Fabrication of novel gallic acid functionalized chitosan/pullulan active bio-films for the preservation and shelf-life extension of green chillies
Received
11th January 2023
, Accepted 10th March 2023
First published on 13th March 2023
Abstract
The need for eco-friendly and sustainable packaging films for the preservation of fresh food products has received considerable interest. Hence, here we developed green and active bio-films based on chitosan (CS)/pullulan (PL) bio-polymers functionalized with different weight percentages of gallic acid (GA) through the solvent casting technique. The developed CS/PL/GA (CPG) films' properties were investigated through different characterization techniques. The CPG films containing 15 wt% GA exhibited excellent UV ray blocking and high tensile properties (∼27.53%) compared to the pristine CS/PL blend film. Further, the barrier property towards water vapor (∼21.41%) and oxygen (∼34.14%) was significantly enhanced with the GA content, while moisture retention capacity and surface wettability improved but not significantly. Besides these, CPG films showed an overall migration lower than the acceptable limit of 10 mg dm−2. In addition, compared to the neat CS/PL film, CPG films exhibited excellent antioxidant (∼42.54%) and antimicrobial activities against foodborne pathogens. Moreover, the developed CPG15% film was practically tested for green chilli packaging at room temperature. Comparing CPG15% packed green chillies to unpacked green chillies, the shelf life of the green chillies was extended up to 18 days without affecting the quality. Overall, these findings imply that the developed CPG films attain promising conditions for active packaging applications of fresh food products.
1 Introduction
Nowadays, the preservation and shelf-life extension of fresh food products without affecting their quality is the biggest challenge for the food industry. Hence, the industry is extensively using synthetic and artificial food preservatives. For instance, butylated hydroxyanisole, butylated hydroxytoluene and tert–butyl hydroquinone are some important synthetic antioxidants being used in the food industry. However, these synthetic additives cause alteration to food flavors and produce a distinctive taste.1 Hence, there is a huge consumer demand for fewer and safer additives in processed food products; therefore, it has become a trend for researchers to be interested in the usage of natural plant extracts in packaging systems, which have bioactivity such as antimicrobial and antioxidant properties. However, the usage of crude plant extracts may lead to a lack of reproducibility due to the unknown composition of the crude extracts. In this direction, purified plant polyphenols such as boswellic acid, rutin, quercetin, gallic acid and citric acid are utilized as natural active components in food preservation formulations.2–5 Among these, gallic acid was considered as a suitable active component due to its large availability and convenient synthesis protocol. Gallic acid (GA) (3,4,5-trihydroxybenzoic acid) is a green, commercially available non-toxic and simple compound, which contains three hydroxyl groups.6 It is widely found in vegetables and fruits and is mainly responsible for the antioxidant process. It is known that GA has been suggested in numerous research studies to have potent antioxidant properties;7,8 due to this, GA is attracting much more attention from researchers for food packaging films.
The majority of the food industries depend on conventional petroleum-based plastics for food packaging purposes. However, these plastics cause serious effects on the environment due to their non-degradability under atmospheric conditions.9 Therefore, researchers are much more interested in the development of degradable plastics using natural polymers. Recently, the idea of bio-films, edible films and coatings for food preservation has become an emerging area of research in the packaging sector to safeguard food products, minimizing the accumulation of solid waste and reducing the wastage of food. The present methods of food preservation go beyond simple preservation, with an emphasis on two additional goals: the compatibility of the technologies utilized and the creation of ecologically friendly products without any adverse health effects.10 In this regard, currently several bio-polymers like cellulose, carrageenan, starch, natural gums, zein, polylactic acid, chitosan and pullulan are being used to prepare degradable packaging films.11,12 Among these, chitosan and pullulan are predominantly considered as suitable candidates for the development of eco-friendly food packaging plastics due to their bio-active properties.13,14 Chitosan (CS) is a naturally available abundant active polysaccharide extracted from the skeletons of fish and shrimp shells consisting of β–(1→4) glycosidic linked 2-amino-2-deoxy-D-glucosamine and N-acetyl-2-amino-2-deoxy-D-glucosamine repeating units.15 It is widely used in the development of food packaging systems due to its non-toxicity, excellent film forming and water resistant capacity, and it has the ability to inhibit a wide range of pathogens.16 Further, in most of the film formulations, CS is used as a base material for the development of bio-films due to its strong ability to interact with other polymer matrices and additives to produce new blend films with improved physico-mechanical properties.17 However, there are some drawbacks such as high brittleness and low flexibility, which prevent CS from being used commercially for food packaging purposes. To overcome these confines, CS is frequently blended with hydrophilic degradable synthetic macromolecules like polyvinyl alcohol, carrageenan and pullulan. Pullulan (PL) is another straight chain glucose polysaccharide isolated from a fungus called Aureobasidium pullulans composed of maltotriose repeating monomer units joined by α-1,6 linkages.18 It is largely employed in various applications such as drug delivery, wound care, and as a preservative in food products. In addition, it has good film forming capacity, high transparency, flexibility, and degradability and has good microbial resistant capacity, which makes it a suitable candidate for the development of active food packaging films.19
Development of food packaging systems with the incorporation of natural polyphenols like citric acid, dextran, ascorbic acid and GA has been explored to improve the multifunctional properties of the food packaging films.20–22 Further, the use of additives like green deep eutectic solvents (DES) in the development of CS based films has shown improved solubility, tensile stability and flexibility.23–25 For instance, Zarandona et al. (2020) claimed that the incorporation of GA greatly enhanced the antimicrobial and antioxidant property of the chitosan films,26 and also tensile strength and elongation at break were significantly tailored when it was formulated with gelatin.27 Moreover, GA grafted CS films have shown the maximum water vapor barrier and thermal stability reported by Zhang et al. (2019).28 It is also observed that the shelf-life of mushrooms was noticeably extended up to 24 days when packed with dextran integrated CS film.29 On the basis of the findings above, it has been established that the use of green components as additives significantly affects the functional properties of polymeric film matrices. The hypothesis of the present work is to develop novel CS/PL based active bio-films functionalized with different wt% of GA. The physico-mechanical and functional properties of the CPG composite films were investigated and compared with those of the pristine CS and CS/PL films. Based on the literature survey, no works have been performed using CS/PL film matrices with GA. Further, this research aims to test the realistic packaging efficiency of the CPG film towards preservation and shelf-life protraction of green chillies at room temperature.
2 Materials and methods
2.1. Materials
Chitosan with a high molecular weight (200–600 kDa) extracted from shrimp shell (viscosity 5–20 mPa s and deacetylation degree 75–85%), pullulan (viscosity 15–180 mPa s) and gallic acid were bought from the Tokyo Chemical Industry, Japan. Sigma-Aldrich India provided 2,2–diphenyl-1-picrylhydrazyl (DPPH). Loba Chemie India provided the acetic acid, ethanol, and needed bioactive media. Throughout the work, Milli-Q water was used.
2.2. Method
2.2.1. Fabrication of bio-films.
Bio-films were prepared by the conventional solvent casting technique. Initially, 1.7 g of CS was dissolved in 70 mL of 2% acetic acid solution and filtered through cotton to eliminate undissolved particles. On the other hand, 0.5 g of PL and different weight percent of gallic acid (0, 5, 10, and 15 wt% with respect to the dry weight of CS and PL) were separately dissolved in distilled water. Thereafter, all solutions were blended together with stirring for 2 h. The resulting film forming solutions were cast on a dry Petri plate and dried in a cardboard box for 48 h keeping the temperature constant at 35 ± 2 °C. For comparison, pristine CS without PL and a film with only PL without GA (CS/PL) were also prepared according to the above-mentioned protocol. The dried bio-films were peeled from the Petri plates and named CS, CS/PL, CPG5%, CPG10%, and CPG15%, depending on the composition and GA concentration in the film. The films were conditioned for 5 days in a desiccator containing anhydrous CaCl2 before further study.
