.1 Some hydrophilic polymer materials have the ability to swell in water by absorbing large quantities of water, in many cases several times their own weight, to form three-dimensional (3D) crosslinked hydrogels.2 Films based on these hydrogels can absorb large quantities of water when they come in contact with fluid materials. Due to their easy and abundant availability, biodegradability, and biocompatibility, biopolymer-based hydrogels have found use in various medical, pharmaceutical, and food packing industry applications.3
Biopolymers derived from renewable resources, like algal biomass, are an important source of hydrogels. In particular, algal polysaccharides have received much attention due to their extraordinary biodegradability and compatibility.4 Alginate extracted from brown algal seaweed is an anionic polymer consisting of copolymer blocks of β-D-mannuronic acid and α-L-guluronic acid. Alginates are non-toxic, biocompatible, and have film-forming properties, and they are therefore utilized in films.5 However, films purely based on sodium alginate lack mechanical strength and functional properties; therefore, the blending of alginate films with other polymer materials is necessary to enhance their mechanical and functional properties to make them suitable for food-packaging applications.6 The properties of alginate films can be improved by mixing them with two or more naturally occurring polymer materials.7 Aloe vera (AV) (Aloe barbadensis Miller) is one such natural polysaccharide and contains mainly water and some active compounds, like phenolics, amino acids, sugars, and saponins, which provide it with remarkable therapeutic and functional characteristics.8
Gum tragacanth (GT) is another naturally occurring polymeric hydrogel capable of blending with synthetic polymers.9 It is a complex heterogenous anionic polysaccharide extracted from various species of Astragalus plants. GT has been used as a thickener and emulsifier in the food industry but its ability to serve as a component of composite food-packaging films has not been studied thoroughly.10 Cellulose and its ester derivatives play an important role in the reinforcement of many composite biomaterials. Hydroxypropyl methylcellulose (HPMC) is a hydrogel-forming biodegradable polymer with industrial significance. It is capable of forming transparent, flexible, oil-resistant, low-cost water-soluble edible films. These unique characteristics of HPMC make it a promising material for use in food-packaging films.11,12
This study is a continuation of the research work that started with the development of alginate–aloe vera composite films for food packaging. In our previous study, the optimum concentration of alginate and aloe vera were determined that gave the desired film-forming properties.13 These films were reinforced by the addition of the hydrogel-forming polymers GT and HPMC. The present study was carried out to perform a comparative assessment of the reinforcing behavior of GT and HPMC and their influence on other physicochemical and functional properties of alginate–aloe vera films.
2. Materials and methods
2.1. Materials
Brown algae (Sargassum spp.) was collected from the Karachi coast, Pakistan, washed, dried, cut into small pieces, and stored under refrigeration until use. Aloe vera (Aloe barbadensis Miller) leaves were collected from plants growing in the premises of Karachi University. HPMC (Mw = 4.3 × 105 Da, Methocel® F50) was obtained from Dow Chemical Co. (Midland, MI, USA). Tragacanth gum (GT) was procured from a local market. All the analytical grade laboratory chemicals and sorbitol (purity ≥ 98%) were acquired from Sigma-Aldrich Chemical Ltd.
2.2. Extraction of alginate
For the extraction of sodium alginate from brown seaweed, the method of Gholamipoor et al. (2013) was followed.14
2.3. Extraction of aloe vera gel
After thoroughly washing with water, the outer peel of the aloe vera leaves was removed with a knife. The inner gel collected after removing the peel was mixed at slow speed in a blender and sieved through a 200-mesh screen. The filtrate was centrifuged at 4800 rpm for 10 min. The supernatant clear liquid of aloe vera gel was used for the preparation of films for the further experiments.
2.4. Films preparation
The alginate–aloe vera (AA) films were made according to a previously reported method.13 Varying concentrations of GT and HPMC (2% to 14%) were blended into the alginate–aloe vera solution (Table 1). The ternary solution was again heated with mixing for another 30 min. This homogenized solution was cast onto Petri dishes and oven dried at 40 °C for 24 h. Fig. 1 shows the flow diagram for the development of the ternary blend films.
Table 1 Details of the generated films: the film's code and ratios
Samples |
Alginate% |
Aloe vera% |
GT/HPMC% |
AA |
90% |
10% |
0% |
AA/GT2 |
90% |
10% |
2% |
AA/GT4 |
90% |
10% |
4% |
AA/GT6 |
90% |
10% |
6% |
AA/GT8 |
90% |
10% |
8% |
AA/GT10 |
90% |
10% |
10% |
AA/GT12 |
90% |
10% |
12% |
AA/GT14 |
90% |
10% |
14% |
AA/HPMC2 |
90% |
10% |
2% |
AA/HPMC4 |
90% |
10% |
4% |
AA/HPMC6 |
90% |
10% |
6% |
AA/HPMC8 |
90% |
10% |
8% |
AA/HPMC10 |
90% |
10% |
10% |
AA/HPMC12 |
90% |
10% |
12% |
AA/HPMC14 |
90% |
10% |
14% |
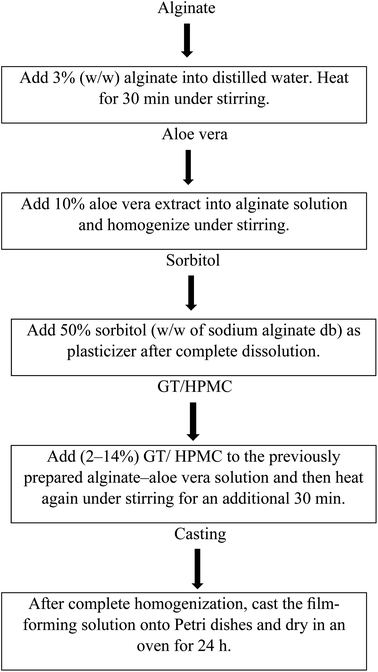 |
| Fig. 1 Flow diagram showing the preparation of the alginate–aloe vera films. | |
2.5. Film thickness
The film thickness was measured by a digital micrometer (Mitutoyo, Tokyo, Japan) by taking readings at five different points of the films. The mean film thickness was then calculated from these readings.
