In situ crosslinked Schiff base biohydrogels containing Carica papaya peel extract: application in the packaging of fresh berries†
Received
27th June 2023
, Accepted 12th September 2023
First published on 13th September 2023
Abstract
Although endowed with antimicrobial and antioxidant properties, fruit peel is often regarded as waste. With the focus largely on sustainable development and sustainability, this study explores the valorisation of aforesaid waste. In this work, the influence of C. papaya peel powders on the functional properties of Schiff base hydrogels of chitosan (Cs) and dialdehyde starch (DAS) was investigated for active food packaging. In this work, the hydrogels were fabricated by virtue of in situ crosslinking of –CHO moieties of DAS and –NH2 groups of Cs. The utilization of C. papaya peel extract in hydrogel films enhanced their thickness, moisture content and opacity. Furthermore, the developed hydrogel film offered versatile performances in terms of antimicrobial and antioxidant activities, water-barrier properties, high tensile strength, stretched/twisted flexibility, anti-puncture resistance, thermal stability and biodegradability. Noteworthy, the shelf life of fresh blueberries, strawberries, gooseberries and Indian jujube was extended by at least one week upon packaging with the fabricated material as compared with unpackaged ones. Thus, our work paves the path for the design of a versatile hydrogel film for potential food packaging and preservation.
Sustainability spotlight
The present study highlights the valorization of Carica papaya peel extract towards the fabrication of biohydrogels for the packaging of fresh berries. In situ formed Schiff base hydrogels composed of dialdehyde starch and chitosan were fabricated and the peel extract was incorporated into the matrix. The utilization of the C. papaya peel extract in the film enhanced its thickness, moisture content and opacity. Furthermore, the developed hydrogel offered versatile performances in terms of antimicrobial and antioxidant activities, water-barrier properties, high tensile strength, stretched/twisted flexibility, anti-puncture resistance, thermal stability and biodegradability. The shelf life of fresh blueberries, strawberries, gooseberries and Indian jujube were extended by at least one week upon packaging with the fabricated biohydrogels as compared with the unpackaged ones. Thus, the developed biohydrogel films ensure enhanced shelf lives of the berries and show potential as sustainable packaging materials.
|
1. Introduction
With the world population striking 8 billion on 15 November 2022 and projected to escalate to 8.5 billion by 2030,1 the need for food supply shall inevitably surge. To keep up with the rising food demand, the generation of food packaging materials will predictably rise. Indisputably, plastics are still the most desirable choice for packaging materials as they are cheaper and can be attuned to different packaging formats. However, the overwhelming use of plastic packaging in food industries has not only led to alarming environmental concerns, but also startled the scientific community from the data pertaining to human consumption of microplastics.2 Thus, the food industry is in urgent need of promising materials that will altogether be a superior alternative to plastics. Besides their biodegradable and sustainable nature, such materials should be endowed with functionalities in terms of antioxidants or antimicrobials to prevent food spoilage and enhance their quality and shelf life.
Polymeric hydrogels have been on the fore towards the fabrication of food packaging materials, as they are bestowed with a suitable conformation that regulates the moisture generated by food products.3 Hydrogel applications in the food sector have seen an upward trend as hydrogel is a viable alternative to plastics for preventing food spoilage. Their inherent attributes such as swelling, mechanical integrity and biodegradability render them as commendable choices compared to conventional packaging. With judicious choice of parent polymers, the designed hydrogels have been bestowed with improved gas/vapour barrier properties and antimicrobial activities.4 Of particular interest are the bio-inspired polysaccharide hydrogels that find prominence today owing to their noble attributes such as nontoxicity and biodegradability.4 Literature is invariably abound with reports signifying the high relevance of natural polysaccharides such as chitosan,5 cellulose,6 pectin,7 alginate,8 guar gum,9 xanthan gum,10 gellan gum,11 and gelatin12 in active food packaging.
Nonetheless, one of the major shortcomings associated with hydrogels is their low stability when subjected to some stimuli.4 In order to overcome this limitation, the incorporation of compounds, mainly natural extracts from peels of fruits and vegetables, has allured researchers. Such compounds are associated with an array of bioactive functionalities such as polyphenols, sugars, terpenes, and carotenoids that play a vital role in engineering the hydrogels for various applications. The phenolic compounds, in particular, have attracted significant attention as they show great potential as antioxidant, anticancer, antibacterial, and anti-biofilm agents in the fields of health and environment.13,14 Diverse studies have reported active food packaging hydrogels designed from the extracts of pomegranate peels,15 green tea,16 neem,17 shallot wastes,18 banana leaves,19 black grapes,20 and potato peels.21
Papaya (Carica papaya L.) belonging to the Caricaceae family is a popular fruit with great health benefits and cultivated mostly in tropical regions. Papaya is enriched with a plethora of bioactive compounds that are highly important in human diet. Thus, it comes as no surprise that papaya harvesting generates tremendous wastes, mostly in the form of peels. Conventionally, these papaya peels (PPs) have been used in cattle feed, nutraceutical supplements and domestic remedies. However, with the booming knowledge on the importance of natural extracts, the utilization of PPs to develop value-added products has provoked researchers. Endeavours in this direction has led to the valorization of PPs as biofuels, dietary fibers and biomaterials.22 PP extract (PPE) has also been effectually utilized as a corrosion inhibitor for aluminium alloy.23 A couple of reports have noted the efficacy of gelatin and PPE hydrogels in packaging.24,25
Starch and chitosan are two important biopolymers that are widely used in the food processing sector.26 Although the aforesaid polymers have several advantages in food packaging,26 when used alone they suffer from poor mechanical properties. Hence, we combined these two biopolymers to alleviate the disadvantages in the use of hydrogels derived from monolithic materials. Currently adopted hydrogel synthesis strategies have often relied on the use of chemical crosslinking reagents to accomplish a three-dimensional network structure. With the focus on green chemistry looming large today, a bio-based crosslinker will be beneficial in the long run. Of late, hydrogels employing in situ crosslinking Schiff base bonds have garnered prominence owing to their high sensitivity, good recyclability and fast response to external stimuli.27 To be specific, the oxidation of natural polysaccharides by periodates has gained substantial interest. First, the synthesis ensues a bio-based material, and second, the periodate can be recycled.28 In addition, these aldehyde groups serve as crosslinking hotspots for a variety of other chemical reactions. One such promising reaction that has gained eminence is the Schiff base forming crosslinking reaction utilizing imine bonds. Thus, in this context, we prepared in situ crosslinked Schiff base hydrogels devoid of any external crosslinkers.
In the present research contribution, in situ forming Schiff base hydrogels of starch and chitosan were explored for their utility as potential food packaging materials. First, pristine starch was functionalized to dialdehyde starch (DAS) via periodate oxidation. The Schiff base hydrogel resulted from the crosslinking of aldehyde groups of DAS and amino groups of chitosan (designated as CsDAS hydrogel). An active food packaging system was developed by incorporating the C. papaya peel extract into the hydrogels. The hydrogel characteristic parameters and its utility in extending the shelf life of berries were evaluated (Scheme 1).
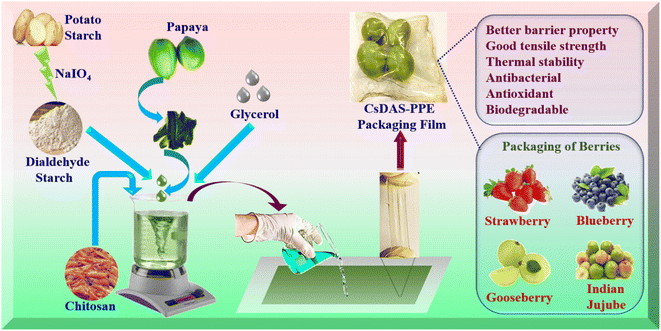 |
| Scheme 1 Diagrammatic depiction of the fabrication of chitosan (Cs)-dialdehyde starch (DAS) Schiff base hydrogels containing C. papaya peel extract (CsDAS-PPE hydrogel) as novel packaging materials for fresh berries. | |
2. Materials and methods
2.1. Materials
Cs (de-acetylation degree > 75%) and starch were obtained from Sigma Aldrich, India and used as received. We estimated Mv, which is the viscosity-average molecular mass of Cs, to be 1.78 × 105. The detailed procedure for the estimation of Mv is reported in our earlier published paper.29 All other reagents procured from Sigma-Aldrich (India) and/or HiMedia Laboratories (India) were of the highest purity and used without further purification.
