Use of response surface methodology to optimise vacuum impregnation of β-carotene from Daucus carota in Pachyrhizus erosus
Received
28th October 2022
, Accepted 13th March 2023
First published on 27th March 2023
Abstract
Consuming carotenoid-rich foods prevent and reduce certain types of cancer and cardiovascular and degenerative diseases. In this work, response surface methodology (RSM) was used to maximise β-carotene (from Daucus carota juice) vacuum impregnation into Pachyrhizus erosus (PEC) cylinders (1 mm diameter by 2 mm length). The impregnation was carried out at 40 °C, an absolute pressure of 51 mm Hg, and a 10 g carrot juice/fresh product ratio. The factors considered were the immersion time (t = 20, 30, and 40 min) and the osmotic agent concentration (C = 20, 35, and 50 °Brix). The polynomial equations obtained to predict solute gain (SG), water loss (WL), total carotenoids (TCs), total soluble solids (TSSs), and water activity (aw) of the product had R2 > 0.846. Increasing the osmotic agent concentration increased SG, TC, and TSS values and decreased WL and aw values. From the obtained polynomial equations of the analysed responses, a quadratic effect was observed in the processing time due to the saturation of solutes on the product surface. The optimum conditions were t = 31 min and 50 °Brix, resulting in an impregnated PEC with a value of 269 μg of β-carotene/100 g dry base, 19.3 °Brix, and aw = 0.946. It was possible to incorporate carotenoids in PEC by optimising the vacuum impregnation conditions; however, a drying treatment is necessary to increase the shelf life of the obtained product.
1. Introduction
Pachyrhizus erosus is known as Mexican yam, Mexican turnip, yam bean, or “jicama”.1 It is rich in fibre, vitamins (C and B6), and minerals (K, Ca, and P, among others). However, due to its moisture content ranging from 80 to 85% (w.b.), it requires storage in a cool and dry place to avoid spoilage.1–3 Therefore, drying processes have been used to increase its shelf life. Some quality problems of dried jicama are browning (enzymatic browning), flavour (sweetness) losses, and nutritional value reduction (regarding vitamins, minerals, and antioxidant content).4
Food fortification has been a strategy to combat malnutrition due to a lack of micronutrients such as iron, zinc, and vitamin A,5 which are responsible for various ailments. These include physical deficiencies (congenital disabilities) or cognitive deficiencies (reduced or undeveloped cognitive ability and reduced productivity).5,6 In this context, food fortification is the addition of micronutrients to increase nutritional value. The term biofortification involves using micronutrients, such as vitamins or minerals, to improve nutritional value through agronomic practices, conventional plant breeding, or modern biotechnology.7 In this attempt, Kruger et al.8 mentioned that food-to-food addition could be a strategy to achieve this objective. In addition, food enrichment and fortification have been implemented as a drying pre-treatment to compensate for the nutrient loss or to incorporate new nutrients into foods.9,10
An important source of these compounds is the juice from fruits and vegetables, which has high content of these nutrients. In particular, orange carrots contain α and β-carotene,11 which have a β-ring and ε-ring at the beginning and end of a long chain of alternating double bonds. This chain is responsible for the orange, red, and yellow colours.12 The presence of β-carotene has been related to disease prevention caused by oxidative stress (some cancer types, ageing, vascular atheroma, etc.) because they are vitamin A precursors.13 Therefore, it is important to increase carotenoid content to improve nutritional value and human health.14
Impregnation with concentrated juices is currently used because it avoids adding refined sugar and increases bioactive compounds.15–17 However, this technique requires long impregnation times. Moreover, in some cases, the compounds do not penetrate the product centre, causing a barrier of compounds outside and avoiding the total water outflow.16,18 In contrast, vacuum impregnation has been proposed to introduce liquids into the porous matrix,19 improving nutritional characteristics and visual/sensory aspects20 inherent to juices, such as colour, acidity, astringency, and sweetness, among others.
So far, only reagent-grade carotenoids have been used for impregnation, such as diluted β-carotene in water13 or sugar solutions,21 that are incorporated into the food matrix using vacuum pressure. In another study, apples were dehydrated and subsequently immersed in a solution of β-carotene and water to stain the tissue and incorporate carotenoids with a coloured solution.22
The use of response surface methodology (RSM) as an optimisation tool has been widely adopted in the food industry to increase the efficiency of food processes. Factorial,23,24 Box–Behnken,16 and orthogonal25 experimental designs have been used to maximise the incorporation of sucrose solutions or bioactive compounds through the optimal combination of process conditions such as temperature, vacuum pressure or concentration of osmotic solution.16,20,26
This study aimed to evaluate the effect of vacuum pressure, processing time, and concentration of carrot juice concentrate on the quality of jicama impregnation using response surface methodology. The responses assessed were mass transfer parameters (water loss and solute gain), water activity (aw), total soluble solids (TSSs), colour, and total carotenoids (TCs). Also, the impregnation of carotenoids in jicama (P. erosus) was optimised.
2. Materials and methods
2.1. Materials
Fresh jicamas (P. erosus) (aw = 0.979 ± 0.005; 6.6 ± 0.3 °Brix, and pH = 6.30 ± 0.03) were purchased at a local market (San Andrés Cholula, Puebla, Mexico) and processed on the same day. The jicamas were selected for their uniform shape and size (200 g per sample). Then, they were washed, sanitised with a peracetic acid solution (by immersion in a 100 mg L−1 solution for 10 min), peeled, and cut into cylinders (1 mm diameter × 2 mm length) using a handmade sharpened metal tube. Each cylinder weighed 1.241 ± 0.007 g.
Osmotic solutions of 20, 30, 40 and 50 °Brix were prepared by diluting carrot juice concentrate (Daucus carota, 70.0 ± 0.2 °Brix and pH = 5.36 ± 0.07) with distilled water. It was generously donated by Kerr by Ingredion (Ingredion Mexico S.A. de C.V.).
2.2. Physicochemical analysis
Moisture was determined by drying the samples for 24 h at 105 °C in an oven to constant weight according to AOAC International16,27 method 950.466. Eqn (1) and (2) were considered to report the moisture fraction on a wet basis (w.b.) and dry basis (d.b.), respectively. Determinations of total soluble solids (TSS, in °Brix) of homogenised samples were performed, with a digital refractometer (Atago Co., Pocket PAL-RI, Tokyo, Japan).28 Water activity (aw) was determined at 25.0 ± 0.5 °C using a hygrometer (AquaLab, 4TEV, USA). |  | (1) |
|  | (2) |
where Mk is the moisture fraction of the sample k, k = 0 is the fresh sample, and k = VI is the impregnated sample.
Real porosity of fresh jicama (εr) was calculated with eqn (3), using apparent and real densities (in kg m−3).24 It was estimated in triplicate.
| 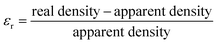 | (3) |
Apparent density was determined using Archimedes' principle, which gives the relationship between the weight of a jicama cylinder and the volume displacement of n-heptane when introducing the sample.29 Jicama cylinders were ground to puree, and the real density was measured with a pycnometer.24 Fresh and impregnated jicama cylinders were homogenised with a mortar and pestle for pH determination. The pH value of 10 g of homogenised samples and 10 mL of carrot juice solutions at 20.0 ± 1.0 °C was measured by direct immersion using a pH meter (Orion Research, 420A, IL, USA).