2.6. Moisture content
Previously weighed film samples were dried in an oven at 110 °C for 24 h to remove the moisture. These samples were weighed again after placing in a desiccator for 30 min. The moisture content was calculated using the following equation.15 | 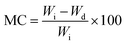 | (1) |
2.7. Swelling capacity
Film samples were cut into square pieces of 2 × 2 cm dimensions, weighed, and placed in distilled water at room temperature for 30 min. The water adhering on the films' outer surface was absorbed by filter paper. The sample pieces were weighed again and the swelling capacity was calculated with the help of the following equation.16 | 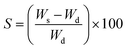 | (2) |
2.8. Solubility
The film solubility was determined by following the method of Jangchud and Chinnan.17 Here, 2 × 2 cm square pieces of films were weighed and dipped in 20 ml distilled water in 50 ml flasks. The flasks were shaken in a water bath for 24 h at 25 °C. The remaining undissolved films pieces were dried at 80 °C and weighed. The solubility was evaluated as per the following equation. |  | (3) |
2.9. Scanning electron microscopy (SEM)
The films' microstructure was studied with the help of scanning electron microscopy (SEM; JSM, 6380A, JEOL, Japan) at an accelerating voltage of 10 kV. The films' surface and cross-section were photographed at 1000× magnification to study the morphological structure of the films.
2.10. Water vapor permeability
The method of Hadi et al. (2022) was followed for determining the water vapor permeability (WVP) values of the films.13
2.11. Oxygen permeability
The oxygen permeability of the films was determined using the method of Ayranci et al. (2003).18
2.12. Mechanical properties
The previously reported method of Nawab et al. (2017) was used to investigate the mechanical properties of the films.19
2.13. Biodegradation analysis
The biodegradation behavior of the films was studied using the method of Martucci.20 Here, 2 cm × 4 cm rectangular pieces of the films were dried at 105 °C for 24 h and their initial weights (Wi) were taken. These samples were buried under garden soil containing a compost fertilizer. The degrading samples were taken out at regular intervals of 4 days and weighed (Wt). The percent weight loss at each interval and finally at the completion of the experiment at 32 days was calculated using the equation below. | 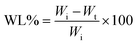 | (4) |
2.14. Statistical analysis
The data from triplicate analysis of the samples were subjected to one way analysis of variance (ANOVA) and Duncan's multiple range tests to differentiate the treatments at p < 0.05. SPSS version 17.01 for Windows (SPSS Inc., Chicago, IL, USA) was utilized for the statistical analyses.
3. Results and discussion
3.1. Thickness
The film thickness plays an important role in defining the physicochemical and mechanical properties of a film. The shelf life of packaged food is also affected by the film thickness as the barrier properties of the film largely depend on the composition as well as the thickness of the film. Here, the film thickness increased from 0.067 (AA) for the control to 0.084 mm at a maximum GT concentration (AA/GT14). This increase in thickness was due to the extraordinary capacity of GT to absorb water and swell. Similarly, the film thickness increased from the control's 0.067 mm to 0.092 mm at the highest concentration of HPMC (AA/HPMC14). As the concentration of the various film components increased, the total solids content of the film-forming solution also increased, which resulted in an increase in film thickness. GT and HPMC both are hydrophilic polymers with a very high capacity to absorb large amounts of water. In earlier studies, it was reported that as the total solids in the film-forming dispersion increased, the thickness of HPMC films also increased.21,22
3.2. Moisture content
Table 2 shows that the films' moisture content increased from 6.61% in (AA) to 9.55% at the highest concentration of GT (AA/GT14). A similar trend of increasing moisture content with the increasing concentration of HPMC could be seen in the HPMC-reinforced films. The moisture content increased from the control's (AA) 6.61% to 9.35% at 14% HPMC (AA/HPMC14). Since GT and HPMC both have a high affinity for water, as their concentration increased the films' moisture content also increased. Both GT and HPMC contain hydroxyl and carboxyl groups, which are responsible for their high hydrophilic nature, therefore as their concentration in the film increased, the overall hydrophilicity of the film increased, resulting in an increasing moisture content.23,24
Table 2 Effect of GT/HPMC on the thickness, moisture content, swelling index, and solubility of the alginate–aloe vera composite filmsa,b
Film Samples |
Thickness (mm) |
Moisture Content (%) |
Swelling Index (%) |
Solubility (%) |
Values are means ± SD of triplicates.
Values in the same column with a different superscript are significantly different (p < 0.05).