2.2. Preparation of PPE and analysis by GCMS
Green papayas were purchased from the local market in Cuttack, Odisha and peeled. The papaya peel extract (PPE) was obtained by a method reported in the literature;15 the detailed procedure is presented in Section S2 in ESI.† The phytochemical analysis of PPE was performed using a GC-MS system (Agilent 7890B GC coupled with 5977A MSD). The experimental conditions were set as: 1 μL injection volume; splitless injection; oven programmed for 50 °C (1 min hold) at a rate of 5 °C per min to 300 °C (5 min hold). He carrier gas was set as: flow rate: 1 mL min−1; purge flow: 3 mL min−1. Data analysis was performed by MSD Chem Station Data and compared with the standard NIST14 Library.
2.3. Preparation of DAS, CsDAS, and CsDAS-PPE hydrogels
Dialdehyde starch (DAS) was obtained by the conventional periodate oxidation method.30 The yield and aldehyde content were determined by a method reported previously.31
For hydrogel preparation, 2 wt% Cs solution was prepared in 0.1 M acetic acid. Then, the DAS solution (1 wt%) was dissolved separately in water. Both the polymer solutions were mechanically agitated at 350 rpm for 3 h to allow for in situ crosslinking. The obtained mixture solution was casted and dried in vacuo. For PPE-incorporated hydrogels, the PPE was added at three different concentrations of 1%, 3%, and 5% (w/v) into the CsDAS solution and stirred for 30 min to ensure proper homogenization. The samples, designated as CsDAS1, CsDAS3, CsDAS5 respectively, were dried in a similar manner as described earlier. In all the prepared samples, glycerol (1% w/v) was added as a plasticizer.
2.4. Characterization
The thickness, moisture content, solubility and opacity of the CsDAS and CsDAS-PPE hydrogels were estimated as per the procedures reported in the literature.25 The water vapour permeability (WVP) and oxygen permeability (OP) were determined by a method reported in the literature.32 The FTIR spectra were recorded using a Thermo Nicolet iS5 FTIR spectrophotometer. The morphology was examined using a field emission scanning electron microscope (Carl Zeiss-Gemini FESEM 300). Thermogravimetric analysis (TGA) was performed using a NETZSCH STA 449F3 at a temperature ranging from ambient to 1000 °C in a N2 atmosphere. For the mechanical tests, the hydrogel films of dimensions (50 mm × 15 mm) were equipped with a 1 kN load cell and a strain rate of 10 mm min−1 was employed in an Instron 5567 (Instron, USA) at room temperature of 25 °C. The methodologies are presented in Sections S3–S5 in ESI.†
2.5. Antimicrobial studies
In this study, the disc diffusion method was employed.25 The antimicrobial effects of the hydrogels against Escherichia coli and Micrococcus lysodeikticus were estimated by taking 5 mm discs of the hydrogels and investigating the zones of inhibition against the respective pathogens. All experiments were performed in triplicate.
2.6. Antioxidant studies
The antioxidant properties of the hydrogels were examined for their TPC and DPPH radical scavenging activities as reported in the literature.25 The data are presented as mean ± SD of three independent readings.
2.7. Biodegradation test
The extent of biodegradability of the prepared hydrogels was studied by the soil burial method as reported in the literature.18 The hydrogels (approximately weighing 0.5 g) were buried in 100 g soil and the change in weight was measured at specific intervals. The detailed method is presented in Section S6 in ESI.†
2.8. Application in preservation of fresh berries
The potential of the prepared hydrogels as packaging materials was investigated by wrapping them on strawberries, gooseberries, Indian jujube and blueberries procured locally. Prior to the conduction of experiment, the berries were thoroughly washed to get rid of any traces of dirt or impurity. Packages were made (15 cm × 15 cm) by heat sealing. For every package, four small holes were punctured to enable cell respiration. Subsequently, berries were segregated into four batches according to their type; control batch without any packaging, berries were wrapped with a commercially available polyethylene film, a CsDAS film and a CsDAS5 film. For each berry type, five replicate packages were prepared and noted for their freshness during the experimental tenure. Ambient storage conditions were maintained throughout (25–28 °C). Berries with any signs of contamination or brown spots were considered for the calculation of fungal decay. According to Zhao et al.33 the rate of fungal decay (%) was calculated as: (the number of decayed fruits/total number of fruits) × 100. The weight loss (%) was measured as: (initial weight − final weight)/initial weight × 100, where the initial weight refers to the original weight of the berries and the final weight is the weight of the respective berries after storage.33 For strawberries in particular, the titratable acid content and total soluble solid content were also evaluated by previously reported methods.33,34 The evaluation parameters were monitored every day for each group and determined in triplicate.
3. Results and discussion
3.1. GC-MS analysis of C. papaya peel extract
The compositional analysis was performed by GC-MS and the phyto-compounds were identified from the matches in the mass spectra in the NIST14 library. The phytochemicals present in the C. papaya peel extract with their corresponding retention time (RT), molecular formula (MF) and molecular weight (MW) are presented in Table 1. The GCMS profiling revealed the presence of an array of metabolites, as projected in Table 1, that include fatty acids, esters, alkanes, alkenes, tocopherols and sterols. Previous reports on the C. papaya leaf, fruit, seed and peel extracts have also demonstrated the presence of a wide variety of bioactive compounds that have exhibited antimicrobial, antioxidant, anticancer and other medicinal properties.35–38
Table 1 Phytochemicals identified in the C. papaya peel extract by GC-MS
Sl. No. |
RT (min) |
Phytocomponentsa |
MF |
MW |
Compounds identified at 90% similarity with the standard mass spectra in NIST 14 library. RT: retention time; MW: molecular weight; MF: molecular formula.
|
1 |
3.221 |
17-Octadecynoic acid |
C18H32O2 |
280 |
2 |
3.749 |
Octadecanoic acid |
C18H36O2 |
284 |
3 |
4.398 |
n-Hexadecanoic acid |
C16H32O2 |
256 |
4 |
5.505 |
Tetradecanoic acid |
C14H28O2 |
228 |
5 |
5.734 |
Oleic acid |
C18H34O2 |
282 |
6 |
10.824 |
9-Decenoic acid |
C10H18O2 |
170 |
7 |
14.507 |
Phytol |
C20H40O |
296 |
8 |
15.149 |
p-Xylene |
C8H10 |
106 |
9 |
16.136 |
Phytol acetate |
C22H42O2 |
338 |
10 |
19.005 |
Campesterol |
C28H48O |
400 |
11 |
19.826 |
Limonene 1,2-epoxide |
C10H16O |
152 |
12 |
20.672 |
Squalene |
C30H50 |
410 |
13 |
21.225 |
Stigmasterol |
C29H48O |
412 |
14 |
21.357 |
Tetracosanoic acid |
C24H48O2 |
368 |
15 |
22.072 |
Tetracosanoic acid, methyl ester |
C25H50O2 |
382 |
16 |
23.231 |
Tetradecyl alcohol |
C14H30O |
214 |
17 |
23.79 |
γ-Sitosterol |
C29H50O |
414 |
18 |
24.520 |
Linoleic acid, phenylmethyl ester |
C25H38O |
370 |
19 |
25.667 |
Hexadecanoic acid, isopropyl ester |
C19H38O2 |
298 |
20 |
30.114 |
1-Hexacosene |
C26H52 |
364 |
21 |
30.776 |
1-Tetradecyl acetate |
C16H32O2 |
256 |
22 |
31.232 |
Hexadecanoic acid, ethyl ester |
C18H36O2 |
284 |
23 |
31.711 |
Oleic acid |
C18H34O2 |
282 |
24 |
32.069 |
10-Undecenal |
C11H20O |
168 |
25 |
33.332 |
Hexadecanoic acid, 2,3-dihydroxypropyl ester |
C19H38O4 |
330 |
3.2. Synthesis and FTIR spectral analysis of DAS
Periodate oxidation selectively cleaves the carbon–carbon bond of vicinal –OH groups of the glucopyranose residues, resulting in the ring opening of the polysaccharide. In our study, starch was selectively oxidized to form dialdehyde starch (DAS) (Fig. 1A). The yield and degree of oxidation were evaluated to be 88.66% and 42% respectively. Fig. 1B illustrates the FTIR spectra of starch and DAS. Pristine starch showed a broad band around 3500–3000 cm−1 attributed to the hydroxyl stretching vibration and a band at 1640 cm−1 assigned to the scissoring of two O–H bonds of absorbed water molecules.39 However, besides the aforementioned peaks, there appeared a new peak at 1735 cm−1 in the spectrum of DAS, which is the characteristic absorption peak of C
O vibrations of aldehyde groups in DAS.40 In addition, a sharp band is clearly evident around 750 cm−1 in the spectrum of DAS. This could be ascribed to the C–H out-of-plane bending arising from the formation of hemiacetals between –CHO and –OH groups.41 Thus, the FTIR analyses validated the formation of DAS.
