Comparative study of different particle sizes of added olive leaves for the content of target polyphenols in virgin olive oil
Received
19th July 2023
, Accepted 12th September 2023
First published on 25th September 2023
Abstract
The addition of olive leaves during processing of olive oil has been studied and reviewed from different perspectives but there is a paucity of information on the roles of particle sizes of the added leaves in phenolic content of the oil. Dry ground olive leaves with a range of particle size fractions (0.07–3.0 mm) were added to crushed olives prior to the malaxation to compare their effects on: (i) the content of total and selected polyphenols (particularly oleuropein and verbascoside), (ii) antioxidant capacity (in vitro), and (iii) physicochemical quality and the yield of the extracted olive oil. Besides particle size, that was the main factor of study, olive pitting and malaxation time (30 and 60 min) were also considered as independent variables. Olive leaves with 0.3 mm followed by 0.15 mm favorably exhibited significant effects (p < 0.001) in all assays. Indeed, the oil samples with 0.3 mm leaves produced from the pitted olives showed maximum values when the malaxation time was (i) 30 min – for oleuropein (5.85 mg per kg oil), verbascoside (4.02 mg per kg oil), luteolin (15.44 and mg per kg oil), and total phenolic content (TPC) (368.01 mg per kg oil), and (ii) 60 min – for hydroxytyrosol (19.14 mg per kg oil) and tyrosol (16.89 mg per kg oil). These findings indicate that the particle size of added olive leaves can play a significant role in the content of principal polyphenols of the resulting olive oil; a topic that has not been approached in the literature.
Sustainability statement
The United Nations Sustainable Development Goals (SDG) – 2030 Agenda. From the perspective of the United Nation’s 2030 Agenda on Sustainable Development Goals, this research project will have contribution to improving sustainability in agri-food system. Among 17 SDG Goals stated by United Nations’, following Goals are applicable to the manuscript submitted: Goal 2 – “End hunger, achieve food security and improved nutrition and promote sustainable agriculture”; Goal 7—“Ensure Access to Affordable, Reliable, Sustainable and Modern Energy for All”; Goal 12 – “Ensure sustainable consumption and production patterns. Sustainable consumption and production”. The ideas of this research well correspond with the abovementioned Goals. The findings of our study revealed that dry ground olive leaves (residual biomass) can be affordably re-used and valorized directly to enrich olive oil phenolic properties (when appropriate particle size reduction of the leaves is taken into consideration). The outcome of this study is of value to layout a basis for future/relevant studies that can partly contribute to addressing undernutrition, food insecurity, agricultural distress, environmental degradation, and high production expenditure. Although the agro-industrial system is striving responsively to accommodate the global nutritional needs, more efforts need to be accomplished to formulate resilient and sustainable production approaches to effectively satisfy: (i) population dietary needs – through developing nutritive density/accessibility, health, and affordability, and (ii) sustainable production and consumption, low-energy intensive/cost, and zero-waste agri-food system.
|
Introduction
Fresh olive fruit, from which virgin olive oil (VOO) is mechanically produced, contains a large proportion of phenolic antioxidants that are decidedly responsible for the nutritional quality and stability of its extracted oil. The question arises whether these valuable compounds are proportionally recovered and preserved in the oil following the extraction process. Research studies have demonstrated that this is not the case as polyphenols are prone to degradation/oxidation especially during malaxation of olive paste as well as during the storage of oil. In particular, certain types of phenols including oleuropein (a secoiridoid glycoside) and verbascoside (a phenylethanoid glycoside), the highly prized antioxidants, may suffer from biochemical degradations due to enzymatic and chemical reactions throughout the processing of the oil. As a result, their concentrations may decline significantly, and hence only trace quantities may be found in the resulting oil. In the literature, numerous processing techniques have been discussed to intensify the transfer of polyphenols to the extracted olive oil. These include the addition of olive leaves during processing to compensate phenolic loss and support the stability of olive oil. Indeed, a large volume of research, dealing with valorization of olive leaves in olive oil processing, exhaustively investigated a broad range of factors including (i) mechanical processing parameters of oil extraction such as malaxation time/temperature, pitting (de-stoning of olives), types of crushers, etc., (ii) fruit ripeness, (iii) dose-dependency of added leaves, and (iv) cultivars/growing regions. Having said that, there is no clear evidence on the efficacy of particle size of added leaves, while a substantial body of evidence reports that size reduction is among the key mechanical factors responsible for the physicochemical properties (associated with enhanced surface area and diffusivity/mass transfer) and extractability of the desired phytonutrients.1,2 It is therefore important to take the particle size of olive leaves into consideration when addressing sustainable exploitation of these biomass residues, especially when selective extraction of bio-molecules is of interest. Given this, the present study aimed at investigating the effect of particle size of dry ground olive leaves on olive oil quality when added to the crushed olives in advance of malaxation process. The specific objectives were as follows: (i) primarily – to evaluate the influence of different particle sizes of dry leaves on principal polyphenols and antioxidant capacity of the leaf-added virgin olive oil. Special attention was placed on the content of oleuropein and verbascoside, and (ii) secondarily – to validate the assumption of favorable effects of shorter malaxation time (30 min) and pitting of olive paste (particularly before malaxation) on the increment of oleuropein and verbascoside in the oil enriched with optimal particle size of olive leaves.
Materials and methods
Plant materials and chemicals
Olive leaves (the residues from olive oil processing) and olive fruits of Picual cultivar were kindly supplied by “Center for Advanced Studies in Energy and Environment”, University of Jaén, Campus of Las Lagunillas, Jaén, Spain. Upon arrival to the University of Minho, Portugal, they were prepared as follows: (i) the leaves were manually cleaned (to remove stems and foreign objects), washed, and dried at 37 °C for 48 h. The dried leaves were then ground and passed through a range of sieves (200–7 mesh) to separate them into five particle fractions (0.07, 0.15, 0.3, 1.6, and 3.0 mm). Each fraction was vacuum packed in polypropylene bags, refrigerated (4 ± 2 °C) for further processing within two weeks. (ii) Olive fruits, were manually cleaned to remove foreign objects/bruised olives, laid flat on food-grade/dry trays, refrigerated, and processed for oil extraction within two weeks.