2.3. Impregnation treatment
Vacuum impregnation procedures were performed in a desiccator (150 mm internal diameter by 275 mm height) at 40 °C (to avoid the juice from boiling) and at 51 mm Hg of absolute pressure. The system's temperature was controlled using a thermos-regulated bath (Büchi, B-300 Base, Flawil, Switzerland). And the pressure was controlled with a vacuum pump (Büchi, V-300, Flawil, Switzerland). One jicama cylinder in an upright orientation (with its circular face perpendicular to the bottom of the system) was immersed into glass tubes (20 × 150 mm) with the osmotic solutions. A 10 g osmotic solution/g jicama ratio was considered to avoid excessive dilution of osmotic solution concentrations during the experiment. Also, this ratio was adequate to guarantee contact between the product and the osmotic solution. The treatment was performed at different immersion times (20, 30, and 40 min). This time corresponds to two periods: the first was the impregnation under vacuum (10, 20, and 30 min), and the second was the relaxation period (10 min) at atmospheric pressure (598 mm Hg).8 The jicama cylinders were removed from the osmotic solution, weighed, and blended for different determinations. One impregnated cylinder was used for each determination, and all the experiments were performed in triplicate.
2.4. Mass transfer properties
Water loss (WL) and solute gain (SG) were determined with eqn (4) and (5), respectively,10,17 | 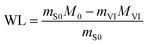 | (4) |
| 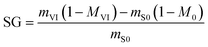 | (5) |
where WL = water loss (g water/g fresh product), SG = solute gain (g solutes/g fresh product), mS0 = fresh product weight (g), mVI = impregnated product weight (g), and M0 and MVI are the moisture fraction (wet basis) of fresh and impregnated products, respectively.
2.5. Colour characteristics
A colour-meter (Konica Minolta CR-400, Osaka, Japan) was used to measure the colour parameters (L*, a*, and b*) of impregnated jicama and juice. The measurement was performed in reflectance mode on the CIELab* scale, illuminating C, and with an observer angle of 2°. L*, a* and b* values were used to calculate the yellow index (YI), the total colour difference (ΔE), chroma (C*), and hue (Hue°) using eqn (6)–(9), respectively.30–32 | 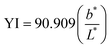 | (6) |
|  | (7) |
|  | (8) |
| 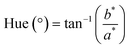 | (9) |
where L*, a*, and b* are the sample values.
,
and
are the colour parameters for fresh jicama or carrot juice.
2.6. Total carotenoids
The methodology reported by Desobry et al.33 with some modifications was used to determine total carotenoids.34 For carotene extraction, 2.50 ± 0.14 g of impregnated samples and 5 mL of acetone (99.5%) were homogenised with a mortar. The solution was then macerated in the dark for 10 min; the extracts were centrifuged for 10 min at 3900×g at 5 °C and filtered through Whatman filter paper No. 4. Solvent extractions were performed until colour exhaustion was reached with a total volume of 35 mL acetone. The absorbance of extraction was read at 450 nm using a UV-visible spectrophotometer (Shimadzu, UV-1900i, Tokyo, Japan). Total carotenoids were calculated according to Beer's law described in eqn (10) and expressed as mg β-carotene/100 g product d.b.where Abs is the absorbance at 450 nm, ε is the molar extinction coefficient of β-carotene in acetone (134 × 103 L mol−1 cm−1).
2.7. Rheological parameters
The apparent viscosity (in mPa s) of carrot juice at different concentrations (20–50 °Brix) and 40 °C was determined by the application of linearly increasing shear rate values using a Brookfield DV-III Ultra Programmable Rheometer (Brookfield Engineering Laboratories, Stoughton, M.A., USA) fitted with a No. 2 spindle. A volume of 10 mL of osmotic solution was added to the sample cup and maintained at 40 ± 0.2 °C with a recirculating water bath (PolyScience, ADO7R, USA). Shear rate values ranged from 2.4 to 40 s−1. The rheogram of fluid dynamic viscosity vs. the shear rate was fitted to the Ostwald de Waele power law model (viscosity = k[shear rate]n−1, where k represents the consistency index and is an analogue of the apparent viscosity (Pa sn) to analyse the fluid behaviour; if n = 1 the fluid was Newtonian, n > 1 dilatant fluid or n < 1 pseudoplastic.35
2.8. Statistical analysis
Response surface methodology (RSM) was applied to analyse the effect of immersion time (t) and osmotic agent concentration (C), since both variables modify the quality and mass transfer of foods impregnated with juice concentrate.8,36,37 Experimental conditions were set up according to a 3 × 4 factorial design. Independent variables were coded at each level according to eqn (11). Independent variables of the design (actual and coded) are listed in Table 1. A total of 12 experiments with their replicates were performed. Some parameters of impregnated jicama such as WL, SG, aw, TSSs (°Brix), colour parameters (L*, a*, b* and ΔE), and total carotenoids were analysed. | 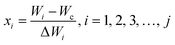 | (11) |
where Wi = the coded value of the independent variable, xi = the dimensionless value of Wi, Wc = Wi at the central point and ΔWi = step change of the i variable.
Table 1 Actual and coded values of the 3 × 4 factorial design for jicama impregnationa
Run |
Immersion time t (min) |
Carrot juice concentration C (°Brix) |
Numbers in parenthesis are coded values.
|
1 |
30 (0) |
20 (−1) |
2 |
30 (0) |
30 (−0.333) |
3 |
30 (0) |
40 (0.333) |
4 |
30 (0) |
50 (1) |
5 |
40 (1) |
20 (−1) |
6 |
40 (1) |
30 (−0.333) |
7 |
40 (1) |
40 (0.333) |
8 |
40 (1) |
50 (1) |
9 |
20 (−1) |
20 (−1) |
10 |
20 (−1) |
30 (−0.333) |
11 |
20 (−1) |
40 (0.333) |
12 |
20 (−1) |
50 (1) |
Eqn (12) was used to fit each experimental value with a second-order polynomial model. ANOVA was used to analyse the statistical significance of the models. A statistical test was performed with the Minitab v.18 Statistical Software (Minitab Inc., State College, PA, USA). The “backward elimination procedure” of non-significant terms was used for the model fitting. A p ≤ 0.05 value was used to establish significant differences within means.
| Y = β0 + β1X1 + β2X2 + β11X12 + β22X22 + β12X1X2 | (12) |
The estimated responses Y were mass transfer (WL, SG and WR), physicochemical (aw and TSS), colour (L*, a*, b*, ΔE* and YI), and nutraceutical (TC) parameters. Xi (i = 1, immersion time (t, in min) and i = 2, osmotic agent concentration (C, in °Brix)) represents the independent variables; β0 is the intercept coefficient, β1, and β2 are the linear coefficients; β11, and β22 are the quadratic coefficients and β12 is the interaction coefficient.
A Pearson's correlation was established using Minitab v.18 Statistical Software to establish a relationship between TCs and ΔE.
The TC impregnated in jicama was optimised by maximising this value. Finally, an impregnation experiment was conducted under the predicted process conditions to validate the obtained model.
3. Results and discussions
Tables 2 and 3 show the mean of experimental responses of mass transfer, aw, total soluble solids, carotenoid impregnation in jicama, and colour parameters for the 12 runs of jicama vacuum impregnation according to a 3 × 4 factorial design in Table 1.