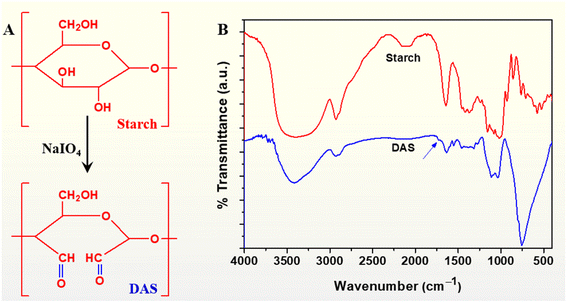 |
| Fig. 1 Oxidation of starch to dialdehyde starch (DAS). (A) Representation and (B) FTIR spectra of starch and DAS. | |
3.3. Schiff base hydrogel formation
Fig. 2A depicts the plausible scheme of Schiff base hydrogel formation between Cs and DAS. The CsDAS hydrogel was accomplished via the in situ Schiff base reaction, which was ascertained from the peak at 1635 cm−1 in the FTIR spectrum of CsDAS (Fig. 2B). This peak is attributed to the C
N linkages (imine bonds) of the Schiff base reaction between the aldehyde and amine. After the addition of C. papaya peel extract (PPE) into the CsDAS films, no new peaks or significant wavelength shifts were observed, indicating the absence of any covalent bonds between PPE and CsDAS.42 Thus, it could be inferred that the interaction between CsDAS and PPE was likely a physical response. It was also witnessed that the broad peak around 3400 cm−1 flattened with the increase in PPE content, which indicated the increasing hydrogen bonds between the polyphenols of PPE and the –OH or –NH groups of the CsDAS hydrogel.43,44 Therefore, the FTIR spectral data affirmed the formation of CsDAS hydrogel and signified the interaction of the C. papaya peel extract with the parent hydrogel.
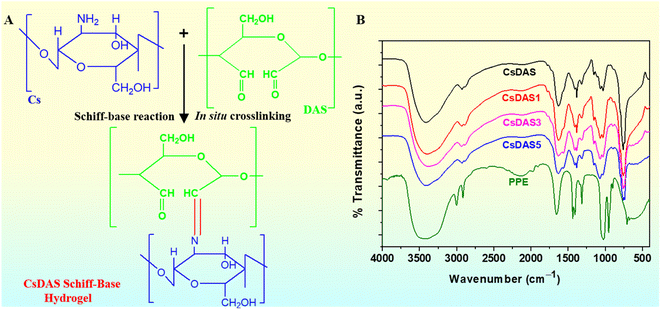 |
| Fig. 2 (A) Schematic of the Schiff base CsDAS hydrogel formation from chitosan (Cs) and dialdehyde starch (DAS) and (B) FTIR spectra of the C. papaya peel extract (PPE), CsDAS hydrogel and C. papaya peel extract-incorporated hydrogel films at 1% (CsDAS1), 3% (CsDAS3), and 5% (CsDAS5). | |
3.4. Physical properties of hydrogel packaging films
Various physical properties of the constructed CsDAS and CsDAS-PPE hydrogel films in terms of their appearances, thickness, moisture content, solubility, swelling, permeability to water and oxygen and their opacity were estimated, and the results are presented in Table 2.
Table 2 Summary of the physical parameters of the packaging filmsa
Films |
Thickness (mm) |
Moisture content (%) |
Water solubility (%) |
Swelling (%) |
WVP × 10−11 (g ms−1 Pa−1) |
OP × 10−6 (g per day m−1 Pa−1) |
Opacity |
Data presented as mean ± SD (n = 3) and the superscripts in the same column indicate significant differences (p ≤ 0.05) between the samples. CsDAS is the blank hydrogel film; CsDAS1, CsDAS3, CsDAS5 are the hydrogel films incorporated with C. papaya peel extract at 1%, 3%, 5% respectively. WVP is water vapour permeability and OP represents oxygen permeability.
|
CsDAS |
0.083 ± 0.01a |
17.31 ± 0.05b |
57.33 ± 0.04a |
177.33 ± 11.14c |
8.6 ± 0.05b |
7.2 ± 0.11a |
0.66 ± 0.15c |
CsDAS1 |
0.097 ± 0.01d |
20.21 ± 0.11a |
60.27 ± 0.81c |
280.66 ± 20.31d |
8.1 ± 0.09c |
6.7 ± 0.13c |
1.06 ± 0.11b |
CsDAS3 |
0.109 ± 0.01b |
22.13 ± 0.07c |
62.44 ± 0.13d |
355.21 ± 19.23a |
7.5 ± 0.11a |
6.1 ± 0.21b |
1.17 ± 0.13a |
CsDAS5 |
0.117 ± 0.01c |
25.22 ± 0.11d |
68.33 ± 0.11b |
419.07 ± 23.51b |
7.2 ± 0.05d |
6.0 ± 0.05d |
1.26 ± 0.11d |
3.4.1. Film appearance and thickness.
In the visual depiction (Fig. 3A), the blank CsDAS hydrogel was transparent and devoid of any color. However, with the increase in PPE content in the hydrogels, a greenish tinge develops gradually, which is the color of the extract originally. We could further witness that the thickness of the hydrogel films gradually increased with the increase in PPE concentration. The thickness varied from 0.083 mm to 0.117 mm with a significant difference (p ≤ 0.05) (Table 2). This observation might be attributed to the incomplete dissolution of the PPE powder in the hydrogel emulsion, as it is composed of both soluble and insoluble fibers.45 This result was further justified from the SEM images (Fig. 3B), which revealed the presence of solid particles embedded on the hydrogel surface incorporated with PPE. Literature reports also point to similar changes in thickness with the addition of such extracts.25,46
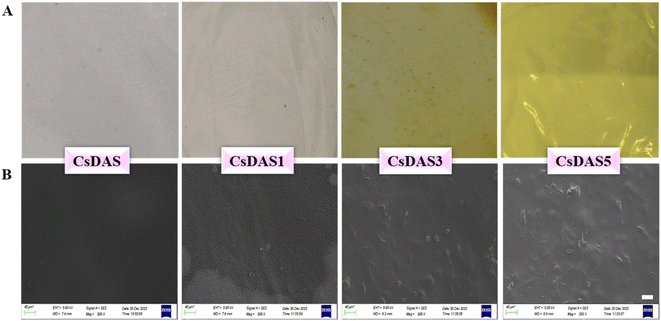 |
| Fig. 3 (A) Photographs and (B) FESEM images (scale bar = 40 μm) of the chitosan-dialdehyde starch (CsDAS) film and C. papaya peel extract-incorporated films at 1% (CsDAS1), 3% (CsDAS3), and 5% (CsDAS5). | |