The following reagents were purchased from Sigma-Aldrich (Saint Louis, MO, USA): (i) analytical grade – Folin–Ciocalteu, anhydrous gallic acid (≥98.0%), anhydrous sodium carbonate (≥99%), hydrochloric acid, 2,4,6-tris(2-pyridyl)-s-triazine (TPTZ), ferric chloride (FeCl3), ethanol (99.8%), 2,2-diphenyl-1-picrylhydrazyl (DPPH), 2,20-azino-bis-3-ethylbenzothiazoline-6-sulfonic acid (ABTS), and (±)-6-hydroxy-2,5,7,8-tetramethylchromane-2-carboxylic acid (trolox), acetic acid (99.8%), chloroform (99%), potassium iodide (≥99.0%), hexane (≥99%), and methanol (99%), (ii) HPLC grade – phenolic standards (oleuropein, verbascoside, luteolin, hydroxytyrosol, apigenin, and tyrosol with 98% w/w purity), formic acid, and acetonitrile (≥99.9%).
Study design
In this study, a range of particle size fractions of dry ground olive leaves were added to pitted olives during crushing, to compare their effects on the quality of the extracted olive oil. The response variables (dependent variables) consisted of: (i) total phenolic content (TPC), (ii) principal polyphenols (oleuropein, verbascoside, hydroxytyrosol, tyrosol, luteolin, and apigenin), (iii) antioxidant capacity (in vitro), (iv) physicochemical quality comprising free fatty acids (FFA), peroxide value, pigments (carotenoids and chlorophylls), and the yield (%) of the resulting olive oil.
The key independent variable (factor) was the particle size of the added olive leaves. Additionally, in order to extract further data, the following auxiliary factors were investigated: (i) malaxation time (30 and 60 min), and (ii) pitting/un-pitting of olive paste in advance of malaxation to assess their interactive effects on the response variables. The quality of leaf-added oil samples was compared to that of control samples (oils processed without the addition of olive leaves). The selected cultivar, growing region, and handling/storage conditions were the same for all experiments.
Mechanical processing – extraction of olive oil
The mechanical operations employed for the extraction of virgin olive oil (Fig. 1) consisted of: (i) crushing–olives (100 g) were initially crushed using a blender equipped with a rotation speed/temperature adjustment (Vorwerk Bimby® TM6, Germany) at 1500 rpm for 2 min. The blender was stopped and the stones were removed (using a pair of stainless steel forceps tweezers). Thereafter, dry olive leaves (3%) was added (separately for each fraction) to the crushed olives and further blended at 2000 rpm for 4 min (the machine stopped in between and stirred manually every 1 min interval). Final crushing (pressing) was then applied for 4 min using a ceramic mortar and pestle, to confirm the release of oil from the cell tissues driven by the pressure exerted on the food matrix. (ii) Malaxation–the crushed olive paste was then mixed under slow spinning for various times (30 and 60 min for each experiment), with the same blending appliance, to coalesce the oil droplets through which the extraction of free oil is enabled. (iii) two-phase separation (centrifugation decanting)–the mixed olive paste was centrifuged at 3500 rpm for 5 min in Thermo-IEC polypropylene centrifuge bottles at 27 °C. The oil fraction was separated, weighed, bottled, nitrogen flushed, capped, and stored in dark, cool/ventilated place until experimental analysis.
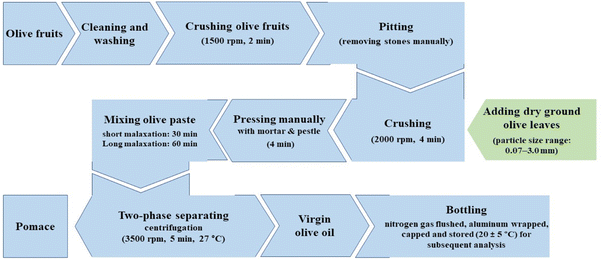 |
| Fig. 1 Summary of the mechanical processing technique used in this study for the extraction of virgin olive oil. | |
The same procedure was repeated for unpitted samples (stones remained in the paste) for each malaxation time separately. Likewise, the control samples (free of olive leaves) were obtained accordingly for both pitted and unpitted experiments. Temperature remained constant at 27 °C throughout the extraction. The choice of adding dry leaves rather than fresh ones was based on the extensive studies reviewed previously.2,3
Analysis of lipid matrix (olive oil samples prior to phenolic extraction)
Olive oil samples with/without olive leaves were initially analyzed for physicochemical quality parameters as follows:
Peroxide value and free acidity.
The quality of olive oil samples in respect of peroxide value and free fatty acids was measured according to the official method of American Oil Chemists Society (AOCS) with minor modifications.4
Peroxide value.
The peroxide value of oil that represents the degree of oxidative rancidity was assessed through iodometric titration. Briefly, one gram of oil was dissolved with 10 mL acetic acid: chloroform (3
:
2 v/v), gently shaken before adding 2 mL saturated potassium iodide. The mixture was incubated in the dark for 5 min to enable the release of iodine from saturated potassium iodide. After adding 10 mL distilled water (shaken for 1 min) the mixture was then titrated with 0.01 N sodium thiosulphate standard solution (the initial burette reading was recorded). One mL starch solution (1.5%) was used as indicator. The titration was continued until the color changed from dark to pale/white. The final burette reading was recorded. The peroxide value was calculated according to eqn (1). The results were expressed as milliequivalents of active oxygen per kilogram oil (mEq. O2/kg). |  | (1) |
where: Ws = weight of sample (g), V = volume of sodium thiosulphate (mL) that is: (final burette reading – initial burette reading), N = normality of sodium thiosulphate (0.01 N).
Free fatty acids (FFA).
The percentage of free fatty acids was determined by titration where the oil samples were dissolved with ethanol/ether (1
:
1, v/v) and 0.1 M sodium hydroxide. Phenolphthalein was used as an indicator. The results were expressed as percentage of oleic acids (g/100 g w/w).
Extraction yield of olive oil.
The yield percentage (%) of the extracted oil was calculated according to eqn (2): | 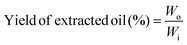 | (2) |
where: Wo = weight of extracted oil, Wi = initial weight of olive fruits (g)
Pigment determination.
The content of chlorophylls and carotenoids was determined spectrophotometrically5 by dissolving 7.5 g of oil with 25 mL of cyclohexane. The absorbance readings were measured at 670 nm (for chlorophyll) and 470 nm (for carotenoid), and the results were expressed as mg per kg oil, respectively.
Analysis of phenolic extracts of olive oil samples
In this section, the polar fraction of olive oil was extracted and assessed for the content of total and individual polyphenols, as well as antioxidant capacity.
Sample preparation – isolation of phenolic extracts.