|
AA |
0.067 ± 0.002a |
6.61 ± 0.01a |
162.34 ± 2.45b |
45.23 ± 1.15d |
AA/GT2 |
0.070 ± 0.001a |
5.42 ± 0.02a |
106.81 ± 1.68a |
43.03 ± 1.26b |
AA/HPMC2 |
0.074 ± 0.003c |
5.57 ± 0.21b |
109.24 ± 2.54b |
52.34 ± 2.28a |
AA/GT4 |
0.073 ± 0.003b |
5.75 ± 0.03a |
124.45 ± 2.23c |
40.46 ± 1.67c |
AA/HPMC4 |
0.076 ± 0.002c |
6.25 ± 0.26c |
110.36 ± 3.32e |
58.42 ± 1.89b |
AA/GT6 |
0.075 ± 0.001a |
5.95 ± 0.21a |
154.78 ± 1.26a |
37.97 ± 2.37d |
AA/HPMC6 |
0.078 ± 0.002c |
6.36 ± 0.21a |
138.53 ± 2.29c |
61.16 ± 2.78b |
AA/GT8 |
0.077 ± 0.003d |
6.32 ± 0.19b |
164.56 ± 1.27a |
32.86 ± 3.54d |
AA/HPMC8 |
0.080 ± 0.001c |
6.87 ± 0.17c |
166.13 ± 2.19d |
69.65 ± 2.45b |
AA/GT10 |
0.079 ± 0.004c |
7.12 ± 0.11b |
198.56 ± 3.65b |
29.78 ± 2.89e |
AA/HPMC10 |
0.083 ± 0.001b |
7.02 ± 0.23d |
221.44 ± 2.27d |
75.76 ± 1.69b |
AA/GT12 |
0.082 ± 0.003d |
7.36 ± 0.12b |
236.99 ± 4.23a |
25.16 ± 2.36e |
AA/HPMC12 |
0.088 ± 0.002e |
7.23 ± 0.21e |
324.56 ± 3.76c |
86.62 ± 3.12d |
AA/GT14 |
0.084 ± 0.004c |
9.55 ± 0.11b |
452.12 ± 2.89a |
23.12 ± 2.88b |
AA/HPMC14 |
0.092 ± 0.005f |
9.35 ± 0.24d |
392.46 ± 4.25d |
88.23 ± 3.92e |
3.3. Swelling index
The swelling property of a film has a direct effect on the quality of the packed food as the barrier and mechanical properties of the film largely depend on its swelling behavior. Table 2 shows that the values increased from 162.34% (AA) to 452.12% when the highest concentration of gum was added (AA/GT14). The highly branched polysaccharide structure of GT was responsible for its high swelling and gelling behavior, which led to increasing the swelling capacity of the films with increasing the GT concentration.25
In the case of the HPMC films, the increasing value was from 162.34% (AA) to 392.46% at the highest HPMC concentration (AA/HPMC14). This increase was expected as both GT and HPMC are hydrogel-forming polymers and one of the most important functional properties of hydrogels is their high water absorption capacity, which directly influences the swelling behavior.26
3.4. Solubility
The film solubility is an important parameter responsible for determining the suitability of a film for a particular food-packaging application. The solubility of the GT films was reduced from 45.23% (AA) to 23.12% (AA/TG14), as shown in Table 2; whereas in the case of HPMC, the solubility increased from 45.23% to 88.23% at the highest concentration of HPMC (AA/HPMC14). The reduction in solubility with increasing the concentration of GT was due to the presence of the water-insoluble compound bassorin in GT, which was responsible for the overall decrease in the GT film solubility. The reason for the increase in solubility of the HPMC films with increasing the HPMC concentrations was the presence of a large number of hydrophilic hydroxyl groups in HPMC.27 Various food-packaging applications require varying degrees of solubility of the packaging material. For example, if the edible packaging material is to be consumed along with the packed foodstuff, a higher solubility is preferable. Whereas water insolubility and water resistance will be preferred properties for a packaging film meant to protect the foodstuff and enhance its shelf life.28 Therefore, the target property of high or low solubility depends on the nature of the end use of the packaging films.
3.5. Microstructure of the films
Scanning electron microscopy (SEM) was performed to study the morphological microstructure of the alginate–aloe vera films reinforced with GT. The microstructure gives an indication of the compatibility and miscibility of the various components of the composite film (Fig. 2a and b). The surface micrographs of both the alginate–aloe vera and GT-reinforced films were smooth and uniform, which shows that the film network after adding GT to the alginate–aloe vera film-forming system was homogenous. The incorporation of GT into the alginate–aloe vera matrix did not result in an irregular microstructure, as indicated by the absence of any breaks, cracks, or openings in the structure of the film. Different concentrations of GT were uniformly dispersed in the film-forming matrix without deforming the film's surface microstructure, which shows the compatibility of GT as a reinforcing agent with the alginate–aloe vera film-forming matrix. The micrographs of the cross-sections of the films also did not show any irregularity or breaks in the film microstructure, indicating the compatibility between the components of the films due to the crosslinking between functional groups of the alginate–aloe vera and GT. The morphology of the alginate–aloe vera films reinforced with HPMC was also investigated by SEM analysis. Here also the micrographs of the film surface and its cross-section showed the uniform microstructure of the films up to a 10% concentration of HPMC. Both GT and HPMC were compatible with the film-forming matrix of alginate–aloe vera as indicated by the uniform microstructure of the composite films, with the only difference being that at the two higher most concentration of HPMC, that is at 12% and 14%, the morphology showed some irregularity in the cross-section micrographs of the films. This could be attributed to the lack of complete miscibility and dispersibility of HPMC in the film matrix at a concentration higher than 10%. Similar observations were reported in some previous studies.29,30