3.4.2. Physical characteristics of hydrogel films.
From Table 2, an enhancement in the moisture content and water solubility of the PPE-incorporated hydrogel films is evident in comparison to the blank hydrogel film. The C. papaya peel extract comprises various hydrophilic and hydrophobic polyphenols (as presented in Table 1), which, when added to the hydrogel emulsions, largely influences the aforementioned parameters. The pristine CsDAS hydrogel film exhibited a moisture content close to 17.31%. However, the moisture content increased with higher PPE concentrations. The water solubility also displayed the same trend. This could be explained by the improved hydrophilic nature of CsDAS films, which also marks its higher swelling, as shown in Table 2. The incorporation of PPE into the hydrogel matrix facilitated the water content of the films possibly due to the interactions between the polysaccharides and polyphenols of the extract.47 As per a report by Sun and team, such an enhancement in the swellability of the films added with fruit peel extracts may be ascribed to the enhanced networking between the hydrophilic groups of the extracts with water molecules.43
3.4.3. Permeability of hydrogel films.
One of the crucial aspects of a food packaging film is preventing/retarding food deterioration. Water vapour permeability (WVP) and oxygen permeability (OP) are important parameters to assess the aforementioned permeation at a given temperature. To maintain the freshness of foods, the WVP should be retained as low as feasible. The data marked that inclusion of PPE had a diminutive influence on the WVP and OP of the CsDAS films. This reduction with the increase in PPE content suggested that the addition of PPE enhanced the water and oxygen barrier properties of the films, which may likely be ascribed to the increased film thickness (Table 2). As a result, the water vapour and/or oxygen molecules are blocked easily, i.e. the permeation time is longer. It is well established that the polyphenols in the PPE may induce interaction with the films, which resulted in restricting the interactions between hydrophilic groups of CsDAS and water oxygen molecules, thereby improving the barrier properties.48,49
3.4.4. Opacity of hydrogel films.
The color and opacity remain explicit factors that directly impact the consumer acceptance rate for the developed packaging films. The opacity of the hydrogel films is listed in Table 2. The neat CsDAS hydrogel film had higher transparency (seen from Fig. 3A) and opacity than those of the others. After the addition of PPE, the opacity was significantly (p < 0.05) increased. As a result, the packaged foods ensured protection from the visible and UV radiation that lead to losses in flavour and nutrition index.49 Thus, the results indicate that the CsDAS5 hydrogel is endowed with higher opacity and better color (from Fig. 3A) that validate its potential as a wrapping food material.
3.5. Mechanical analysis of hydrogel packaging films
In practical scenarios, the lifespan of food packaging films is recommended by their mechanical properties. Visually, the stretched CsDAS5 hydrogel film quite clearly depicted impressive stretching and twisting deformations, as shown in Fig. 4A and B respectively. The anti-puncture characteristic offered by the CsDAS5 hydrogel packaging film is also evident from Fig. 4C. The mechanical parameters in terms of E modulus, tensile strength and elongation at break (ε%) are presented in Table 3. The optimal elastic properties are displayed by the CsDAS5 hydrogel packaging film. ε% is the decisive factor for a material to acquire flexibility or brittleness. The CsDAS5 hydrogel film has better ε% and tensile strength properties among the lot. This observation could be probably attributed to the greater interaction of polyphenols in PPE with biopolymers, which may alter the hydrogen bond formation and impart stiffness to the film.18 Earlier studies reported similar effects for hydrogel films with apple peel and ginseng extracts.50,51 Thus, taken altogether, the aforementioned virtues including the mechanical strength, ε%, deformation and anti-puncture resistance put forth the high significant application of the CsDAS5 hydrogel in packaging films.
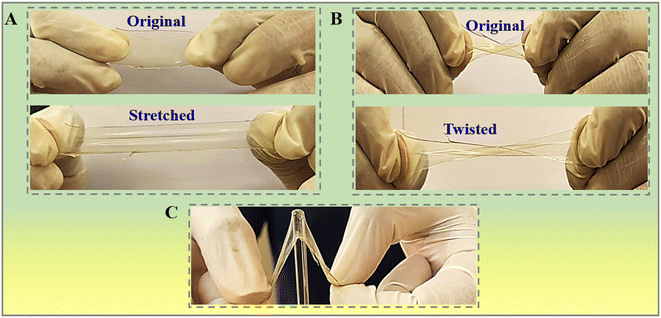 |
| Fig. 4 Photographs of (A) stretched, (B) twisted flexibility and (C) anti-puncture resistance of chitosan-dialdehyde starch films incorporated with 5% C. papaya peel extract (CsDAS5). | |
Table 3 Summary of E, σ and ε of packaging films
Films |
E (GPa) |
σ (MPa) |
ε (%) |
CsDAS |
0.34 ± 0.08 |
17.6 ± 1.3 |
27.0 ± 2.0 |
CsDAS1 |
0.41 ± 1.11 |
20.1 ± 1.1 |
26.8 ± 1.2 |
CsDAS3 |
0.48 ± 0.09 |
23.2 ± 1.2 |
25.4 ± 1.5 |
CsDAS5 |
0.51 ± 0.08 |
27.6 ± 1.1 |
23.0 ± 1.3 |
3.6. Thermal analysis of hydrogel packaging films
The thermogravimetric curves of chitosan, starch and DAS are displayed in Fig. 5A. Both Cs and starch began to decompose at about 300 °C, as witnessed in Fig. 5A. For DAS, the thermal decomposition occurred at about 190 °C and was quite slow with almost 40% remaining. This lowering in the decomposition temperature of DAS might be justified by the pyranose ring-opening in DAS upon oxidation leading to the destruction of the original structure of starch [52]. Such thermal behaviour for DAS has been reported previously.40,52–54 From the TG curves in Fig. 5B, it could be seen that the decomposition temperature for the hydrogels loaded with the C. papaya peel extract is higher than that of the neat CsDAS hydrogel. The CsDAS5 hydrogel film revealed a higher thermal stability among all the other specimens that might be associated with the hydrogen bonding interactions of the polyphenols of PPE with the biopolymer.
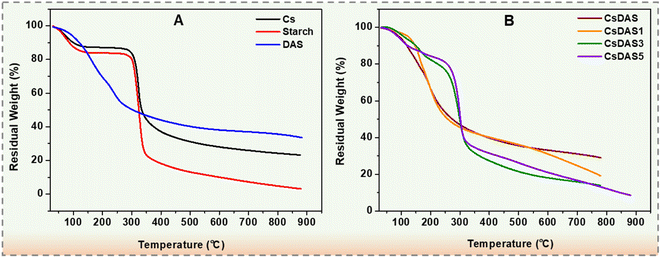 |
| Fig. 5 TG curves of (A) chitosan (Cs), starch, dialdehyde starch (DAS) and (B) chitosan-dialdehyde starch (CsDAS) film, and C. papaya peel extract incorporated films at 1% (CsDAS1), 3% (CsDAS3), and 5% (CsDAS5). | |
3.7. Antioxidant activities of hydrogel packaging films
The antioxidant activities of the CsDAS hydrogel packaging films were investigated in terms of total phenolic content (TPC) and DPPH radical scavenging assays, and are illustrated in Fig. 6A and B respectively. As witnessed from Fig. 6A, the TPC value increased when 1% C. papaya peel extract was added to the CsDAS hydrogel. An increase in PPE amount further led to an increase in TPC values. While it accounted to 1.47 μg GAE per g for pristine CsDAS, the TPC was estimated to be 2.55 μg GAE per g for the CsDAS5 hydrogel film. This behaviour could be attributed to the wide variety of phytochemicals present in PPE (as observed from the GC-MS data in Table 1), which contribute to the antioxidant activity. A high presence of antioxidants in such films will be advantageous in reducing the food oxidation and, subsequently, the deterioration.