The fraction of polyphenols from olive oil was separated through a liquid–liquid extraction method using the method described by Lozano-Castellón et al.6 with minor modifications. Briefly, 2 g of oil was mixed with n-hexane (2 mL) and methanol/water (4 mL), vortex mixed (1 min) and centrifuged (3000 rpm for 5 min). The polar phase was taken and the hexane phase (lipophilic fraction) was re-extracted thrice to obtain an oil-free solution. The extracted polar phase was then subjected to a rotary evaporator, dissolved in HPLC-grade methanol/water (50
:
50, v/v),nitrogen flushed (using Reacti-therm™ Heating Module, Pierce), filtered through a 0.22 μm polytetrafluoroethylene membrane and stored at −20 °C before analysis.
HPLC determination of selected phenolic compounds.
The chromatographic separations of target polyphenols were carried out on a reverse-phase Aquity UPLC BEH C18 column (100 mm × 2.1 mm i.d., 1.7 μm particle size, Waters Corporation) using ultra-high-pressure liquid chromatography (UHPLC) (Shimadzu Nexera X2 UHPLC) connected to a diode array detector (Shimadzu SPD-M20A), and an integration system (Shimadzu LabSolutions software, Kyoto, Japan). The gradient elution program (Table 1) was based on the method described by Quero et al.7 with slight modifications. The solvent flow rate and the injection volume were 0.3 mL min−1, and 5 μL, respectively. The column temperature was maintained at 40 °C. Prior to the analysis, the eluents (mobile phase) were initially filtered through 0.22 μm membrane nylon filters, and the samples/standards were filtered through 0.22 μm syringe filters.
Table 1 Gradient elution program for HPLC analysis used in this study
Time period (min) |
Flow rate (mL min−1) |
Mobile phase A (%) water/formic acid (99.9/0.1 v/v) |
Mobile phase B (%) acetonitrile |
0–5.5 |
0.3 |
95 |
5 |
5.5–17 |
0.3 |
40 |
60 |
17–18.5 |
0.3 |
0 |
100 |
18.5–30 |
0.3 |
95 |
5 |
The chromatograms were registered at 280 nm and the target phenols of the samples were identified by referring to the retention times of the commercial standards and the UV spectra detector. The standard calibration curve was plotted (peak area vs. the known concentration) and the selected phenolic content was quantified against the linear calibration curve of the corresponding standard. The results were reported as mg target phenol per kg oil.
Antioxidant activity (in vitro).
The reactivity of free radicals, due to their unpaired-electron structure, can bring about lipid oxidation in edible oils which is a major issue as it can render the oil nutritionally and organoleptically degraded. In this regard. antiradical activity of olive oil can be an ideal marker to determine the potential of the endogenous antioxidants for reducing free radicals. In this study, phenolic extracts of oil samples were examined by Trolox Equivalent Antioxidant Capacity (TEAC) assay through the following in vitro methods:
DPPH radical scavenging activity.
The ability of phenolic extracts of oil samples to diminish activities of free radical 2,2-diphenyl-1-picrylhydrazyl (DPPH˙) was assessed using the method of Brand-Williams et al.8 with slight modifications as described in our previous study.9 This stable radical (a dark purple color) can be reduced/neutralized by the reaction of antioxidants usually through their electron transfer.8 The data from the decrease in absorbance (515 nm) were calculated against Trolox standard curve and the results were expressed as mM Trolox equivalents/kg oil (mM TE per kg).
FRAP radical scavenging activity.
The assay of ferric reducing antioxidant power (FRAP) was performed following the method of Benzie & Strain10 with slight modification. It determines the antioxidant potential of samples for reducing ferric ion (Fe3+) to ferrous ion (Fe2+). The FRAP reagent was initially prepared by mixing: (i) acetate buffer, and (ii) 2,4,6-tris(2-pyridyl)-s-triazine (TPTZ) in hydrochloric acid and FeCl3·6H2O (10
:
1
:
1 v/v/v). The mixture was incubated (37 °C, 10 min). Into a test tube containing FRAP reagent, an aliquot of sample was added, vortex mixed and incubated (37 °C, 30 min). A blank containing only FRAP reagent was also prepared. The absorbance readings were measured at 593 nm and the values were calculated against Trolox standard curve and the results were reported as mM TE per kg oil.
ABTS radical scavenging activity.
The ABTS˙+ radical scavenging assay was carried out according to the method of Re et al.11 with slight modifications as described in our previous study.9 The principle behind this assay: (i) initial generation of 2,2′-azinobis-(3-ethylbenzothiazoline-6-sulfonic acid) ABTS˙+ through the reaction of ABTS and potassium persulfate (in absence of antioxidants), and (ii) subsequent decolorization and reduction of ABTS˙+ after adding antioxidants, changing from bluish green to colorless. Following this assay, the data from reduction of absorbance at 734 nm were calculated against Trolox calibration curve and expressed as mM TE per kg oil.
Total phenolic content (TPC).
Total phenolic content of olive oil was determined using Folin–Ciocalteau assay as described by Singleton & Rossi12 with slight modifications. Into 1 mL phenolic extract of oil, the following reagents were added: 1 mL of 20% sodium carbonate (freshly prepared) and 1 mL of Folin–Ciocalteau reagent. The solution was gently mixed and incubated in the dark for 30 min to develop a blue color prior to centrifugation (2500 rpm for 1 min). The blue color fraction was collected for absorbance measurement at 765 nm and the total phenolic content was calculated against gallic acid standard curve. The results were reported as mg of gallic acid equivalents/kg oil (mg GAE per kg).
Statistical analysis
The significant differences (p < 0.05) between the mean values (±SD) of all determinations were statistically assessed via Analysis of Variance (ANOVA) using SPSS software, version 27.0. The effect of independent variables on each dependent variable was analyzed through factorial ANOVA (two-way ANOVA). The interactions between independent variables: (i) particle size vs. malaxation time, and (ii) particle size vs. pitting/un-pitting, were assessed using pairwise comparisons. The assumption of homogeneity of equal variance was assessed through the Levene's test (homogeneity of variance assumption was not violated when p-value was greater than 0.05). Each experiment was carried out in triplicates.
Results and discussion
Effect of particle size of added leaves on physicochemical quality and yield of extracted oils
Peroxide value (PV).
The measurement of peroxide value in olive oil is vital as it reflects the status of oxidative rancidity (at the primary stage of oxidation) in olive oil. The lower values of PV thus represents higher stability and longer shelf-life. As shown in Fig. 2, peroxide values ranged from 5.82 to 9.36 mEq. O2 per kg oil. According to the quality criteria designated for trade standards, the peroxide value of extra virgin olive oil (EVOO) must not exceed 20.0 mEq. kg−1.13,14 In this respect, the results here meet the standard criteria suggesting that they did not undergo the primary oxidation during and after oil processing. In all malaxation groups, the addition of 0.3 mm leaves showed significantly the most effectiveness in lowering peroxide values (p < 0.05).