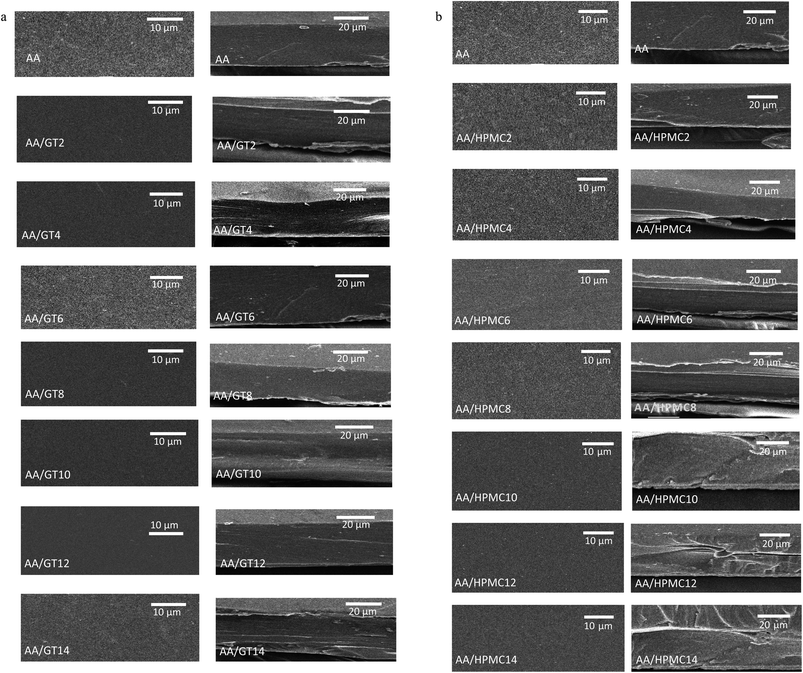 |
| Fig. 2 (a) Scanning electron micrography (SEM) images of the surface (left) and cross-section (right) of the alginate–aloe vera composite films. The bars in the left and right images indicate 10 μm and 20 μm, respectively. Shown are the film samples: AA, AA/GT2, AA/GT4, AA/GT6, AA/GT8, AA/GT10, AA/GT12, and AA/GT14. (b) Scanning electron micrography (SEM) images of the surface (left) and cross-section (right) of the alginate–aloe vera composite films. The bars in the left and right images indicate 10 μm and 20 μm, respectively. Shown are the film samples: AA, AA/HPMC2, AA/HPMC4, AA/HPMC6, AA/HPMC8, AA/HPMC10, AA/HPMC12, and AA/HPMC14. | |
3.6. Water vapor permeability (WVP)
A film's ability to act as a barrier against the transference of water vapor through it has a direct effect on the quality and shelf life of the packaged food. Fig. 3 indicates that the WVP increased from 1.26 × 10−10 (AA) to 8.35 × 10−10 g m−1 s−1 Pa−1 in (AA/GT14). While in HPMC it increased from 1.26 × 10−10 to 4.89 × 10−10 gm−1 s−1 Pa−1 in (AA/HPMC14). Both GT and HPMC thus caused an increase in the WVP of the films, but the effect of GT was more pronounced. The two important parameters affecting the water vapor permeability are the solubility and the rate of diffusion.31 The free volume within the polymer matrix that is available for the diffusion of water vapor is responsible for the permeability.32 In most edible films, the solubility coefficient and diffusion rate act as a driving force for the permeation of water vapor.33 GT and HPMC both are hydrophilic gel-forming polymers, but GT has a higher affinity for water, which resulted in a higher WVP of the films as GT can swell by absorbing a large quantity of water, which can create a gap between the polymer matrix of the alginate–aloe vera films, which then facilitates the transport of water vapor through the films. This behavior of GT was in agreement with the results of a study on starch/TG/nanoclay films.34 Pastor et al. (2010) also reported that the WVP of HPMC films increased with incorporating different concentrations of methanolic compounds extracted from an organic gum.35
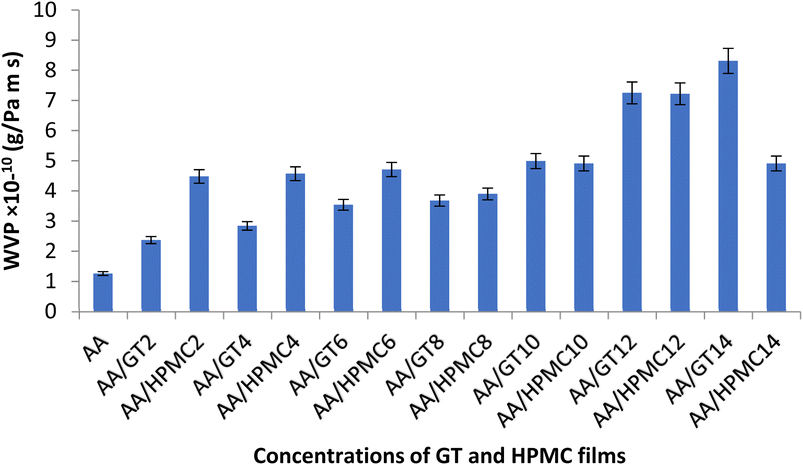 |
| Fig. 3 Effect of GT and HPMC on the WVP of the alginate–aloe vera films. | |
3.7. Oxygen permeability
The ability of a packaging material to act as a barrier against gases has a direct bearing on the shelf life of the packaged food products; therefore, packaging materials should have a high barrier ability.36 Packaging materials for food stuffs should have a good oxygen barrier ability to protect the packed food from damage due to oxidation so that the shelf life of the packaged food can be enhanced. Polysaccharides-based films show considerable resistance to oxygen permeability (OP) at medium to low relative humidity. These films possess superior elasticity.37 Gums of plant origin are examples of polysaccharides that have been used in packaging on account of their good oxygen barrier properties.38 In the present study, the effect of increasing concentrations of GT/HPMC on the oxygen permeability of alginate–aloe vera composite films was studied. It was found that the OP decreased as the concentration of GT increased. The value of OP at AA/GT2 was 1.46 × 10−15, which decreased to 0.45 × 10−15 g m−1 s−1 Pa−1 at a maximum concentration of AA/GT14. Whereas in the case of HPMC, these values showed a reduction in OP, which decreased from that in AA/HPMC2 at 1.28 × 10−15 to 0.52 × 10−15 g m−1 s−1 Pa−1 for AA/HPMC14. The value of OP at the maximum concentration of GT was found to be lower than the OP value of the control at 0.49 × 10−15 g m−1 s−1 Pa−1. The reason for the decrease in OP, or in other words the improvement in the barrier ability of the films with the increasing concentration of GT, was the highly crystalline structure of gum tragacanth, which is an organic fiber. The high crystallinity of fibers restricts the passage of oxygen through the fiber material. Further, fibers that are small in size are capable of packing with one another closely enough not to leave much space for the passage of oxygen through the film matrix.39 In our study, it was observed that the addition of GT and HPMC to the alginate–aloe vera composite films resulted in a decrease in the oxygen permeability values of the films. This means that both the added organic fibers improved the oxygen barrier performance of the composite films, but the impact of GT in enhancing the oxygen barrier ability was more profound compared to that of HPMC (Fig. 4).