Table 2 Mass transfer parameters (weight reduction = WR, moisture fraction = Mk, water loss = WL, and solid gain = SG), water activity (aw), total soluble solids (TSSs), and total carotenoids (TCs) of impregnated jicama cylinders with solutions of different concentrationsa
Run |
M
k
(g g−1 w.b.) |
WR (g g−1) |
WL (g g−1) |
SG (g g−1) |
TSSs (°Brix) |
a
w
|
TCs (mg β-carotene/100 g d.b.) |
Mean ± standard deviation, N = 3.
|
1 |
0.855 ± 0.001 |
0.276 ± 0.016 |
0.303 ± 0.014 |
0.027 ± 0.002 |
9.479 ± 0.199 |
0.977 ± 0.008 |
0.09 ± 0.007 |
2 |
0.796 ± 0.009 |
0.428 ± 0.009 |
0.467 ± 0.008 |
0.039 ± 0.002 |
15.289 ± 0.025 |
0.968 ± 0.002 |
0.17 ± 0.006 |
3 |
0.708 ± 0.004 |
0.570 ± 0.019 |
0.618 ± 0.013 |
0.047 ± 0.006 |
21.890 ± 0.41 |
0.955 ± 0.003 |
0.27 ± 0.001 |
4 |
0.700 ± 0.001 |
0.613 ± 0.016 |
0.651 ± 0.01 |
0.038 ± 0.005 |
18.808 ± 0.142 |
0.940 ± 0.009 |
0.29 ± 0.003 |
5 |
0.851 ± 0.003 |
0.167 ± 0.014 |
0.196 ± 0.012 |
0.029 ± 0.002 |
9.043 ± 0.200 |
0.983 ± 0.002 |
0.05 ± 0.004 |
6 |
0.819 ± 0.002 |
0.345 ± 0.019 |
0.369 ± 0.016 |
0.024 ± 0.004 |
11.402 ± 0.195 |
0.984 ± 0.004 |
0.14 ± 0.003 |
7 |
0.811 ± 0.004 |
0.433 ± 0.010 |
0.445 ± 0.008 |
0.013 ± 0.002 |
14.309 ± 0.201 |
0.967 ± 0.009 |
0.22 ± 0.006 |
8 |
0.763 ± 0.009 |
0.521 ± 0.014 |
0.539 ± 0.011 |
0.019 ± 0.003 |
15.493 ± 0.030 |
0.954 ± 0.003 |
0.20 ± 0.001 |
9 |
0.856 ± 0.008 |
0.124 ± 0.009 |
0.172 ± 0.008 |
0.048 ± 0.001 |
8.727 ± 0.055 |
0.978 ± 0.002 |
0.09 ± 0.007 |
10 |
0.831 ± 0.005 |
0.268 ± 0.030 |
0.314 ± 0.025 |
0.046 ± 0.005 |
9.139 ± 0.036 |
0.975 ± 0.004 |
0.11 ± 0.002 |
11 |
0.803 ± 0.002 |
0.346 ± 0.009 |
0.397 ± 0.007 |
0.051 ± 0.002 |
13.012 ± 0.001 |
0.966 ± 0.001 |
0.14 ± 0.001 |
12 |
0.788 ± 0.003 |
0.418 ± 0.010 |
0.463 ± 0.008 |
0.045 ± 0.002 |
13.451 ± 0.002 |
0.959 ± 0.009 |
0.19 ± 0.007 |
Table 3 Colour parameters of impregnated jicama cylinders with solutions of different concentrations of concentrated carrot juicea
Run |
L*
|
a* |
b* |
ΔE |
YI |
Mean ± standard deviation, N = 3. ΔE is the total colour difference, with respect to fresh jicama, = 71.40 ± 3.98, = −0.75 ± 1.43, and = 7.34 ± 5.89. YI is the yellow index.
|
1 |
58.55 ± 1.25 |
2.54 ± 0.46 |
21.09 ± 5.02 |
19.40 ± 3.07 |
32.66 ± 7.24 |
2 |
60.35 ± 1.91 |
3.75 ± 0.80 |
22.45 ± 0.47 |
19.28 ± 1.67 |
33.85 ± 1.82 |
3 |
56.37 ± 0.73 |
4.83 ± 0.58 |
22.63 ± 0.98 |
22.19 ± 1.64 |
36.53 ± 2.17 |
4 |
57.28 ± 0.73 |
6.57 ± 0.21 |
27.20 ± 0.67 |
25.46 ± 0.26 |
43.17 ± 0.59 |
5 |
58.51 ± 1.90 |
4.54 ± 0.71 |
18.99 ± 0.37 |
18.21 ± 1.27 |
29.52 ± 0.43 |
6 |
56.24 ± 1.27 |
3.14 ± 0.65 |
18.89 ± 1.13 |
19.46 ± 1.72 |
30.56 ± 2.42 |
7 |
57.34 ± 1.50 |
3.39 ± 1.53 |
20.84 ± 2.14 |
20.08 ± 0.58 |
33.00 ± 2.55 |
8 |
57.77 ± 1.41 |
6.05 ± 0.88 |
26.11 ± 1.50 |
24.23 ± 1.04 |
41.08 ± 1.83 |
9 |
64.66 ± 0.77 |
1.83 ± 0.75 |
18.20 ± 1.28 |
13.06 ± 1.49 |
25.60 ± 1.99 |
10 |
61.41 ± 1.32 |
2.86 ± 0.08 |
22.00 ± 1.81 |
18.19 ± 0.70 |
32.54 ± 2.00 |
11 |
56.62 ± 3.94 |
4.53 ± 1.68 |
21.66 ± 3.12 |
21.58 ± 2.47 |
32.59 ± 0.08 |
12 |
55.35 ± 1.10 |
6.2 3 ± 0.45 |
24.87 ± 1.14 |
24.81 ± 0.35 |
40.84 ± 1.12 |
A second-order polynomial equation fitted to each response variable was used to analyse the significant effects of the process variables. Polynomial models obtained after eliminating the non-significant terms (p ≥ 0.05) are shown in Table 4. According to the ANOVA, all the non-coded models (except for solute gain, SG) were sufficiently accurate in predicting the responses and presented a non-significant lack of fit (p ≥ 0.05). The polynomial model equation properly fitted the experimental data, with coefficients of determination values of R2 > 0.8 for Mk, WR, WL, aw, TSSs, TCs, ΔE, and YI. On the other hand, the polynomial model equations for L*, a* and b* colour parameters have R2 > 0.6, which indicates that they are not suitable for predicting these experimental values.
Table 4 Non-coded regression coefficients of second-order polynomial modelsa
Model |
R
2
|
Lack of fit |
Parameters in bold have a significant (p ≤ 0.05) effect on response. Mk, moisture fraction (g g−1); WR, weight reduction (g g−1); WL, water loss (g g−1); SG, solute gain (g g−1); awM water activity of the sample; awAO water activity of osmotic solution; TSSs, total soluble solids (°Brix) and TCs, total carotenoids (mg β-carotene/100 g d.b.); L*, lightness; a*, red to green; b*, yellow to blue; ΔE, total colour difference with respect to fresh jicama; YI, yellow index. ND: not determined.