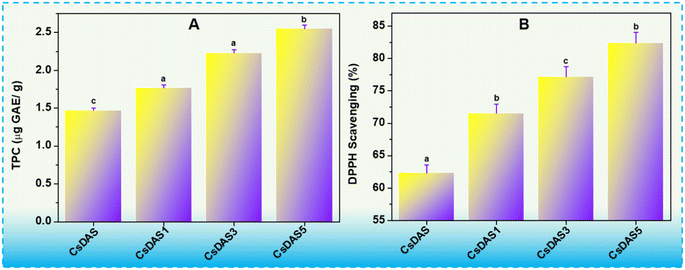 |
| Fig. 6 Antioxidant efficacy of packaging films. (A) Total phenolic content (TPC) and (B) DPPH radical scavenging activity of the chitosan-dialdehyde starch (CsDAS) film and C. papaya peel extract-incorporated films at 1% (CsDAS1), 3% (CsDAS3), and 5% (CsDAS5). Letters indicate significant differences (p < 0.05) between the samples. | |
The antioxidant capacity is suggested by the inhibition of DPPH radicals. From the results depicted in Fig. 6B; the control film (CsDAS) also showed radical scavenging activity as noted from its DPPH value, which might be ascribed to the inherent property of Cs and DAS. The hydrogel films incorporated with the C. papaya peel extract demonstrated higher antioxidant activity in comparison to the control, the CsDAS5 hydrogel being the optimal of the lot. The report by Hanani and team showed that the addition of C. papaya peel extract improved the DPPH value, as the presence of antioxidants in the films led to the acceptance of electrons and/or H-atoms.25 Thus, the incorporation of higher amounts of extract endowed the films with the desirable antioxidant activity and validated the CsDAS5 hydrogel as an apt candidate in packaging applications.
3.8. Antimicrobial activities of hydrogel packaging films
The antimicrobial activity for packaging is much an essential criterion in preventing pathogenic infections in food. Thus, the antimicrobial property of the CsDAS packaging films was evaluated. E. coli and M. luteus were chosen for this purpose. The inhibitory effects of the fabricated films on the growth of the microorganisms are presented in Table 4. Amoxicillin served as the positive control here. As witnessed, the CsDAS5 film had significant antimicrobial properties as compared to the pristine CsDAS film. The zone formed by CsDAS5 against E. coli and M. luteus bacteria was 16 and 24 mm respectively (Fig. S1 in ESI†). Recent studies have demonstrated that the bioactive components in natural extracts can damage the bacterial cell membranes leading to their inhibition.17,31 These data recommend the efficacy of CsDAS5 as the potential packaging system.
Table 4 Summary of the inhibition zones of the packaging filmsa
Films |
Zone of inhibition (mm) |
E.coli
|
M. luteus
|
Data expressed as mean ± SD. Different letters in the same column indicate significant differences (p < 0.05).
|
CsDAS |
10.05 ± 0.04a |
10.00 ± 0.11a |
CsDAS1 |
10.23 ± 0.00b |
20.01 ± 0.01b |
CsDAS3 |
12.11 ± 0.10c |
22.12 ± 0.13c |
CsDAS5 |
16.00 ± 0.01d |
24.00 ± 0.00d |
3.9. Application of hydrogels for packaging and preservation of berries
3.9.1. Application in packaging of strawberries.
Strawberry is an economically important berry fruit and much in demand by virtue of its nutritional and health effects. Unfortunately, its highly perishable nature reduces the shelf life and subsequently offers huge postharvest losses. Moreover, the berries are largely susceptible to mechanical damages and microbial infection during their harvesting and storage. Thus, the strawberry packaging industries are in urgent need of an efficient and sustainable packaging and preservation system. In this work, the potential application of the developed hydrogel films as packaging systems for strawberries was determined. Fresh strawberries were packaged in different films and changes in their weight loss, decay, TA content, TSS content, and visual appearances were periodically monitored (Fig. 7 and 8).
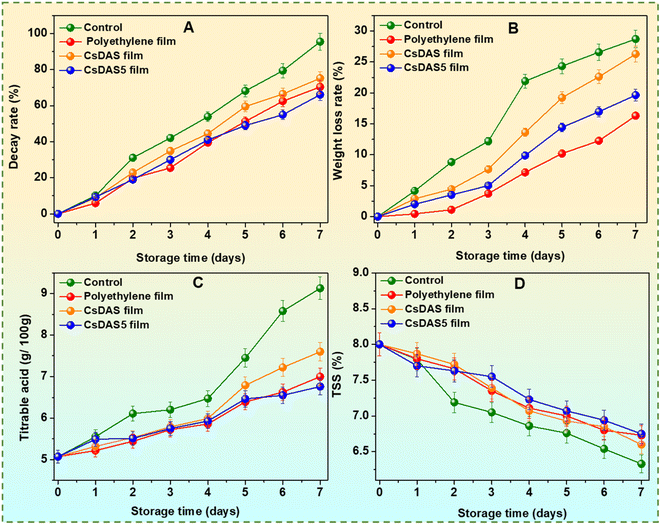 |
| Fig. 7 Quality attributes of strawberry. (A) Fungal decay rate, (B) weight loss, (C) titratable acid (TA) content and (D) total soluble solid (TSS) content of unpackaged strawberries (control) and strawberries packaged with polyethylene, a chitosan-dialdehyde starch (CsDAS) film and a 5% C. papaya peel extract-incorporated film (CsDAS5) during ambient storage period. | |
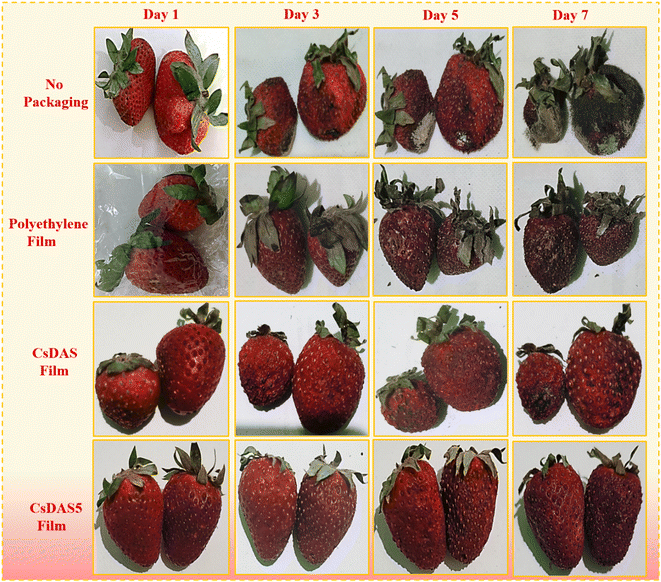 |
| Fig. 8 Photographs of unpackaged strawberries and strawberries packaged with polyethylene, a chitosan-dialdehyde starch (CsDAS) film and a 5% C. papaya peel extract incorporated film (CsDAS5) during ambient storage period. | |
Strawberries rot quite easily owing to their high susceptibility to the microbial invasion of their delicate skin. As depicted in Fig. 7A, the decay rate of strawberries devoid of any packaging peaked to almost 95% after 7 days. The presence of a packaging material inhibited the rot rate of the strawberries substantially. It was evident that the CsDAS5 hydrogel packaging film displayed a slower decay rate that could be attributed to the good anti-oxidation and bacterial inhibition attributes of the C. papaya peel extract in the film.32
The loss of water content in strawberries usually leads to cell aging and reduction of nutritional values. Fig. 7B reveals that the weight loss of all samples increased during the tenure. As expected, the loss in unpackaged strawberries was higher than that in the packaged ones. This could be attributed to the fact that the moisture could easily escape from unpackaged strawberries, while the packaging hydrogel films offered a hindrance in moisture loss due to their water vapour barrier property to some extent. The lowest rate was realized from the commercial polyethylene film because of its rigid and denser structure. The CsDAS5 hydrogel packaging film exhibited a lower weight loss rate than that of the CsDAS hydrogel, which could be justified to its better barrier properties.
The titratable acid (TA) content in strawberries is suggestive of their flavour which is proportional to the quantity of organic acids present in them.55 The TA content of all groups demonstrated a continuous upward trend (Fig. 7C), which is likely attributed to the acid production by the microorganisms on the berries.33 This observation was further corroborated from the photographs during the storage tenure (Fig. 8). In particular, the CsDAS5 hydrogel packaging film offered the lowest TA content, owing to its good antioxidant and antibacterial effects.