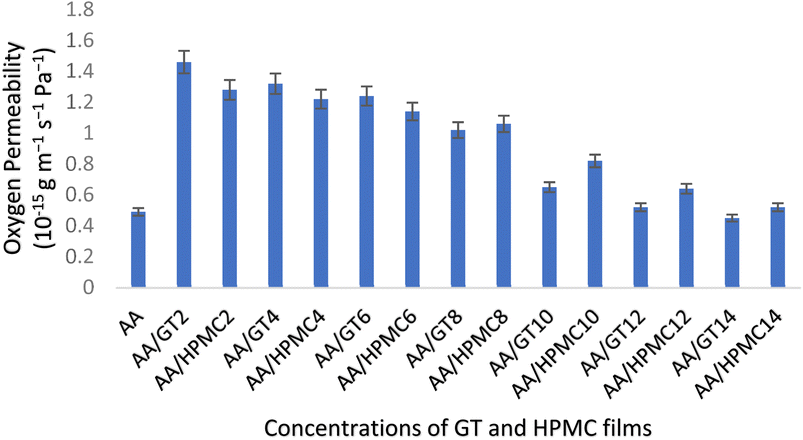 |
| Fig. 4 Effect of GT and HPMC on the oxygen permeability of the alginate–aloe vera films. | |
3.8. Mechanical properties of the films
Strength and flexibility are important requirements for food-packaging films. The present study was carried out to perform a comparative assessment of the reinforcing behavior of the two hydrogels GT and HPMC, and to evaluate their effects on the tensile strength (TS) and flexibility of composite films. It was observed that both GT and HPMC positively influenced these two important physical properties and resulted in a remarkable improvement in strength and flexibility of the films. GT caused an almost threefold improvement in TS at a 12% concentration of GT; whereas HPMC gave a more than twofold increase in TS only at a 4% concentration. These concentrations of 12% for GT and 4% for HPMC were their optimum values, as increasing the concentration beyond these levels resulted in a decrease in tensile strength of the films. Fig. 5a shows that the TS value increased from 22.15 (AA) to 68.25 MPa (AA/GT12). In the case of HPMC, the value increased from 22.15 (AA) to 52.19 MPa at a 4% concentration of HPMC. A similar trend was observed for the effect of GT and HPMC on the elasticity of the films, as both contributed positively toward the flexibility of the films. The values increased from 0.8% (AA) to 5.57% (AA/GT12). The elongation at break (EB) value rose from 0.8% in (AA) to 3.21% at a 4% concentration of HPMC (Fig. 5b). In the case of the elasticity as well, the concentrations of 12% GT and 4% HPMC were threshold values, because the EB values started decreasing when the concentration was further increased. The apparent reason for this behavior was the large molecular size of both GT and HPMC, which restricted their ability to be accommodated within the polymer matrix of the alginate beyond a certain concentration. The increase in tensile strength with increasing gum concentration was in conformity with some previous studies of guar gum hydrogel films and tapioca starch/decolorized hsian-tsao leaf gum films.40,41 The incorporation of locust bean gum into tragacanth-based films resulted in an improvement in the physical and mechanical properties of the films.25 The reinforcing effect of GT and HPMC was due to their perfect compatibility and miscibility with the alginate–aloe vera matrix as reflected in the microstructure of the films studied by SEM. The surface morphology as well as cross-sections of the films both showed a homogenous and uniform structure in the control and GT/HPMC-reinforced films, which indicated the compatibility of these two biopolymers with the alginate matrix due to the similarity in their chemical structure. Both GT/HPMC possess hydroxyl groups, which can interact with the carboxyl and hydroxyl group present in alginate.
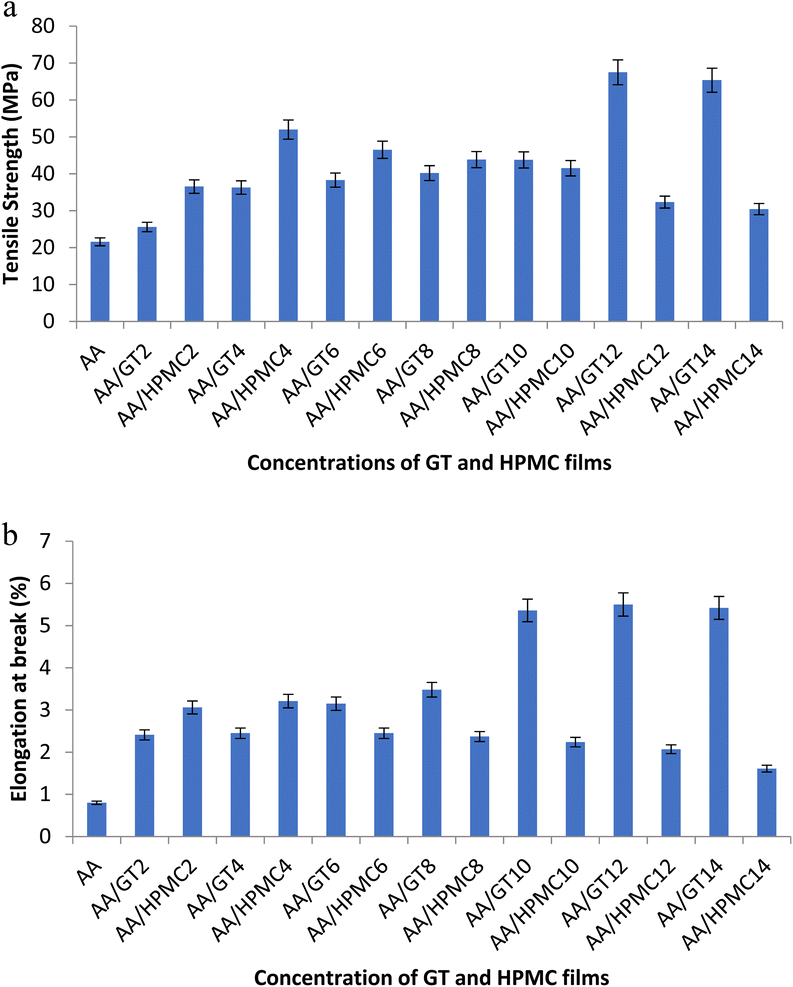 |
| Fig. 5 (a) Effect of GT and HPMC on the mechanical property of the alginate–aloe vera films. (b) Effect of GT and HPMC on the flexibility of the alginate–aloe vera films. | |
3.9. Biodegradation
The most important criterion for the suitability of food-packaging films in the future is their ability to degrade in the environment so that they can replace the nonbiodegradable synthetic polymer-based packaging films presently used that are causing environmental pollution. A comparative study of the rate of biodegradation of the alginate–aloe vera films reinforced with GT and HPMC was performed. Table 3 shows that HPMC increased the rate of biodegradation of the films compared with control, whereas GT slowed down the biodegradation process. At the end of the trial period, that is, at day 32, the films containing HPMC at a maximum concentration (AA/HPMC14) had degraded almost completely, with a 95.12% loss in weight of the film sample; whereas the films reinforced with GT had decomposed at a much slower rate, with a 70.56% weight loss on day 32. The weight loss in the control film (AA) was 90.59%. The reason for the higher biodegradability of the HPMC films was their higher solubility compared to both the control as well as GT-containing films. As discussed before, GT contains the water-insoluble component bassorin, which reduces the solubility of the GT films and subsequently resulted in the slower rate of biodegradation of the films.