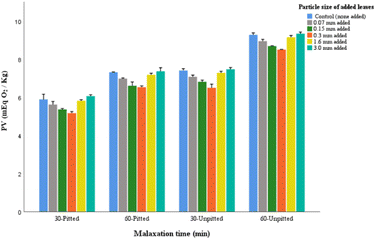 |
| Fig. 2 Effect of particle size of added olive leaves on peroxide value (PV) (mEq. O2 per kg) of the extracted olive oils from pitted crushed olives (30-pitted and 60-pitted, mixing for 30 and 60 min, respectively), and unpitted olives (30-unpitted and 60-unpitted, mixing for 30 and 60 min, respectively). | |
In all processing groups, with the exception of 0.3 mm and 0.15 mm leaves, the addition of leaves did not greatly change the peroxide values compared to the control samples. Examining the interactive effects of the auxiliary factors (pitting and malaxation time), it was found that the pitted groups represented significantly lower peroxides compared to the unpitted corresponding samples. Likewise, shorter malaxation in both pitted and unpitted groups represented significantly lower PVs. This can be explained by the fact that olive stones are a source of peroxidase enzymes. If the stones remain in the paste, particularly during longer malaxation, their endogenous enzymes are activated causing oxidation and degradation of bioactive compounds including polyphenols. Thus, pitting and short malaxation may both contribute to complementing the efficacy of the preferred particle size of added leaves (0.3 mm) in reducing PV in the extracted oil.
In previous studies, there is no clear consensus on the ideal addition of olive leaves that may assist in the reduction of peroxides. Considering the factor of dosage of added olive leaves, some studies observed that the addition of 2% and 3% leaves exerted effect on the reduction of peroxides,15,16 while others found 3% and 1–10% addition of leaves raised the numbers in the resulting extracted oil.17,18
Free fatty acids (FFA).
Free fatty acids (also known as free acidity) in olive oil refers to the broken-off fatty acids within the fat molecules. It is commonly measured as a marker of hydrolytic rancidity that may arise from the reaction of triglycerides with oxygen and water.
There was no significant interaction between malaxation time and particle size of leaves, suggesting that the acidity level for corresponding oil samples was not reliant on mixing time (Fig. 3). However, there was significant interaction between pitting factor and particle size as it was found that the free acidity of each pitted sample (produced from the same malaxation time) was significantly lower than the corresponding sample from unpitted paste.
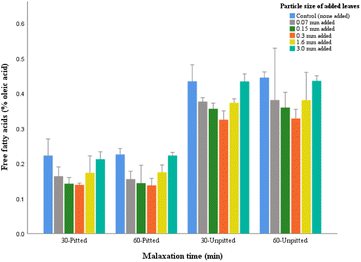 |
| Fig. 3 Effect of particle size of added olive leaves on free fatty acids (% oleic acid) of the extracted olive oils from pitted crushed olives (30-pitted and 60-pitted mixed for 30 and 60 min, respectively), and unpitted olives (30-unpitted and 60-unpitted mixed for 30 and 60 min, respectively). | |
In all groups of experiments, the acidity values did not exceed the limited criteria (0.8%, grams of oleic acid/100 g oil) approved by International Olive Council (IOC),13 hence, in this respect, can be acceptable as extra virgin olive oil. Among all groups, the oil with 0.3 mm leaves obtained from pitted olives (30 min) represented the lowest values (0.14%).
Yield of extracted olive oil (%).
The yield percentage of the extracted olive oils were measured to determine the extent of the effect of leaf particle size and its interaction with other two factors (pitting and malaxation time) on the extractability of the oil. As seen in Fig. 4, there were no significant differences between malaxation times for corresponding samples, although the extended malaxation (60 min) enabled slightly higher amount of oil. Indeed, malaxation may solely assist in easier separation of oil phase from solid phase (during centrifugation) and greater extractability of oil through the following phenomena: (i) coalescence of small oil droplets, (ii) destabilization of oil/water emulsion (as occurs during crushing operation), and (iii) flocculation of larger oil drops to the surface of the olive paste.
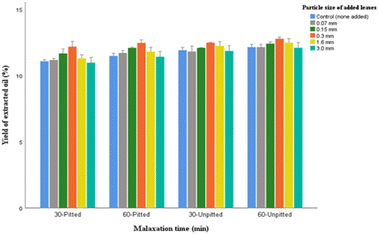 |
| Fig. 4 Effect of particle size of added olive leaves on the yield (%) of the extracted olive oils from pitted crushed olives (30-pitted and 60-pitted mixed for 30 and 60 min, respectively), and unpitted olives (30-unpitted and 60-unpitted mixed for 30 and 60 min, respectively). | |
The highest yield percentage was found in oils with 0.3 mm leaves processed from unpitted olives (13.19%) that was relatively higher than that obtained from the pitted olives (12.13%). However, regardless of the fact that the unpitted samples yielded slightly greater portions of oils compared to the pitted ones, malaxation in the presence of stones has been viewed as an issue due to the potential incidence of phenolic degradation induced by the peroxidase enzymes present in stones. In addition, as described thus far (Fig. 2) the peroxide values in unpitted samples were significantly higher than oils obtained from the pitted ones. Therefore, the marginal increase in the oil extractability may not give grounds for the need to leave the stones in the paste during malaxation.
Pigments.
The combined data from chlorophylls and carotenoids (Fig. 5) show that the addition of olive leaves significantly increased the content of both pigments in all groups of malaxations and it was evident that the pitted samples contained significantly more pigments compared to unpitted corresponding samples. Moreover, for all malaxation trials, the effect of particle sizes for each pigment was correspondingly comparable. However, the maximum levels belonged to the pitted samples with 0.3 mm leaves (60 min malaxation) that reached up to 16.71 mg kg−1 (for chlorophyll) and 8.74 mg kg−1 (for carotenoid).
