Modification of starch by novel and traditional ways: influence on the structure and functional properties
Received
18th November 2022
, Accepted 20th February 2023
First published on 22nd March 2023
Abstract
Starch, a polymeric carbohydrate widely present in plant biomass, has gained popularity owing to its functional characteristics, lower cost and ability to completely decompose without any toxic byproducts. Native starches have the fewest applications among the food and non-food sectors. Thus, modifications to eliminate their shortcomings, viz., low pH, reduced viscosity, easy retrogradation, gelling, and syneresis during cooking, will prove to be useful through application extension. Physical modifications are simple and less expensive. Meanwhile, chemical modifications involve the extraction of the hydroxyl group from the starch molecule, yielding the desirable outcome for the extensive use of starch. With the advent of green chemistry, considerable attention has been paid to the development of novel physical modifications with the advantages of high efficiency, environmentally friendly operation, and ease of starch modification. High hydrostatic pressure (HHP) processing, microwave processing, pulsed electric field (PEF), and cold plasma are some of the tactics that govern this modification accordingly, keeping in mind the environment suitability and application broadness as well. Recently, there has been increasing interest in the chemical alteration of starches by means of oxidation, succinylation and acetylation to explore new horizons to improve their utilization potential beyond food, viz., pharmaceuticals, textiles and other fields. Increases in the solubility features, in vitro digestibility terms, resistant starches proportion and application extension are the selected traits discussed herein.
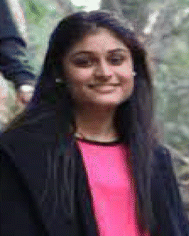 Shweta Suri | Dr Shweta Suri is an Assistant Professor at Amity University, Uttar Pradesh. She acquired her PhD from Govind Ballabh Pant University of Agriculture and Technology, Uttarakhand. Her field of interest are extrusion processing, food analysis, micronutrient deficiencies and waste utilization. Dr Suri served at NIFTEM as a research associate in a World Bank-funded project. She received the achievement of best poster presentation award at the 4th Annual Conference, organized by the AOAC India Chapter at New Delhi on November 11–12, 2016. She has contributed to book chapters, and research and review articles with publishing houses of international repute. |
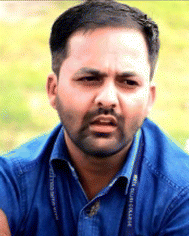 Ajay Singh | Dr Ajay Singh has about 8+ years of teaching and research experience. He has published around 30 papers and more than 25 book chapters in publishing houses of international repute, edited 5 books (03 CRC Press, 01 NIPA and 01 Objective Food Science with Brillion Publishers). Dr Singh has presented his talks, and demonstrate workshops at various national and international venues. He is the potential reviewer for many of international journals (Springer, Elsevier, Wiley and Taylor & Francis), and serving as an academic editor for Journal of Food Processing and Preservation (Wiley). His area of research and teaching is composite-blended foods, gluten-free baked optimization and functional approaches among new product development. |
1 Introduction
Starch is a natural storage polysaccharide found in abundance in plant roots, stems/stalks, and seeds, and is considered the second major biomass found on Earth.1 Chemically, it is a polymeric carbohydrate constituting amylose (linear molecule) and amylopectin (branched molecule) units joined by glycosidic linkages.2 Due to structural differences, these two units are distinct molecules in both physical and chemical nature.
The biochemical processes involved in the synthesis of starch include the production of glucose molecules by plant cells through the process of photosynthesis. It is formed in the chloroplasts and amyloplasts in green leaves. These are organelles involved in the synthesis of starch stored in cereal grains and tubers. Owing to its abundant presence, starch is extensively employed in different sectors as a raw material, such as papermaking, adhesives, bookbinding, corrugated board, plastics, textiles, construction, paints/varnishes, chemicals, pharmaceuticals, oil fields, and others.3,4 In addition, it is widely used in food, including ice creams, soups/curries/gravies/batters, bakery items, dairy items, and meat-based products as a stabilizer, thickener, and processing ingredient.5 Its broadened suitability as an ingredient and additive governs and regulates the homogeneity, texture variability, and stability of liquid foods, resists the breakdown of gels all through processing treatment, and extends the storage stability of products.6 Starch is also utilized in biodegradable packaging, thin-films, alcohol-containing fuels, and thermoplastics having enhanced thermal and mechanical activity.7
Native starch granules are usually modified to enhance the beneficial properties and eliminate shortcomings, such as low pH, reduced viscosity, easy retrogradation, gelling, and syneresis during cooking, thereby enhancing their applications in the food and non-food sectors. Starch processing is an ever-evolving industry, and there are many opportunities to create novel starches that possess innovative functional and value-added terms. Different starch modification methods include physical, chemical, and combination of methods/dual modification8 (Fig. 1). The starch oxidation, acetylation, succinylation, acid/alkali treatment, and cross-linking correspond to chemical modifications, while high hydrostatic pressure (HHP) processing, microwave processing, pulsed electric field (PEF), and cold plasma are novel and environmentally friendly operations. However, annealing (hydrothermal) and pre-gelatinization (thermal) are categorized as traditional physical modification methods.9 Different modifications/processing treatments also lead to alterations in the functional properties of starch. Depending on the requirements, various starch processing methods are used at the industrial level. Thermal processing treatment of starch in an aqueous environment has been shown to induce a shift from amorphous to crystalline starch, causing starch gelatinization.10 Chemical methods are often highly effective in modifying starch, but their disadvantages are related to chemical costs, chemical residues, time, and waste.11 Moreover, current novel physical methods through the use of moisture, thermal energy, radiation energy, high pressure, and cold plasma have progressively attracted interest due to their advantages of greater efficiency, simple operation, and generation of no chemical by-products. In addition, these physical and chemical starch modification methods can be used to design tailor-made food and non-food items containing starch and its derivatives.12 Focusing on the importance of starches and their modification, the present review is an in-depth discussion of the same, summarizing the various novel and traditional physical and chemical methods used to provide them with quality attributes through functional and structural terms. A discussion about the beneficial properties of altered starches compared to the native one qualifies their potential beyond food application, and thus may direct future upcoming research studies accordingly. Selection of starches from lists of sources i.e. tubers, cereals, coarse cereals and pulse crops were subjected to traditional to novel, cum physical to chemical reformations govern their existing potential to much broader application terms.
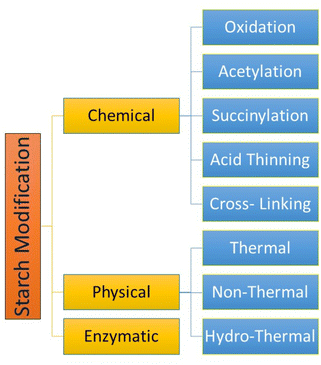 |
| Fig. 1 Methods of starch modification. | |
2 Modification of starch by different processes
Native starches have complex granular and crystalline organizations that vary in size among plants. Different parameters have an impact on the physical, chemical, structural, and functional characteristics of starch. Any alteration/variation in the molecular structure of starch through the environment, operations, and processing treatments is called starch modification. These changes can be beneficial or non-beneficial to the structure and function of starch molecules.13 Native starches show diversity in their properties. Thus, to improve the quality of these starches and achieve better results in various food and non-food applications, the scientific community has committed to modifying the starch structure in different ways.
2.1 Physical processes for modification of starches
The physical modification of starch is the alteration of starch properties caused by physical treatment. It does not result in chemical changes in the starch, other than the cleavage of a few glycosidic bonds.14 These are novel starch modification methods, which can be used to obtain environmentally friendly starch, called green technology. Generally, pre-gelatinization tactics are the common adopted method to carry out modifications among starches of any origin. Besides this, high hydrostatic pressure (HHP) processing, microwave processing, pulsed electric field (PEF) processing, and cold plasma technology15 are other non-conventional/green starch modification means that can achieve novelty among starches, as presented in Table 1.