Table 3 Effect of GT/HPMC on biodegradation (% weight loss) of the alginate–aloe vera composite filmsa,b
Film Samples |
Day 4 |
Day 8 |
Day 12 |
Day 16 |
Day 20 |
Day 24 |
Day 28 |
Day 32 |
Values are means ± SD of triplicates.
Values in the same column with a different superscript are significantly different (p < 0.05).
|
AA |
20.21 ± 0.12a |
29.22 ± 0.12a |
39.24 ± 0.02c |
48.49 ± 1.81b |
58.23 ± 1.98b |
69.78 ± 1.35b |
75.87 ± 1.98c |
90.59 ± 1.96d |
AA/GT2 |
21.54 ± 0.47e |
27.58 ± 0.02b |
38.56 ± 0.05b |
49.52 ± 1.54b |
56.54 ± 1.23a |
64.56 ± 1.98b |
70.58 ± 2.96a |
78.38 ± 2.76b |
AA/HPMC2 |
13.59 ± 0.65a |
23.27 ± 0.09b |
41.87 ± 0.86e |
53.18 ± 1.85d |
64.65 ± 1.19b |
69.98 ± 2.56c |
74.62 ± 2.23c |
79.97 ± 2.87b |
AA/GT4 |
19.25 ± 0.28b |
26.43 ± 0.02c |
37.77 ± 1.25b |
47.48 ± 1.39a |
56.87 ± 2.48a |
63.77 ± 1.64b |
70.78 ± 1.97a |
77.65 ± 1.96c |
AA/HPMC4 |
14.33 ± 0.72a |
25.59 ± 0.07b |
38.99 ± 1.48e |
52.81 ± 1.57c |
63.61 ± 1.89b |
74.87 ± 1.89c |
77.82 ± 3.13d |
82.56 ± 1.43d |
AA/GT6 |
18.15 ± 0.56b |
27.12 ± 0.08c |
36.64 ± 0.51b |
46.89 ± 2.15d |
55.58 ± 1.35a |
62.23 ± 2.44b |
69.68 ± 2.89c |
76.62 ± 3.15c |
AA/HPMC6 |
15.63 ± 0.15b |
27.61 ± 0.04b |
36.84 ± 0.12c |
48.71 ± 1.46b |
65.94 ± 1.14c |
74.45 ± 1.64d |
78.78 ± 1.64d |
85.69 ± 1.73d |
AA/GT8 |
17.56 ± 0.42c |
26.83 ± 0.01a |
36.87 ± 0.25b |
45.78 ± 1.86a |
54.57 ± 2.32b |
61.57 ± 2.49a |
68.25 ± 1.53b |
75.63 ± 3.18c |
AA/HPMC8 |
16.59 ± 0.65b |
28.89 ± 0.05d |
38.81 ± 0.58a |
49.13 ± 1.47c |
59.51 ± 2.58c |
72.39 ± 2.85d |
79.56 ± 2.65d |
87.25 ± 2.49d |
AA/GT10 |
16.26 ± 0.25c |
25.54 ± 0.03c |
35.57 ± 1.39a |
44.53 ± 1.38a |
54.82 ± 1.60a |
61.71 ± 2.98a |
67.78 ± 1.58b |
74.69 ± 2.87a |
AA/HPMC10 |
18.44 ± 0.35d |
30.33 ± 0.08d |
40.89 ± 0.87c |
53.76 ± 1.23c |
64.89 ± 1.49d |
76.74 ± 1.23d |
82.35 ± 2.90e |
89.94 ± 3.49c |
AA/GT12 |
15.76 ± 0.54b |
24.61 ± 0.06b |
35.47 ± 0.49a |
43.55 ± 1.98a |
53.57 ± 2.21a |
60.57 ± 1.54a |
65.36 ± 1.46b |
72.28 ± 1.93a |
AA/HPMC12 |
17.25 ± 0.34c |
31.68 ± 0.05d |
46.15 ± 1.59d |
61.53 ± 1.34e |
82.35 ± 2.38e |
85.86 ± 2.67e |
83.68 ± 2.97e |
92.34 ± 2.32e |
AA/GT14 |
14.68 ± 0.67a |
22.45 ± 0.11a |
34.98 ± 0.86a |
43.65 ± 1.45b |
53.34 ± 2.51a |
60.76 ± 1.75a |
63.74 ± 2.19a |
70.56 ± 1.98a |
AA/HPMC14 |
21.41 ± 0.71e |
37.93 ± 0.01e |
62.06 ± 0.53d |
79.31 ± 1.67e |
82.63 ± 1.59e |
86.45 ± 2.18e |
89.69 ± 2.86f |
95.12 ± 3.21e |
4. Conclusion
The results of this study showed that both GT and HPMC had a reinforcing effect on the mechanical properties of alginate–aloe vera composite films. It could be concluded that the two very important characteristics of food-packaging films, that is, tensile strength and flexibility, could be significantly improved by blending GT and HPMC into the alginate–aloe vera films. GT gave an approximately threefold increase in tensile strength, whereas the addition of HPMC to the composite films resulted in a twofold increase in tensile strength. However, the impact of these two reinforcing agents on the film solubility was opposite to one another; whereby HPMC addition resulted in increasing the solubility, whereas GT reduced it. The effect of HPMC in increasing the film solubility can be exploited in the development of edible food-packaging films, where better solubility is required for digestibility. The role of GT in decreasing the film solubility is important in the development of food-packaging films for ensuring a higher shelf life of the packaged food.