|
M
k
= 1.353 − 0.031t − 0.0035C + 0.0005t2 |
0.846 |
0.35 |
WR = −1.471 + 0.087t + 0.024C − 0.0014t2 − 0.0002C2 + 9.6 × 10−5tC |
0.986 |
0.28 |
WL = −1.453 + 0.088t + 0.025C − 0.0015t2 − 0.0002C2 + 7.6 × 10−5tC |
0.984 |
0.43 |
SG = 0.075 − 0.001t |
0.733 |
ND |
a
wM = 1.043 − 0.0055t + 0.001C + 0.0001t2 − 0.00002C2 − 2 × 10−5tC |
0.798 |
0.23 |
a
wAO = 1.045 − 0.0007t + 0.003C + 2 × 10−5tC |
0.997 |
0.11 |
TSSs = −44.70 + 2.801t + 0.835C − 0.0455t2 − 0.00836C2 + 1 × 10−9tC |
0.856 |
0.58 |
TCs = −0.58 + 0.034t + 0.01C − 6 × 10−4t2 − 0.0001C2 + 1 × 10−4tC |
0.891 |
0.33 |
L* = 82.89 − 0.655t − 0.613C + 0.0158tC |
0.620 |
0.16 |
a* = −0.74 + 0.198t − 0.047C + 0.0044C2 − 0.0051tC |
0.730 |
0.18 |
b* = −0.74 + 1.250t + 0.508C − 0.0152t2 + 0.0085tC |
0.600 |
0.28 |
ΔE = −10.99 + 1.250t + 0.508C − 0.0152t2 + 0.0085tC |
0.807 |
0.19 |
YI = −5.16 + 1.848t + 0.397C − 0.0307t2 |
0.799 |
0.26 |
In general, the second-order polynomial equations showed that the parameters related to WR, WL, awM, TSSs and TCs were significantly affected mainly by linear coefficients (time and concentration), quadratic coefficients of time and concentration, and the time–concentration interaction (p ≤ 0.05). The models for b* and ΔE were not significantly affected (p ≥ 0.05) by quadratic coefficients of concentration. In the case of the model for a*, the quadratic coefficients of time had no significant effect (p ≥ 0.05). On the other hand, Mk and YI were not significantly affected (p ≥ 0.05) by quadratic coefficients of concentration of the osmotic agent or the time–concentration interaction. SG was only affected by the time of the process.
3.1. Influence of process variables on water activity and mass transfer parameters
The pH of the impregnated samples does not show significant differences (p > 0.05) between each experimental condition (pH = 6.00 ± 0.02). The aw value of fresh jicama was 0.982 ± 0.005 (25.01 ± 0.10 °C). Initial aw values of osmotic solutions were 0.972 ± 0.003, 0.960 ± 0.004, 0.940 ± 0.006, and 0.912 ± 0.002, for 20, 30, 40, and 50 °Brix concentrations, respectively. After the process, the impregnated samples achieve an awM value between 0.933 and 0.987, corresponding to a percentage variation of around 5%. The relationship between the jicama WL and the water gain in the osmotic solution was determined by analysing the effects of time and concentration of the osmotic agent. The experimental data were fitted to a second-order polynomial equation for each aw. For both models, the linear and interaction coefficients of t–C were significant (p ≤ 0.05). The model allowed for the observation that when the awM decreases, the awAO increases.
Fig. 1 shows the effect of the t–C interaction on awM. As shown, the higher the initial concentration of the osmotic agent, the lower the awM value that is reached. Some authors relate this behaviour to the osmotic pressure caused by the concentration gradient between the impregnated sample and the osmotic solution; the higher the gradient, the greater the mass transfer.10 Xie & Zhao38 found that during the impregnation of Royal Gala apples with corn syrup solutions, solutions at 50% reduced the final aw (aw,sample = 0.97) more than those at 20% (aw,sample = 0.98). It is important to note that in some osmodehydrated and impregnated products, the aw may remain high, so these techniques are used as pre-treatments for a following drying step that reduces the aw (aw < 0.6) to have microbiologically stable products.
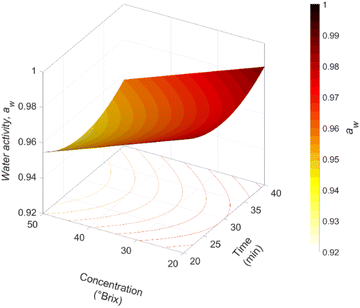 |
| Fig. 1 Time–concentration interaction (t–C) effect on water activity (aw = 1.043 − 0.0055t + 0.001C + 0.00011t2 − 0.00002C2 − 0.00002tC) of jicama cylinders impregnated with carrot juice concentrate. | |
On the other hand, increasing the immersion time improves the reduction of awM. However, at processing times longer than 30 min, an increase in the awM is observed. This is because the longer the vacuum pressure was applied, the more water was lost from the jicama. This water is diluted more slowly in the juice because the system had no mechanical force to homogenise the system concentration. Therefore, when the system pressure is restored, most of the previously removed water is returned to the sample along with the osmotic solution. Since the water reincorporated into the food matrix was not as tightly bound as before, the water activity increased with respect to the fresh product. This behaviour was similar to that reported by Castagnini et al.;19 these authors found that the aw of apples vacuum impregnated with cranberry juice did not show significant differences with respect to the fresh product despite having reached a moisture reduction of more than 2%. Also, the literature reports an increase in moisture compared to fresh samples during vacuum impregnation of mangoes with grape residue solution,15 apple slices with quercetin solutions,39 and pineapples with calcium solutions.40 Such a result can be due to the hydrodynamic mechanism induced by the pressure drop.
The moisture fraction of the fresh sample was 0.921 ± 0.001 g g−1, and after the impregnation treatment, this value was reduced between 7 and 24% (Table 2). The obtained mass transfer parameters for water loss and solid gain ranged between 0.172–0.65 g g−1, and 0.013–0.51 g g−1, respectively. It can be observed that the water transfer rate was higher than the solute transfer rate. The gain of solutes was similar to that reported during the impregnation of apples with Victoria grape juice concentrate and watermelons with sucrose solutions.16,41
Fig. 2 shows the effect of the t–C interaction on the mass transfer parameters obtained with the models in Table 4. As noticed, both WL and WR increase when the concentration of the osmotic agent increases. Similar behaviour is observed at processing times lower than 30 min. However, at higher times, a lower WL and WR are achieved. González-Pérez et al.16 described a similar behaviour in SG; this occurred mainly because a solute layer was formed on the external part of the food matrix, which limited mass transfer. In the case of jicama, a solute layer was not formed, but a water barrier was formed on the product's surface, limiting the permeation of the osmotic solution. In addition, some studies have found that matrices with low real porosities (such as fresh mangoes, εr = 0.01, and melons εr = 0.07) showed a reduction in solute transfer during vacuum impregnation with concentrated sucrose solutions, since it is more difficult to introduce a viscous osmotic solution into small pores17,42 as those of jicama (εr = 0.070 ± 0.004).
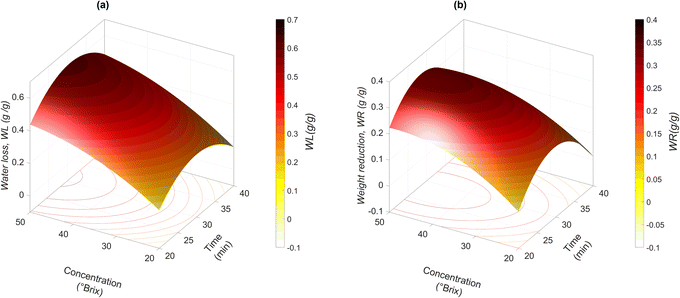 |
| Fig. 2 Time–concentration interaction (t–C) effect on (a) water loss (WL = −1.453 + 0.088t + 0.025C − 0.0015t2 − 0.00024C2 + 0.000076tC) and (b) weight reduction (WR = −1.471 + 0.087t + 0.024C − 0.0014t2 − 0.0002C2 + 0.000096tC) of jicama cylinders impregnated with carrot juice concentrate. | |
3.2. Influence of process variables on colour characteristics
A comparison of the general appearance and colour parameters of fresh and impregnated jicama with carrot juice concentrate is shown in Fig. 3 and Table 3. All the impregnated samples presented significant changes (p < 0.05) in the total colour difference (ΔE), taking the fresh sample as a reference. The ΔE of the impregnated samples was due to the colour of the osmotic solution. The total colour differences between the impregnated sample and the corresponding osmotic solutions were 24.02–33.86, 26.4–31.15, 23.61–29.66, and 18.72–20.92 for 20 °Brix (
= 47.66 ± 1.49,
= 18.77 ± 1.22, and
= 43.19 ± 2.33), 30 °Brix (
= 46.92 ± 0.49,
= 21.13 ± 0.31, and
= 42.79 ± 0.20), 40 °Brix (
= 45.34 ± 0.05,
= 21.42 ± 0.06, and
= 41.54 ± 0.06), and 50 °Brix (
= 44.52 ± 0.83,
= 20.13 ± 1.09, and
= 39.23 ± 0.28), respectively. Therefore, the smaller the difference, the closer the colour was to that of the juice.