Table 1 Starch modification by novel non-thermal methods
Modification type |
Raw material |
Findings |
References |
High hydrostatic pressure (HHP) processing |
Mung bean starch |
Complete gelatinization at 600 MPa for 30 min |
Li et al. (2011)18 |
HPP lowered the gelatinization temperature, gelatinization enthalpy, swelling capacity and solubility of starch |
Lotus seed starch |
Decrease in the starch solubility and swelling power at 85 to 95 °C while an increase was observed in same at 55 to 75 °C |
Guo et al. (2015)78 |
Quinoa starch |
Increase in the water holding capacity and particle volume upon treatment at pressure (0.1 to 600 MPa) and temperature (25 to 75 °C) |
Ahmed et al. (2018)79 |
Microwave processing |
Waxy maize starch |
α-(1,6) Glycosidic linkages were destroyed readily, as compared to the α-(1,4) glycosidic linkages of starch |
Yang et al. (2017)34 |
Microwave treatment for 5 min led to degradation of the internal chain (amorphous area), while external chains (crystalline area) were destroyed at 10 min treatment |
Potato starch |
The gelatinization temperature of starch was higher in the microwave-treated sample |
Xia et al. (2018)35 |
High peak viscosity, breakdown viscosity, and amylose content of microwave-treated sample |
Pulsed electric field (PEF) |
Aqueous starch suspension (wheat starch) |
PEF treatment with field strength (25 kV cm−1) and total specific energy input (50 kJ kg−1) showed a great impact on wheat starch (fractures on the granule surfaces, lowering of peak apparent viscosity, & creating firm gels), therefore beneficial for 3D printing of starch |
Maniglia et al. (2021)4 |
Pea starch |
Increase in resistant starch from 11.73% to 12.24%, and rapidly digested starch increased from 50.88% to 59.40% upon PEF treatment |
Li et al. (2019)41 |
Potato starch |
Improved emulsion stability after PEF treatment of potato starch acetic anhydride system |
Hong et al. (2018)80 |
Cold plasma |
Corn starch |
Dielectric barrier discharge plasma led to molecular oxidation, and degradation of starch molecules |
Bie et al. (2016b)23 |
Decreased crystallinity was observed upon plasma treatment |
Parboiled rice (Oryza sativa) |
Enhanced cooking quality of plasma-treated rice |
Sarangapani et al. (2015)43 |
Improved textural properties with a decline in hardness and stickiness with an increase in time and power |
2.1.1 Novel physical starch modification methods.
2.1.1.1 High hydrostatic pressure (HHP).
HHP processing is also known as cold gelatinization. Unlike the thermal treatment of starch, HHP processing can change the native granular organization of starch into a gelatinized structure. Starch gelatinization can be more simply achieved through HHP processing than thermal ways under defined settings.16 The main features of gelatinized starch processed through HHP processing is a partly disintegrated grain structure, transformed crystalline starch organization and lower viscosity of starch solution as compared to the thermal gelatinization of starch.17 A schematic view of HHP processing of starchy food is presented in Fig. 2. Generally, the effect of HHP processing on the level of starch gelatinization depends on the type of starch, water and pressure level, processing temperature, and time. Cereal-based starch shows high sensitivity to HHP treatment, while the legume and tuber starches are less sensitive.18 HHP offers many advantages over the thermal processing methods: (a) starch retrogradation is slower than the thermal process; (b) increase in the swelling and solubility of starch; (c) changes in the thermal and pasting characteristics; (d) alteration in the amount of fast/slow or resistant starch.19 Moreover, HHP of starch is helpful in its utilization in films. It was observed that HPP led to improvement in the physical attributes and water-resistance of the films.20
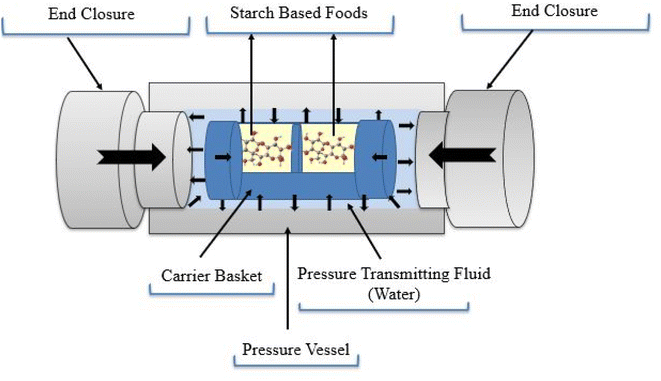 |
| Fig. 2 Schematic view of high hydrostatic pressure (HHP) processing. | |
For instance, HHP processing at a pressure of 600 MPa for 30 min led to complete gelatinization of mung bean starch.18 The lowering of the gelatinization temperature and gelatinization enthalpy was also noticed through a differential scanning calorimeter. Another study reported an upsurge in the slowly digested starch content of waxy wheat (31.12%) upon HHP retrogradation treatment at 600 MPa. The processing treatment also led to a decrease in the enthalpy of gelatinization, the relative crystallinity, and the peak viscosity of the waxy wheat starch samples. Moreover, changes in the in vitro digestibility, physico-chemical and structural characteristics of waxy wheat starch were noticed upon HHP retrogradation treatment.16 For the preparation of the starch-based film, buckwheat and tapioca starch were gelatinized using HHP treatment at 600 MPa, 20 °C for a period of 20 min. As a result, films made with HHP showed higher tensile strength and elongation at break than films made with thermal processing, regardless of starch type.20
Regarding the changes in the structural properties of starch upon HHP treatment, limited research has been conducted on the alterations in the molecular structure of starch upon HHP. However, studies have shown that the modification in the molecular structure under high pressure is closely linked to the origin of starch. Nonetheless, further studies are needed to know whether amylopectin chains could be more susceptible to breakdown by high pressure.1
In terms of the relative crystallinity of the starch molecules, it can be reduced with high pressure in HHP.20,21 The rationale behind the reduction in starch crystallinity is that HHP encourages the penetration of water molecules into the starch granules. It thus weakens the double helices in the crystalline area and destroys the internal crystal assembly, leading to a decrease in relative crystallinity.22
Studies on the influence of HHP on the starch granule morphology showed that the shape of sorghum starch remains intact with only some surface cracks upon the processing treatment with HHP at 120–360 MPa. However, when the processing pressure reached 480 MPa, the starch granules swelled and collapsed. Meanwhile, further increasing the processing pressure to 600 MPa led to deformation and fusion of the starch granules.23 Likewise, similar results of HHP treatment were obtained for corn starch24 and mung beans.18 Hence, HHP processing could be utilized to obtain desired starch properties without much alteration to the structure and functionality of starch.
An HHP-driven investigation on cassava starch25 showed transformation from crystallinity to an amorphous state. HPP-induced gelatinization (500 to 600 MPa) with a decrease in flour concentration and increase in holding time favors an increase in rapidly digestible starch with decreased resistant starch fraction. In general, high pressure processing increases the swelling power and solubility if subjected at 55–75 °C, but decreases these properties if subjected 85–95 °C. Starch granules also swell on pressure processing at 500 MPa. However, they are completely ruptured as the pressure increases, while keeping the birefringence intact.26
2.1.1.2 Microwave processing.
Microwave processing technology is well-known for its applications in several food technology operations. The energy created by the microwave generates electric and magnetic fields having spectral frequencies ranging from 300 MHz to 300 GHz. When exposed to microwaves, polarized or charged atoms have a tendency to align with components of the microwave electric field that rapidly change the direction of the microwaves (Fig. 3). Microwaves can create pores, bumps, and cracks in the starch granules to improve the efficiency of extraction. For example, microwave-treated granules of potato starch prior to acetylation processing exhibited more apparent pores than those without microwave processing, and further roughness after the acetylation process. The moisture level of starch is also an important factor that affects grain morphology.27 High moisture levels of food starch are very sensitive to microwave irradiation because water allows microwave energy to penetrate, causing pores to form and starch to collapse.28 However, regarding the mechanism of starch processing by microwaves, there is presently no definite explanation. Nonetheless, it is usually believed that the impact of microwave irradiation is mainly dependent on the dielectric heating causing the rapid dipole reorientation of the polar molecules of starch, thus leading to the development of structural variations.29
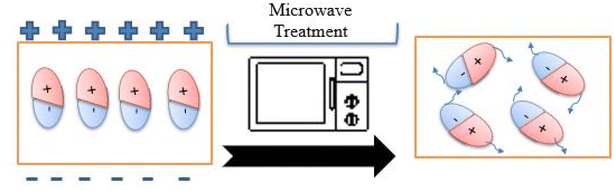 |
| Fig. 3 Mechanism of microwave processing. | |
A number of researchers worked on checking the influence of microwave processing on starch characteristics. For instance, Xie et al.30 reported that the molecular weight of potato starch granules decreased with longer microwave treatment. The variation in the morphology of starch is also strictly associated with the exposure time of the microwave. Research done by Oyeyinka et al.31 described that pores are formed on the starch granules of Bambara groundnut subsequently after 10 seconds of the microwave, and collapsed after 60 seconds. Cracks or holes in the surface might occur due to the higher internal vapor pressure caused by water upon the quick heating process, and the collapse may be caused by the destructive action on the internal assembly of starch.32
Ma et al.33 argued that all of the physical characteristics of the potato starch–water system, including the polarization-cross, thermal properties, and particle size, are influenced by microwave heating. The precise influence of microwave is important in the initial phases of gelatinization, but the thermal influences have a greater effect in later phases. Furthermore, Yang et al.34 detected a decrease in starch viscosity, enthalpy, and synergism of starch after microwave processing. In addition, α-(1,6)-glycosidic bonds were more effortlessly disrupted during microwave processing than α-(1,4)-glycosidic bonds. They also investigated the chain length profile of waxy maize starch using a high-performance anion exchange chromatography pulsed amperometric detector, and determined that the A-chain content was altered by microwave irradiation. The B-chain content increased with increasing microwave time, although a short irradiation time (5 minutes) exposure had little effect. The outer/external A-chains reside predominantly in crystalline domains that form double helices in starch granules, while the inner/internal B-chains are found predominantly in amorphous regions.35 Therefore, it is conceivable that starch modification by microwave chiefly encompasses the disruption of amorphous regions (inner B-chains) in the initial step, and crystalline regions (outer A-chains) mainly in the subsequent steps.34
Furthermore, intense microwave radiation can change the crystallographic construction of starch. A study done by Nawaz et al.36 showed that microwave processing of lotus (Nelumbo nucifera) seed starch led to changes in the morphology from the C-type crystalline edifice to a mixed morphology; for example, a blend of type C and type A, or else a mix of type C and type B crystal structure. Likewise, the crystal organization of microwave-treated potato starch (type B) was converted to type A.37