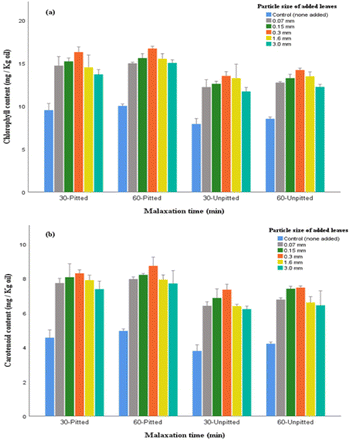 |
| Fig. 5 Effect of particle size of added olive leaves on chlorophylls (a), and carotenoids (b) of the extracted olive oils (mg per kg oil) from pitted crushed olives (30-pitted and 60-pitted mixed for 30 and 60 min respectively), and unpitted olives (30-unpitted and 60-unpitted mixed for 30 and 60 min, respectively). | |
Chlorophyll and carotenoid both account for a large proportion of pigments in olive leaves. Owing to their characteristic bio-functionalities, in terms of antioxidant effects (providing that products are not exposed to light) and natural color enhancements, they are considered as value-addition ingredients in the food system such as in olive oil products.19 The obtained results here, regardless of the factor of particle size, concur with previous research that observed significant effects of adding olive leaves on the increment of aforementioned pigments in the final extracted oil.20
Examining the durations of malaxations (in both pitted and unpitted), slight increase in pigments were found in oils obtained within longer malaxation. This relative changes may not justify the need for extending malaxation time, particularly when it was found that the shorter malaxation (30 min) surpassed the prolonged process in terms of peroxide value and acidity (Fig. 2 and 3). Hence, the use of 0.3 mm leaf addition through a 30-pitted processing system can be considered suitable for pigment enhancement in olive oil.
Effect of particle size of added leaves on the content of total polyphenols and antioxidant capacity of oils
Total phenolic content (TPC).
In general, regardless of the factor of particle size, previous studies justified the favorable effects of adding leaves on TPC enhancements17,20–22, though there is no consensus on their findings due to variations in percentages of added leaves,23–26 processing designs,27 harvesting times,20 along with others.
The data from this section confirm the assumption that pitting, as a preferred method, can assist in the rise of TPC, as compared to the corresponding oils produced from unpitted olives. The TPC variations in all corresponding samples showed the same pattern across different malaxations (Fig. 6). It was evident that the addition of 0.3 mm leaves exhibited the maximum values (the 30-pitted oil sample contained 368.01 mg kg−1). The ranking of other fractions for TPC determined to be in descending order as follows: 0.15 mm > 0.07 mm > 1.6 mm > control > 3.0 mm. The mean differences between oils with 3.0 mm leaves and control (without leaves) were not significant, p > 0.05.
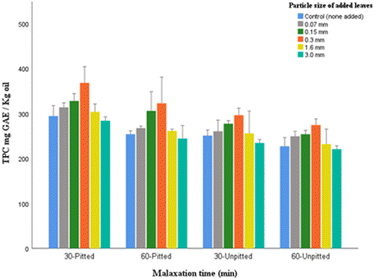 |
| Fig. 6 Effect of particle size of added olive leaves on total phenolic content (TPC) of the extracted olive oils (mg GAE per kg oil) from pitted crushed olives (30-pitted and 60-pitted mixed for 30 and 60 min respectively), and unpitted olives (30-unpitted and 60-unpitted mixed for 30 and 60 min, respectively). | |
Results also validated the hypothesis that longer malaxation is in part responsible for a significant drop in polyphenols. This can be due to the oxidation/catalyzing reactions of enzymes such as polyphenol oxidase (PPO). Therefore, the short term malaxation (30 min) is preferred in this respect.
Likewise, pitting was found to be of a better choice as the TPC values were significantly higher in each pitted sample compared to the corresponding unpitted sample with the same malaxation time p < 0.05. It should also be pointed out that the favorable results of polyphenolic content in this study may be attributed to the time point of leaf addition, that was during the crushing step prior to malaxation which potentially assisted in ideal mixing of leaves with crushed olives, and hence eliminated the necessity of the use of prolonged malaxation.
Trolox equivalent antioxidant capacity (TEAC).
Determination of antioxidant potential of oils with different size fractions of added leaves can be of value to ascertain whether the particle size of leaves has a significant role in this respect. The results here demonstrate that this is the case. In exception of 3.0 mm leaves, the inclusion of different fractions exhibited significant variations (in descending order of antiradical exertion: 0.3 mm, 0.15 mm, 0.07 mm, and 1.6 mm leaves). Relatively the same trends of variations were observed in all three types of TEAC assays (DPPH, FRAP, and ABTS) and the maximum levels belonged to 30-pitted oils with 0.3 mm leaves (1.51, 2.13, and 0.90 mM TE per kg oil detected by DPPH, FRAP, and ABTS, respectively).
The in vitro antioxidant assays partly reflect the presence and bio-functionality of polyphenols due to their strong antioxidant potential to suppress reactivities of free radicals (the reactive oxygen species). In other words, the antioxidant activity potentially relies on the quantities of potent polyphenols. This was evident in our study as both TPC values and, correspondingly, antioxidant activities showed relatively similar trends of changes for each malaxation condition (Fig. 7).
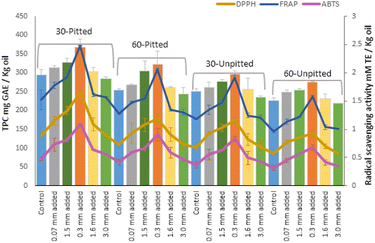 |
| Fig. 7 Comparison of antiradical activities (detected by DPPH, FRAP, and ABTS) (mM TE per kg), and their relations with total phenolic content (mg GAE per kg) of the extracted olive oils from pitted crushed olives (30-pitted and 60-pitted) and unpitted olives (30-unpitted and 60-unpitted) mixed for 30 and 60 min respectively. | |
Polyphenols indeed constitute a large proportion of phytonutrients in plant tissues. Their quantities, thus, play a potential role in minimizing activities of free radicals and maximizing oxidative stability of olive oil. In this regard, the positive relations between total polyphenols and antioxidant capacity has been well justified in the literature.27–34
Effect of particle size of olive leaves on the recovery of target polyphenolic compounds
Quantitative analysis of the selected polyphenols (from polar fraction of olive oil samples) was examined by HPLC. The data were analyzed to explore the potential role of particle size of added leaves in phenolic concentrations of the oil samples.
Oleuropein.
As shown in Fig. 8, the levels of oleuropein in oil samples with 0.3 mm leaves were significantly higher, particularly in 30-pitted samples (5.85 mg per kg oil). The control groups (oils without leaves) contained traces of oleuropein (0.29–0.89 mg kg−1). This may be explained by that fact that oleuropein, that is abundantly found in the unprocessed olive fruits, is likely to suffer from activities of the endogenous enzymes when subjected to handling and processing. This phenomenon is more likely to occur during longer malaxation wherein the catalyzing active enzymes (particularly PPO) have the opportunity to exert degrading effects. On the other hand, during shorter malaxation, the catalyzing actions of such enzymes can be suppressed by a large numbers of intact/potent polyphenols, including oleuropein.