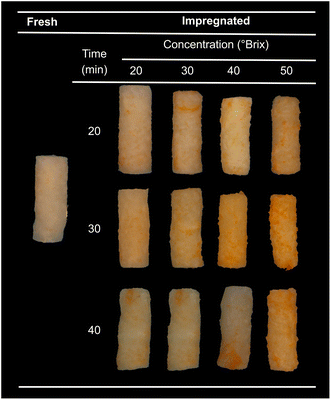 |
| Fig. 3 General appearance of fresh and vacuum-impregnated jicama cylinders with carrot juice concentrate. | |
Fig. 4 shows the effect of the t–C interaction on the colour variation. The total colour variation increased with an increasing concentration, showing a significant (p < 0.05) increase at 30 min of processing. A significant (p < 0.05) reduction of the mass transfer parameters was observed at longer times. This behaviour was similar to that reported during vacuum impregnation (P = 500 mm Hg and t = 7 min) of lycopene
:
β-carotene solution (ratio of 50
:
50, 40
:
60 and 25
:
75) in bananas, mangoes, peaches and papayas. These showed that colour variation increased when the β-carotene concentration increased.43 The ΔE was similar to the behaviour of mass transfer parameters (WL and WR). Some authors relate the impregnated sample's mass transfer parameters with the osmotic solution's colour parameters; the higher the WL or SG values, the greater the ΔE.43,44
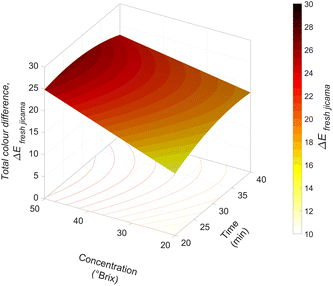 |
| Fig. 4 Time–concentration interaction (t–C) effect on the total colour difference (with respect to fresh jicama, ΔE = −10.99 + 1.250t + 0.508C − 0.0152t2 + 0.0085tC) of impregnated jicama using carrot juice concentrate. | |
The YI of the carrot juice concentrate solutions was between 82.38 and 83.9. This value significantly increased as the juice concentration increased, indicating an increment in the carotenoids' concentration, which translated into the yellow colour intensity.43,45 The impregnated samples had YI values of 25.6–43.17. According to the YI model in Table 4, the YI value increases significantly (p < 0.05) when increasing the concentration and is related to the amount of the impregnated osmotic solution.
3.3. Influence of process variables on total soluble solids
TSSs of fresh jicama were 6.6 ± 0.3 °Brix. After the impregnation process, the food matrix increased the amount of TSS by between 32 and 232% (8.72 and 21.89 °Brix).
According to the model in Table 4 and Fig. 5, increasing the osmotic solution concentration favours a TSS increment in the food matrix. However, at concentrations close to 50 °Brix of carrot juice, a slight decrease in TSSs is observed; during the vacuum stage, the jicama loses a greater amount of water due to the concentration gradient (between the food matrix and the osmotic solution), which increases the osmotic pressure of the system. Likewise, as mentioned above, all the water removed forms a barrier on the food matrix; for low water content, this barrier can be dissolved with the juice, but as more water is eliminated at longer times, it becomes insufficient to dissolve it completely. Therefore, when atmospheric pressure is restored, the water that failed to dilute the juice and the juice diluted with part of the removed water, are impregnated into the jicama tissue, resulting in a product with fewer TSSs (compared to the experiments not affected by a significant dilution of the osmotic solution). This behaviour depends on the type of osmotic agent since it has been found that in grape juice concentrate and fructose corn syrup, the increase in TSS incorporation by vacuum is favoured by increasing the time and concentration of the osmotic agent.16,38 However, our findings indicate that at high concentrations of the osmotic agent, TSS impregnation began to decrease due to the viscosity of the osmotic agent.
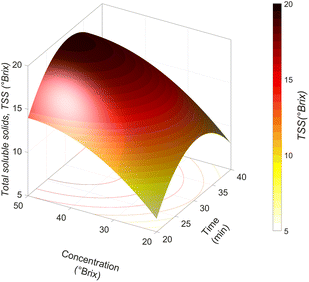 |
| Fig. 5 Time–concentration interaction (t–C) effect on total soluble solids (TSSs = −44.70 + 2.801t + 0.835C − 0.04545t2 − 0.00836C2 + 0.0008tC) of jicama cylinders impregnated with carrot juice concentrate. | |
In this work at concentrations of 20 and 30 °Brix of carrot juice, the solutions behave as Newtonian fluids, keeping the fluid viscosity during the whole process at 28.32 ± 0.46 and 45.12 ± 01.04 mPa s, respectively. However, at concentrations of 40 and 50 °Brix, the osmotic solutions behaved as dilatant fluids (n > 1 power law) with viscosity variations of 76.64–78.56 and 142–146.2 mPa s, respectively. These results would explain why the incorporation of the osmotic solution decreases when the atmospheric pressure is re-established since a dilatant fluid increases its viscosity as the strain rate increases (caused by force generated when the pressure is re-established).
3.4. Influence of process variables on total carotenoids
Impregnated jicama TCs were 0.05–29 mg β-carotene/100 g d.b. Carrot juice concentrate had 154.66 mg β-carotene/100 g d.b. The average recommended daily carotenoid intake requirements for adults worldwide vary from 0.81 to 22 mg β-carotene per day.46,47 Therefore, under the maximum impregnation conditions, a portion of 100 g of the obtained product may contribute a considerable percentage to the minimum daily requirement of β-carotene.
According to the polynomial model for predicting TCs (Fig. 6), the effect of t–C interaction is remarkably similar to that of TSS impregnation since the higher the concentration of the osmotic solution, the higher the amount of TCs in the food matrix. Likewise, a reduction of TC impregnation is observed at times higher than 30 min. In most of the studies on the impregnation of β-carotene in fruits, TCs increased with an increasing solution concentration, as in the vacuum impregnation of bananas, papayas, peaches and mangoes with solutions of β-carotene and lutein in water or apples in sucrose solutions with β-carotene powder.21,43
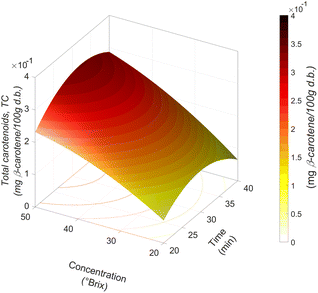 |
| Fig. 6 Time–concentration interaction (t–C) effect on total carotenoids (TCs = −0.58 + 0.034t + 0.01C − 6 × 10−4t2 − 0.0001C2 + 1 × 10−4tC) of jicama cylinders impregnated with carrot juice concentrate. | |
3.5. Model validation
Optimisation of the impregnation process was carried out by maximising the TCs or ΔE. In each case, the same optimum process conditions were found. To validate this, a Pearson's correlation was used to analyse the existence of a relationship between the two. The analysis revealed a strongly (r2 = 0.6645) significant positive correlation (p < 0.01). This result establishes that higher values of TCs lead to higher ΔE, indicating higher juice incorporation.
