Carnauba wax-based sustainable coatings for prolonging postharvest shelf-life of citrus fruits†
Received
30th November 2022
, Accepted 17th March 2023
First published on 22nd March 2023
Abstract
Citrus fruits are extremely popular for their rich nutritional attributes and health benefits, but they are perishable in nature, and growers and suppliers often face financial losses due to rapid postharvest decay in the fruits. In this study, carnauba wax (CW) based sustainable active coatings were developed, characterized and applied for postharvest shelf-life extension of Khasi mandarin and sweet lemon. A neem oil nanoemulsion (NONE) was used as the active agent to improve antimicrobial properties of the composite coatings. The developed coatings reduced weight loss, maintained firmness, retained total soluble solids, maintained titratable acidity, and slowed down deterioration in coated/treated Khasi mandarin and sweet lemon during storage under ambient conditions. Due to antimicrobial properties of the NONE, the composite coatings containing a 5% NONE (CW/NONE-5) and 10% NONE (CW/NONE-10) significantly reduced decay in the coated fruits compared to the uncoated control and extended the shelf-life of the citrus fruits by at least 3 weeks. The developed active composite coatings can be effective alternatives to synthetic wax coating for shelf-life extension of citrus fruits and may provide growers and suppliers with a sustainable mitigation strategy to minimize their postharvest losses.
Introduction
Citrus fruits are members of the Rutaceae family and are grown in temperate and tropical climates around the world. Citrus fruits are very popular because of their pleasant flavour and because they are rich sources of vitamin C and essential minerals including potassium, iron, calcium, phosphorus and magnesium.1,2 Among citrus fruits, Khasi mandarin (Citrus reticulata Blanco) is commonly grown in the North Eastern regions of India with an annual production of about 673 thousand metric tons,3 whereas, India is the largest producer of sweet lemon (Citrus limetta Risso) with an annual production of about 4 million metric tons, which is almost 65% of the total global production.4 However, these citrus fruits are perishable having about 1–2 weeks of postharvest shelf-life, and their quality deteriorates mainly due to fungal and bacterial spoilage, which leads to significant economic losses for growers and suppliers.5 For instance, blue rot and green rot in citrus fruits are caused by fungi, namely Penicillium italicum and Penicillium digitatum, respectively, whereas Listeria monocytogenes and Alicyclobacillus spp. cause bacterial spoilage in the fruits.6 Different postharvest preservation methods such as low-temperature storage, synthetic wax-coating, chemical treatment, and modified atmospheric storage/packaging have been used to extend their shelf-life.7,8 However, low-temperature and modified atmosphere storage are costly and inconvenient methods, while synthetic wax-coating and chemical treatment are harmful for consumers and the environment, and thus, numerous efforts have been made to develop sustainable and edible coatings as effective alternatives to these synthetic, costly, and inconvenient counterparts.9,10
Carnauba wax (CW) is extracted from the leaves of Copernicia prunifera, a species of palm tree native to Brazil, and the wax mainly consists of aliphatic esters and cinnamic acid diesters having high melting points (82–86 °C) and low solubility.11 Carnauba wax has inherent moisture barrier properties and antifungal activities and is recognized as a generally recognised as safe (GRAS) substance by the United States Food and Drug Administration.11,12 Coatings of CW alone or incorporated with active agents have been applied effectively to preserve or extend the shelf-life of egg plant,13 golden papaya,14 satsuma mandarin,15 and Valencia orange,.16 Carnauba wax coating of fruits and vegetables reduces transpiration and respiration by acting as a semipermeable barrier to gases and water vapour thereby preventing moisture loss, maintaining firmness and other qualities, delaying repining and senescence, and thus extending their shelf-life.17 Active antimicrobial and antioxidant agents such as essential oils may improve functional properties of CW coating.18
Neem oil is an essential oil obtained from the seeds of the neem plant (Azadirachta indica) and has been used for centuries due to its excellent antibacterial, antifungal, antioxidant and health-promoting properties.19,20 However, its applications in food packaging and preservation are limited due to photosensitivity of neem oil and its impacts on the sensory attributes of food. To address these challenges, neem oil can be nano-emulsified to improve bioactivity, bioavailability, and photo-stability. A nanoemulsion of neem oil has been reported to be effective against several phytopathogenic fungi including Rhizoctonia solani and Sclerotium rolfsii.21 Besides having increased functionalities, a neem oil nanoemulsion (NONE) can also be used for targeted delivery along with controlled release, leading to more effective applications in active food packaging and preservation.22,23 Thus, a NONE is a promising biodegradable and non-toxic agent that can be incorporated in CW coating to make it more effective for coating of fruits and vegetable. Therefore, in this study, a superior and active coating formulations from carnauba wax and NONEs were prepared, characterized, and applied on two citrus fruits i.e., Khasi mandarin and sweet lemon as sustainable coating for their postharvest shelf-life extension.