2.3. Characterization
2.3.1. Optical properties.
A LAMDA 365 (PerkinElmer, USA) ultraviolet-visible spectrophotometer was used to investigate the optical parameters. A film sample (3 × 1 cm2) was placed inside a UV cell, and the spectra were recorded between the wavelengths of 250 and 800 nm while using air as a reference. The film opacity and the transparency were calculated by using eqn (1) and (2). | 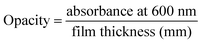 | (1) |
|  | (2) |
2.3.2. Attenuated total reflection-FT-IR analysis.
A NICOLET iZ10 (Thermo Scientific) attenuated total reflectance – Fourier transform infrared spectrometer was used to assess the bonding interaction between the CS, PL and GA. The spectrum was recorded between the wavelengths from 400 to 4000 cm−1.
2.3.3. Mechanical properties.
The film sample (2.5 × 10 cm2) with a uniform thickness was placed in a grip separation of 4 cm and stretched out at a crosshead speed of 1 mm min−1 at room temperature and mechanical parameters were assessed through stress–strain curves obtained using a universal testing machine (ASTM D882-91 standard, DAK, India) with pre-installed software.
2.3.4. Surface morphology.
A scanning electron microscope (Sigma-Zeiss) was employed to study the microstructure of the prepared bio-film surface. The film sample (1 × 1 cm2) was sputtered with gold and placed on the specimen and analysis was performed at an applied voltage of 5 kV.
2.3.5. Surface wettability.
A contact angle meter was used to examine the surface wettability of the created bio-films. The film sample (2 × 2 cm2) was fixed on a sample holder, a drop of distilled water (2 μL) was dispensed onto the film surface using a micro-syringe, and the shape of water drop image was taken using a digital camera, followed by the calculation of contact angle between the film surface and water with the help of pre-installed software. The contact angle was calculated using the mean standard deviation from five different measurements made on the same sample.
2.3.6. Water vapor transmission rate (WVTR) and oxygen permeability (OP).
In order to determine the WVTR of the prepared bio-films, an amber colored glass bottle was filled with 10 mL distilled water and the mouth of the test bottle was closed with the film sample (3 × 3 cm2) and further sealed with Teflon tape. The weight of the test bottle was noted (W1) and it was placed in a hot air oven at a constant temperature of 40 ± 2 °C for 24 h. Thereafter, the test bottle was withdrawn from the oven, cooled, and weighed again (W2). The WVTR of the films was calculated by using eqn (3).30 | 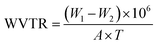 | (3) |
where T – 24 h and A – area of the mouth of the bottle (mm).
To determine the OP of the prepared bio-films, the mouth of the test bottle was sealed with the prepared bio-film (3 × 3 cm2) using Teflon tape and placed on a desiccator containing anhydrous calcium chloride. The weight of the test bottle was recorded every 24 h up to 72 h. The slope of every test film was calculated by linear regression of weight change vs. time. The oxygen permeability transmission rate (OPTR) and OP were calculated by using eqn (4) and (5), respectively.14
| 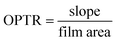 | (4) |
|  | (5) |
where Δ
P is the difference in partial pressure of oxygen across the bio-film's sides.
2.3.7. Overall migration limit (OML).
The OML of the developed bio-films was analyzed in line with the IS: 9845-1998 standard. Distilled water, 3% acetic acid and 50% ethanol were used as food simulants. Each sample (2 × 2 cm2) was placed in a beaker containing three food simulants and kept in a hot chamber at 40 °C for 10 days. Thereafter, the immersed film sample was removed and left out simulant was evaporated and weighed (W1). On the other hand, a simulant without the test sample was evaporated and blank weight was recorded (W2). The OML of the film samples was determined by using eqn (6). | 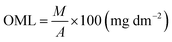 | (6) |
where M is the (W1 − W2) residual weight (mg) and A is the area of the film (cm2) used.
2.3.8. Antimicrobial activity.
To investigate the antimicrobial efficacy of the developed bio-films, widespread foodborne microbes such as Gram-negative Escherichia coli, fungi yeast Aspergillus niger and Gram-positive Staphylococcus aureus were selected. For this study, the broth culture of test bacteria and fungi were prepared aseptically by using nutrient broth and potato dextrose broth. Thereafter, 100 μL of each inoculated test bacteria and fungi of 0.5 McFarland standards were dispensed on the nutrient agar and potato dextrose agar plates, respectively, and dried under sterile laminar air flow. Then, the test film samples were directly placed on the agar and potato dextrose plates, followed by incubation for 24 h at 37 °C and 30 °C for bacterial and fungal activities, respectively. The antimicrobial efficacy of the test samples was recorded by measuring the inhibition area beneath or surrounding them.
2.3.9. DPPH free-radical assay.
The DDPH free-radical assay was used to determine the anti-oxidant efficacy of the developed bio-films. For the test, 2 mL of methanolic solution of DPPH (1 M) and 1 mL of film solution (20–100 μg mL−1) were mixed, followed by 10 times dilution using distilled water and a violet colored reaction mixture was vortexed for 5 min and kept in a dark place at room temperature for 40 min. Thereafter, the yellow colored test specimen was exposed to UV absorbance at 517 nm. The same procedure was used for commercial ascorbic acid and blank solution. The three tests were performed for each sample and the results were mentioned as mean and standard deviation. The DPPH assay (%) was determined by using eqn (7),31 and the IC50 value is calculated from the slope (y = mx + c) obtained from linear regression of the concentration of test sample v/s DPPH assay (%). |  | (7) |
2.3.10. Green chilli preservative efficacy.
The developed composite films were tested for the preservation of green chillies stored at room temperature. Based on the above functional properties, the film sample PGS15% was selected for the packaging test. However, unpacked and pristine CS/PL packed chillies were used for comparison. The green chillies were packed in 8 × 4 cm2 film pouches and placed at room temperature. The changes in the color of the chillies were captured every two days using a digital camera. Further, the physiological weight loss (PWL) of the unpacked and packed chilli was noted every two days and the percentage of PWL was determined using eqn (8). |  | (8) |
2.3.11. Statistical analysis.
One-way ANOVA was used in the statistical analysis, which was conducted by using Origin-9 software (Origin, USA). The average standard deviation of the test results from three replicates was shown (at P ≤ 0.05), and the statistical level for Tukey's test was P ≤ 0.05.
3 Results and discussion
3.1. Attenuated total reflection-FT-IR analysis
Fig. 2 depicts the FT-IR spectra of prepared nanocomposite films. The CS exhibited a broad absorption band at 3349 and 3274 cm−1 due to the hydroxyl and N–H stretching, respectively, while the band appearing at 2922 cm−1 was corresponding to C–H stretching. The C
O stretching of amide-I and amide-II was observed at 1530–1640 cm−1 and the band appearing at 1015 cm−1 was due to the stretching vibration of C–O–C.31 Similarly, the CS/PL film showed a shift in the position of hydroxyl frequency to the higher wavelength, which confirms the good compatibility between the CS and PL. GA exhibited strong and intense absorption bands around 3260–3490 cm−1 and 1702 cm−1, pertaining to the O–H and carbonyl (C
O) stretching, respectively, whereas the absorption bands at 1618, 1533, and 1443 cm−1 are related to the characteristic stretching vibrations of C–C bonds in the aromatic ring of GA.32 On the other hand, on incorporation of GA into the CS/PL film, it is observed that the band positions of hydroxyl stretch and amide stretch at 3346–3266 cm−1 and characteristic bands of C
O stretching around 1659–1545 cm−1 were shifted to the lower wavelength with a rise in the concentration of GA in the film matrices, indicating the existence of intermolecular H–bonding between the film components. Moreover, the lack of several GA bands in the CPG bio-films around 1002–1400 cm−1 was due to the dominant character of the macromolecules.