Impregnation experiments were conducted under the optimum process conditions for model validation. The optimum process parameters were at t = 31 min and C = 50 °Brix carrot juice. The experimental values (mean of the three experiments) are shown in Table 5. It was observed that the estimated values of each response are consistent with the fitted data. Therefore, the models are adequate to assess the behaviour of the impregnation process. The amount of TCs reached provides ∼30% of the minimum recommended percentage of daily carotenoid intake requirements for adults.46 The aw value of impregnated tissue under optimum conditions is very high. This excess water must be removed by a drying process to avoid spoilage and prolong its shelf life. Some studies have analysed the shelf life of vacuum-impregnated products, such as melons with calcium lactate. However, microbiological deterioration of the product was observed after 5 days.48 On the other hand, the use of drying after an impregnation process increases the shelf life of kiwifruit vacuum impregnated with 60 °Brix sucrose solutions up to 9 months.49 Thus, the vacuum impregnation process is suitable as pretreatment to fortify the food matrix tissue with some solutes of interest, but it requires a drying step.
Table 5 Predicted and experimental values of response under optimum process conditions (t = 31 min and 50 °Brix) for vacuum impregnation of jicama with carrot juice
Response |
Predicted value (CI 95%) |
Experimental value |
M
k
(g g−1 w.b.) |
0.7133 (0.698/0.728) |
0.710 ± 0.0001 |
a
wM
|
0.943 (0.939/0.948) |
0.941 ± 0.002 |
WL (g g−1) |
0.650 (0.634/0.694) |
0.661 ± 0.010 |
WR (g g−1) |
0.617 (0.602/0.632) |
0.616 ± 0.011 |
TSSs (°Brix) |
19.2 (17.9/20.6) |
19.00 ± 0.02 |
TCs (mg β-carotene/100 g d.b.) |
0.269 (0.248/0.290) |
0.271 ± 0.007 |
L*
|
56.423 (55.327/57.520) |
56.44 ± 0.71 |
a*
|
6.211 (5.600/6.822) |
6.39 ± 0.18 |
b*
|
26.376 (24.787/27.964) |
26.47 ± 0.52 |
ΔE |
25.389 (24.142/26.637) |
25.32 ± 0.26 |
YI |
42.490 (40.264/44.716) |
42.63 ± 0.30 |
The colour parameters for the sample under the optimum conditions were L* = 56.44 ± 0.71, a* = 6.39 ± 0.18, and b* = 26.47 ± 0.52; these correspond to values of yellow/slightly orangey (Hue° = 76.4 ± 0.12 and Chrome = 27.2 ± 0.7). Hue° values are like those reported for orange sweet potatoes.31
Some authors have reported that some carotenoid-rich fruits and vegetables have yellow and orange flesh.32,33 Compared with the fresh sample, all chromatids had a change giving a net colour difference ΔEjicama = 25.29 ± 0.25 (
= 71.4 ± 3.9,
= −0.746 ± 0.2,
= 7.34 ± 5.89,
= 98.8 ± 0.04, and Chrome0 = 11.0 ± 0.02). The colour of the impregnated sample was mainly due to the colour of the osmotic agent because the net colour difference between the impregnated sample and 50 °Brix juice was less than that of the fresh jicama 18.58 ± 0.24 (juice chromatids showed
= 77.3 ± 0.02,
= −2.52 ± 0.18,
= 7.75 ± 0.35, Hue° = 64.4 ± 0.20, and Chrome = 41.8 ± 1.1).
4. Conclusions
In conclusion, the overall findings demonstrate the potential of vacuum impregnation to incorporate bioactive compounds, such as carotenoids, into jicama effectively. The increase of the osmotic agent concentration favoured the increase of mass transfer parameters (WL and WR), the reduction of aw, and the impregnation of TSSs, ΔE, YI, and TCs. At long immersion times, the water removed during the vacuum pressure stage reduced the juice impregnation during the pressure build-up stage, which increased the awM and Mk and reduced the WL, WR, TSS, ΔE, YI, and TC values. Optimisation of the impregnation process parameters using response surface methodology to maximise TCs or ΔE resulted in finding optimal processing conditions. These findings highlight the potential of vacuum impregnation and response surface methodology in improving the nutritional quality of jicama and other food products. It is recommended for future studies to perform a drying treatment to reduce the aw of a product in order to increase its shelf life.
Author contributions
Julio E. González-Pérez: conceptualization, data curation, formal analysis, investigation, writing – original draft; Oscar Jiménez-González: writing – review & editing, methodology; Nelly Ramírez-Corona: formal analysis, writing – review & editing; Aurelio López-Malo: conceptualization, resources, writing – review & editing.
Conflicts of interest
No conflict of interest related to this work.
Acknowledgements
The authors González-Pérez and Jiménez-González gratefully acknowledge financial support for their PhD studies from Universidad de las Américas Puebla (UDLAP) and the National Council for Science and Technology (CONACyT) of Mexico. In addition, the authors thank Ingredion México S.A. de C.V. for providing carrot juice concentrate.
References
- V. Acharya, R. Arutselvan, K. Pati, A. K. Rout, B. Dehury, V. B. S. Chauhan and M. Nedunchezhiyan, Structural Insights into the RNA Interaction with Yam Bean Mosaic Virus (Coat Protein) from Pachyrhizus erosus Using Bioinformatics Approach, PLOS One, 2022, 17(7), e0270534, DOI:10.1371/journal.pone.0270534.
- A. Bhanja, S. K. Paikra, P. P. Sutar and M. Mishra, Characterization and Identification of Inulin from Pachyrhizus erosus and Evaluation of Its Antioxidant and in-Vitro Prebiotic Efficacy, J. Food Sci. Technol., 2023, 60(1), 328–339, DOI:10.1007/s13197-022-05619-6.
- V. Jaiswal, S. Chauhan and H.-J. Lee, The Bioactivity and Phytochemicals of Pachyrhizus Erosus (L.) Urb.: A Multifunctional Underutilized Crop Plant, Antioxidants, 2021, 11(1), 58, DOI:10.3390/antiox11010058.
- E. Buckman, W. Plahar, I. Oduro and E. Carey, Effects of Sodium Metabisulphite and Blanching Pretreatments on the Quality Characteristics of Yam Bean (Pachyrhizus erosus) Flour, Braz. J. Aquat. Sci. Technol., 2015, 6(2), 138–144, DOI:10.9734/BJAST/2015/14773.
- R. Olson, B. Gavin-Smith, C. Ferraboschi and K. Kraemer, Food Fortification: The Advantages, Disadvantages and Lessons from Sight and Life Programs, Nutrients, 2021, 13(4), 1118, DOI:10.3390/nu13041118.
-
CDC, Mictronutrient Malnutrition, Centers for Disease Control and Prevention, 2021, https://www.cdc.gov/nutrition/micronutrient-malnutrition/index.html, accessed 2022-10-27.
-
WHO, Staple crops biofortified with increased vitamins and minerals: considerations for a public health strategy, WHO, 2020, http://www.who.int/nutrition/callforauthors_staplecrops_biofortified_vitminarels/en/, accessed 2022-10-27 Search PubMed.