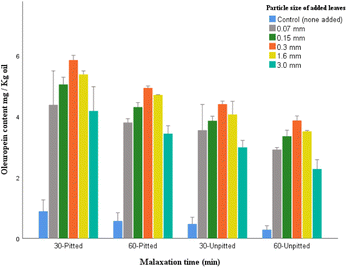 |
| Fig. 8 Effect of particle size of added olive leaves on oleuropein content of the extracted olive oils from pitted crushed olives (30-pitted and 60-pitted mixed for 30 and 60 min respectively), and unpitted olives (30-unpitted and 60-unpitted mixed for 30 and 60 min, respectively). | |
Verbascoside.
As shown in Fig. 9, the variations of verbascoside content across the oil samples followed the same pattern as those observed for oleuropein. The highest values (4.02 and 3.34 mg kg−1) belonged to the oils of pitted olives with 0.3 mm leaves processed for 30 min and 60 min, respectively. Examining the pitting factor, results showed that the addition of olive leaves enabled significantly higher yield in pitted samples (with leaves) compared to the corresponding ones in unpitted groups. This is in agreement with the research conducted previously.35
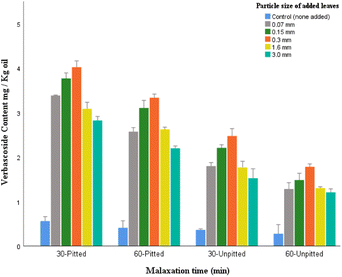 |
| Fig. 9 Effect of particle size of added olive leaves on verbascoside content of the extracted olive oils from pitted crushed olives (30-pitted and 60-pitted mixed for 30 and 60 min respectively), and unpitted olives (30-unpitted and 60-unpitted mixed for 30 and 60 min, respectively). | |
Verbascoside, is characterized by a heterosidic ester of hydroxytyrosol and caffeic acid which makes it a distinctively valuable antioxidant due to its double di-phenolic structure (containing two phenolic hydroxyl groups; caffeic acid and hydroxytyrosol). While being largely available in olive fruits, verbascoside is bound to be degraded or enzymatically hydrolyzed following the ripeness of fruits, storage, and processing, remaining in trace levels in olive oil. The results here confirm this postulation as the control samples contained minute amounts (0.28–0.56 mg per kg oil).
Hydroxytyrosol.
As shown in Fig. 10, unlike the pattern of changes observed for oleuropein and verbascoside, the longer malaxation represented more of hydroxytyrosol in both pitted and unpitted samples. Within 60 min and 30 min malaxations respectively, the oil samples with 0.3 mm leaves contained 19.14 mg kg−1 and 18.05 mg kg−1 (in pitted samples) and 17.82 and 17.51 mg kg−1 (in unpitted samples). This may explain the partial hydrolysis of oleuropein (often by glycosidase and esterase enzymes) through extended malaxation, giving rise to the breakage of the ester bond and the liberation of hydroxytyrosol.
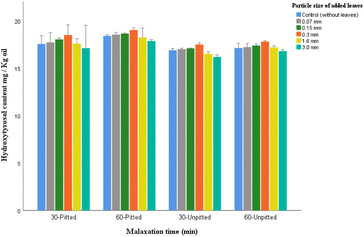 |
| Fig. 10 Effect of particle size of added olive leaves on hydroxytyrosol content of the extracted olive oils from pitted crushed olives (30-pitted and 60-pitted mixed for 30 and 60 min respectively), and unpitted olives (30-unpitted and 60-unpitted mixed for 30 and 60 min, respectively). | |
Moreover, compared to oleuropein and verbascoside, the content of hydroxytyrosol was much less affected by the enzymes of the olive stones present during malaxation, although the oils from pitted samples (for both malaxation times) yielded significantly greater amounts of hydroxytyrosol than those from unpitted ones.
Tyrosol.
The obtained data from tyrosol (Fig. 11) showed a similar pattern, in respect of malaxation time, to that observed for hydroxytyrosol as the extended malaxation comparably enabled a rise in tyrosol content. The highest values belonged to the oils with 0.3 mm leaves (16.89 and 16.53 mg kg−1 from pitted paste), and (16.12 and 15.83 mg kg−1 from unpitted paste) for 60 min and 30 min, respectively.
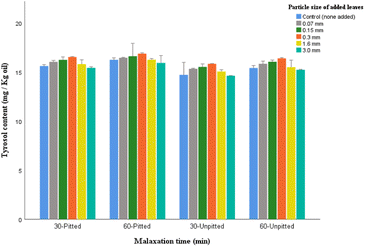 |
| Fig. 11 Effect of particle size of added olive leaves on tyrosol content of the extracted olive oils from pitted crushed olives (30-pitted and 60-pitted mixed for 30 and 60 min respectively), and unpitted olives (30-unpitted and 60-unpitted mixed for 30 and 60 min, respectively). | |
Compared to the majority of phenolic groups, tyrosol while having weaker antioxidant potential, is able to remain more stable, or become less affected by prolonged malaxation, hence a large numbers of tyrosol may readily be transferred from olive fruits to the resulting olive oil. This was the case in the present study as the control groups represented comparably a sizable proportion of tyrosol (16.24 mg kg−1 in 60-pitted, and 15.61 mg kg−1 in 30-pitted).
Luteolin.
Irrespective of the factor of particle size, olive leaves when appropriately added during the extraction of olive oil, reportedly assist in intensification of flavonoid phenols including flavone luteolin in olive oil (in free or glycosylated form).23,36,37 Examining the effect of particle size, it was evident (Fig. 12) that the oils with 0.3 mm leaves yielded maximum content of luteolin in all malaxation experiments (15.44 mg kg−1 and 14.92 mg kg−1 in 30 min pitted and 60 min unpitted, respectively).
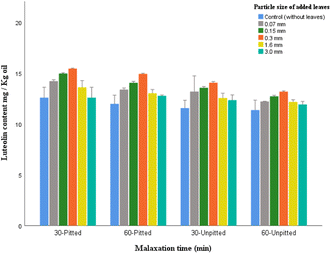 |
| Fig. 12 Effect of particle size of added olive leaves on luteolin content of the extracted olive oils from pitted crushed olives (30-pitted and 60-pitted mixed for 30 and 60 min respectively), and unpitted olives (30-unpitted and 60-unpitted mixed for 30 and 60 min, respectively). | |
Among the leaf-added oil samples, those with large particles (3.0 mm), showed the lowest levels in all experiments (11.35–12.59 mg per kg oil). Also, insignificant variabilities were found between the control samples and the oils with 0.07 mm and 1.6 mm leaves (p > 0.05).