Materials and methods
Chemicals and raw materials
The materials used in the study were neem seed oil (Himedia Laboratory Pvt. Ltd., India), Tween 80 (Merck Life Science Pvt. Ltd., India), carnauba wax No-1 yellow (Sigma-Aldrich), shellac flakes from Lacca (Himedia Laboratory Pvt. Ltd., India), sweet lemon (Citrus limetta) collected from Karigaon located in Kokrajhar district in the State of Assam, India, and Khasi mandarin (Citrus reticulata) collected from Ngunraw village of South West Khasi Hills, Meghalaya, India.
Preparation and characterization of neem oil nanoemulsions (NONEs)
Neem oil nanoemulsions (NONEs) were prepared by adopting the method reported by de Castro e Silva et al., 2019 with a slight modification, in which neem oil and Tween 80 were used in different weight ratios of 1
:
1, 1
:
2, and 1
:
3.24 Initially, Tween 80 was added dropwise to the distilled water at room temperature with stirring at 500 rpm for 30 min on a magnetic stirrer (REMI, Mumbai, India). Afterward, neem oil was added dropwise to the Tween 80 solution while stirring continuously at 1000 rpm for 15 min. Each prepared emulsion was then sonicated using an ultrasonic processor (Vibra cell VCX-750, Sonics & Materials, Inc., Newton, USA) for 10 min at 30 °C and 20 kHz with a fixed amplitude of 40% to make nanoemulsions. The prepared nanoemulsions with ratios of 1
:
1, 1
:
2, and 1
:
3 of neem oil and Tween-80 were termed NONE-1, NONE-2, and NONE-3, respectively.
The pH of the prepared NONEs was measured using a calibrated pH meter (EUTECH ION 2700, Thermo Fisher Scientific India Pvt. Ltd, Mumbai, India) at room temperature (25 °C). The viscosity of the NONEs was determined using a viscometer, (DV3T, Brookfield Ametek, USA) at room temperature using a cylindrical spindle of LV6 at 80 rpm, and the results were recorded in cP (centipoise). The turbidity of the prepared NONEs was assessed by measuring the transmittance of undiluted samples at 600 nm using a UV-vis spectrophotometer (2375 Double Beam, Electronic India, Haryana, India). To investigate the interaction between neem oil and the surfactant, IR spectra of the prepared NONEs were obtained using FTIR (IRAffinity-1S, Shidmazu, Japan). For FTIR analysis, the mixture was pressed into pellets and the IR spectrum was examined in a wavelength range of 4000 to 500 cm−1 at a resolution of 4 cm−1. The droplet size (zeta size) and polydispersity index (PDI) of the prepared NONEs were measured using dynamic light scattering (DLS) (Malvern Zetasizer Nano ZS90, Malvern Instruments Ltd., Worcestershire, United Kingdom) after 15 days of storage at room temperature for a better understanding of their stability.
Preparation and characterization of coating formulations
Coating formulations were prepared from carnauba wax (CW) and NONEs. In a typical process, CW was first melted, and the melted wax (4%, w/v) was mixed with Tween 80 solution (5%, v/v) at 85 °C for 15 min, followed by ultrasonication using an ultrasonic processor (Vibra cell VCX-750, Sonics & Materials, Inc., Newton, USA) at 20 kHz for 10 min at 30 °C. An equal volume (100 mL) of the prepared solution was poured in three beakers, and then, 5% and 10% (w/v) of NONEs were added in two beakers, and mixed well by continuous stirring on a magnetic stirrer at 1000 rpm for 15 min, while in the third beaker the NONE was not added (having only CW). Thus, three different coating formulations, namely CW, CW/NONE-5, and CW/NONE-10 were prepared.