2.1.1.3 Pulsed electric field (PEF) processing.
Pulsed electric field (PEF) treatment is a physical modification that has gained much interest from researchers due to its green technology and other benefits, such as environmental friendliness, cost-effectiveness, profitability, and energy-saving. PEF processing is primarily done on the liquid materials by passing high-intensity electrical pulses (more than 10 kV cm−1) in a short duration of time (usually microseconds) for carrying out processes like pasteurization, inactivation of enzymes, recovery of biological constituents/extraction, modification at the molecular level, and enhancing the chemical process.1 Furthermore, PEF helps in modifying the morphological characteristics of the starch granules.38 PEF can cause modification in the starch characteristics by reducing the relative crystallinity, gelatinization and enthalpy temperature, viscosity and gelatinization temperature. Relative crystallinity exhibits a noteworthy correlation with the strength of PEF.39 In a recent study, tapioca starch processed with PEF at 30, 40 and 50 kV cm−1 exhibited a decrease (24.2% to 18.1%, 11.4%, and 7.2%, respectively) in the relative crystallinity of the processed tapioca starch.40 On a similar note, the variations in the lamellae structure of starch also show dependence on the electric field strength. This is due to the steady decrease of the scattering peak as the PEF strength increases.39
Additionally, regarding the starch particle morphology, the literature showed that PEF treatment leads to enhanced roughness and formation of cracks, gaps, holes, corrosion or small deposits on the surface of starch granules.1 PEF can modify digestible starch content, although it sustains resistant starch content. Li et al.41 showed that the PEF treatment increased the fraction of resistant starch from 11.73% to 12.24%, and the content of rapidly digested starch increased by 50.88% to 59.40%.
Furthermore, it has been reported that PEF treatment substantially alters the microstructural and macromolecular linkages between biomolecules under non-thermal conditions. In contrast, a recent study by Zeng et al.39 reported that PEF processing was unable to disturb the starch chains. No significant change in the molecular size distribution was observed for waxy rice starch upon PEF processing at 30, 40, and 50 kV cm−1. Regarding the combinational methods of starch modification, PEF-assisted succinylation increased the degree of substitution of modified starch. The pasting temperature at 2–6 kV cm−1 also decreased to 7.6–15.1 °C for PEF-assisted starch esterified with octenyl succinic anhydride. Nonetheless, it was only 3 °C for the non-PEF-treated esterified starch compared with native starch.42 Therefore, PEF can be utilized as an appropriate green processing technology for starch modification.
2.1.1.4 Cold plasma processing.
Plasma processing obeys the simple physical principle that the added energy is supplied to the gas by electric discharge, turning the gas into a high-energy plasma state, which is the fourth state of matter. The plasma is composed of positive and negative charged ions, free electrons, free radicals, and intermediate extremely reactive atoms, molecules, and UV photons having a clear neutral charge.43 These reactive species favor diffusion into the surface, and endure multiple physical and chemical modifications.44 Cold plasma processing of foodstuffs possesses various beneficial effects, such as extended shelf life, increasing germination rate of seeds, shortening of rice cooking time, starch modification, microbial and enzyme inactivation, and changes in hydrophilic/hydrophobic effects.45 The experimental setup utilized in existing studies revealed that the exposure of starches with low-temperature plasma or glow discharge plasma induces starch crosslinking among the existing molecular pattern of starches. It is directly involved with the activation of free radicals, and hence accelerates the leaching of amylose. It also decreases the relative crystallinity because of the depolymerization of the active plasma species. In summary, plasma processing alters the physical and functional aspects of starch (Fig. 4). This technology improves the pasting properties and viscosity, but reduces the tendency of retrogradation.15,43 Cold plasma treatment combined with microwave assistance on starches imparts elevated solubility features, and the same can be attributed to amylose leaching after treatment for hours.46
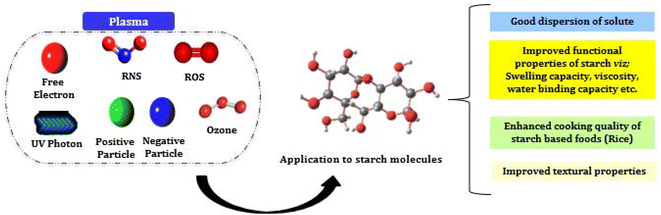 |
| Fig. 4 Effects of plasma treatment on the properties of the starch molecules. | |
Studies have shown that plasma-modified starch may cause little or no change in the starch crystallization morphology. However, it may induce changes in the relative crystallinity.15 Nonetheless, it must be noted that the amorphous region of the starch is more sensitive as compared to the crystalline region for two probable reasons: (a) the branched chains of starch molecules in the amorphous region are organized in a loose manner and easily compatible to interact with the reactive species of plasma,43 and (b) the effect of plasma is usually restricted to the limited nanometer layers inside the starch granules and does not modify the overall activities of starch.47
A literature survey indicated a decline in the relative crystallinity of cassava starch (8% decrease) upon treatment with helium glow plasma, whereas a significant decrease of 12% was observed upon treatment with oxygen glow plasma under identical experimental settings. Correspondingly, Thirumdas et al.15 reported a decrease in the relative crystallinity of rice starch from 43.06% (with no treatment) to 37.47% (40 W, 10 min air plasma treatment). Hence, it appears that the lowering of the relative degree of crystallization is substantially dependent on the type/kind of starch, as well as the plasma type and duration of treatment. Regarding the lamellar structure of starch, Zhang et al.11 observed that helium and nitrogen glow plasma treatment enhanced “d” (which is the lamellae repeat distance) and “dc” (average crystalline lamellae thickness), while it marginally decreased “da” (average amorphous lamellae thickness) in potato-based starch. Moreover, the treatment with glow plasma led to polymerization or crosslinking between the starch molecules. The study also suggested that starch modified with the glow plasma could assist as a potentially highly safe thickening or gelling agent for different food products. Thus, starch processing can be performed by cold plasma as a new technique. However, more studies need to be conducted to verify the applications of plasma-modified starch in current food systems.
2.1.2 Conventional physical starch modification methods.
2.1.2.1 Pre-gelatinization.
Pre-cooking is a physical starch modification technique that turns natural starch from insoluble in water to a fully soluble form at room temperature termed as pre-gelatinized starch or instant starch.48 Pre-gelatinized starch can be employed in ready-to-eat foods without cooking, and also has the benefit of reducing the cooking time. In pregelatinized starch obtained through extrusion, starch granules having moisture levels of 13–20% are exposed to high temperatures (up to 200 °C) along with high pressures (more than 10 MPa), and sheared during the extrusion process. As a result, the starch molecules are severely damaged and decomposed, causing low water absorption and viscosity.49 Yan and Zhengbiao50 also studied the effect of extrusion and drum drying on the morphology of pre-gelatinized starch molecules by scanning electron microscopy (SEM). Granules of pre-gelatinized starch made by extrusion technology showed irregular stones, shapes and holes under 300× magnification (Fig. 5). In contrast, the pregelatinized starch granules formed through the drum drying process showed an irregular laminar structure, while entirely losing the common granular shape (Fig. 6). This is attributable to the higher temperature during the development of pre-gelatinized starch. During the process, degradation of starch occurs and the intermolecular hydrogen bonds are broken by high temperature, which can gelatinize the starch and also destroy its crystallinity.
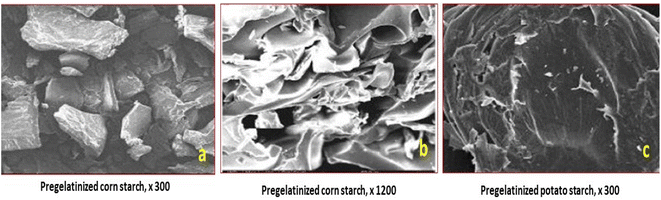 |
| Fig. 5 SEM illustration showing the pre-gelatinized starch from extrusion. | |
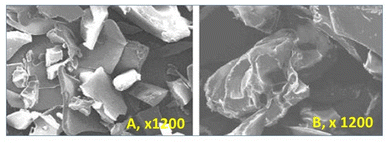 |
| Fig. 6 SEM illustration showing the pre-gelatinized starch from drum drying. | |
Taro starch was adopted for dry heating with carboxymethyl cellulose and sodium alginate. It was then analysed in comparison with potato and sweet potato, and showed remarkable features of high solubility, least transmittance, color diffraction and swelling intensity as ruptured starch granules.51 Banana starch exhibits 50% high water binding properties on treatment with hydroxyl-propyl on exposure to an elevated gelatinization temperature of 90 °C.52
2.1.2.2 Hydro-thermal methods.
Hydro-thermal processing is a method that alters starch, while preserving the intact grain structure. This method involves maintaining the starch molecules in a mobile state above the glass transition temperature (Tg) and below the gelatinization temperature (Tgel).53 Annealing and heat moisture temperature are examples of hydrothermal treatment. Annealing is a hydrothermal treatment of starch granules in the presence of heat and water (40–76% w/w) between the temperature of commencement of gelatinization and the glass transition temperature.54,55 The parameters that are required for annealing to occur are moisture content, time, and temperature. Changes in these conditions alter the scope of treatment. Other properties of modified starch are reduced solubility, retrogradation, increased enzymatic hydrolysis and crystallinity. Heat-moisture treatment is a combination of heat and moisture treatment that changes the physicochemical attributes of starch. This processing method is comparable to annealing, except it is done at low moisture (<35% w/w), usually between 15 minutes and 16 hours, and at 80–140 °C. Heat-moisture treatment modifies the physicochemical properties without destroying the granular structure of starch.54 It also improves the weak functional property of native starch, which contributes to a variety of applications in food industries.