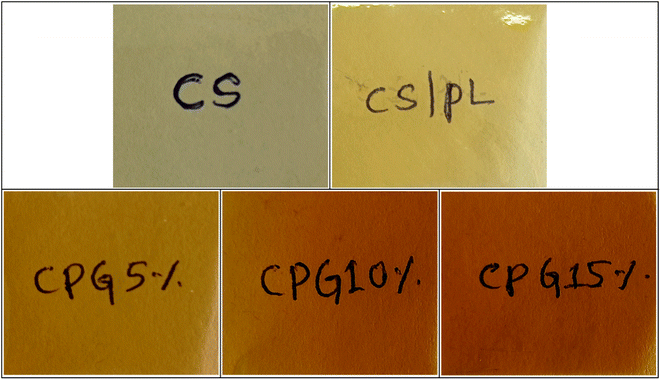 |
| Fig. 1 Digital appearance of the prepared bio-films. | |
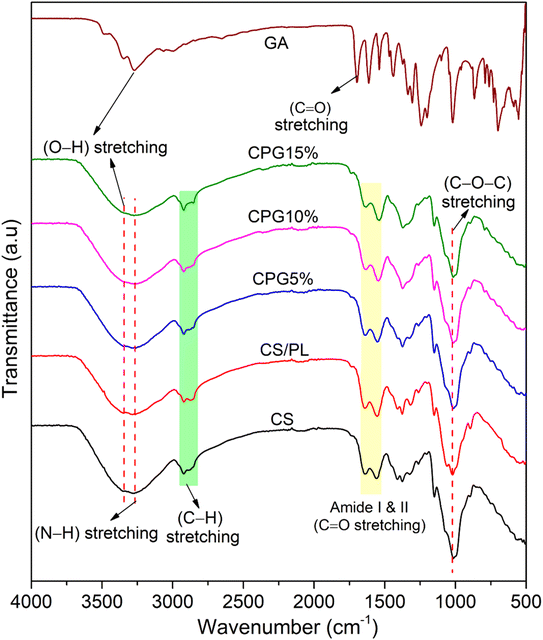 |
| Fig. 2 FT-IR spectra of the prepared bio-films. | |
3.2. Optical properties
Optical parameters such as film transparency and opacity are the key parameters for food packaging materials. The packaging film should have a negligible UV transmittance value to reduce the oxidation process of the packed food products. Fig. 3 depicts the optical properties of the prepared CPG bio-films. The neat CS and CS/PL films have shown lower UV absorption capacity, whereas the UV absorption capacity of CPG bio-films significantly improved with the addition of GA (Fig. 3a). Furthermore, it was observed from Fig. 3b that the GA incorporated CPG bio-films remarkably blocked the passage of UV rays around 300–380 nm and an increase in the concentration of GA in the film matrices significantly reduced the film transparency (Fig. 3d). Nevertheless, the incorporation of GA had a great effect on the film opacity (Fig. 3c). However, the increase in the film opacity is due to the presence of GA, which turns the pale yellow colored CS/PL film into a brown color (Fig. 1), which causes higher opacity. Additionally, the increase in the UV ray blocking capacity and decrease in the transparency of CPG bio-films are mainly due to reduction in the polymer inter-chain spacing with GA content and a dense polymeric network formed between the CS, PL and GA, which reduces the amount of light that passes through the bio-films.33 It is reported that the presence of phenolic –OH in the plant phytochemicals like ethyl vanillin and GA molecule in the polymer matrices have undergone a n–π* electronic transition at 200–300 nm that led to an enhancement in the UV absorption.34,35 Hence this suggested that the prepared CPG bio-films could be used as excellent UV ray barriers.
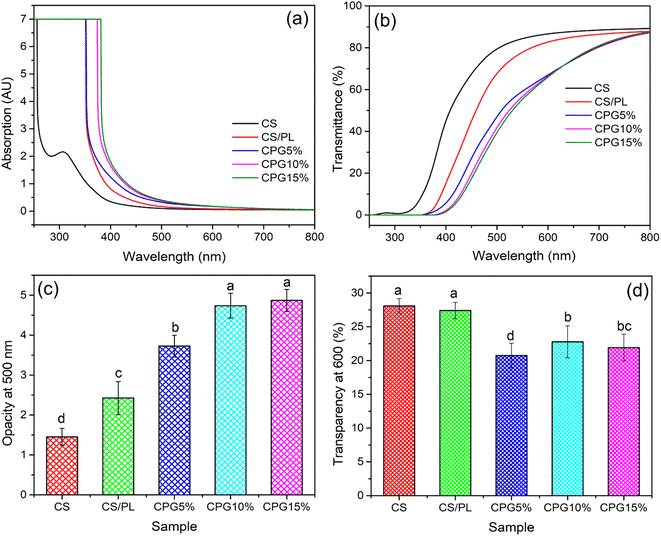 |
| Fig. 3 (a) UV-Vis spectra, (b) UV-Vis transmittance, (c) opacity and (d) transparency of the prepared bio-films (a–dsignificantly different at P ≤ 0.05). | |
3.3. Mechanical properties
Any material used for food packaging purposes should possess high mechanical attributes to protect the packed food product during storage and transport. It is believed that good tensile strength is necessary for plastic films particularly when they are implemented in the food industry. Fig. 4 shows the stress–strain curves of the developed bio-films. The corresponding thickness-dependent mechanical parameters such as tensile strength (TS), elongation at break (EB) and elastic modulus (Y) are shown in Table 1. The average thicknesses of all prepared bio-films have not been significantly affected by the GA content. Besides thickness, the neat CS film had a TS value of 0.063 GPa, while this TS was significantly reduced to 0.05 GPa with an increase in the EB value of 5.73% when it was blended with PL, indicating the hydrophilic and plasticizing effect of PL. The Y of the bio-films is considerably affected by the GA content in the film matrices. Film samples with lower GA have shown a higher Y value, suggesting the highly stiff character of the CPG5% bio-film. Further, it is observed that the Y value of bio-films tends to decrease slightly with an increase in the GA content. It is reported that the lower concentration of additive yields a higher Y value and it was reduced with the higher concentration due to a reduction in the plasticizing property.36
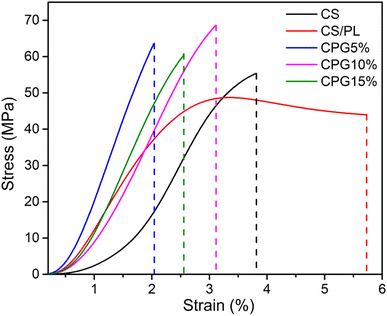 |
| Fig. 4 Stress–strain curves of the prepared bio-films. | |
Table 1 Thickness, tensile properties, WVTR and OP of the prepared bio-filmsa
Sample |
Thickness (mm) |
TS (Gpa) |
EB (%) |
Y (Gpa) |
WVTR (g m−2 h−1) |
OP × 10−5 (cm3 per m per day per atm) |
a–eDifferent letters show the significantly different means of obtained data (P ≤ 0.05), according to the Tukey's test.
|
CS |
0.087 ± 0.001a |
0.063 ± 0.002c |
4.18 ± 0.41b |
0.69 ± 0.01e |
22.29 ± 2.17b |
4.1 ± 0.76bc |
CS/PL |
0.082 ± 0.002a |
0.050 ± 0.004e |
5.73 ± 0.27a |
0.85 ± 0.03d |
24.23 ± 1.74a |
4.8 ± 1.19a |
CPG5% |
0.075 ± 0.000a |
0.064 ± 0.002b |
2.05 ± 0.63de |
2.28 ± 0.01a |
21.75 ± 1.81c |
4.2 ± 0.87b |
CPG10% |
0.079 ± 0.001a |
0.069 ± 0.001a |
3.12 ± 0.49c |
1.41 ± 0.04bc |
19.02 ± 2.61d |
3.3 ± 0.82d |
CPG15% |
0.083 ± 0.001a |
0.061 ± 0.002cd |
2.55 ± 0.17d |
1.70 ± 0.03b |
19.04 ± 1.17d |
2.7 ± 1.94e |
However, the addition of GA to the CS/PL resulted in both an increase in TS (0.069 GPa for 10 wt% GA) and a fall in EB value. The reduction in the free space between the polymer chains that restricted the flexibility of the film is what causes the TS value to rise with increasing GA concentration. However, with a further increase in the concentration of GA in the film matrices, the TS was dropped to 0.061 Gpa. This reduction in the TS of the bio-films was due to the agglomeration of GA particles and irregular microstructural arrangement of film components evidenced by SEM images (Fig. 5), which most likely contributed to a decline in the polymer matrix cohesiveness and as a result, in the tensile strength.37 As phase separation between CS/PL polymers increased as GA was added to the matrix, it may have also reduced the intermolecular forces between CS/PL molecules, lowering the mechanical resistance of the films.