The viscosity of the prepared coating formulations was measured using a viscometer (DV3T, Brookfield Ametek, USA) equipped with a cylindrical spindle (LV4) at a speed of 80 rpm at room temperature. The pH was measured using a digital pH meter (Eutech Ion 2700, Thermo Fisher Scientific India Pvt. Ltd., Mumbai, India). Optical absorption of the coating formulations was also measured using a UV-vis spectrophotometer (2375 Double Beam, Electronic India, Haryana, India). The stability of the prepared coating formulations was tested under different conditions, in which the samples were centrifuged at 3500 rpm for 30 min to check the phase separation, followed by a heating–cooling cycle, which involved holding them alternately at 40 °C and 4 °C for 48 h.
Application of the prepared coating formulations on citrus fruits
The mature fruits (Khasi mandarin and sweet lemon) without any defects were collected, washed with 0.1% (v/v) sodium hypochlorite solution, and air-dried at room temperature. Both the types of citrus fruits were randomly distributed into 4 groups, with each group having 14-fruits. Out of 4-groups, 3 groups of each type of fruit were dipped manually in the three different (prepared) coating formulations for 1.0 min, then the excess coating was allowed to drain out, and the fruits were air-dried at room temperature. The 4th group of each type of fruit was left uncoated as the control. The coated and uncoated fruits were stored on trays under ambient conditions (25 °C) for 28 days. The effects of coatings on the weight loss, firmness, TSS, TA, maturity index, pH, decay index and sensory attributes of the fruit were measured at a 4-day interval during the storage period.
Shelf-life study of the coated citrus fruits
Weight loss and firmness.
Weight loss of the coated/treated fruits was determined using the method described by Basumatary et al., 2022.10,25 The % weight loss of the fruits was measured at each 4-day interval using eqn (1). | 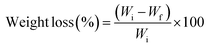 | (1) |
where ‘Wi’ and ‘Wf’ are the initial and the final weights of the fruit samples, respectively.
The firmness of the coated fruits was measured using a texture analyser (TA. XT. Plus Stable micro systems, UK) using a 6 mm cylindrical stainless-steel probe (P/6) at a compression speed of 10 mm s−1. The maximal force needed to break the fruits was recorded, and mean values of triplicate analysis were taken.
pH, total soluble solids (TSS), titratable acidity (TA) and maturity index (TSS/TA).
Citrus fruit samples were peeled and juice was extracted using a manual juicer, filtered, and immediately analysed for pH, TSS, and TA using a pH meter and digital refractometer (Atago, Tokyo, Japan) and by the titration method, respectively. Titration was carried out with 0.1 N NaOH using phenolphthalein as an indicator, and TA was expressed as grams of citric acid per 100 g of fruit juice according to the method discussed by Nourozia and Sayyari, 2020 with little modification.26 The maturity index of the fruits was expressed as the ratio of TSS/TA according to Perdones et al., 2022.27
Decay index (DI) and sensory attributes.
DI was measured by visually assessing the degree of microbial decay symptoms on each fruit surface using the following scale: 0 = healthy fruit, 1 = one very small lesion (beginning of infection), 2 = one lesion less than 10 mm in diameter, 3 = several lesions or 25% of fruit infected, and 4 = 26–50% of fruit infected.28 The results were expressed as percentage decay, calculated using the following eqn (2). |  | (2) |
Sensory analyses of the citrus fruits were carried out by untrained consumers of ages 18–45 years among students and employees of the Central Institute of Technology Kokrajhar, Assam India. A 9-point hedonic scale ranging from 9 for “like extremely” to 1 for “dislike extremely” was used to conduct the sensory test.
Statistical analysis
The experimental data obtained from different analyses were subjected to ANOVA with treatment and storage time as sources of variation. Mean comparisons among the treatments were performed using the Tukey's test at a significance level of p < 0.05. The analyses were performed using OriginPro 9.0 software and were performed in triplicate.