The hydrothermal methods of starch modification show a wide range of effects on the functional properties. Hoover55 reported that the swelling property of starch granules was reduced due to heat-moisture treatment. Chung et al.56 studied the modification of pea, lentil, and navy bean starch by heat-moisture treatment, annealing, and dual modification (heat moisture-treated starch subjected to annealing) & (annealing subjected to heat-moisture treatment). The study showed that both swelling power and amylose leaching of native, annealed, heat-moisture treated and dual-modified pea, lentil and navy bean starch were in the order of pea > lentil > navy bean. The heat moisture treatment decreases the swelling and amylose leaching of starch. In addition, the heat-moisture treated starch subjected to annealing displayed the lowest swelling and amylose leaching. According to a study done by Adebowale et al.,57 the swelling power and solubility of Bambara groundnut starch were reduced by both heat-moisture treatment and annealing treatment. A study by Simsek et al.58 exhibited improvement in the swelling power of black bean starch and pinto bean starch upon annealing treatment.
Regarding the effect of hydrothermal treatment on the gelatinization temperature, it is reported that the enthalpy of gelatinization either remains unchanged or decreases with heat-moisture treatment.55 Simsek et al.58 reported a decrease in the gelatinization temperature range and an increase in the transition temperatures (onset, peak and conclusion temperature) of black bean and pinto bean starches. Annealing also increases gelatinization enthalpy of black bean and pinto bean starches as compared to their native forms. Güzel et al.59 reported a significant increase in the transition temperatures of borlotti bean, white kidney bean, and chickpea on heat-moisture treatment. A significant decrease in gelatinization enthalpy was observed in white kidney bean, yet there was no significant decrease in chickpea and borlotti bean. Legumes, on its exposure through physical modification means (heat-moisture treatment), undergo a significant increase in resistant starch proportion.60
The pasting property of starch is greatly influenced by hydrothermal treatment. With an increase in pasting temperature and thermal stability, a decrease in peak viscosity and either decrease or increase in the setback of starches were reported.55 Likewise, Adebowale et al.57 also stated that the pasting temperature on Bambara groundnut starch increases on heat moisture treatment, whereas annealing has no significant impact on the pasting temperature. The peak viscosity of Bambara groundnut starch is reduced by hydro-thermal treatment. According to Simsek et al.,58 annealing treatment of black bean and pinto bean starch results in lowering the peak viscosity, hot paste viscosity, cold paste viscosity, breakdown and setback. Meanwhile, annealing of bean starch shows an increase in the pasting temperature.
2.2 Chemical processes for modification of starches
The chemical modification of starch involves the transformation of starch, which is usually attained by introducing or blocking a functional group through the processes of etherification, oxidation, esterification, crosslinking, succinylation, and acid thinning. Owing to the presence of abundant hydroxyl groups in the starches, several chemical modifications could be carried out. In comparison with other modification methods like genetic engineering and physical methods, the chemical ways of starch modification offer wide possibilities for starch functionalization. Thus, the range of applications are greatly expanded, particularly due to emergent chemical methods.3 The following section describes some of the frequently used chemical modification techniques of starch (Table 2).
Table 2 Recent studies on the chemical modification of starch
S. no. |
Modification type |
Raw material |
Key findings |
Reference |
1 |
Starch modification by esterification and acid alcohol |
Rice bean (Vigna umbellata) starch |
Alterations in the physicochemical, pasting, particle size, and morphological action of rice bean starch |
Thakur et al. (2021)81 |
Lowering of the amylose content of rice bean starch and swelling power |
2 |
Starch modification by acetylated distarch adipate |
Cassava root starch |
Improved water binding activity (89.69%) and solubility (80.33%) |
Jankar et al. (2020)82 |
3 |
Met extrusion of alkali-treated starch |
High amylose corn starch |
Increase in NaOH-treated starch elongation at break |
Qian et al. (2019)41 |
Improved tensile strength (10.03 MPa) and thickness (0.229 mm) were observed at 2% NaOH treatment |
4 |
Esterification (acetylation) & process |
African star apple fruit starch |
Enhanced oil absorption capacity (2.00%), swelling capacity and solubility |
Ahmed et al. (2019)83 |
Etherification (carboxymethylation) process |
Improved water absorption capacity (1.49%), swelling capacity and solubility |
Succinylation process |
Increased swelling capacity and solubility |
5 |
Succinylation process |
White sorghum (Sorghum bicolor) starch |
Succinyl groups led to improved swelling power |
Mehboob et al. (2015)69 |
Lowered rearrangement of leached-out amylose units |
6 |
Cross-linking (sodium trimetaphosphate and sodium tripolyphosphate) |
Tapioca starch |
Enhanced thermal stability of cross-linked starch |
Wongsagonsup et al. (2014)84 |
Better textural and sensory quality of soups |
2.2.1 Oxidation.
Commercial preparation of oxidized starch includes a reaction between native starch and a specific oxidant under controlled conditions, and results in the introduction of carbonyl and carboxyl groups. The hydroxyl groups contained in starch are sequentially oxidized, first to a carbonyl group and then to a carboxyl group.61–63 Oxidation of starch can be done by using different oxidants, such as hydrogen peroxide, peracetic acid, sodium hypochlorite, potassium permanganate, ozone, nitrogen dioxide, and chromic acid.61 The level of starch oxidized can be determined by the carboxyl and carbonyl content, and the degree of oxidation depends on conditions, such as pH, time of reaction, and the oxidizing agent.63 The oxidizing agents popularly used for the oxidation of starch are sodium hypochlorite and ozone.58,63 Starch oxidation usually reduces its swelling capacity, viscosity during pasting, and enthalpy change during gelatinization, while increasing the melting point of the retrograded starch.
Oxidation processes have a wide impact on the starch properties. Adebowale et al.57 oxidized the starch of Bambara groundnut (Voandzeia subterranean) and reported an increase in water absorption. The water absorption capacity of the native starch form was 2.00 ± 0.10 g g−1, but the oxidized starch showed a water absorption capacity of 2.40 ± 0.01 g g−1. Ogungbenle et al.64 reported that the percentage of water-binding capacity of native starch obtained from pumpkin seeds, white melons, yellow seeds, benni seeds, and bulma cotton seeds were 85, 81, 78, 83, and 80, which when oxidized changed to 96, 94, 92, 90 and 89, respectively. Likewise, Lawal and Adebowale65 also reported on similar results of oxidation on jack bean starch. They concluded that the water absorption capacity of modified starch is much higher than that of the native jack bean starch, and the oxidized jack bean starch showed the highest water absorption capacity. Oladebeye et al.66 oxidized starches obtain from pigeon pea, lima bean, and jack bean by gaseous ozone. Ozone-oxidized starches exhibited higher swelling power than their native forms. The solubility of pigeon pea and lima bean also increased upon ozonation. However, the jack bean exhibited lower solubility after 15 minutes of ozone-generation time.
In the previous research, it has been shown that the oxidation of jack bean starch improves the oil absorption capacity. Modified starch shows a much higher oil absorption capacity than native jack bean starch, and oxidized jack bean starch shows the highest oil absorption capacity compared to other starches.65 Comparable findings were reported for Bambara groundnut starch by Adebowale et al.57 Bambara native starch has 1.76 ± 0.10 oil absorption capacity, which on oxidation shifted to the higher value of 3.04 ± 0.18.
Enthalpy of gelatinization decreases on oxidation of starch. In a study, it was observed that the native jack bean starch exhibited a gelatinization value of 0.75 J g−1, which on oxidation decreased to 0.4 J g−1.65 A study by Wojeicchowski et al.63 found that the enthalpy of gelatinization of oxidized common bean starch is lower than its native form, and the values of onset temperature and mid-point temperature do not change. According to Adebowale et al.,57 the oxidation of starch lowers the gelatinization temperature. Oladebeye et al.66 reported that the highest onset temperature at 5 minutes of ozone generation in lima bean was 76.61 ± 0.06 °C, and that for jack bean was 77.22 ± 0.02 °C. However, it was found that pigeon pea starch had no change in onset temperature. Lima starch also showed a slight difference in conclusion temperature.