The total soluble solid (TSS) content pertains to the quality evaluation of the sweetness of strawberries.56Fig. 7D presents the variations in the TSS content of strawberries during experimental span. As evident, the TSS content declined for all groups, which might be due to the aging and even decay of the berries. This decrease was slower in the CsDAS5 hydrogel film group than in the CsDAS hydrogel film group, thus demonstrating its superior efficacy.
As depicted in Fig. 8, the unpackaged strawberries revealed the presence of moulds, dark coloration and evident signs of deterioration from the 3rd day onwards. Subsequently, the microbial infection gradually enhanced leading to the decay of strawberries by the 7th day. In contrast, because the CsDAS5 hydrogel packaging film was endowed with good antibacterial, antioxidant and barrier properties; the strawberries still preserved the bright red coloration and were free of molds until the 7th day. Although slight dehydration was visible, they are still acceptable for consumption. Therefore, the developed CsDAS5 hydrogel packaging film can effectually prolong the shelf life of strawberries.
3.9.2. Application in the packaging of blueberries, gooseberries and Indian jujube.
Since the fabricated CsDAS5 hydrogel film demonstrated good results on strawberries, these films were further employed in the packaging of blueberries, gooseberries and Indian jujube. As illustrated in Fig. 9A, 10A and 11A; the berries packaged with the CsDAS5 hydrogel film remained afresh even after storage for 7 days under ambient conditions. However, the deterioration phenomenon could be clearly observed in the unpackaged groups. The decay rate of the unpackaged berries was much faster than that of the berries packaged with the CsDAS5 packaging film, as revealed from Fig. 9B, 10B and 11B. Further estimation of weight loss also supported the supremacy of the CsDAS5 packaging film, shown in Fig. 9C, 10C and 11C, which could be attributed to its improved barrier properties. Thus, taken altogether, it could be recommended that the multifunctional CsDAS5 hydrogel packaging film is indeed suitable for the packaging and preservation of different types of fresh berries.
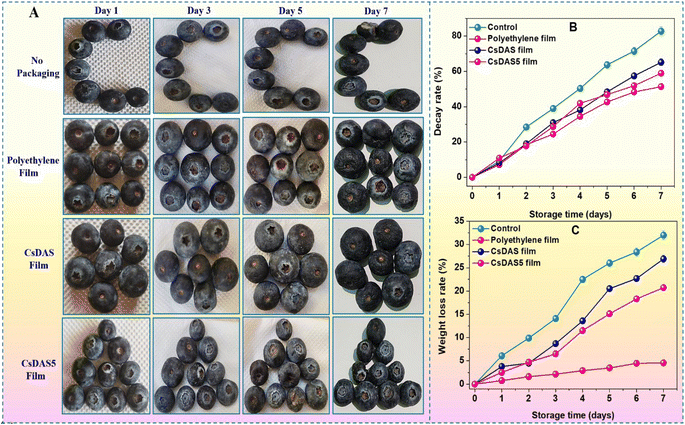 |
| Fig. 9 (A) Visual appearance, (B) fungal decay rate and (C) weight loss of unpackaged and blueberries packaged with polyethylene, a chitosan-dialdehyde starch (CsDAS) film and a 5% C. papaya peel extract–incorporated film (CsDAS5) during ambient storage period. | |
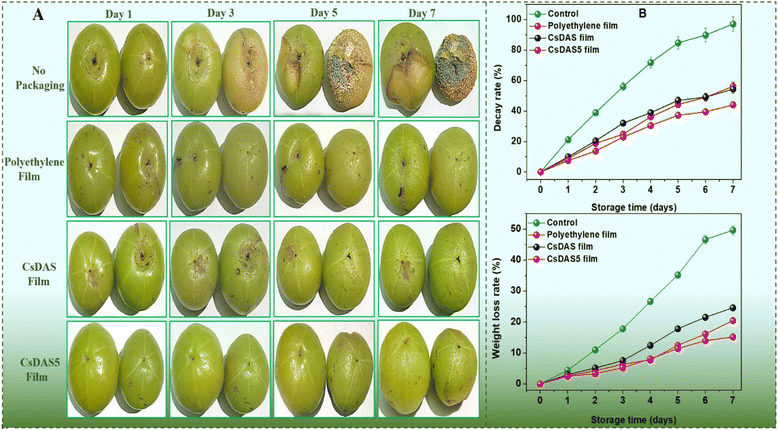 |
| Fig. 10 (A) Visual appearance, (B) fungal decay rate and (C) weight loss of unpackaged and gooseberries packaged with polyethylene, a chitosan-dialdehyde starch (CsDAS) film and a 5% C. papaya peel extract-incorporated film (CsDAS5) during ambient storage period. | |
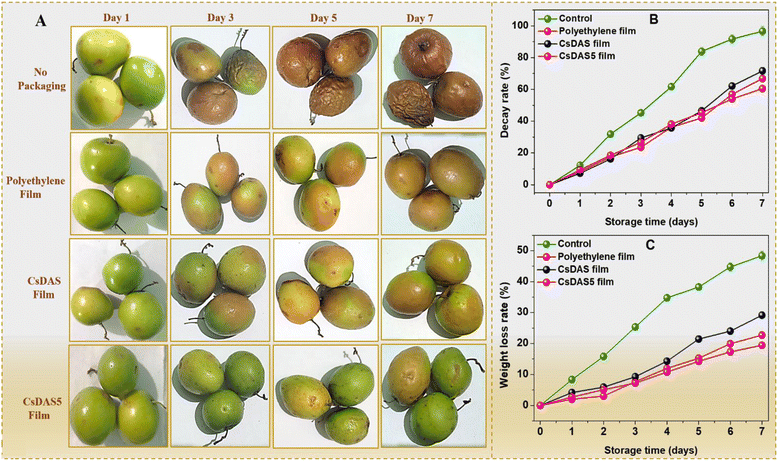 |
| Fig. 11 (A) Visual appearance, (B) fungal decay rate and (C) weight loss of unpackaged and Indian jujube packaged with polyethylene, a chitosan-dialdehyde starch (CsDAS) film and a 5% C. papaya peel extract-incorporated film (CsDAS5) during ambient storage period. | |
3.10. Biodegradation of hydrogel packaging films
Owing to budding concerns pertaining to waste management, biodegradation of the developed packaging hydrogel films was taken into consideration in this work. The biodegradability of the CsDAS5 film was estimated by measuring its weight loss as a function of time. Fig. 12A depicts the photographs of degradation of the films. This degradation is caused by the microbes in the soil leading to the chemical breakdown of films owing to biological stimulation. The results in Fig. 12B reveal that almost 80% of the film has underwent degradation by the 30th day. These data projected that the developed CsDAS5 packaging film is bestowed with good biodegradability.
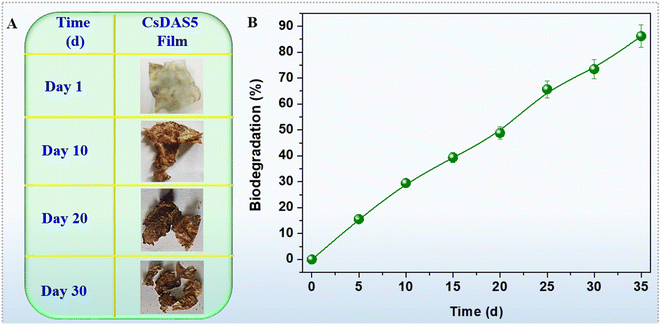 |
| Fig. 12 Biodegradation study of the 5% C. papaya peel extract-incorporated chitosan-dialdehyde starch (CsDAS5) hydrogel packaging film. (A) Visual appearance of the film with gradual degradation and (B) rate of degradation in soil. | |
4. Conclusion
The purpose of this work was to utilize papaya peel wastes towards the design of a value-added product in the form of a packaging and preservation system for fresh berries. The film fabrication was accomplished via the facile strategy of in situ crosslinking reaction between the aldehyde groups of DAS and the amino groups of chitosan. C. papaya peel powders were incorporated onto the film before casting. The incorporation of C. papaya peel extract into the films enhanced the film thickness, moisture content and opacity. Furthermore, the fabricated CsDAS5 hydrogel film offered versatile performances in terms of antimicrobial and antioxidant activities, water-barrier properties, high tensile strength, stretched/twisted flexibility, anti-puncture resistance, thermal stability and biodegradability. The CsDAS5 film demonstrated better quality attributes for the berries and effectively prolonged their shelf-life for 7 days. In conclusion, the prepared CsDAS5 hydrogel packaging film will have tremendous application prospects in the food packaging industry.