Data availability
Data sharing is not applicable as all the generated data has already been presented in the article.
Conflicts of interest
The authors declare no competing interests.
Acknowledgements
The authors did not receive any funding for this research work.
References
- A. Mansur, M. Rodrigues, N. Capanema, S. Carvalho, D. Gomes and H. J. R. a. Mansur, Functionalized bioadhesion-enhanced carboxymethyl cellulose/polyvinyl alcohol hybrid hydrogels for chronic wound dressing applications, RSC Adv., 2023, 13(19), 13156–13168 RSC
.
- G. Dalei, S. K. Das, S. S. Mohapatra and S. J. S. F. T. Das, In situ crosslinked Schiff-base biohydrogels containing Carica papaya peel extract: Application in packaging of fresh berries, Sustainable Food Technol., 2023, D3FB00096F Search PubMed
.
- S. Bhatia, A. Al-Harrasi, Y. A. Shah, H. W. K. Altoubi, S. Kotta, P. Sharma, M. K. Anwer, D. S. Kaithavalappil, E. Koca and L. Y. J. G. Aydemir, Fabrication, Characterization, and Antioxidant Potential of Sodium Alginate/Acacia Gum Hydrogel-Based Films Loaded with Cinnamon Essential Oil, Gels, 2023, 9(4), 337 CrossRef CAS PubMed
.
- A. I. Lopes, A. Melo, C. Caleja, E. Pereira, T. C. Finimundy, T. B. Afonso, S. Silva, M. Ivanov, M. Soković and F. K. J. C. Tavaria, Evaluation of Antimicrobial and Antioxidant Activities of Alginate Edible Coatings Incorporated with Plant Extracts, Coatings, 2023, 13(9), 1487 CrossRef CAS
.
- T. Fazal, B. N. Murtaza, M. Shah, S. Iqbal, M.-u. Rehman, F. Jaber, A. A. Dera, N. S. Awwad and H. A. J. R. a. Ibrahium, Recent developments in natural biopolymer based drug delivery systems, RSC Adv., 2023, 13(33), 23087–23121 RSC
.
- A. Mayrhofer, S. Kopacic and W. J. P. Bauer, Extensive Characterization of Alginate, Chitosan and Microfibrillated Cellulose Cast Films to Assess their Suitability as Barrier Coating for Paper and Board, Polymers, 2023, 15(16), 3336 CrossRef CAS PubMed
.
- H. C. Oyeoka, C. M. Ewulonu, I. C. Nwuzor, C. M. Obele and J. T. J. J. o. B. Nwabanne, Bioproducts, Packaging and degradability properties of polyvinyl alcohol/gelatin nanocomposite films filled water hyacinth cellulose nanocrystals, J. Bioresour. Bioprod., 2021, 6(2), 168–185 CrossRef CAS
.
- I. B. Basumatary, A. Mukherjee and S. J. I. J. o. B. M. Kumar, Chitosan-based composite films containing eugenol nanoemulsion, ZnO nanoparticles and Aloe vera gel for active food packaging, Int. J. Biol. Macromol., 2023, 242, 124826 CrossRef CAS PubMed
.
- A. Azadi, F. Rafieian, M. Sami and A. J. I. J. o. B. M. Rezaei, Fabrication, characterization and antimicrobial activity of chitosan/tragacanth gum/polyvinyl alcohol composite films incorporated with cinnamon essential oil nanoemulsion, Int. J. Biol. Macromol., 2023, 245, 125225 CrossRef CAS PubMed
.
- P. Foroughi, N. J. J. o. V. Koupaei and A. Technology, Physically crosslinked polyvinyl alcohol/chitosan/gum tragacanth hydrogels loaded with vitamin E for wound healing applications, J. Vinyl Addit. Technol., 2023, 29(2), 268–282 CrossRef CAS
.
- J. Wang, X. Han, C. Zhang, K. Liu and G. J. N. Duan, Source of nanocellulose and its application in nanocomposite packaging material: A review, Nanomaterials, 2022, 12(18), 3158 CrossRef CAS PubMed
.
- E.-S. Khater, A. Bahnasawy, B. A. Gabal, W. Abbas and O. J. S. R. Morsy, Effect of adding nano-materials on the properties of hydroxypropyl methylcellulose (HPMC) edible films, Sci. Rep., 2023, 13(1), 5063 CrossRef CAS PubMed
.
- A. Hadi, A. Nawab, F. Alam and K. Zehra, Physical, mechanical, optical, barrier, and antioxidant properties of sodium alginate–aloe vera biocomposite film, J. Food Process. Preserv., 2021, 45(5), e15444 CAS
.
- S. Gholamipoor, Y. Nikpour-Ghanavati, A. Oromiehie and M. Mohammadi, Extraction and Characterization of Alginate from Sargassum angustifolium collected from northern coasts of Persian Gulf, Bushehr, Int. Symp. Adv. Polym. Sci. Technol., 2013, 1–5 Search PubMed
.
- K. Norajit, K. M. Kim and G. H. Ryu, Comparative studies on the characterization and antioxidant properties of biodegradable alginate films containing ginseng extract, J. Food Eng., 2010, 98(3), 377–384 CrossRef CAS
.
- O. Sarheed, B. K. A. Rasool, E. Abu-Gharbieh and U. S. Aziz, An investigation and characterization on alginate hydogel dressing loaded with metronidazole prepared by combined inotropic gelation and freeze-thawing cycles for controlled release, AAPS PharmSciTech, 2015, 16(3), 601–609 CrossRef CAS PubMed
.
- A. Jangchud and M. Chinnan, Peanut protein film as affected by drying temperature and pH of film forming solution, J. Food Sci., 1999, 64(1), 153–157 CrossRef CAS
.
- E. Ayranci and S. J. F. C. Tunc, A method for the measurement of the oxygen permeability and the development of edible films to reduce the rate of oxidative reactions in fresh foods, Food Chem., 2003, 80(3), 423–431 CrossRef CAS
.
- A. Nawab, F. Alam, M. A. Haq, Z. Lutfi and A. Hasnain, Mango kernel starch-gum composite films: Physical, mechanical and barrier properties, Int. J. Biol. Macromol., 2017, 98, 869–876 CrossRef CAS PubMed
.