Pasting temperature, peak viscosity, hot paste viscosity, and cold paste viscosity of native Bambara groundnut (Voandzeia subterranean) starch were reported to be higher than its oxidized form. Moreover, oxidized Bambara starch showed higher stability and lower setback than native Bambara starch. It was also revealed in previous literature that the setback viscosity decreases on oxidation.57 In contrast to the Bambara starch, the oxidized common bean starch showed higher peak viscosity, hardness, and adhesiveness than the native starch form.63 A study by Lawal and Adebowale65 showed that the pasting temperature, peak viscosity, hot paste viscosity, viscosity after 30 min of holding, and cold paste viscosity of jack bean starch decreased by oxidation. Oladebeye et al.66 reported an upsurge in the pasting properties, such as peak viscosity, cold paste viscosity, and hot paste viscosity of pigeon pea, lima bean and jack bean upon ozonation. With an increase in ozone generation time, the setback viscosity decreased. However, in the case of jack bean at 5 minutes of ozone generation time, the setback viscosity initially increases, but then decreases on further ozone generation time.
Swelling capacity and solubility of starches are usually associated with temperature. The swelling power of all native and oxidized starches increases with an increase in temperature. On oxidation, the swelling power of Bambara groundnut starch decreases and has values lower than its native form. The swelling power of native Bambara groundnut starch at 65 °C was 138.50%. On further heating at 75 °C, 85 °C, and 95 °C, it increases to 249.30%, 456.90%, and 498.70%, respectively. The swelling power of oxidized starch at temperatures of 65 °C, 75 °C, 85 °C, and 95 °C was in the order of 126.30%, 210.30%, 390.90%, and 452.90%, respectively.57 Likewise, Vanier et al.62 reported that upon oxidation, the swelling power of starch granules decreases and the solubility increases. Wojeicchowski et al.63 reported that common bean starch has the maximum swelling power at 90 °C. In a comparison of native and modified common bean starch, dual-modified (oxidized-acetylated) starch showed superior swelling properties over oxidized and native starch. Modified starches have more stability terms, and are known for their high water and oil binding capacities. Native starch and oxidized starch reveal high (26.02 ± 0.04) and low (15.46 ± 0.12)% syneresis, respectively thus able to produce more stable starches.67
Regarding the solubility of starch, it is well known that heating leads to an increase in the solubility of Bambara groundnut starch. The oxidized starch has the highest solubility percentage as compared to other acetylated and modified starches. Studies also showed that hypochlorite oxidation increases solubility by weakening the internal structure of starch granules. According to Adebowale et al.,57 the solubility of oxidized starch is more than that of the native starch. Following the order, the percent solubilities of oxidized and native forms at 65 °C are 2.90% and 2.70%, respectively. Bambara oxidized starch shows the highest solubility of 16.70% at 95 °C. Wojeicchowski et al.63 reported that the solubility of oxidized common bean (Phaseolus vulgaris L.) starch is greater than that of the native forms. The low solubility value of native starch could be due to its gelatinization, which starts at a temperature near 70 °C.
2.2.2 Acetylation.
Acetylation involves the reaction of starch with acetic anhydride and vinyl acetate, and could be done in the presence of an alkaline catalyst (sodium hydroxide), water or organic solvent.61 The amylose content increases steadily with an increase in the degree of substitution of the hydroxyl group of sugar with the acetyl group. The high amylose content of acetylated modified starch may induce a beneficial effect on human health, such as reduction of glycemic load and insulin impact, owing to the fact that amylose gets digested more slowly.68 The United States Food and Drug Administration has authorized a 0.2 substitution level of acetylated starch for applications in the food and pharmaceutical sector. These acetylated starches act as a thickener, binder, stabilizer, texturizer, and film-forming agent. Meanwhile, acetylated starches are used in baked foods, sauces, frozen items, salads, baby food products, and other products.8
Acetylation influences the functional properties of native starch. With the acetylation process, the water absorption capacity of starch also increases. Since the native starch granules are closely interrelated, they have lower water-binding capacity. By the process of acetylation, the acetyl groups are introduced. This may reduce the compactness of the starch polymer, provide steric hindrance, facilitate access of water to the amorphous domain, and increase the water absorption capacity. According to Olagunju et al.,68 the water absorption capacity of native pigeon pea starch was 1.13 mL g−1, which increased to 1.93 mL g−1 on acetylation. Similarly, Adebowale et al.57 reported a water absorption capacity of 2.10 g g−1 in the acetylated Bambara groundnut (Voandzeia subterranean) starch. Ogungbenle et al.64 reported that the percent water-binding capacities of native gourd seed, white melon, yellow seed, benniseed starch, and bulma cotton seed were 85, 81, 78, 83, and 80, which increased to 95, 91, 90, 88 and 86 on acetylation, respectively. Hence, it is well demonstrated that the acetylation process led to the enhancement in the water absorption capacity. The modified starch enhances the water absorption capacity, which may improve the textural property, thickening property bulking, and gelling properties, and thus contribute to increasing the industrial application of legume starch.68
According to Lawal and Adebowale,65 acetylation of jack bean starch improves the oil absorption capacity. On a similar note, Adebowale et al.57 reported an oil absorption capacity of 1.76 ± 0.10 in the native Bambara groundnut starch, which shifted to a higher value of 2.55 ± 0.21 on acetylation.
In the context of gelatinization, the enthalpy of gelatinization (ΔH) decreases on acetylation.65 Native jack bean starch exhibited a gelatinization value of 0.75 J g−1, which decreased to 0.56 J g−1 on acetylation. A study by Wojeicchowski et al.63 stated that in the case of acetylated common bean, the values of ΔH and onset-temperature (To) decrease. According to Adebowale et al.,57 the acetylation process lowers the gelatinization temperature of starches.
The previous research showed that the acetylation process led to a decline in the pasting properties of the starch. Pasting temperature, peak viscosity, hot paste viscosity, and cold paste viscosity of native Bambara starch were observed to be higher than those of its acetylated form. Acetylated common bean starch has the lowest value of breakdown viscosity.63 A study by Lawal and Adebowale65 stated that the pasting temperature, the peak viscosity, hot paste viscosity, viscosity after 30 min of holding, and cold paste viscosity decreased upon acetylation of jack bean starch.
Acetylation of starches led to an upsurge in their swelling properties. Acetylated starch has more swelling power as compared to oxidized starch and native starch.58,63 An increase in heating also increases the solubility of Bambara groundnut starch. As per the study done by Adebowale et al.,57 the solubility of starch is in the order of oxidized > native starch > acetylated. Following the order, the percent solubility of oxidized, native, and acetylated forms at 65 °C was 2.90%, 2.70%, and 2.50%. Bambara oxidized starch exhibited the highest solubility of 16.70% at 95 °C, while the acetylated and native form of Bambara starch has a solubility of 12.50% and 14.59%, respectively, at the same temperature. Wojeicchowski et al.63 observed that in the case of common bean (Phaseolus vulgaris L.), the solubility of native and modified starch is in the order of oxidized – acetylated (dual modified) > oxidized > acetylated > native starch.
2.2.3 Succinylation.
Succinylation is done by esterification of native starch with succinic anhydride. Starch succinylation takes place under alkaline conditions, resulting in the addition of hydrophilic, negatively charged succinyl groups, which give hydrophilicity to the starch. Therefore, starch succinate delivers a variety of attractive properties, such as greater consistency, improved thickening, lower gelatinization temperature, and improved stability during refrigeration/cold storage.69 Succinylation of starches is approved by the United States Food and Drug Administration for use in the food sector with a processing level of 4%. The starch succinates are basically known for their low gelatinization temperature, swelling capacity in cold water, cryogenic stability, outstanding viscosity stability, enhanced clarity of starch paste, low retrogradation effects, as well as their stability for acid/alkali. These properties of starch succinate are an asset for their candidature for several foods or non-food usage. In certain studies, the succinylation process is carried out through octenyl succinic anhydride.70 Starches modified with octenyl succinic anhydride are used to stabilize oil or water-based emulsions. The glucose portion of the starch is bound to water and the lipophilic octenyl part is bound to the oil, thus preventing the parting of the oil and water phases. The succinylation process also impacts the physical and functional characteristics of the native starch. The next section presents some changes in starch upon succinylation.
According to research done by Bushra et al.,71 the swelling power of native mung bean starch is enhanced by succinylation and increases continuously as the temperature increases. A decreasing solubility trend was reported with the effect of succinylation upon the increase in temperature. Bushra et al.71 stated that the water absorption capacity of native mung bean starch increases following succinylation. By the introduction of a succinyl group, there is a reduction in the starch retrogradation, which leads to improvement in the water holding capacity and water binding capacity. The oil absorption capacity of native mung bean starch also decreases on succinylation.71
On succinylation, the transition temperatures (onset, peak and conclusion temperature) of native mung bean starch decrease. Enthalpy of gelatinization also decreases on succinylation of mung bean.71 Acetylation and succinylation in comparison to their application reformation among taro starch brings about a decrease in viscosity and increase in viscosity characteristics, respectively.72 Octenyl-succinylated anhydride esterification improves emulsifying characteristics, and is suited well to A-type starches provided with the least degree of substitutions.73
2.2.4 Acid thinning.
Acid-thinned starch is prepared by treating a concentrated slurry of native starch with an acid, such as hydrochloric acid, nitric acid and sulphuric acid, below the gelatinization temperature for a specific time depending upon the viscosity or degree of conversion.61 The starches employed for the preparation of gum candies are often treated with hydrochloric acid, and therefore are known as thinned starches. This type of starch is widely utilized in the food, textile, as well as paper industries. During acid hydrolysis, the hydroxonium ion reacts with the oxygen atom of the glycosidic bond and then hydrolyses the bond. Before entering the starch interior, an acid first acts on the surface of the starch granules. Acidic modifications substantially alter the structural and functional effects of starch without affecting its grain morphology.8
According to Lawal and Adebowale,65 the water absorption capacity of native jack bean starch is reduced by following acid thinning treatment. This may be due to an increase in crystallinity, which probably restricts the entry of water into granules of acid-thinned starch. The study also revealed that the acid thinning of jack bean starch causes a reduction in the oil absorption capacity of native jack bean starch.