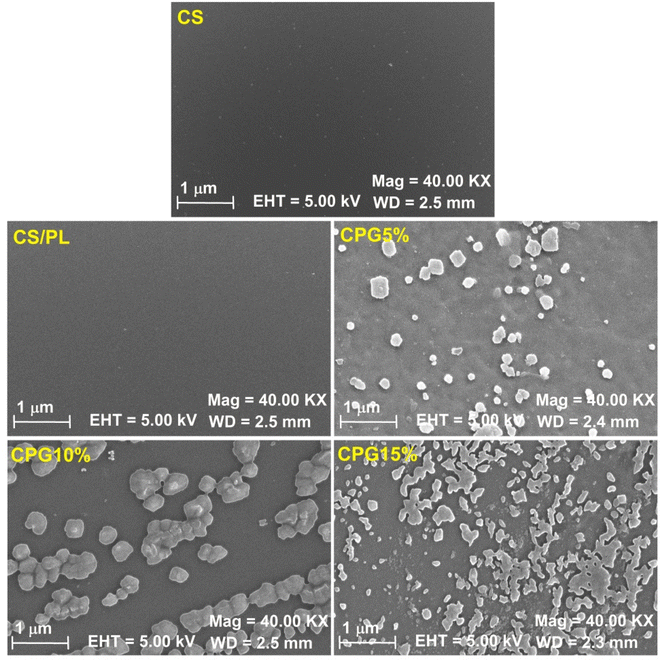 |
| Fig. 5 SEM images of the prepared bio-films. | |
3.4. Surface morphology
The microstructural arrangements of the prepared bio-films have been recorded using a scanning electron microscope (SEM) and are shown in Fig. 5. The pristine CS and CS/PL films have a smooth, crack- and bubble-free surface, confirming their higher miscibility. However, after the addition of GA, all the CPG bio-films exhibited a crack-free morphology with some noticeable changes. At lower concentration (5 wt%), some agglomerated GA particles were observed, whereas an increase in the surface roughness and irregular morphology was observed with a rise in the proportion of GA in the CS/PL film matrices. The increase in the covalent and non-covalent interaction between the CS/PL matrices and the additive was what caused the increase in the GA agglomerates and surface roughness.38 This irregular morphology and surface roughness of the CPG bio-films at higher GA content led to the phase separation that resulted in reducing the tensile resistance of the films. Analogous outcomes were seen with the GA-induced PVA films.4
3.5. Moisture retention capacity (MRC) and surface wettability (WCA)
Moisture retention capacity plays an important role in the preservation of packaged food. Before any material is used for food packaging purposes, it must have high moisture retention capacity to retain the original flavor and quality of the packaged food. The MRC of the prepared CPG bio-films is shown in Fig. 6a. As depicted by Fig. 6a, the CS had around 95.5% MRC value, indicating the hydrophobic nature of the film, while the MRC value decreased to 90.9% when it was blended with PL. Reduction of MRC value with the addition of PL was due to the relatively hydrophilic property of the PL. The MRC values of CPG5%, CPG10% and CPG15% were found to be 87.85, 89.2 and 90.21%, respectively. Further, MRC values begin to decrease but not significantly with the addition of GA. This slight reduction in the MRC is due to the slight hygroscopic nature of the GA. Overall, the prepared CPG bio-films have shown good moisture barrier capacity over entire formulations.
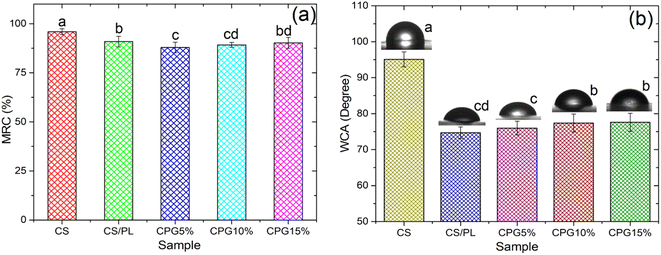 |
| Fig. 6 MRC (a) and WCA (b) of the prepared bio-films (a–dsignificantly different at P ≤ 0.05). | |
The surface wettability is one of the important parameters for the material used for food packaging applications. The surface wettability is assessed by means of water contact angle (WCA). According to Gontard et al. (1993), WCA > 65° and WCA < 65° are considered as hydrophobic and hydrophilic surfaces.39 The WCA of the developed CPG bio-films is presented in Fig. 6b. The neat CS films had a WCA of 95.1°, which agrees well with the earlier report.31 However, the WCA of the CS was significantly reduced to 74.7° when blending it with PL. This remarkable reduction in the WCA was associated with the hydrophilic nature of the PL. The WCA of CPG5%, CPG10% and CPG15% was found to be 76°, 77.4° and 77.6°, respectively. This implies that the addition of GA into the CS/PL matrices did not significantly affect the surface wettability. Overall, all the prepared CPG bio-films have acquired a hydrophobic surface, which is a key factor for food packaging films.
3.6. Water vapor transmission rate and oxygen permeability
Materials that come into contact with food must effectively control the moisture to keep the food product fresh and prevent the growth of mould and mildew. Hence, WVTR is an essential variable in the wet and dry food industry. It is known that food packaging films should have minimum WVTR values. The WVTR of the CPG bio-films is mentioned in Table 1. The neat CS and CS/PL films have a WVTR of 22.29 and 24.23 g m−2 h−1, respectively, which are found to be lower than the previously reported WVTR value of 24.6 and 52.73 g m−2 h−1 for the CS film. It appears that the lower WVTR may be due to the high molecular weight chitosan used for the film preparation. Nakayama et al. (2020) reported that the film made of CS with a high molecular weight has the lowest water vapor permeability and therefore the decreased WVTR.40 The GA functionalized CPG bio-films exhibited a WVTR of 21.75, 19.02 and 19.04 g m−2 h−1 for CPG5%, CPG10% and CPG15%, respectively. The decreased WVTR trend was observed with the increase in GA content within the CS/PL matrices. Due to the presence of a significant amount of GA crystals associated with the more compact chain segments and the strong coupling with the amorphous regions of the polymers, which restrict the passage of the adsorbed water vapor, the WVTR was reduced.41 Overall, the prepared bio-films have shown a superior WVTR to the earlier reported GA induced bio-films.38
One of the most frequently investigated transport characteristics of food packing films is oxygen permeability (OP). Fresh product preservation is significantly impacted by the OP of the plastics used for food storage and transport. The OP of the developed bio-films is shown in Table 1. The neat CS film exhibited 4.1 × 10−5 cm3 per m per day per atm, while 4.8 × 10−5 cm3 per m per day per atm was observed for the CS/PL film. This slight increase in the OP value might be due to the hydrophilicity of the PL. Like WVTR, the OP value of the CPG bio-films gradually decreases with an increase in the GA content. A noticeable decrease of about 43.75% in the OP value was observed with the CS/PL film matrices containing 15 wt% GA. This remarkable improvement in the OP value was due to the accumulation of GA particles at higher concentration that led to the minimization of the free space between the polymeric chains that creates a tortuous path and compact microstructure observed in SEM images, resulting in lower oxygen permeation. It is well known that the oxygen permeation is reduced with the increase in the concentration of additives due to the intermolecular interaction and the irregular arrangement of the film components led to a strong closely packed diffusive path for the gas molecules.42 The lower OP value (high oxygen barrier capacity) means that the prepared films have the ability to prolong shelf-life, and avoid quality, flavor, and fragrance deterioration of the food product.