Author contributions
GD: conceptualization, investigation, formal analysis, writing – original draft. SKD: investigation, formal analysis. SSM: investigation, formal analysis. SD: conceptualization, supervision, writing – review and editing.
Conflicts of interest
The authors declare no conflicts of interest.
Acknowledgements
This research did not receive any specific grant from funding agencies in the public, commercial, or not-for-profit sectors. GD and SKD acknowledge Odisha University of Technology and Research, Bhubaneswar for providing the necessary facilities. SD and SSM express their gratitude to Department of Chemistry, Ravenshaw University, Cuttack, Odisha for providing the essential amenities to carry out this study.
References
-
World Population Prospects 2022: Summary of Results, https://www.un.org/, accessed 20 June 2023 Search PubMed.
- K. D. Cox, G. A. Covernton, H. L. Davies, J. F. Dower, F. Juanes and S. E. Dudas, Human consumption of microplastics, Environm. Sci. Technol., 2019, 53, 7068–7074 CrossRef CAS PubMed.
- R. A. Batista, P. J. Perez Espitia, J. de Souza Siqueira Quintans, M. M. Freitas, M. Cerqueira, J. A. Teixeira and J. C. Cardoso, Hydrogel as an alternative structure for food packaging systems, Carbohydr. Polym., 2019, 205, 106–116 CrossRef CAS PubMed.
- F. J. Leyva-Jimenez,
et al., Comprehensive review of natural based hydrogels as an upcoming trend for food packing, Food Hydrocoll., 2023, 135, 108124 CrossRef CAS.
- J. Yang, M. Shen, Y. Luo, T. Wu, X. Chen, Y. Wang and J. Xie, Advanced applications of chitosan-based hydrogels: from biosensors to intelligent food packaging system, Trend Food Sci. Technol., 2021, 110, 822–832 CrossRef CAS.
- K. Huang and Y. Wang, Recent applications of regenerated cellulose films and hydrogels in food packaging, Curr. Opin. Food Sci., 2022, 43, 7–17 CrossRef CAS.
- S. P. Ishwarya, R. Sandhya and P. Nisha, Advances and prospects in the food applications of pectin hydrogels, Critic. Rev. Food Sci. Nutr., 2022, 62, 4393–4417 CrossRef PubMed.
- L.-F. Wang and J.-W. Rhim, Preparation and application of agar/alginate/collagen ternary blend functional food packaging films, Int. J. Biol. Macromol., 2015, 80, 460–468 CrossRef CAS PubMed.
- S. Bandyopadhyay, N. Saha, U. V. Brodnjak and P. Saha, Bacterial cellulose and guar gum based modified PVP-CMC hydrogel films: characterized for packaging fresh berries, Food Packag. Shelf Life, 2019, 22, 100402 CrossRef.
- A. Mohsin,
et al., Xanthan-Curdlan nexus for synthesizing edible food packaging films, Int. J. Biol. Macromol., 2020, 162, 43–49 CrossRef CAS PubMed.
- B. Rukmanikrishnan, F. R. M. Ismail, R. K. Manoharan, S. S. Kim and J. Lee, Blends of gellan gum/xanthan gum/zinc oxide-based nanocomposites for packaging application: rheological and antimicrobial properties, Int. J. Biol. Macromol., 2020, 148, 1182–1189 CrossRef CAS PubMed.
- L. Y. Maroufi, M. Ghorbani, M. Tabibiazar, M. Mohammadi and A. Pezeshki, Advanced properties of gelatin film by incorporating modified kappa-carrageenan and zein nanoparticles for active food packaging, Int. J. Biol. Macromol., 2021, 183, 753–759 CrossRef PubMed.
- U. Das, S. S. Behera, S. Singh, S. I. Rizvi and A. K. Singh, Progress in the development and applicability of potential medicinal plant extract-conjugated polymeric constructs for wound healing and tissue regeneration, Phytother. Res., 2016, 30, 1895–1904 CrossRef CAS PubMed.
- L. A. Ditta,
et al., Agarose/κ-carrageenan-based hydrogel film enriched with natural plant extracts for the treatment of cutaneous wounds, Int. J. Biol. Macromol., 2020, 164, 2818–2830 CrossRef CAS PubMed.
- L. Y. Maroufi, M. Tabibiazar, M. Ghorbani and A. Jahanban-Esfahlan, Fabrication and characterization of novel antibacterial chitosan/dialdehyde guar gum hydrogels containing pomegranate peel extract for active food packaging application, Int. J. Biol. Macromol., 2021, 187, 179–188 CrossRef CAS PubMed.
- L. Y. Maroufi, M. Ghorbani and M. Tabibiazar, A gelatin-based film reinforced by covalent interaction with oxidized guar gum containing green tea extract as an active food packaging system, Food Bioprocess Technol., 2020, 13, 1633–1644 CrossRef.
- R. Rajasekharan,
et al., Neem extract-blended nanocellulose derived from jackfruit peel for antibacterial packagings, Environ. Sci. Pollut. Res., 2023, 30, 8977–8986 CrossRef CAS PubMed.
- P. Thivya, Y. K. Bhosale, S. Anandakumar, V. Hema and V. R. Sinija, Development of active packaging film from sodium alginate/carboxymethyl cellulose containing shallot waste extracts for anti-browning of fresh-cut produce, Int. J. Biol. Macromol., 2021, 188, 790–799 CrossRef CAS.
- M. Hailu, T. Seyoum Workneh and D. Belew, Effect of packaging materials on shelf life and quality of banana cultivars (Musa spp.), J. Food Sci. Technol., 2014, 51, 2947–2963 CrossRef CAS.
- E. Kowsalya, K. MosaChristas, P. Balashanmugam, A. Tamil Selvi and J. C. Rani I, Biocompatible silver nanoparticles/poly(vinyl alcohol)electrospun nanofibers for potential antimicrobial food packaging applications, Food Packag. Shelf Life, 2019, 21, 100379 CrossRef.
- K. Miller, C. L. Reichert, M. Schmid and M. Loeffler, Physical, chemical and biochemical modification approaches of potato (peel) constituents for bio-based food packaging concepts: a review, Foods, 2022, 11, 2927 CrossRef CAS PubMed.
- P. D. Pathak, S. A. Mandavgane and B. D. Kulkarni, Waste to wealth: a case study of papaya peel, Waste Biomass Valorization, 2019, 10, 1755–1766 CrossRef.
- N. Chaubey, V. K. Singh and M. A. Quraishi, Papaya peel extract as potential corrosion inhibitor for Aluminium alloy in 1 M HCl: electrochemical and quantum chemical study, Ain Shams Eng. J., 2018, 9, 1131–1140 CrossRef.
- T. de Moraes Crizel, A. de Oliveira Rios, V. D. Alves, N. Bandarra, M. Moldao-Martins and S. H. Flores, Biodegradable films based on gelatin and papaya peel microparticles with antioxidant properties, Food Bioprocess Technol., 2018, 11, 536–550 CrossRef CAS.
- Z. A. N. Hanani, A. B. A. Husna, S. N. Syahida, M. A. B. N. Khaizura and B. Jamilah, Effect of different fruit peels on the functional properties of gelatin/polyethylene bilayer films for active packaging, Food Packag. Shelf Life, 2018, 18, 201–211 CrossRef.
- A. Manzoor, A. H. Dar, V. K. Pandey, R. Shams, S. Khan, P. S. Panesar, J. F. Kennedy, U. Fayaz and S. A. Khan, Recent insights into polysaccharide-based hydrogels and their potential applications in food sector: a review, Int. J. Biol. Macromol., 2022, 213, 987–1006 CrossRef CAS.