In a study, the pasting temperature of native jack bean starch increased, but the peak viscosity, hot paste viscosity, viscosity after 30 min of holding, and cold paste viscosity decreased on acid thinning. The setback value also increases on acid thinning. Citric acid addition among banana starch carry out gelatinized modification in them with enhanced functional attributes hence make their way to consume it as routine meal to reduce body fat and also to favor the healthy microflora count.74
2.2.5 Cross-linking.
Cross-linked starch is rigid with low swelling power and solubility, and can withstand severe processing conditions without breaking. Sodium trimetaphosphate (STMP), sodium tripolyphosphate (STPP), epichlorohydrin (ECH), and phosphoryl chloride (POCl3) are the commonly used cross-linking agents. During the crosslinking process, the new covalent bonds are introduced with pre-existing hydrogen bonds between the starch particles. Crosslinking leads to improved acid, heat, and mechanical resistance of starch.75 Cross-linking of carboxymethyl starches with multifunctional carboxylic acids, for example citric, tartaric, malic, and many more offers a significant swelling capacity, which is helpful in forming stable hydrogels. The strength of cross-linked starches is based on the length of the spacer and the presence of functional groups. Among all, citric acid forms the utmost distinct network structure.76
Cross-linking affects the functional properties of the native starch. Cross-linked starch has low solubility because of the reinforcement of the structure starch granules, which limits the mobility of the starch chain in the amorphous region and limits the water absorption capacity.61 Cross-linking also decreases the water-binding capacity of legume starch.62
A group of researchers studied the cross-linking of the kudzu starch (a vine of the leguminous plant) by treating it with sodium tri-metaphosphate at varying time, temperature, and concentration, where the swelling capacity and solubility of the modified starch decrease as the degree of cross-linking increases compared to the native form. A reduction in the swelling property of starch may occur due to structural rearrangement of amylose and amylopectin, and a decrease in solubility may be due to the fact that the cross-linking bonds are stronger than the hydrogen bonds. As a result, the granules of starch become hard to dissolve.77 Likewise, Sharma et al.75 also mentioned a decrease in both swelling capacity and solubility on crosslinking of faba bean starch by STMP, while the swelling capacity and solubility decrease progressively with an increase in cross-linking. According to Güzel et al.59 there is a decrease in the swelling power, but an increase in the solubility of borlotti bean, white kidney bean, and chickpea starch upon cross-linking.
Cross-linking of starch leads to an increase in the starch transition temperatures (onset, peak, and conclusion temperature) and enthalpy of gelatinization.75 On a similar note, Güzel et al.59 reported a significant upsurge in the onset gelatinization temperature of borlotti bean, chickpea bean, and white kidney bean on STMP cross-linking. Peak and conclusion temperature also increase on crosslinking, but the gelatinization enthalpy of all three starches decreases on cross-linking. With the addition of STMP, the retrogradation property of cross-linked starch increases. Native kudzu starch was reported to take 48 h for its maximum retrogradation, while modified kudzu starch takes 12 h to reach the maximum.77
In a recent study, it was reported that the cross-linked kudzu starch has high freeze–thaw stability and a lower syneresis rate.77 It was also stated that the cross-linked starch with the addition of STMP (12%) at a reaction temperature of 55 °C could be frozen and thawed three times.
Peak viscosity and breakdown viscosity of faba bean starch decrease on cross-linking treatment. Studies have reported that with an increasing degree of crosslinking treatment, the peak viscosity decreases. However, the final viscosity and setback of native starch increased at lower levels of crosslinking, while the starch shrinkage of native faba bean starch decreased as the degree of crosslinking increased from 1% STMP. An increase in pasting temperature is observed as the level of cross-linking increases.75
3 Conclusion
Starch is one of the vital yet versatile food ingredients, possessing value-added properties for a multitude of industrial usages both in food and non-food sectors. Innovative functional and value-added properties boosted among modified starches. Modified achievements in starch through thermal application induce transition characteristics from amorphous to crystalline, resulting in its gelatinization. Etherification, oxidation, esterification, crosslinking, succinylation and acid thinning are the processes involving the introduction or blocking of functional groups under chemically driven modification. Furthermore, physical modification of starch carries out changes in the properties of starch resulting from physical processing. Pre-gelatinization, annealing and heat moisture temperature treatments are some conventional physical treatments, while some non-conventional treatments, viz., high hydrostatic pressure (HHP) processing, microwave processing, pulsed electric field (PEF) processing, and cold plasma technology provide environmentally friendly alternatives to the starch modification. These green technologies can produce starch with broadened properties that are useful in food and non-food fields. The present review summarizes the physical and chemical techniques adopted on starch isolated from various sources, and revealed significant variations in properties that benefit society. However, these novel physical approaches that carry out starch alterations in turn possess broad application traits. These highlighted practices even demand much more exploration in terms of the optimization of the process parameters, revealing more sources for starch, and application extension to pharmaceuticals and textiles. To some other miscellaneous fields, they still demand more research studies in the same direction.
Conflicts of interest
There are no conflicts to declare.
References
- Z. Han, R. Shi and D. W. Sun, Effects of novel physical processing techniques on the multi-structures of starch, Trends Food Sci. Technol., 2020, 97, 126–135 CrossRef CAS.
-
T. V. Singh and L. S. Shagolsem, Biopolymer based nano-structured materials and their applications, Nanostructured Materials and their Applications, 2021, pp. 337–366 Search PubMed.
- Y. Fan and F. Picchioni, Modification of starch: A review on the application of “green” solvents and controlled functionalization, Carbohydr. Polym., 2020, 241, 116350 CrossRef CAS PubMed.
- B. C. Maniglia, G. Pataro, G. Ferrari, P. E. Augusto, P. Le-Bail and A. Le-Bail, Pulsed electric fields (PEF) treatment to enhance starch 3D printing application: effect on structure, properties, and functionality of wheat and cassava starches, Innovative Food Sci. Emerging Technol., 2021, 68, 102602 CrossRef.
- J. Coria-Hernández, A. Méndez-Albores, R. Meléndez-Pérez, M. E. Rosas-Mendoza and J. L. Arjona-Román, Thermal, structural, and rheological characterization of waxy starch as a cryogel for its application in food processing, Polymers, 2018, 10(4), 359 CrossRef PubMed.
- Á. L. Santana and M. A. Meireles, New starches are the trend for industry applications: a review, Food and Public Health, 2014, 4(5), 229–241 CrossRef.
- B. Kaur, F. Ariffin, R. Bhat and A. A. Karim, Progress in starch modification in the last decade, Food Hydrocolloids, 2012, 26(2), 398–404 CrossRef CAS.
- S. Punia, Barley starch modifications: Physical, chemical and enzymatic-A review, Int. J. Biol. Macromol., 2020, 144, 578–585 CrossRef CAS PubMed.
- R. N. Tharanathan, Starch—value addition by modification, Crit. Rev. Food Sci. Nutr., 2005, 45(5), 371–384 CrossRef CAS PubMed.
- L. Kaur, S. B. Dhull, P. Kumar and A. Singh, Banana starch: Properties, description, and modified variations-A review, Int. J. Biol. Macromol., 2020, 165, 2096–2102 CrossRef CAS PubMed.
- B. Zhang, L. Chen, X. Li, L. Li and H. Zhang, Understanding the multi-scale structure and functional properties of starch modulated by glow-plasma: A structure-functionality relationship, Food Hydrocolloids, 2015, 50, 228–236 CrossRef CAS.
-
J. M. Milani and A. Golkar, Starch modification by novel technologies and their functionality, in Food Structure and Functionality, Academic Press, 2021, pp. 157–179 Search PubMed.
- H. Nawaz, R. Waheed, M. Nawaz and D. Shahwar, Physical and chemical modifications in starch structure and reactivity, Chem. Prop. Starch, 2020 Mar 11, 9, 13–35 Search PubMed.
-
J. N. BeMiller, Physical modification of starch, in Starch in Food, Woodhead Publishing, 2018, pp. 223–253 Search PubMed.
- R. Thirumdas, A. Trimukhe, R. R. Deshmukh and U. S. Annapure, Functional and rheological properties of cold plasma treated rice starch, Carbohydr. Polym., 2017, 157, 1723–1731 CrossRef CAS PubMed.
- X. P. Hu, B. Zhang, Z. Y. Jin, X. M. Xu and H. Q. Chen, Effect of high hydrostatic pressure and retrogradation treatments on structural and physicochemical properties of waxy wheat starch, Food Chem., 2017, 232, 560–565 CrossRef CAS PubMed.