3.7. Overall migration limit
It is necessary to investigate the total quantity of film components released into food simulants before commercializing them because excess release of components may lead to serious effects on human health. According to the European permissible limits for migration, the food packaging material must have a permitted migration limit of 60 mg kg−1 or 10 mg dm−2.43 In this context, the developed CPG bio-films were exposed to three food simulants, which are distilled water, ethanol, and acetic acid representing aqueous, alcohol beverages and acidic foods. The OLM values of developed bio-films are given in Table 2. It is seen from the data that after 10 days of observation, the pure CS and CS/PL exposed to water and ethanol showed the lower migration rate compared to the 3% acetic acid solution. However, the increase in the OML value against 3% acetic acid was associated with the relatively high solubility of the CS polymer matrix, allowing the diffusion of the active components to the acidic food simulant. There is a notable improvement in the migration rate that when PL is blended with CS it reduces the migration rate due to polymer interactions, whereas it further reduces with the addition of GA. The observed reduction in the migration rate indicating the accumulation of GA particles and within the CS/PL matrices held via strong intermolecular interactions further evidenced by FT-IR spectroscopy. These molecular interactions cause a compact and random structural packing network that restricts the diffusion of GA into the simulant.26 On the other hand, the OML value gradually increases with the addition of GA and it reaches a maximum OML of 0.724, 0.971, and 1.487 mg dm−2 for water, 50% ethanol and 3% acetic acid, respectively. Further, the OML value of the CPG film sample in 50% ethanol is comparatively higher than that of the water simulant. This increase in the migration rate was attributed to the quick solubility of GA in ethanol compared to water. Similar results have been observed when gallic acid is incorporated into PVA/tragacanth gum film matrices.38 Overall, the prepared CPG bio-films exhibited OLM values that were within the permitted limit, indicating the potential of these films for application in the food packaging industry.
Table 2 Overall migration limit of prepared CPG bio-filmsa
Sample |
OML at 40 °C for 10 days (mg dm−2) |
Distilled water |
50% ethanol |
3% acetic acid |
a–dDifferent letters show the significantly different means of obtained data (P ≤ 0.05), according to the Tukey's test.
|
CS |
0.414 ± 0.002c |
0.371 ± 0.001d |
1.831 ± 0.009a |
CS/PL |
0.483 ± 0.012c |
0.338 ± 0.002d |
1.291 ± 0.001c |
CPG5% |
0.541 ± 0.003b |
0.572 ± 0.005c |
1.272 ± 0.050c |
CPG10% |
0.574 ± 0.017b |
0.773 ± 0.013b |
1.211 ± 0.001c |
CPG15% |
0.724 ± 0.020a |
0.971 ± 0.008a |
1.487 ± 0.007b |
3.8. Antimicrobial efficacy
In order to safeguard the packed food product from surrounding and foodborne pathogens, it is necessary for packaging films to have antimicrobial properties. The antimicrobial efficacy of the prepared bio-films in terms of inhibition zone against S. aureus, E. coli and yeast fungi A. niger is presented in Fig. 7. The pure CS and CS/PL bio-films showed inherent inhibition capacity against all three pathogens. The antimicrobial activity was mainly due to the microbial inhibition capacity of the CS and PL biopolymers.44,45 It is worth noting that the plant originated active ingredient has the ability to inhibit different bacteria and fungi.46 Hence, the antimicrobial efficacy of CS/PL significantly improved with GA. It was observed that a significant increase in the inhibition zone was observed with the GA content. This increment in the inhibition efficiency was mainly due to the bio-active property of the GA. The CPG bio-films exhibited the highest inhibition zone for S. aureus and A. niger followed by E. coli. This variation in the activity was brought about by the difference in the thickness of the cell wall of tested microorganisms. As seen in Fig. 7, there is no microbial growth observed below the test sample and its surroundings, which means that the CPG bio-films exhibited both bactericidal and bacteriostatic effects against all the tested fungi and bacteria, which indicates that they not only prevent the growth of pathogens, but also damage their DNA by cell membrane rupture, which leads to pathogen death. The accurate mechanism of the increased antimicrobial activity of CPG bio-films is the release of reacting oxygen species (ROS) from the GA moiety that induced irreversible modifications in the cell membranes properties which caused subsequent loss of intracellular components necessary for infections to remain alive.47 Overall, the film containing 15 wt% GA showed superior antibacterial efficacy against all three tested pathogens. A similar inhibition trend was observed for an earlier reported work on plant polyphenol rutin induced CS based films.3
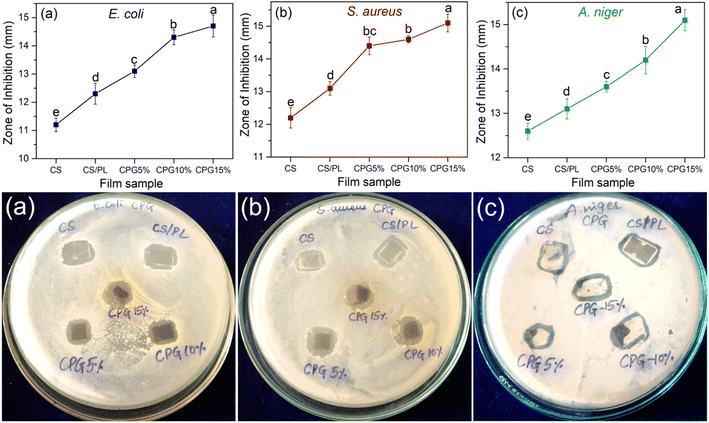 |
| Fig. 7 Antimicrobial activity and the corresponding inhibition zones of the prepared bio-films against E. coli (a), S. aureus (b) and A. niger (c) (a–dsignificantly different at P ≤ 0.05). | |
3.9. Antioxidant study
The packed food products undergo rapid deterioration due to the oxidation process of proteins and lipids present in the food stuff. Hence, it is very necessary to delay the oxidation process by stabilizing the reactive free radicals with active packaging systems. Fig. 8 represents the antioxidant profile of the prepared CPG bio-films. The pristine CS and CS/PL films had lower antioxidant capacity. However, GA induced CPG films showed a significant increment in the free-radical DDPH scavenging assay. The result showed that the antioxidant efficiency is dose dependent; as the content of GA increases in the film matrices, the free-radical scavenging activity also increases. The film samples CPG10% and CPG15% showed excellent antioxidant capacity even at low concentration (80 and 100 μg mL−1). Nevertheless, the half-maximal inhibitory concentrations (IC50) of CS and CS/PL films were found to be 40.1 and 33.87 μg mL−1, indicating good antioxidant activity. Further, the IC50 value was significantly reduced with the increase in the GA concentration. The film sample containing 15 wt% of GA showed an excellent antioxidant property (20.04 μg mL−1) compared to the commercial anti-oxidizing agent ascorbic acid (25.74 μg mL−1). This ultimate increment in the antioxidant activity was due to the cumulative impact of natural antioxidants CS, PL and GA. Moreover, the phenolic ring of GA containing three hydroxyl groups and one carboxylic group gets activated when it comes into contact with polar media, which facilitates H-atom transfer and electron-donating-based radical scavenging48 and hence, an increase in the antioxidant activity. According to Balasubramaniam et al. (2021), a DPPH free-radical scavenging assay found more than 90% scavenging activity when GA was grafted over a K-carrageenan/cellulose blend matrix.49 Also, 95% scavenging activity was achieved with a GA-Inulin composition.50 Hence, the result suggests that these films behave as strong antioxidant materials.