- S. Shekhar, V. Chaudhary, B. Sharma, A. Kumar, A. K. Bhagi and K. P. Singh, Sustainable polysaccharide hydrogels based on dynamic schiff base linkages as versatile building blocks for fabricating advanced functional materials, J. Polym. Environ., 2023, 31, 1257–1278 CrossRef CAS.
- G. Dalei, S. Das and M. Pradhan, Dialdehyde cellulose as a niche material for versatile applications: an overview, Cellulose, 2022, 29, 5429–5461 CrossRef CAS.
- G. Dalei, S. Das, S. R. Jena, J. Nayak, D. Jena and L. Samanta,
In situ crosslinked dialdehyde guar gum-chitosan Schiff-base hydrogels for dual drug release in colorectal cancer therapy, Chem. Eng. Sci., 2023, 269, 118482 CrossRef CAS.
- S. M. A. S. Keshk, A. M. Ramadan, A. G. Al-Sehemi, E. S. Yousef and S. Bondock, Peculiar behavior of starch 2,3-dialdehyde towards sulfanilamide and sulfathiazole, Carbohydr. Polym., 2016, 152, 624–631 CrossRef CAS PubMed.
- C. G. Gomez, M. Rinuado and M. A. Villar, Oxidation of sodium alginate and characterization of oxidized polysaccharides, Carbohydr. Polym., 2007, 67, 296–304 CrossRef CAS.
- V. G. Bhat,
et al., Development and evaluation of Moringa extract incorporated chitosan/guar gum/poly(vinyl alcohol) active films for food packaging applications, Int. J. Biol. Macromol., 2022, 200, 50–60 CrossRef CAS PubMed.
- Y. Zhao,
et al., High-performance carboxymethyl cellulose-based hydrogel film for food packaging and preservation system, Int. J. Biol. Macromol., 2022, 223, 1126–1137 CrossRef CAS PubMed.
- Y. He,
et al., Carboxymethyl cellulose/cellulose nanocrystals immobilized silver nanoparticles as an effective coating to improve barrier and antibacterial properties of paper for food packaging applications, Carbohydr. Polym., 2021, 252, 117156 CrossRef CAS PubMed.
- H. L. Al-Seadi, M. Z. Sabti and D. A. Taain, GC-MS analysis of papaya leaf extract (Carica papaya L.), IOP Conference Ser.: Earth Environ. Sci., 2021, 910, 012011 Search PubMed.
- A. C. Emeruwa, Antibacterial substance from Carica papaya fruit extract, J. Natur. Prod., 1982, 45, 123–127 CrossRef CAS.
- C. Baskaran, V. R. bai, S. Velu and K. Kumaran, The efficacy of Carica papaya leaf extract on some bacterial and a fungal strain by well diffusion method, Asian Pac. J. Trop. Dis., 2012, 2, S658–S662 CrossRef.
- E. Dagne, B. Dobo and Z. Bedewi, Antibacterial activity of papaya (Carica papaya) leaf and seed extracts against some selected gram-positive and gram-negative bacteria, Pharmacogn. J., 2021, 13, 1727–1733 CrossRef CAS.
- T. S. Anirudhan and J. Parvathy, Novel semi-IPN based on crosslinked carboxymethyl starch and clay for the in vitro release of theophylline, Int. J. Biol. Macromol., 2014, 67, 238 CrossRef CAS PubMed.
- P. Kuchaiyaphum, C. Chotichayapong, N. Butwong and W. Bua-ngern, Silk fibroin/poly(vinyl alcohol)hydrogel cross-linked with dialdehyde starch for wound dressing applications, Macromol. Res., 2020, 28, 844–850 CrossRef CAS.
- U.-J. Kim, S. Kuga, M. Wada, T. Okano and T. Kondo, Periodate oxidation of crystalline cellulose, Biomacromolecules, 2000, 1, 488–492 CrossRef CAS PubMed.
- D. S. Lee, J. Y. Woo, C. B. Ahn and J. Y. Je, Chitosan-hydroxycinnamic acid conjugates: preparation, antioxidant and antimicrobial activity, Food Chem., 2014, 148, 97–104 CrossRef CAS PubMed.
- L. Sun, J. Sun, L. Chen, P. Niu, X. Yang and Y. Guo, Preparation and characterization of chitosan film incorporated with thinned young apple polyphenols as an active packaging material, Carbohydr. Polym., 2017, 163, 81–91 CrossRef CAS PubMed.
- Y. Peng, Y. Wu and Y. Y. Li, Development of tea extracts and chitosan composite films for active packaging materials, Int. J. Biol. Macromol., 2013, 59, 282–289 CrossRef CAS PubMed.
- N. Hasnaoui, B. Wathelet and A. Jiménez-Araujo, Valorization of pomegranate peel from 12 cultivars: dietary fibre composition, antioxidant capacity and functional properties, Food Chem., 2014, 160, 196–203 CrossRef CAS PubMed.
- Z. Emam-Djomeh, A. Moghaddam and S. A. Yasini Ardakani, Antimicrobial activity of pomegranate (Punica granatum L.) peel extract, physical, mechanical, barrier and antimicrobial properties of pomegranate peel extract-incorporated sodium caseinate film and application in packaging for ground beef, Packag. Technol. Sci., 2015, 28, 869–881 CrossRef CAS.
- E. Matta, M. J. Tavera-Quiroz and N. Bertola, Active edible films of methylcellulose with extracts of green apple (Granny Smith) skin, Int. J. Biol. Macromol., 2019, 124, 1292–1298 CrossRef CAS PubMed.
- U. Siripatrawan and B. Harte, Physical properties and antioxidant activity of an active film from chitosan incorporated with green tea extract, Food Hydrocoll., 2010, 24, 248–252 CrossRef.
- J. F. Rubilar, M. S. C. Rui, H. D. Silva, A. A. Vicente, I. Khmelinskii and M. C. Vieira, Physico-mechanical properties of chitosan films with carvacrol and grape seed extract, J. Food Eng., 2013, 115, 466–474 CrossRef CAS.
- A. Riaz, S. Lei, H. M. S. Akhtar, P. Wan, D. Chen, S. Jabbar, M. Abid, M. M. Hashim and X. Zeng, Preparation and characterization of chitosan-based antimicrobial active food packaging film incorporated with apple peel polyphenols, Int. J. Biol. Macromol., 2018, 114, 547–555 CrossRef CAS PubMed.
- K. Norajit, K. Myong and G. Hyung, Comparative studies on the characterization and antioxidant properties of biodegradable alginate films containing ginseng extract, J. Food Eng., 2010, 98, 377–384 CrossRef CAS.
- Y. Zuo, W. Liu, J. Xiao, X. Zhao, Y. Zhu and Y. Wu, Preparation and characterization of dialdehyde starch by one-step acid hydrolysis and oxidation, Int. J. Biol. Macromol., 2017, 103, 1257–1264 CrossRef CAS PubMed.
- Z. Li, X. Wang, J. Miao, L. Xing and S. Zhang, Antibacterial activity of dodecylamine dialdehyde starch Schiff base derivatives, Starch, 2022, 74, 100178 Search PubMed.
- J. Li, A. Riley, L. Pu, H. Long and Z. Li, Preparation and characterization of a starch-based adsorbent for the effective removal of environmental pollutants Hg (II), Starch, 2020, 72, 1900148 CrossRef CAS.
- N. B. Gol, P. R. Patel and T. V. R. Rao, Improvement of quality and shelf-life
of strawberries with edible coatings enriched with chitosan, Postharv. Biol. Technol., 2013, 85, 185–195 CrossRef CAS.
- L. M. Ali, A. E. A. E. Ahmed, H. E. Hasan, A. E. E. Sulima and S. S. Saleh, Quality characteristics of strawberry fruit following a combined treatment of laser sterilization and guava leaf-based chitosan nanoparticle coating, Chem. Biol. Technol. Agric., 2022, 9, 80 CrossRef CAS.
|
This journal is © The Royal Society of Chemistry 2023 |
Click here to see how this site uses Cookies. View our privacy policy here.