- M. R. Song, S. H. Choi, S. M. Oh, H. Y. Kim, J. E. Bae, C. S. Park, B. Y. Kim and M. Y. Baik, Characterization of amorphous granular starches prepared by high hydrostatic pressure (HHP), Food Sci. Biotechnol., 2017, 26, 671–678 CrossRef CAS PubMed.
- W. Li, F. Zhang, P. Liu, Y. Bai, L. Gao and Q. Shen, Effect of high hydrostatic pressure on physicochemical, thermal and morphological properties of mung bean (Vigna radiata L.) starch, J. Food Eng., 2011, 103(4), 388–393 CrossRef CAS.
- L. M. Castro, E. M. Alexandre, J. A. Saraiva and M. Pintado, Impact of high pressure on starch properties: A review, Food Hydrocolloids, 2020 Sep 1, 106, 105877 Search PubMed.
- S. Kim, S. Y. Yang, H. H. Chun and K. B. Song, High hydrostatic pressure processing for the preparation of buckwheat and tapioca starch films, Food Hydrocolloids, 2018, 81, 71–76 CrossRef CAS.
- J. Wang, H. Zhu, S. Li, S. Wang, S. Wang and L. Copeland, Insights into structure and function of high pressure-modified starches with different crystalline polymorphs, Int. J. Biol. Macromol., 2017, 102, 414–424 CrossRef CAS PubMed.
- H. Liu, L. Wang, R. Cao, H. Fan and M. Wang, In vitro digestibility and changes in physicochemical and structural properties of common buckwheat starch affected by high hydrostatic pressure, Carbohydr. Polym., 2016, 144, 1–8 CrossRef CAS PubMed.
- H. Liu, H. Fan, R. Cao, C. Blanchard and M. Wang, Physicochemical properties and in vitro digestibility of sorghum starch altered by high hydrostatic pressure, Int. J. Biol. Macromol., 2016b, 92, 753–760 Search PubMed.
- T. Liu, Y. Ma, S. Xue and J. Shi, Modifications of structure and physicochemical properties of maize starch by γ-irradiation treatments, LWT--Food Sci. Technol., 2012, 46(1), 156–163 CrossRef CAS.
- L. A. Conde, B. Kebede, S. Y. Leong and I. Oey, Effect of High Hydrostatic Pressure Processing on Starch Properties of Cassava Flour, Appl. Sci., 2022, 12(19), 10043 CrossRef CAS.
- S. B. Dhull, A. Chandak, M. N. Collins, S. P. Bangar, P. Chawla and A. Singh, Lotus seed starch: a novel functional ingredient with promising properties and applications in food—a review, Starch/Staerke, 2022, 74(9–10), 2200064 CrossRef CAS.
- K. Zhao, B. Li, M. Xu, L. Jing, M. Gou, Z. Yu, J. Zheng and W. Li, Microwave pretreated esterification improved the substitution degree, structural and physicochemical properties of potato starch esters, LWT, 2018, 90, 116–123 CrossRef CAS.
- S. Rahmati, A. Abdullah and O. L. Kang, Effects of different microwave intensity on the extraction yield and physicochemical properties of pectin from dragon fruit (Hylocereus polyrhizus) peels, Bioact. Carbohydr. Diet. Fibre, 2019, 18, 100186 CrossRef CAS.
- M. Braşoveanu and M. R. Nemţanu, Behaviour of starch exposed to microwave radiation treatment, Starch/Staerke, 2014, 66(1–2), 3–14 CrossRef.
- Y. Xie, M. Yan, S. Yuan, S. Sun and Q. Huo, Effect of microwave treatment on the physicochemical properties of potato starch granules, Chem. Cent. J., 2013, 7(1), 1–7 CrossRef PubMed.
- S. A. Oyeyinka, E. Umaru, S. J. Olatunde and J. K. Joseph, Effect of short microwave heating time on physicochemical and functional properties of Bambara groundnut starch, Food Biosci., 2019, 28, 36–41 CrossRef CAS.
- N. Mollekopf, K. Treppe, P. Fiala and O. Dixit, Vacuum microwave treatment of potato starch and the resultant modification of properties, Chem. Ing. Tech., 2011, 83(3), 262–272 CrossRef CAS.
- S. Ma, D. Fan, L. Wang, H. Lian, J. Zhao, H. Zhang and W. Chen, The impact of microwave heating on the granule state and thermal properties of potato starch, Starch/Staerke, 2015, 67(5–6), 391–398 CrossRef CAS.
- Q. Yang, L. Qi, Z. Luo, X. Kong, Z. Xiao, P. Wang and X. Peng, Effect of microwave irradiation on internal molecular structure and physical properties of waxy maize starch, Food Hydrocolloids, 2017, 69, 473–482 CrossRef CAS.
- T. Xia, M. Gou, G. Zhang, W. Li and H. Jiang, Physical and structural properties of potato starch modified by dielectric treatment with different moisture content, Int. J. Biol. Macromol., 2018, 118, 1455–1462 CrossRef CAS PubMed.
- H. Nawaz, M. A. Shad, S. Saleem, M. U. Khan, U. Nishan, T. Rasheed, M. Bilal and H. M. Iqbal, Characteristics of starch isolated from microwave heat treated lotus (Nelumbo nucifera) seed flour, Int. J. Biol. Macromol., 2018, 113, 219–226 CrossRef CAS PubMed.
- X. Xu, Y. Chen, Z. Luo and X. Lu, Different variations in structures of A-and B-type starches subjected to microwave treatment and their relationships with digestibility, LWT, 2019, 99, 179–187 CrossRef CAS.
- D. Niu, X. A. Zeng, E. F. Ren, F. Y. Xu, J. Li, M. S. Wang and R. Wang, Review of the application of pulsed electric fields (PEF) technology for food processing in China, Food Res. Int., 2020, 137, 109715 CrossRef CAS PubMed.
- F. Zeng, Q. Y. Gao, Z. Han, X. A. Zeng and S. J. Yu, Structural properties and digestibility of pulsed electric field treated waxy rice starch, Food Chem., 2016, 194, 1313–1319 CrossRef CAS PubMed.
- Z. Han, X. A. Zeng, N. Fu, S. J. Yu, X. D. Chen and J. F. Kennedy, Effects of pulsed electric field treatments on some properties of tapioca starch, Carbohydr. Polym., 2012, 89(4), 1012–1017 CrossRef CAS PubMed.
- Q. Li, Q. Y. Wu, W. Jiang, J. Y. Qian, L. Zhang, M. Wu, S. Q. Rao and C. S. Wu, Effect of pulsed electric field on structural properties and digestibility of starches with different crystalline type in solid state, Carbohydr. Polym., 2019, 207, 362–370 CrossRef CAS PubMed.
- B. R. Chen, Q. H. Wen, X. A. Zeng, R. Abdul, U. Roobab and F. Y. Xu, Pulsed electric field assisted modification of octenyl succinylated potato starch and its influence on pasting properties, Carbohydr. Polym., 2021, 254, 117294 CrossRef CAS PubMed.
- C. Sarangapani, Y. Devi, R. Thirundas, U. S. Annapure and R. R. Deshmukh, Effect of low-pressure plasma on physico-chemical properties of parboiled rice, LWT--Food Sci. Technol., 2015, 63(1), 452–460 CrossRef CAS.
- M. Mozetič, Discharge cleaning with hydrogen plasma, Vacuum, 2001, 61(2–4), 367–371 CrossRef.
- S. Punia Bangar, S. Suri, P. Nayi and Y. Phimolsiripol, Cold plasma for microbial safety: Principle, mechanism, and factors responsible, J. Food Process. Preserv., 2022, e16850 CAS.
- X. Sun, A. S. Saleh, Z. Sun, X. Ge, H. Shen, Q. Zhang, X. Yu, L. Yuan and W. Li, Modification of multi-scale structure, physicochemical properties, and digestibility of rice starch via microwave and cold plasma treatments, LWT, 2022, 153, 112483 CrossRef CAS.
- S. Banura, R. Thirumdas, A. Kaur, R. R. Deshmukh and U. S. Annapure, Modification of starch using low pressure radio frequency air plasma, LWT, 2018, 89, 719–724 CrossRef CAS.
-
Starch in Food: Structure, Function and Applications, ed. A. C. Eliasson, CRC Press, 2004 Search PubMed.
- M. Sauceau, J. Fages, A. Common, C. Nikitine and E. Rodier, New challenges in polymer foaming: A review of extrusion processes assisted by supercritical carbon dioxide, Prog. Polym. Sci., 2011, 36(6), 749–766 CrossRef CAS.
- H. Yan and G. U. Zhengbiao, Morphology of modified starches prepared by different methods, Food Res. Int., 2010, 43(3), 767–772 CrossRef CAS.
- D. Singla, A. Singh, S. B. Dhull, P. Kumar, T. Malik and P. Kumar, Taro starch: Isolation, morphology, modification and novel applications concern-a review, Int. J. Biol. Macromol., 2020, 163, 1283–1290 CrossRef CAS PubMed.
- K. N. Waliszewski, M. A. Aparicio, L. A. Bello and J. A. Monroy, Changes of banana starch by chemical and physical modification, Carbohydr. Polym., 2003, 52(3), 237–242 CrossRef CAS.
- S. C. Alcázar-Alay and M. A. Meireles, Physicochemical properties, modifications and applications of starches from different botanical sources, Food Sci. Technol., 2015, 35, 215–236 CrossRef.