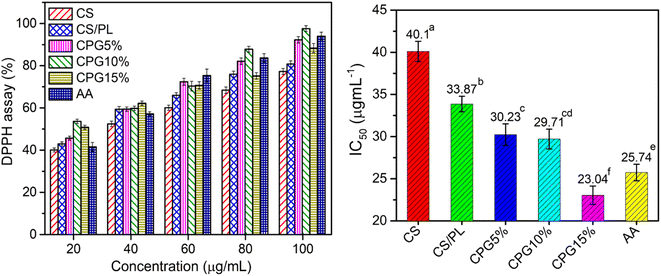 |
| Fig. 8 Antioxidant profile of the prepared bio-films (AA – ascorbic acid) (a–esignificantly different at P ≤ 0.05). | |
3.9. Green chilli preservative efficacy
The developed CPG bio-films were practically used to preserve green chillies in order to test the effectiveness of the packaging. Based on the properties discussed above, the CPG15% film was selected for the packaging test. The CS/PL packed and unpacked test was performed for comparison. Green chillies are a living, fresh product that undergo a continual metabolic processes and respiration. The ripening process may be easily seen through changes in color. Additionally, the moisture loss of chillies causes their surface to wrinkle as a result of the cells' loss of turgidity.20 The morphological texture changes in unpacked, CS/PL and CPG15% packed chillies and changes in their physiological weight loss (PWL%) during storage at room temperature are shown in Fig. 9 and 10, respectively. Initially, all three sets of chillies were green in color and showed a glassy appearance. The unpacked chillies turned yellow on the 6th day of storage with a PWL of 5.26%, whereas CS and CS/PL packed chillies remained green with a minimum PWL of 3.71 and 2.66%. Further increasing the storage time, on the 10th day the unpacked chilli completely turned red with a PWL of 8.14% due to a rapid oxidation process, while the CS/PL packed chilli turned slightly red in the middle portion with a PWL of 6.72%; however, the chilli packed with CPG15% remained green with a minimum PWL of 5.96%. Nevertheless, the unpacked chilli lost its maximum moisture content and an excess wrinkle texture was observed with an extreme PWL of 17.17%. The color of the CS/PL packed chilli completely changed to red with a PWL of 9.18%, but the CPG15% packed chilli retained its green color and glassy texture with a PWL of 8.27% on the 18th day. This reduction in the PWL of CPG15% was due to higher moisture retention capacity. Further, results obtained from PWL are in concurred with the WVTR and OP results which showed excellent barrier efficiency that preventing the packed food product from losing its moisture. It is reported that the incorporation of citric acid to the chitosan matrix could enhance the shelf-life of chillies up to seven days.20 Mondal et al. (2022) showed that the incorporation of green algae extract induced chitosan based films to have a prolonged shelf-life up to 10 days with 3–10% physiological weight loss.51 Based on the above discussion, the prepared CPG15% film has the ability to extend the shelf-life and preserve the green chillies up to 18 days. Further, Table 3 compares the current study with the prior film formulations for packaging applications.
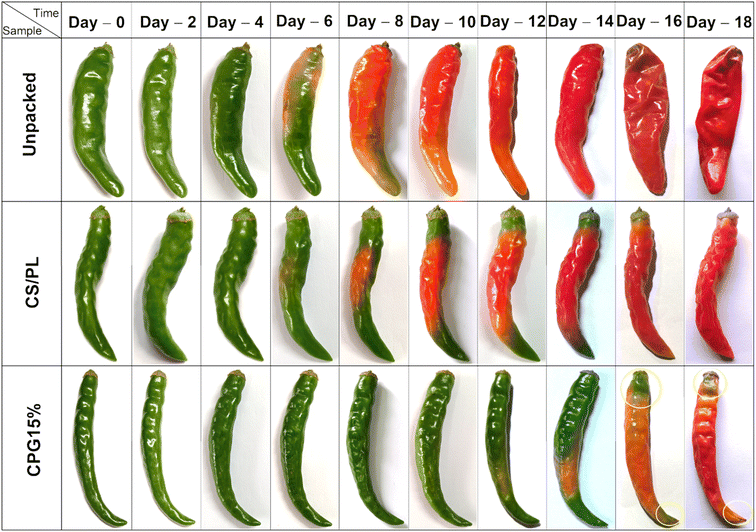 |
| Fig. 9 Change in color of the green chillies during storage. | |
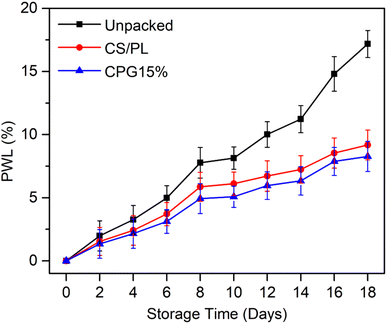 |
| Fig. 10 Physiological weight loss of the packed green chillies during storage. | |
Table 3 Comparison of some recent formulations used for shelf-life extension of fresh products with the present studya
Formulation |
Packaging application |
Shelf-life extension |
EDTA – ethylenediaminetetraacetic acid; CPG – CS/PL/GA.
|
CS/citric acid/glycerol |
Green chilly |
07 days (ref. 20) |
Starch/sodium alginate/EDTA |
Green chilly |
12 days (ref. 52) |
Zein/thyme essential oil |
Strawberry |
15 days (ref. 53) |
CS/crude algae extract |
Green chilly |
10 days (ref. 51) |
CPG |
Green chilly |
18 daysPresent study |
4 Conclusions
The current research work concentrated on the creation of innovative chitosan/pullulan active bio-films functionalized by gallic acid as a green and sustainable packaging material for preserving fresh green foods. The developed CPG bio-films were characterized by different instrumentation techniques. The existence of secondary molecular interactions between the CS, PL and GA was confirmed by ATR-F-IR spectroscopy. The incorporation of GA caused a rough film surface shown by SEM analysis. The UV light barrier, tensile properties and moisture retention capacity were greatly improved and the film transparency reduced with the addition of GA due to the intermolecular interaction between the CS/PL film matrices and GA. Further, the WVTR and OP of the CPG films were significantly enhanced, while surface wettability was not improved. In addition, CPG bio-films exhibited strong antimicrobial efficiency against three foodborne microbes such as S. aureus and A. niger followed by E. coli. Moreover, the film samples containing 10 and 15 wt% of GA in the film matrices showed excellent DPPH free-radical scavenging activity and a lower IC50 value than the standard anti-oxidizing agent ascorbic acid. Nevertheless, the developed CPG15% film has been practically tested for the preservation and shelf-life extension of green chillies during storage at room temperature. The results showed that the CPG15% bio-film interestingly extended the shelf life of the green chillies up to 18 days. Based on all the above objectives the CPG films containing 10 and 15 wt% GA could be considered as green active bio-films for the preservation and shelf-life extension of fresh vegetables and fruits.
Data availability
The data that support the findings of this study are available from the corresponding author upon reasonable request.
Author contributions
TG: conceptualization; investigation; data curation; formal analysis; methodology; resources; software; writing – original draft; writing – review & editing. SD: resources; investigation; methodology; writing – review & editing SPM: validation; investigation; review & editing. RBC: investigation; validation; visualization; review & editing.
Conflicts of interest
The authors declare that there is no conflict of interest.
Acknowledgements
Funding organizations from the governmental, private, or nonprofit sectors did not provide this research with any specific grants. Authors sincerely acknowledge University Scientific Instrumentation Centre (USIC) and SAIF, Karnatak University, Dharwad, for providing necessary facilities. Authors also acknowledge DST PURSE Phase-II Program (Grant No SR/PURSE PHASE 2/13(G)), for providing FT-IR and water contact angle facilities.