Results and discussion
Characteristics of NONEs
The results showed that pH, viscosity, and transmittance of the prepared NONEs increased with an increase in the concentration of the surfactant from a 1
:
1 to a 1
:
3 ratio, keeping the neem oil concentration constant (Table 1). The pH, viscosity, and transmittance of the NONEs increased from 5.50 to 5.69, 8.01 to 60.02 cP, and 0.08 to 0.95%, respectively, with the increase in the surfactant concentration. The increase in transmittance is an indication of decreased turbidity of the nanoemulsion that indicates that the surfactant to oil ratio has a significant impact on the nature of the colloidal dispersion.29
Table 1 pH, viscosity, turbidity, zeta potential, hydrodynamic size, and polydispersity index of the prepared NONEsa
NONE |
pH |
Viscosity (cP) |
Transmittance (%) |
Zeta potential (mV) |
Z-average (d nm) |
(PDI) |
The values are expressed as means ± SD of triplicate assays, and the superscript with the values indicates that they are significantly different (p < 0.05).
|
NONE-1 |
5.50 ± 0.02a |
8.01 ± 0.28a |
0.08 ± 0.005a |
−10.1 |
429.7 |
0.510 |
NONE-2 |
5.58 ± 0.01b |
18.34 ± 0.6b |
0.07 ± 0.005b |
−7.98 |
312.8 |
0.781 |
NONE-3 |
5.69 ± 0.005c |
60.02 ± 1.44c |
0.95 ± 0.025c |
−3.08 |
198.9 |
0.665 |
FTIR spectra of the NONEs are depicted in Fig. 1 that show a broad absorption peak at about 3400 cm−1 corresponding to O
H stretching indicating the presence of water, and a sharp peak at 2919 cm−1 implying C
H stretching of methylene groups in all the emulsions. However, the peak intensity of C
H stretching gradually decreased, as the concentration of the surfactant increased (from 1
:
1 to 1
:
3) in NONEs.30 The other major peaks at wavelengths ranging from 1100–1745 cm−1 correspond to C
O stretching indicating the presence of esters in the neem oil.
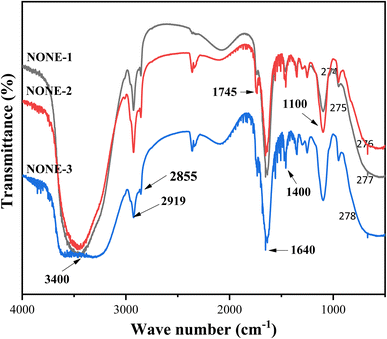 |
| Fig. 1 FTIR spectra of neem oil nanoemulsions; NONE-1, NONE-2, and NONE-3. | |
The zeta potential, droplet size, and polydispersity index of the prepared NONEs are shown in Table 1. Zeta potential of the NONEs varied from −3.08 to −10.1 mV depending on the concentration of the surfactant. With an increase in the concentration of the surfactant, the zeta potential decreased, i.e., NONE-1 has the highest, whereas NONE-3 has the lowest values, which indicate that Tween 80 is absorbed on the surface of oil droplets and stabilized it by creating electrostatic repulsive force amongst oil droplets.31 However, because of the low zeta potential value, there is a chance of flocculation/Ostwald ripening over time. In this study, determination of zeta potential of the prepared nanoemulsions was carried out after 15 days of ambient storage; therefore, up to this period, flocculation/Ostwald ripening was minimum, and thus the NONEs were stable. Other studies have also reported stability of oil in water (O/W) nanoemulsions at a low zeta potential value.32 The PDI value also confirmed the slow rate of flocculation/Ostwald ripening, as shown in Table 1. Also, the increased surfactant concentration resulted in smaller droplet sizes which could be due to their structure and their interaction with the surfactant.33 Theoretically, non-ionic surfactants such as Tween-80 stabilize droplets without adding any droplet charge; however the observed negative charge in our study is probably due to ionic impurities in the prepared NONEs.34
PDI represents the heterogeneity/change in the droplet size of the prepared nanoemulsions because of the Ostwald ripening over time. PDI values close to 1 indicate a heterogeneous distribution, whereas smaller PDI values indicate monomodal distributions and greater stability.35 In this study, the PDI values ranging from 0.50 to 0.78 showed that the prepared NONEs were polydisperse and moderately stable, which is consistent with the findings of Jaworska et al., 2015.36 PDI values greater than 0.5 have also been reported in nanoemulsions stabilized with Tween 80 by several other authors.