- J. Kruger, J. R. N. Taylor, M. G. Ferruzzi and H. Debelo, What Is Food-to-food Fortification? A Working Definition and Framework for Evaluation of Efficiency and Implementation of Best Practices, Compr. Rev. Food Sci. Food Saf., 2020, 19(6), 3618–3658, DOI:10.1111/1541-4337.12624.
- R. Pandiselvam, Y. Tak, E. Olum, O. J. Sujayasree, Y. Tekgül, G. Çalışkan Koç, M. Kaur, P. Nayi, A. Kothakota and M. Kumar, Advanced Osmotic Dehydration Techniques Combined with Emerging Drying Methods for Sustainable Food Production: Impact on Bioactive Components, Texture, Color, and Sensory Properties of Food, J. Texture Stud., 2022, 53(6), 737–762, DOI:10.1111/jtxs.12643.
- J. E. González-Pérez, N. Ramírez-Corona and A. López-Malo, Mass Transfer during Osmotic Dehydration of Fruits and Vegetables: Process Factors and Non-Thermal Methods, Food Eng. Rev., 2021, 13(2), 344–374, DOI:10.1007/s12393-020-09276-3.
- C. Aubert, M. Bruaut and G. Chalot, Spatial Distribution of Sugars, Organic Acids, Vitamin C, Carotenoids, Tocopherols, 6-Methoxymellein, Polyacetylenic Compounds, Polyphenols and Terpenes in Two Orange Nantes Type Carrots (Daucus Carota L.), J. Food Compos. Anal., 2022, 108, 104421, DOI:10.1016/j.jfca.2022.104421.
- H. Tapiero, D. M. Townsend and K. D. Tew, The Role of Carotenoids in the Prevention of Human Pathologies, Biomed. Pharmacother., 2004, 58(2), 100–110, DOI:10.1016/j.biopha.2003.12.006.
- M. A. González-Peña, J. D. Lozada-Ramírez and A. E. Ortega-Regules, Carotenoids from Mamey (Pouteria sapota) and Carrot (Daucus carota) Increase the Oxidative Stress Resistance of Caenorhabditis Elegans, Biochem. Biophys. Rep., 2021, 26, 100989, DOI:10.1016/j.bbrep.2021.100989.
- D. Arias, A. Arenas-M, C. Flores-Ortiz, C. Peirano, M. Handford and C. Stange,
Daucus carota DcPSY2 and DcLCYB1 as Tools for Carotenoid Metabolic Engineering to Improve the Nutritional Value of Fruits, Front. Plant Sci., 2021, 12, 677553, DOI:10.3389/fpls.2021.677553.
- R. A. Batista de Medeiros, E. Vieira da Silva Júnior, J. H. Fernandes da Silva, O. da Cunha Ferreira Neto, S. C. Rupert Brandão, Z. M. Pimenta Barros, O. R. Sá da Rocha and P. M. Azoubel, Effect of Different Grape Residues Polyphenols Impregnation Techniques in Mango, J. Food Eng., 2019, 262, 1–8, DOI:10.1016/j.jfoodeng.2019.05.011.
- J. E. González-Pérez, O. Jiménez-González, N. Ramírez-Corona, J. A. Guerrero-Beltrán and A. López-Malo, Vacuum Impregnation on Apples with Grape Juice Concentrate: Effects of Pressure, Processing Time, and Juice Concentration, Innovative Food Sci. Emerging Technol., 2022, 77, 102981, DOI:10.1016/j.ifset.2022.102981.
- H. Mújica-Paz, A. Valdez-Fragoso, A. López-Malo, E. Palou and J. Welti-Chanes, Impregnation and Osmotic Dehydration of Some Fruits: Effect of the Vacuum Pressure and Syrup Concentration, J. Food Eng., 2003, 57(4), 305–314, DOI:10.1016/S0260-8774(02)00344-8.
- T. Guz, L. Rydzak and M. Domin, Influence of Selected Parameters and Different Methods of Implementing Vacuum Impregnation of Apple Tissue on Its Effectiveness, Processes, 2020, 8(4), 428, DOI:10.3390/pr8040428.
- J. M. Castagnini, N. Betoret, E. Betoret and P. Fito, Vacuum Impregnation and Air Drying Temperature Effect on Individual Anthocyanins and Antiradical Capacity of Blueberry Juice Included into an Apple Matrix, LWT–Food Sci. Technol., 2015, 64(2), 1289–1296, DOI:10.1016/j.lwt.2015.06.044.
- A. S. Panayampadan, M. S. Alam, R. Aslam and J. Kaur, Vacuum Impregnation Process and Its Potential in Modifying Sensory, Physicochemical and Nutritive Characteristics of Food Products, Food Eng. Rev., 2022, 14(2), 229–256, DOI:10.1007/s12393-022-09312-4.
- C. Santacruz-Vázquez, V. Santacruz-Vázquez, M. E. Jaramillo-Flores, J. Chanona-Pérez, J. Welti-Chanes and G. F. Gutiérrez-López, Application of Osmotic Dehydration Processes to Produce Apple Slices Enriched with β-Carotene, Drying Technol., 2008, 26(10), 1265–1271, DOI:10.1080/07373930802307266.
- C. Santacruz-Vázquez and V. Santacruz-Vázquez, The Spatial Distribution of β-Carotene Impregnated in Apple Slices Determined Using Image and Fractal Analysis, J. Food Sci. Technol., 2015, 52(2), 697–708, DOI:10.1007/s13197-013-1097-5.
- M. C. Giannakourou, A. E. Lazou and E. K. Dermesonlouoglou, Optimization of Osmotic Dehydration of Tomatoes in Solutions of Non-Conventional Sweeteners by Response Surface Methodology and Desirability Approach, Foods, 2020, 9(10), 1393, DOI:10.3390/foods9101393.
- H. Mújica-Paz, A. Valdez-Fragoso, A. López-Malo, E. Palou and J. Welti-Chanes, Impregnation Properties of Some Fruits at Vacuum Pressure, J. Food Eng., 2003, 56(4), 307–314, DOI:10.1016/S0260-8774(02)00155-3.
- M. Abud-Archila, D. G. Vázquez-Mandujano, M. A. Ruiz-Cabrera, A. Grajales-Lagunes, M. Moscosa-Santillán, L. M. C. Ventura-Canseco, F. A. Gutiérrez-Miceli and L. Dendooven, Optimization of Osmotic Dehydration of Yam Bean (Pachyrhizus erosus) Using an Orthogonal Experimental Design, J. Food Eng., 2008, 84(3), 413–419, DOI:10.1016/j.jfoodeng.2007.06.003.
- M. Kidoń, E. Radziejewska-Kubzdela, R. Biegańska-Marecik and P. Ł. Kowalczewski, Suitability of Apples Flesh from Different Cultivars for Vacuum Impregnation Process, Appl. Sci., 2023, 13(3), 1528, DOI:10.3390/app13031528.
-
AOAC International, Official Method of Analysis, AOAC International, Gaithersburg, MD, 16th edn, 1995 Search PubMed.
- B. Rongtong, T. Suwonsichon, P. Ritthiruangdej and S. Kasemsumran, Determination of Water Activity, Total Soluble Solids and Moisture, Sucrose, Glucose and Fructose Contents in Osmotically Dehydrated Papaya Using Near-Infrared Spectroscopy, Agric. Nat. Resour., 2018, 52(6), 557–564, DOI:10.1016/j.anres.2018.11.023.
- J. E. González-Pérez, E. M. López-Méndez, C. E. Ochoa-Velasco and I. I. Ruiz-López, Mass Transfer and Morphometric Characteristics of Fresh and Osmodehydrated White Mushroom Pilei during Convective Drying, J. Food Eng., 2019, 262, 181–188, DOI:10.1016/j.jfoodeng.2019.06.017.