References
- A. N. Adilah, B. Jamilah, M. A. Noranizan and Z. A. N. Hanani, Utilization of mango peel extracts on the biodegradable films for active packaging, Food Packag. Shelf Life, 2018, 16, 1–7 CrossRef
.
- S. S. Narasagoudr, V. G. Hegde, V. N. Vanjeri, R. B. Chougale and S. P. Masti, Influence of Boswellic acid on physical, structural and morphological properties of poly (vinyl alcohol) films, Chem. Data Collect., 2020, 27, 100370 CrossRef CAS
.
- S. S. Narasagoudr, V. G. Hegde, R. B. Chougale, S. P. Masti, S. Vootla and R. B. Malabadi, Physico-chemical and functional properties of rutin induced chitosan/poly (vinyl alcohol) bioactive films for food packaging applications, Food Hydrocolloids, 2020, 106096 CrossRef CAS
.
- F. Luzi, E. Pannucci, L. Santi, J. M. Kenny, L. Torre, R. Bernini and D. Puglia, Gallic Acid and Quercetin as Intelligent and Active Ingredients in Poly(vinyl alcohol) Films for Food Packaging, Polymers, 2019, 11, 1999 CrossRef CAS PubMed
.
- L. Wen, Y. Liang, Z. Lin, D. Xie, Z. Zheng, C. Xu and B. Lin, Design of multifunctional food packaging films based on carboxymethyl chitosan/polyvinyl alcohol crosslinked network by using citric acid as crosslinker, Polymer, 2021, 230, 124048 CrossRef CAS
.
- B. Badhani, N. Sharma and R. Kakkar, Gallic acid: a versatile antioxidant with promising therapeutic and industrial applications, RSC Adv., 2015, 5, 27540–27557 RSC
.
- K. K. Sohi, N. Mittal, M. K. Hundal and K. L. Khanduja, Gallic Acid, an Antioxidant, Exhibits Antiapoptotic Potential in Normal Human Lymphocytes: A Bcl-2 Independent Mechanism, J. Nutr. Sci. Vitaminol., 2003, 49, 221–227 CrossRef CAS PubMed
.
- G.-C. Yen, P.-D. Duh and H.-L. Tsai, Antioxidant and pro-oxidant properties of ascorbic acid and gallic acid, Food Chem., 2002, 79, 307–313 CrossRef CAS
.
- T. Gasti, S. Dixit, S. P. Sataraddi, V. D. Hiremani, S. P. Masti, R. B. Chougale and R. B. Malabadi, Physicochemical and Biological Evaluation of Different Extracts of Edible Solanum nigrum L. Leaves Incorporated Chitosan/Poly (Vinyl Alcohol) Composite Films, J. Polym. Environ., 2020, 28, 2918–2930 CrossRef CAS
.
- E. Díaz-Montes and R. Castro-Muñoz, Edible Films and Coatings as Food-Quality Preservers: An Overview, Foods, 2021, 10, 249 CrossRef PubMed
.
- S. Kumar, A. Mukherjee and J. Dutta, Chitosan based nanocomposite films and coatings: Emerging antimicrobial food packaging alternatives, Trends Food Sci. Technol., 2020, 97, 196–209 CrossRef CAS
.
- C. J. Pérez-Guzmán and R. Castro-Muñoz, A Review of Zein as a Potential Biopolymer for Tissue Engineering and Nanotechnological Applications, Processes, 2020, 8, 1376 CrossRef
.
- M. A. Hassan, A. M. Omer, E. Abbas, W. M. A. Baset and T. M. Tamer, Preparation, physicochemical characterization and antimicrobial activities of novel two phenolic chitosan Schiff base derivatives, Sci. Rep., 2018, 8, 1–14 CAS
.
- T. Gasti, S. Dixit, V. D. Hiremani, R. B. Chougale, S. P. Masti, S. K. Vootla and B. S. Mudigoudra, Chitosan/pullulan based films incorporated with clove essential oil loaded chitosan-ZnO hybrid nanoparticles for active food packaging, Carbohydr. Polym., 2022, 277, 118866 CrossRef CAS PubMed
.
- T. Gasti, V. D. Hiremani, S. P. Sataraddi, V. N. Vanjeri, N. Goudar, S. P. Masti, R. B. Chougale and R. B. Malabadi, UV screening, swelling and in-vitro cytotoxicity study of novel chitosan/poly (1-vinylpyrrolidone-co-vinyl acetate) blend films, Chem. Data Collect., 2021, 33, 100684 CrossRef CAS
.
- M. Hosseinnejad and S. M. Jafari, Evaluation of different factors affecting antimicrobial properties of chitosan, Int. J. Biol. Macromol., 2016, 85, 467–475 CrossRef CAS PubMed
.
- E. Díaz-Montes and R. Castro-Muñoz, Trends in Chitosan as a Primary Biopolymer for Functional Films and Coatings Manufacture for Food and Natural Products, Polymers, 2021, 13, 767 CrossRef PubMed
.
- K. C. Cheng, A. Demirci and J. M. Catchmark, Pullulan: Biosynthesis, production, and applications, Appl. Microbiol. Biotechnol., 2011, 92, 29–44 CrossRef CAS PubMed
.
- T. Ghosh, R. Priyadarshi, C. Krebs de Souza, B. L. Angioletti and J.-W. Rhim, Advances in pullulan utilization for sustainable applications in food packaging and preservation: a mini-review, Trends Food Sci. Technol., 2022, 125, 43–53 CrossRef CAS
.
- R. Priyadarshi, Sauraj, B. Kumar and Y. S. Negi, Chitosan film incorporated with citric acid and glycerol as an active packaging material for extension of green chilli shelf life, Carbohydr. Polym., 2018, 195, 329–338 CrossRef CAS PubMed
.
- G. M. Rodríguez, J. C. Sibaja, P. J. P. Espitia and C. G. Otoni, Antioxidant active packaging based on papaya edible films incorporated with Moringa oleifera and ascorbic acid for food preservation, Food Hydrocolloids, 2020, 103, 105630 CrossRef
.
- K. Masamba, Y. Li, J. Hategekimana, F. Liu, J. Ma and F. Zhong, Effect of Gallic acid on mechanical and water barrier properties of zein-oleic acid composite films, J. Food Sci. Technol., 2016, 53, 2227–2235 CrossRef CAS PubMed
.
- M. Khajavian, V. Vatanpour, R. Castro-Muñoz and G. Boczkaj, Chitin and derivative chitosan-based structures — preparation strategies aided by deep eutectic solvents: a review, Carbohydr. Polym., 2022, 275, 118702 CrossRef CAS PubMed
.
- R. Cabezas, E. Zurob, B. Gomez, G. Merlet, A. Plaza, C. Araya-Lopez, J. Romero, F. Olea, E. Quijada-Maldonado, L. Pino-Soto, T. Gonzalez and R. Castro-Muñoz, Challenges and Possibilities of Deep Eutectic Solvent-Based Membranes, Ind. Eng. Chem. Res., 2022, 61, 17397–17422 CrossRef CAS
.
- R. Castro-Muñoz, A. Msahel, F. Galiano, M. Serocki, J. Ryl, S. Ben Hamouda, A. Hafiane, G. Boczkaj and A. Figoli, Towards azeotropic MeOH-MTBE separation using pervaporation chitosan-based deep eutectic solvent membranes, Sep. Purif. Technol., 2022, 281, 119979 CrossRef
.
- I. Zarandona, A. I. Puertas, M. T. Dueñas, P. Guerrero and K. de la Caba, Assessment of active chitosan films incorporated with gallic acid, Food Hydrocolloids, 2020, 101, 105486 CrossRef CAS
.
- K. Limpisophon and G. Schleining, Use of Gallic Acid to Enhance the Antioxidant and Mechanical Properties of Active Fish Gelatin Film, J. Food Sci., 2017, 82, 80–89 CrossRef CAS PubMed
.