The luteolin proportions of non-enriched virgin olive oil tend to differ largely in previous studies, according to variations in cultivars, growing regions, and operating system. Fanali et al.38 examined different growing sites in Italy and reported: 12.34 mg kg−1 (for Sicily), 14.25 mg kg−1 (for Lazio), 13.82 mg kg−1 (for Tuscany), and 14.12 mg kg−1 (for Puglia). López-Yerena et al.,39 through their investigation on the oil extraction methods, suggested that the use of lower crushing temperature (10 °C) and lower malaxation time (30 min) provide better conditions for the content of flavone constituents such as luteolin in olive oil.
Apigenin.
The highest value of apigenin (3.84 mg kg−1) belonged to 0.3 mm leaf-added oils from pitted paste (30 min).
As illustrated in Fig. 13, the levels of apigenin were significantly higher in pitted samples compared to the corresponding oils in unpitted for the same malaxation time. Comparing the malaxation times, particularly in pitted groups, the shorter duration was more effective in the recovery of flavone apigenin. The particle size of 0.07 and 1.6 mm did not show significant effect on the content of apigenin for each malaxation group (p > 0.05).
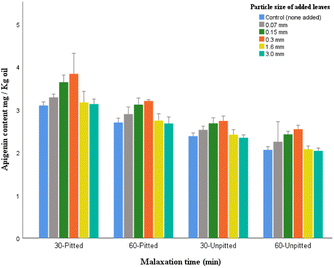 |
| Fig. 13 Effect of particle size of added olive leaves on apigenin content of the extracted olive oils from pitted crushed olives (30-pitted and 60-pitted mixed for 30 and 60 min respectively), and unpitted olives (30-unpitted and 60-unpitted mixed for 30 and 60 min, respectively). | |
The data on apigenin content in virgin olive oil differs largely among studies based on the experimental conditions. A recent study reported that the addition of olive leaves of different proportions (0%, 1%, 2.5%, and 5%) during extraction of olive oil (Buža cultivar) resulted in 0.7, 0.8, 1.6, and 0.8 mg per kg oil, respectively.26 Another research addressed the factor of pitting through their experiment on five different cultivars. Based on their findings, the apigenin concentrations varied depending on different varieties as follows: (i) Picual cultivar: 4.55 mg kg−1 (unpitted) and 4.49 mg kg−1 (pitted), (ii) Koronakii cultivar: 2.30 mg kg−1 (unpitted) and 1.30 mg kg−1 (pitted), (iii) Arbiquene cultivar: 6.19 mg kg−1 (unpitted) and 7.12 mg kg−1 (pitted), (iv) Carotenia cultivar: 5.18 mg kg−1 (unpitted) and 4.50 mg kg−1 (pitted), and (v) Frantoi cultivar: 7.19 mg kg−1 (unpitted) and 7.00 mg kg−1 (pitted).40 Also in the study of López-Yerena et al.,39 the extra virgin olive oil (Corbella cultivar) when processed through a low-temperature crushing (10 °C) yielded more of apigenin content (2.85 mg kg−1) compared to that obtained with higher temperature (1.43 mg kg−1 at 25 °C).
Conclusions
The present study demonstrated that size reduction of added olive leaves is among the key factors affecting the polyphenolic content of the resulting olive oil. Of particular interest was the addition of 0.3 mm leaves during crushing of pitted olives, prior to a 30 min malaxation, that favorably exhibited maximum effects on the enhancement of: (i) polyphenol concentrations, especially oleuropein and verbascoside, (ii) antioxidant capacity, and (iii) oxidative stability, without adverse impact on the yield of the extracted oil.
The data from this study may derive benefit from an in-depth research work based on olive leaf addition at various processing points of oil extraction considering a broader range of size fractions and their possible interactions with other major factors including olive cultivars. Beyond the extensive investigation of polyphenols and antioxidant potency, a need also exists to assess organoleptic quality of the leaf-added oils. In light of further information on the particle size reduction of olive leaves, the commercial entities may receive benefits of re-utilizing dry ground olive leaves sustainably in a range of food applications beyond olive oil production.
Author contributions
Fereshteh Safarzadeh Markhali: investigation; methodology; writing manuscript; conceptualization; data analysis; resources. José A. Teixeira: conceptualization; supervision; methodology; review and editing; resources.
Conflicts of interest
There are no conflicts to declare.
Acknowledgements
We thank Eulogio Castro, Center for Advanced Studies in Energy and Environment, University of Jaén, Campus of Las Lagunillas, Jaén, Spain, for supplying the olive fruits and olive leaves. This study was supported by the Portuguese Foundation for Science and Technology (FCT), under the scope of the strategic funding of UID/BIO/04469/2020 unit.
References
- F. Chemat, M. A. Vian, A. S. Fabiano-Tixier, M. Nutrizio, A. R. Jambrak, P. E. S. Munekata, J. M. Lorenzo, F. J. Barba, A. Binello and G. Cravotto, Green Chem., 2020, 22, 2325–2353 RSC.
- F. Safarzadeh Markhali, Processes, 2021, 9(9), 1662 CrossRef.
- F. Safarzadeh Markhali, Processes, 2021, 9(6), 953 CrossRef.
-
AOCS, American Oil Chemists’ Society, Official Methods and Recommended Practices of the American Oil Chemists' Society, Champaign, 5th edn, III, 1998 Search PubMed.
- M. I Minguez-Mosquera, L. Rejano, B. Gandul, A. H. Sanchez and J. Garrido, J. Am. Oil Chem. Soc., 1991, 68, 322–337 CrossRef.
- J. Lozano-Castellón, A. López-Yerena, A. Olmo-Cunillera, O. Jáuregui, M. Pérez, R. M. Lamuela-Raventós and A. Vallverdú-Queralt, Antioxidants, 2021, 10(4), 540 CrossRef PubMed.
- J. Quero, L. F. Ballesteros, P. Ferreira-Santos, G. R. Velderrain-Rodriguez, C. M. Rocha, R. N. Pereira, J. A. Teixeira, O. Martin-Belloso, J. Osada and M. J. Rodríguez-Yoldi, Antioxidants, 2022, 11(5), 828 CrossRef CAS PubMed.
- W. Brand-Williams, M. E. Cuvelier and C. Berset, LWT--Food Sci. Technol., 1995, 28, 25–30 CrossRef CAS.