- M. Xiao, J. Bi, J. Yi, Y. Zhao, J. Peng, L. Zhou and Q. Chen, Osmotic Pretreatment for Instant Controlled Pressure Drop Dried Apple Chips: Impact of the Type of Saccharides and Treatment Conditions, Drying Technol., 2019, 37(7), 896–905, DOI:10.1080/07373937.2018.1473419.
- M. C. Murillo-Cruz, N. Rodrigues, R. Bermejo-Román, A. C. A. Veloso, J. A. Pereira and A. M. Peres, An Electronic Tongue as a Tool for Assessing the Impact of Carotenoids' Fortification on Cv. Arbequina Olive Oils, Eur. Food Res. Technol., 2022, 248(5), 1287–1298, DOI:10.1007/s00217-022-03964-6.
- J. W. Rhim, Y. Wu, C. L. Weller and M. Schnepf, Physical Characteristics of a Composite Film of Soy Protein Isolate and Propyleneglycol Alginate, J. Food Sci., 1999, 64(1), 149–152, DOI:10.1111/j.1365-2621.1999.tb09880.x.
- S. A. Desobry, F. M. Netto and T. P. Labuza, Comparison of Spray-Drying, Drum-Drying and Freeze-Drying for β-Carotene Encapsulation and Preservation, J. Food Sci., 1997, 62(6), 1158–1162, DOI:10.1111/j.1365-2621.1997.tb12235.x.
- O. Jimenez-Gonzalez, J. J. Luna-Guevara, M. M. Ramírez-Rodrigues, D. Luna-Vital and M. L. Luna-Guevara, Microencapsulation of Renealmia alpinia (Rottb.) Maas Pulp Pigment and Antioxidant Compounds by Spray-Drying and Its Incorporation in Yogurt, J. Food Sci. Technol., 2022, 59(3), 1162–1172, DOI:10.1007/s13197-021-05121-5.
-
D. Friso, Ingegneria Dell'industria Agroalimentare - Agri-Food Industry Engineering, CLEUP, Italy, 2017, vol. 1 Search PubMed.
- J. L. G. Corrêa, D. B. Ernesto, J. G. L. F. Alves and R. S. Andrade, Optimization of Vacuum Pulse Osmotic Dehydration of Blanched Pumpkin, Int. J. Food Sci. Technol., 2014, 49(9), 2008–2014, DOI:10.1111/ijfs.12502.
-
Z. Escobedo-Avellaneda, R. García-García, A. Valdez-Fragoso, H. Mújica-Paz and J. Welti-Chanes, Fruit Preservation and Design of Functional Fruit Products by Vacuum Impregnation, in Fruit Preservation, ed. A. Rosenthal, R. Deliza, J. Welti-Chanes and G. V. Barbosa-Cánovas, Food Engineering Series, Springer, New York, 2018, DOI:10.1007/978-1-4939-3311-2.
- J. Xie and Y. Zhao, Nutritional Enrichment of Fresh Apple (Royal gala) by Vacuum Impregnation, Int. J. Food Sci. Nutr., 2003, 54(5), 387–398, DOI:10.1080/09637480310001595261.
- B. Schulze, E. M. Hubbermann and K. Schwarz, Stability of Quercetin Derivatives in Vacuum Impregnated Apple Slices after Drying (Microwave Vacuum Drying, Air Drying, Freeze Drying) and Storage, LWT–Food Sci. Technol., 2014, 57(1), 426–433, DOI:10.1016/j.lwt.2013.11.021.
- M. M. d. Lima, G. Tribuzi, J. A. R. d. Souza, I. G. d. Souza, J. B. Laurindo and B. A. M. Carciofi, Vacuum Impregnation and Drying of Calcium-Fortified Pineapple Snacks, LWT–Food Sci. Technol., 2016, 72, 501–509, DOI:10.1016/j.lwt.2016.05.016.
- C.-H. Kuo, J. Lin, C.-Y. Huang, S.-L. Hsieh, S. Li, J.-M. Kuo and C.-J. Shieh, Predicting Sugar Content of Candied Watermelon Rind during Osmotic Dehydration, Food Sci. Technol., 2018, 38(suppl. 1), 228–235, DOI:10.1590/fst.18817.
- G. Giraldo, P. Talens, P. Fito and A. Chiralt, Influence of Sucrose Solution Concentration on Kinetics and Yield during Osmotic Dehydration of Mango, J. Food Eng., 2003, 58(1), 33–43, DOI:10.1016/S0260-8774(02)00331-X.
- M. Santana Moreira, D. de Almeida Paula, E. Maurício Furtado Martins, É. Nascif Rufino Vieira, A. Mota Ramos and P. C. Stringheta, Vacuum Impregnation of β-Carotene and Lutein in Minimally Processed Fruit Salad, J. Food Process. Preserv., 2018, 42(3), e13545, DOI:10.1111/jfpp.13545.
- J. E. González-Pérez and A. López-Malo, Sensory Evaluation of Apple Cubes (Granny Smith Var.) Impregnated with Concentrated Grape Juice (Victoria Var.) Subjected to Convection Drying, RIIT, 2022, 9(54), 28–39 Search PubMed.
- S. P. M. Germer, C. C. Ferrari, J. P. Lancha, S. A. G. Berbari, S. M. Carmello-Guerreiro and C. R. G. Ruffi, Influence of Processing Additives on the Quality and Stability of Dried Papaya Obtained by Osmotic Dehydration and Conventional Air Drying, Drying Technol., 2014, 32(16), 1956–1969, DOI:10.1080/07373937.2014.924963.
- V. Böhm, G. Lietz, B. Olmedilla-Alonso, D. Phelan, E. Reboul, D. Bánati, P. Borel, J. Corte-Real, A. R. de Lera, C. Desmarchelier, J. Dulinska-Litewka, J.-F. Landrier, I. Milisav, J. Nolan, M. Porrini, P. Riso, J. M. Roob, E. Valanou, A. Wawrzyniak, B. M. Winklhofer-Roob, R. Rühl and T. Bohn, From Carotenoid Intake to Carotenoid Blood and Tissue Concentrations – Implications for Dietary Intake Recommendations, Nutr. Rev., 2021, 79(5), 544–573, DOI:10.1093/nutrit/nuaa008.
- H. Müller, Die tägliche Aufnahme von Carotinoiden (Carotine und Xanthophylle) aus Gesamtnahrungsproben und die Carotinoidgehalte ausgewählter Gemüse- und Obstarten, Zeitschrift für Ernährungswissenschaft, 1996, 35(1), 45–50, DOI:10.1007/BF01612027.
- S. Tappi, U. Tylewicz, S. Romani, L. Siroli, F. Patrignani, M. Dalla Rosa and P. Rocculi, Optimization of Vacuum Impregnation with Calcium Lactate of Minimally Processed Melon and Shelf-Life Study in Real Storage Conditions, J. Food Sci., 2016, 81(11), E2734–E2742, DOI:10.1111/1750-3841.13513.
- Z. Göksel, A. Atak, C. Tunçkal and A. Yemenicioğlu, Determination of quality characteristics and shelf life of kiwifruit (A. chinensis var. deliciosa ‘Hayward’) slices by drying them in a heat pump dryer at different water content values, Acta Hortic., 2022, 311–320, DOI:10.17660/ActaHortic.2022.1332.41.
|
This journal is © The Royal Society of Chemistry 2023 |
Click here to see how this site uses Cookies. View our privacy policy here.