- X. Zhang, J. Liu, C. Qian, J. Kan and C. Jin, Effect of grafting method on the physical property and antioxidant potential of chitosan film functionalized with gallic acid, Food Hydrocolloids, 2019, 89, 1–10 CrossRef CAS
.
- E. Díaz-Montes, J. Yáñez-Fernández and R. Castro-Muñoz, Dextran/chitosan blend film fabrication for bio-packaging of mushrooms (Agaricus bisporus), J. Food Process. Preserv., 2021, 45, 1–12 Search PubMed
.
- B. Boonkaew, P. Suwanpreuksa, L. Cuttle, P. M. Barber and P. Supaphol, Hydrogels containing silver nanoparticles for burn wounds show antimicrobial activity without cytotoxicity, J. Appl. Polym. Sci., 2014, 131, 1–10 CrossRef
.
- T. Gasti, S. Dixit, O. J. D'souza, V. D. Hiremani, S. K. Vootla, S. P. Masti, R. B. Chougale and R. B. Malabadi, Smart biodegradable films based on chitosan/methylcellulose containing Phyllanthus reticulatus anthocyanin for monitoring the freshness of fish fillet, Int. J. Biol. Macromol., 2021, 187, 451–461 CrossRef CAS PubMed
.
- A. R. Khaskheli, S. Naz, F. Ozul, A. Aljabour, S. A. Mahesar, I. H. Patir and M. Ersoz, Urchin-like cobalt nanostructures for catalytic degradation of nitro anilines, Adv. Mater. Lett., 2016, 7, 748–753 CrossRef CAS
.
- J. Ghaderi, S. F. Hosseini, N. Keyvani and M. C. Gómez-Guillén, Polymer blending effects on the physicochemical and structural features of the chitosan/poly(vinyl alcohol)/fish gelatin ternary biodegradable films, Food Hydrocolloids, 2019, 95, 122–132 CrossRef CAS
.
- S. S. Narasagoudr, V. G. Hegde, V. N. Vanjeri, R. B. Chougale and S. P. Masti, Ethyl vanillin incorporated chitosan/poly(vinyl alcohol) active films for food packaging applications, Carbohydr. Polym., 2020, 236, 116049 CrossRef CAS PubMed
.
- D. Piñeros-Hernandez, C. Medina-Jaramillo, A. López-Córdoba and S. Goyanes, Edible cassava starch films carrying rosemary antioxidant extracts for potential use as active food packaging, Food Hydrocolloids, 2017, 63, 488–495 CrossRef
.
- N. S. Yousef, Statistical Study on Additives Used to Improve Mechanical Properties of Polypropylene, Polymers, 2022, 14, 179 CrossRef CAS PubMed
.
- R. B. Bodini, J. das, G. L. Guimarães, C. A. Monaco-Lourenço and R. Aparecida de Carvalho, Effect of starch and hydroxypropyl methylcellulose polymers on the properties of orally disintegrating films, J. Drug Deliv. Sci. Technol., 2019, 51, 403–410 CrossRef CAS
.
- N. Goudar, V. N. Vanjeri, S. Dixit, V. Hiremani, S. Sataraddi, T. Gasti, S. K. Vootla, S. P. Masti and R. B. Chougale, Evaluation of multifunctional properties of gallic acid crosslinked poly (vinyl alcohol)/Tragacanth Gum blend films for food packaging applications, Int. J. Biol. Macromol., 2020, 158, 139–149 CrossRef CAS PubMed
.
- N. Gontard, S. Guilbert and J.-L. Cuq, Water and Glycerol as Plasticizers Affect Mechanical and Water Vapor Barrier Properties of an Edible Wheat Gluten Film, J. Food Sci., 1993, 58, 206–211 CrossRef CAS
.
- R. I. Nakayama, K. Katsumata, Y. Niwa and N. Namiki, Dependence of water-permeable chitosan membranes on chitosan molecular weight and alkali treatment, Membranes, 2020, 10, 1–13 CrossRef PubMed
.
- V. Siracusa, Food Packaging Permeability Behaviour: A Report, Int. J. Polym. Sci., 2012, 2012, 1–11 CrossRef
.
- G. M. Russo, G. P. Simon and L. Incarnato, Correlation between rheological, mechanical, and barrier properties in new copolyamide-based nanocomposite films, Macromolecules, 2006, 39, 3855–3864 CrossRef CAS
.
- K. Grob, S. Pfenninger, W. Pohl, M. Laso, D. Imhof and K. Rieger, European legal limits for migration from food packaging materials: 1. Food should prevail over simulants; 2. More realistic conversion from concentrations to limits per surface area. PVC cling films in contact with cheese as an example, Food Control, 2007, 18, 201–210 CrossRef CAS
.
- C.-L. Ke, F.-S. Deng, C.-Y. Chuang and C.-H. Lin, Antimicrobial Actions and Applications of Chitosan, Polymers, 2021, 13, 904 CrossRef CAS PubMed
.
- H. Chang, J. Xu, L. A. Macqueen, Z. Aytac, M. M. Peters, J. F. Zimmerman, T. Xu, P. Demokritou and K. K. Parker, High-throughput coating with biodegradable antimicrobial pullulan fibres extends shelf life and reduces weight loss in an avocado model, Nat. Food, 2022, 3, 428–436 CrossRef CAS
.
- R. Barbieri, E. Coppo, A. Marchese, M. Daglia, E. Sobarzo-Sánchez, S. F. Nabavi and S. M. Nabavi, Phytochemicals for human disease: An update on plant-derived compounds antibacterial activity, Microbiol. Res., 2017, 196, 44–68 CrossRef CAS PubMed
.
-
K. Li, G. Guan, J. Zhu, H. Wu and Q. Sun, Antibacterial Activity and Mechanism of a Laccase-Catalyzed Chitosan–Gallic Acid Derivative against Escherichia coli and Staphylococcus aureus, Elsevier B.V., 2019, vol. 96 Search PubMed
.
- S. Alavi Rafiee, R. Farhoosh and A. Sharif, Antioxidant Activity of Gallic Acid as Affected by an Extra Carboxyl Group than Pyrogallol in Various Oxidative Environments, Eur. J. Lipid Sci. Technol., 2018, 120, 1–8 Search PubMed
.
- S. P. Lakshmi Balasubramaniam, A. Singh Patel and B. Nayak, Fabrication of antioxidative food packaging films using cellulose nanofibers, kappa-Carrageenan, and gallic acid, J. Food Process. Preserv., 2021, 45, 1–10 Search PubMed
.
- D. Arizmendi-Cotero, A. Villanueva-Carvajal, R. M. Gómez-Espinoza, O. Dublán-García and A. Dominguez-Lopez, Radical scavenging activity of an inulin-gallic acid graft and its prebiotic effect on Lactobacillus acidophilus in vitro growth, J. Funct. Foods, 2017, 29, 135–142 CrossRef CAS
.
- K. Mondal, S. K. Bhattacharjee, C. Mudenur, T. Ghosh, V. V. Goud and V. Katiyar, Development of antioxidant-rich edible active films and coatings incorporated with de-oiled ethanolic green algae extract: a candidate for prolonging the shelf life of fresh produce, RSC Adv., 2022, 12, 13295–13313 RSC
.
- K. Chitravathi, O. P. Chauhan and P. S. Raju, Postharvest shelf-life extension of green chillies (Capsicum annuum L.) using shellac-based edible surface coatings, Postharvest Biol. Technol., 2014, 92, 146–148 CrossRef CAS
.
- E. Ansarifar and F. Moradinezhad, Preservation of strawberry fruit quality via the use of active packaging with encapsulated thyme essential oil in zein nanofiber film, Int. J. Food Sci. Technol., 2021, 56, 4239–4247 CrossRef CAS
.
|
This journal is © The Royal Society of Chemistry 2023 |