- J. F. Martucci and R. A. Ruseckaite, Biodegradation of three-layer laminate films based on gelatin under indoor soil conditions, Polym. Degrad. Stab., 2009, 94(8), 1307–1313 CrossRef CAS
.
- Q. Feng, F. Chen and H. Wu, Preparation and characterization of a temperature-sensitive lignin-based hydrogel, BioResources, 2011, 6(4), 4942–4952 CAS
.
- M.-J. Akhtar and M. Aïder, Study of the barrier and mechanical properties of packaging edible films fabricated with hydroxypropyl methylcellulose (HPMC) combined with electro-activated whey, J. Package Technol. Res., 2018, 2(3), 169–180 CrossRef
.
- B. Ozel, S. Cikrikci, O. Aydin and M. H. Oztop, Polysaccharide blended whey protein isolate-(WPI) hydrogels: A physicochemical and controlled release study, Food Hydrocolloids, 2017, 71, 35–46 CrossRef CAS
.
- R. Awasthi and G. T. Kulkarni, Development of novel gastroretentive drug delivery system of gliclazide: hollow beads, Drug Dev. Ind. Pharm., 2014, 40(3), 398–408 CrossRef CAS PubMed
.
- F. S. Mostafavi, R. Kadkhodaee, B. Emadzadeh and A. Koocheki, Preparation and characterization of tragacanth–locust bean gum edible blend films, Carbohydr. Polym., 2016, 139, 20–27 CrossRef CAS PubMed
.
- W. A. Laftah, S. Hashim and A. N. Ibrahim, Polymer hydrogels: A review, Polym.-Plast. Technol. Eng., 2011, 50(14), 1475–1486 CrossRef CAS
.
- H. Möller, S. Grelier, P. Pardon and V. Coma, Antimicrobial and physicochemical properties of chitosan−hpmc-based films, J. Agric. Food Chem., 2004, 52(21), 6585–6591 CrossRef PubMed
.
- M. Perez-Gago and J. J. J. o. f. s. Krochta, Denaturation time and temperature effects on solubility, tensile properties, and oxygen permeability of whey protein edible films, J. Food Sci., 2001, 66(5), 705–710 CrossRef CAS
.
- A. Jiménez, M. J. Fabra, P. Talens and A. J. C. P. Chiralt, Influence of hydroxypropylmethylcellulose addition and homogenization conditions on properties and ageing of corn starch based films, Carbohydr. Polym., 2012, 89(2), 676–686 CrossRef PubMed
.
- L. Atarés, R. Pérez-Masiá and A. J. J. o. f. e. Chiralt, The role of some antioxidants in the HPMC film properties and lipid protection in coated toasted almonds, J. Food Eng., 2011, 104(4), 649–656 CrossRef
.
- X. Liu, L. Lebrun, N. Follain and N. J. M. A. Desilles, Bio-based copolyesters involving 1, 4: 3, 6-dianhydrohexitols and sebacic acid: 2, 6-pyridinedicarboxylic acid as platforms for high gas barrier food packaging, Mater. Adv., 2022, 3(1), 389–398 RSC
.
- X. Liu, N. Desilles, B. Jiang, C. Chappey and L. J. P. Lebrun, High barrier semi-crystalline polyesters involving nature occurring pyridine structure towards sustainable food packaging, Polymer, 2022, 247, 124790 CrossRef CAS
.
- X. Liu, N. Desilles and L. J. E. P. J. Lebrun, Polyesters from renewable 1, 4: 3, 6-dianhydrohexitols for food packaging: Synthesis, thermal, mechanical and barrier properties, Eur. Polym. J., 2020, 134, 109846 CrossRef CAS
.
- S. Pirsa, F. Mohtarami and S. J. C. R. Kalantari, Preparation of biodegradable composite starch/tragacanth gum/nanoclay film and study of its physicochemical and mechanical properties, Chem. Rev. Lett., 2020, 3(3), 98–103 CAS
.
- C. Pastor, L. Sánchez-González, M. Cháfer, A. Chiralt and C. González-Martínez, Physical and antifungal properties of hydroxypropylmethylcellulose based films containing propolis as affected by moisture content, Carbohydr. Polym., 2010, 82(4), 1174–1183 CrossRef CAS
.
- J.-W. Rhim, H.-M. Park and C.-S. Ha, Bio-nanocomposites for food packaging applications, Prog. Polym. Sci., 2013, 38(10–11), 1629–1652 CrossRef CAS
.
- M. Anker, J. Berntsen, A.-M. Hermansson and M. Stading, Improved water vapor barrier of whey protein films by addition of an acetylated monoglyceride, Innovative Food Sci. Emerging Technol., 2002, 3(1), 81–92 CrossRef CAS
.
- S. Beikzadeh, A. Khezerlou, S. M. Jafari, Z. Pilevar and A. M. Mortazavian, Seed mucilages as the functional ingredients for biodegradable films and edible coatings in the food industry, Adv. Colloid Interface Sci., 2020, 280, 102164 CrossRef CAS PubMed
.
- C. Aulin, S. Ahola, P. Josefsson, T. Nishino, Y. Hirose, M. Osterberg and L. Wagberg, Nanoscale Cellulose Films with Different Crystallinities and Mesostructures Their Surface Properties and Interaction with Water, Langmuir, 2009, 25(13), 7675–7685 CrossRef CAS PubMed
.
- D. Jussen, S. Sharma, J. K. Carson and K. L. Pickering, Preparation and tensile properties of guar gum hydrogel films, Polym. Polym. Compos., 2020, 28(3), 180–186 CrossRef CAS
.
- C.-H. Chen, W.-S. Kuo and L.-S. Lai, Effect of surfactants on water barrier and physical properties of tapioca starch/decolorized hsian-tsao leaf gum films, Food Hydrocolloids, 2009, 23(3), 714–721 CrossRef CAS
.
|
This journal is © The Royal Society of Chemistry 2023 |
Click here to see how this site uses Cookies. View our privacy policy here.