Properties of the prepared coating formulations
NONE-3 was selected for preparation of carnauba wax-based coating formulations, because of its stability and superior antibacterial activities. The various characteristics such as pH, viscosity and stability of the prepared CW-based coating formulations are summarised in Table 2. The pH and viscosity of the coating formulations increased from 4.36 to 5.92 and from 10.05 to 12.58 cP, respectively with an increase in the concentration of the NONE. Fig. 2 shows that the absorption of the visible light is increased with an increase in the concentration of the NONE in the coating formulation. All the coating formulations were found to be stable with no phase separation during the stress test and during storage. However, all the coating formulations showed some sedimentation after 60 days of ambient storage.
Table 2 Characteristics of the prepared coating formulationsa
Parameter |
CW |
CW/NONE-5 |
CW/NONE-10 |
The values are expressed as means ± SD of triplicate assays, and the superscript with the values indicates that they are significantly different (p < 0.05).
|
pH |
4.36 ± 0.05a |
4.84 ± 0.02b |
5.92 ± 0.01c |
Viscosity (cP) |
10.05 ± 0.57a |
11.83 ± 0.44a,b,c |
12.58 ± 0.3b,c |
Stability |
Stable |
Stable |
Stable |
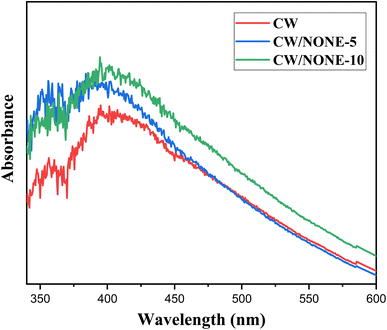 |
| Fig. 2 UV-vis spectra of the developed coating formulations. | |
Effects of coating treatment on the quality of the citrus fruits
Weight loss.
Fruit quality losses such as shrivelling of the fruit surface and diminished juiciness are mainly caused by the loss of water from the fruit.37 Weight loss in the coated and uncoated citrus fruits during ambient storage is given in Fig. 3A and B. The result showed a gradual increase in weight loss in the case of both Khasi mandarin (Fig. 3A) and sweet lemon (Fig. 3B) during the entire storage period; however the control samples show the highest weight loss from day-20 until the end of the storage period (day-28). Pristine CW and CW/NONE-10 coated fruits show maximum reduction in weight loss, in the case of both the fruits. Compared to the control (uncoated), the CW/NONE-10 coated citrus fruit shows reduction in weight loss from 37.35 to 33.71 (about 3.7%) in the case of Khasi mandarin and from 29.01 to 25.70 (about 3.3%) in the case of sweet lemon after 28 days of storage. Several authors reported that coating fresh tomatoes and mandarin fruits with carnauba wax emulsions incorporated with nanoemulsions or grape seed extract resulted in reduced weight loss from the coated/treated fruits.15,38
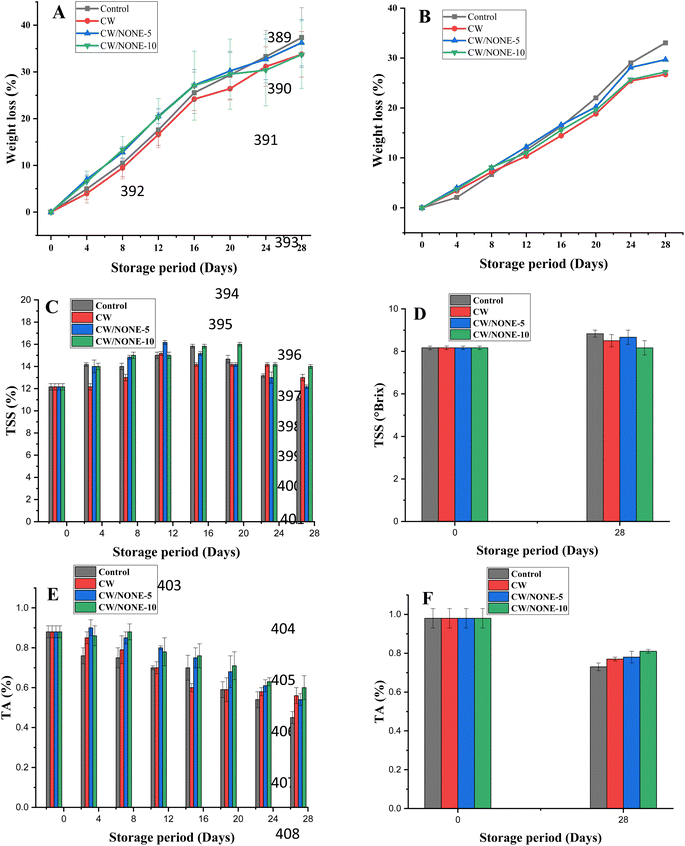 |
| Fig. 3 Weight loss in Khasi mandarin (A) and sweet lemon (B); TSS of Khasi mandarin (C) and sweet lemon (D); and TA of Khasi mandarin (E) and sweet lemon (F). | |
Total soluble solids (TSS).