- A. O. Ashogbon and E. T. Akintayo, Recent trend in the physical and chemical modification of starches from different botanical sources: a review, Starch/Staerke, 2014, 66(1–2), 41–57 CrossRef CAS.
- R. Hoover, The impact of heat-moisture treatment on molecular structures and properties of starches isolated from different botanical sources, Crit. Rev. Food Sci. Nutr., 2010, 50(9), 835–847 CrossRef CAS PubMed.
- H. J. Chung, Q. Liu and R. Hoover, Effect of single and dual hydrothermal treatments on the crystalline structure, thermal properties, and nutritional fractions of pea, lentil, and navy bean starches, Food Res. Int., 2010, 43(2), 501–508 CrossRef CAS.
- K. O. Adebowale, T. A. Afolabi and O. S. Lawal, Isolation, chemical modification and physicochemical characterisation of Bambarra groundnut (Voandzeia subterranean) starch and flour, Food Chem., 2002, 78(3), 305–311 CrossRef CAS.
- S. Simsek, M. Ovando-Martínez, K. Whitney and L. A. Bello-Pérez, Effect of acetylation, oxidation and annealing on physicochemical properties of bean starch, Food Chem., 2012, 134(4), 1796–1803 CrossRef CAS PubMed.
- D. Güzel and S. Sayar, Digestion profiles and some physicochemical properties of native and modified borlotti bean, chickpea and white kidney bean starches, Food Res. Int., 2010, 43(8), 2132–2137 CrossRef.
- T. A. Afolabi, A. O. Opara, S. O. Kareem and F. O. Oladoyinbo,
In vitro digestibility of hydrothermally modified Bambara groundnut (Vigna subterranean L.) starch and flour, Food Sci. Nutr., 2018, 6(1), 36–46 CrossRef CAS PubMed.
- A. O. Ashogbon, E. T. Akintayo, A. O. Oladebeye, A. D. Oluwafemi, A. F. Akinsola and O. E. Imanah, Developments in the isolation, composition, and physicochemical properties of legume starches, Crit. Rev. Food Sci. Nutr., 2021, 61(17), 2938–2959 CrossRef CAS PubMed.
- N. L. Vanier, E. da Rosa Zavareze, V. Z. Pinto, B. Klein, F. T. Botelho, A. R. Dias and M. C. Elias, Physicochemical, crystallinity, pasting and morphological properties of bean starch oxidised by different concentrations of sodium hypochlorite, Food Chem., 2012, 131(4), 1255–1262 CrossRef CAS.
- J. P. Wojeicchowski, G. L. Siqueira, L. G. Lacerda, E. Schnitzler and I. M. Demiate, Physicochemical, structural and thermal properties of oxidized, acetylated and dual-modified common bean (Phaseolus vulgaris L.) starch, Food Sci. Technol., 2018, 38, 318–327 CrossRef.
- H. N. Ogungbenle, Effect of chemical modification on starch of some legume flours, Pak. J. Nutr., 2007, 6(2), 167–171 CrossRef.
- O. S. Lawal and K. O. Adebowale, Physicochemical characteristics and thermal properties of chemically modified jack bean (Canavalia ensiformis) starch, Carbohydr. Polym., 2005, 60(3), 331–341 CrossRef CAS.
- A. O. Oladebeye, A. A. Oshodi, I. A. Amoo and A. A. Karim, Gaseous ozonation of pigeon pea, lima bean, and jack bean starches: functional, thermal, and molecular properties, Starch/Staerke, 2018, 70(11–12), 1700367 CrossRef.
- K. Nakkala, S. Godiyal, S. K. Ettaboina and K. S. Laddha, Chemical modifications of turmeric starch by Oxidation, Phosphorylation, and Succinylation, Starch/Staerke, 2022, 74(9–10), 2200053 CrossRef CAS.
- A. I. Olagunju, O. S. Omoba, V. N. Enujiugha, R. A. Wiens, K. M. Gough and R. E. Aluko, Influence of acetylation on physicochemical and morphological characteristics of pigeon pea starch, Food Hydrocolloids, 2020, 100, 105424 CrossRef CAS.
- S. Mehboob, T. M. Ali, F. Alam and A. Hasnain, Dual modification of native white sorghum (Sorghum bicolor) starch via acid hydrolysis and succinylation, LWT--Food Sci. Technol., 2015, 64(1), 459–467 CrossRef CAS.
- A. García-Gurrola, S. Rincón, A. A. Escobar-Puentes, A. Zepeda, J. F. Pérez-Robles and F. Martínez-Bustos, Synthesis and succinylation of starch nanoparticles by means of a single step using sonochemical
energy, Ultrason. Sonochem., 2019, 56, 458–465 CrossRef PubMed.
- M. Bushra, X. X. Yun, S. Y. Pan, A. Hydamaka, M. W. Hua and W. L. Feng, Effect of oxidation and esterification on functional properties of mung bean (Vigna radiata (L.) Wilczek) starch, Eur. Food Res. Technol., 2013, 236, 119–128 CrossRef CAS.
- D. E. Mweta, M. T. Labuschagne, S. Bonnet, J. Swarts and J. D. Saka, Isolation and physicochemical characterisation of starch from cocoyam (Colocasia esculenta) grown in Malawi, J. Sci. Food Agric., 2010, 90(11), 1886–1896 CAS.
- Q. Wang, Z. Tang, Z. Li, Y. Luan, C. Gu, R. Liu, Q. Ge, H. Yu and M. Wu, Effects of octenyl succinylation on the properties of starches with distinct crystalline types and their Pickering emulsions, Int. J. Biol. Macromol., 2023, 123183 CrossRef CAS PubMed.
- T. Y. Wu, S. J. Tsai, N. N. Sun, F. J. Dai, P. H. Yu, Y. C. Chen and C. F. Chau, Enhanced thermal stability of green banana starch by heat-moisture treatment and its ability to reduce body fat accumulation and modulate gut microbiota, Int. J. Biol. Macromol., 2020, 160, 915–924 CrossRef CAS PubMed.
- V. Sharma, M. Kaur, K. S. Sandhu and S. K. Godara, Effect of cross-linking on physico-chemical, thermal, pasting, in vitro digestibility and film forming properties of Faba bean (Vicia faba L.) starch, Int. J. Biol. Macromol., 2020, 159, 243–249 CrossRef CAS PubMed.
- C. Seidel, W. M. Kulicke, C. Heß, B. Hartmann, M. D. Lechner and W. Lazik, Influence of the cross-linking agent on the gel structure of starch derivatives, Starch/Staerke, 2001, 53(7), 305–310 CrossRef CAS.
- B. Chen, L. Dang, X. Zhang, W. Fang, M. Hou, T. Liu and Z. Wang, Physicochemical properties and micro-structural characteristics in starch from kudzu root as affected by cross-linking, Food Chem., 2017, 219, 93–101 CrossRef CAS PubMed.
- Z. Guo, S. Zeng, X. Lu, M. Zhou, M. Zheng and B. Zheng, Structural and physicochemical properties of lotus seed starch treated with ultra-high pressure, Food Chem., 2015, 186, 223–230 CrossRef CAS PubMed.
- J. Ahmed, N. Al-Ruwaih, M. Mulla and M. H. Rahman, Effect of high pressure treatment on functional, rheological and structural properties of kidney bean protein isolate, LWT, 2018, 91, 191–197 CrossRef CAS.
- J. Hong, X. A. Zeng, Z. Han and C. S. Brennan, Effect of pulsed electric fields treatment on the nanostructure of esterified potato starch and their potential glycemic digestibility, Innovative Food Sci. Emerging Technol., 2018, 45, 438–446 CrossRef CAS.
- Y. Thakur, R. Thory, K. S. Sandhu, M. Kaur, A. Sinhmar and A. K. Pathera, Effect of selected physical and chemical modifications on physicochemical, pasting, and morphological properties of underutilized starch from rice bean (Vigna umbellata), J. Food Sci. Technol., 2021, 1–10 Search PubMed.
- J. Jankar, V. N. Pawar, A. K. Sharma, A. K. Sahoo and R. C. Ranveer, Evaluation of physicochemical properties of guar gum-antioxidants emulsion coatings, Int. J. Mech. Prod. Eng. Res. Dev., 2020, 10(3), 6985–6992 Search PubMed.
- I. A. Ahmed, S. N. Olanrewaju, Y. S. Tolulope, O. A. Lawrence, B. O. Aderanti and O. O. Mary, Effects of chemical modification on functional and physical properties of African star apple kernel (Chrysophyllum albidnum) starch, Afr. J. Pure Appl. Chem., 2019, 13(1), 1–11 CrossRef CAS.
- R. Wongsagonsup, T. Pujchakarn, S. Jitrakbumrung, W. Chaiwat, A. Fuongfuchat, S. Varavinit, S. Dangtip and M. Suphantharika, Effect of cross-linking on physicochemical properties of tapioca starch and its application in soup product, Carbohydr. Polym., 2014, 101, 656–665 CrossRef CAS PubMed.
|
This journal is © The Royal Society of Chemistry 2023 |
Click here to see how this site uses Cookies. View our privacy policy here.