- F. Safarzadeh Markhali, J. A. Teixeira and C. M. Rocha, Clean Technol., 2022, 4(2), 512–528 CrossRef.
- L. F. Benzie and J. J. Strain, Anal. Biochem., 1996, 239(1), 70–76 CrossRef PubMed.
- R. Re, N. Pellegrini, A. Proteggente, A. Pannala, M. Yang and C. Rice-Evans, Free Radical Biol. Med., 1999, 26(9–10), 1231–1237 CrossRef CAS PubMed.
- V. L. Singleton and J. A. Rossi, Am. J. Enol. Vitic., 1965, 16(3), 144–158 CrossRef CAS.
-
IOC, International Olive Council, Trade Standard Applying to Olive Oils and Olive Pomace Oils, 2019 Search PubMed.
-
Commission of the European Communities, Regulation NO. 2568/91, Off. J. Eur. Communities, No L 248, 1991 Search PubMed.
- H. A. Sari and R. Ekinci, J. Food Sci. Technol., 2017, 37, 493–499 CrossRef.
- C. Sanmartin, I. Taglieri, M. Macaluso, C. Sgherri, R. Ascrizzi, G. Flamini, F. Venturi, M. F. Quartacci, F. Luro, F. Curk and L. Pistelli, Molecules, 2019, 24(14), 2625 CrossRef PubMed.
- A. Sonda, Z. Akram, G. Boutheina, F. Guido and B. Mohamed, J. Agric. Food Chem., 2014, 62(1), 251–263 CrossRef PubMed.
- R. Malheiro, S. Casal, H. Teixeira, A. Bento and J. A. Pereira, Food Bioprocess Technol., 2013, 6, 509–521 CrossRef CAS.
- D. Sevim, O. Tuncay and O. Koseoglu, J. Am. Oil Chem. Soc., 2013, 90, 1359–1369 CrossRef CAS.
- F. Safarzadeh Markhali, J. A. Teixeira and C. M. Rocha, Processes, 2020, 8(9), 1177 CrossRef.
- I. Tarchoune, C. Sgherri, J. Eddouzi, A. Zinnai, M. F. Quartacci and M. Zarrouk, Molecules, 2019, 24, 545 CrossRef PubMed.
- B. Baccouri, I. Rajhi, S. Theresa, Y. Najjar, S. N. Mohamed and I. Willenberg, Eur. Food Res. Technol., 2022, 248(11), 2809–2823 CrossRef CAS PubMed.
- S. Ammar, H. Kelebek, A. Zribi, M. Abichou, S. Selli and M. Bouaziz, Food Res. Int., 2017, 100, 477–485 CrossRef CAS PubMed.
- L. Di Giovacchino, F. Angerosa and L. Di Giacinto, J. Am. Oil Chem. Soc., 1996, 73, 371–374 CrossRef CAS.
- M. A. Mezghani, M. Tekaya, A. Mguidich, I. Zouari, M. Ayadi, O. Elloumi, D. Saidana, S. B. Mansour-Gueddes, G. Flamini and B. Mechri, Int. J. Food Prop., 2023, 17(1), 751–764 Search PubMed.
- A. Novoselić, D. Klisović, I. Lukić, M. Lukić and K. Brkić Bubola, Agriculture, 2021, 11(10), 917 CrossRef.
- S. Achat, V. Tomao, K. Madani, M. Chibane, M. Elmaataoui, O. Dangles and F. Chemat, Ultrason. Sonochem., 2012, 19(4), 777–786 CrossRef CAS PubMed.
- F. Ramos-Escudero, M. T. Morales and A. G. Asuero, Int. J. Food Prop., 2015, 18(2), 348–358 CrossRef CAS.
- M. Öğütçü, M. Mendeş and E. Yılmaz, J. Am. Oil Chem. Soc., 2008, 85(5), 441–456 CrossRef.
- K. Kiritsakis, M. G. Kontominas, C. Kontogiorgis, D. Hadjipavlou-Litina, A. Moustakas and A. Kiritsakis, J. Am. Oil Chem. Soc., 2010, 87, 369–376 CrossRef CAS.
- C. Samaniego Sánchez, A. M. Troncoso González, M. C. García-Parrilla, J. J. Quesada Granados, H. López García de la Serrana and M. C. López Martínez, Anal. Chim. Acta, 2007, 593(1), 103–107 CrossRef PubMed.
- N. Pellegrini, F. Visioli, S. Buratti and F. Brighenti, J. Agric. Food Chem., 2001, 49(5), 2532–2538 CrossRef CAS PubMed.
- F. Galvano, L. La Fauci, G. Graziani, R. Ferracane, R. Masella, C. Di Giacomo, A. Scacco, M. D'Archivio, M. L. Vanella and G. Galvano, J. Med. Food, 2007, 10(4), 650–656 CrossRef CAS PubMed.
- S. Gorinstein, O. Martin-Belloso, E. Katrich, A. Lojek, M. Číž, N. Gligelmo-Miguel, R. Haruenkit, Y. S. Park, S. T. Jung and S. Trakhtenberg, J. Nutr. Biochem., 2003, 14(3), 154–159 CrossRef CAS PubMed.
- M. Servili, A. Taticchi, S. Esposto, S. Urbani, R. Selvaggini and G. Montedoro, J. Agric. Food Chem., 2007, 55(17), 7028–7035 CrossRef CAS PubMed.
- D. Cuffaro, S. Bertini, M. Macchia and M. Digiacomo, Nutrients, 2023, 15(5), 1073 CrossRef CAS PubMed.
- V. Sanchez de Medina, F. Priego-Capote and M. D. L. de Castro, J. Agric. Food Chem., 2012, 60(23), 5866–5873 CrossRef CAS PubMed.
- C. Fanali, S. Della Posta, A. Vilmercati, L. Dugo, M. Russo, T. Petitti, L. Mondello and L. De Gara, Molecules, 2018, 23(12), 3249 CrossRef PubMed.
- A. López-Yerena, A. Ninot, N. Jiménez-Ruiz, J. Lozano-Castellón, M. Pérez, E. Escribano-Ferrer, A. Romero-Aroca, R. M. Lamuela-Raventós and A. Vallverdú-Queralt, Antioxidants, 2021, 10(6), 877 CrossRef PubMed.
- M. M. Amany Basuny, M. S. Arafat and M. M. Dalia Mostfa, J. Food Sci. Technol., 2008, 21–29 Search PubMed.
|
This journal is © The Royal Society of Chemistry 2023 |