TSS is one of the major internal quality parameters of citrus fruits, and it change naturally during ripening and storage.39 The TSS of the treated Khasi mandarin increased during the first 16 days of storage, and then significantly decreased afterwards (Fig. 3C), whereas the TSS of the treated sweet lemon increased continuously until 28 days of storage (Fig. 3D). Initially, the Khasi mandarin had TSS of 12.16° Brix, which increased to about 14° Brix in the CW/NONE-10 coated fruit sample and reduced to 11.16° Brix in the control fruit at the end of the storage, and thus the coating treatment significantly reduced TSS loss compared to the uncoated control fruit. In the case of treated sweet lemon, the initial TSS was 8.2° Brix, which increased to about 8.85° Brix in the case of the control, whereas the CW/NONE-10 coated fruits maintained TSS at about 8.2° Brix even after 28 days of storage indicating retention of sweetness of the coated fruits (Table S1†). The findings of this study were like the results reported in previous studies.25,40
Titratable acidity (TA).
Titratable acidity (TA) decreases during ripening of fruit due to the conversion of organic acids into sugar; however coating on fruit can effectively slow down the respiratory process resulting in a reduced rate of conversion of organic acids, as organic acids are used as substrates for respiratory metabolism.41 The TA values of both coated and uncoated Khasi mandarin (Fig. 3E) and sweet lemon (Fig. 3F) fruits decreased throughout the storage period, but the reduction was significantly less in the case of all the coated/treated fruits compared to the uncoated controls. At the end of the storage periods, CW/NONE-10 coated fruits had the highest TA values; 0.60% in Khasi mandarin and 0.81% in sweet lemon, whereas for the uncoated controls the values were the lowest; 0.45% and 0.71%, respectively (Table S1†). Thus, the prepared coatings efficiently maintained TA of both the citrus fruits during the 28 days of storage, which is in agreement with previous reported studies.42,43
Maturity index (TSS/TA).
The maturity index (TSS/TA) determines fruit quality, flavor, and consumer acceptance of fruit. The result showed that the TSS/TA ratio of both coated and uncoated fruits increased consistently during the entire storage period (Fig. 4A and B); however the TSS/TA values of the coated fruits were better maintained compared to the uncoated fruit until the end of the storage period. The CW/NONE-5 and CW/NONE-10 coated fruits show the lowest TSS/TA ratio i.e., 22.51 in Khasi mandarin and 10.08 in sweet lemon, whereas for control samples these values were 24.8, and 12.0, respectively, at the end of the 28 days of storage (Table S1†). Thus, the prepared composite coatings improved the postharvest shelf-life of treated fruits by delaying maturity and reducing the ripening rate. Previous studies have also reported on maintaining the TSS/TA value by CW-based coating on various fruits such as apples,44 Indian jujube fruits,45 Kinnow mandarin,46 and pomegranates.47
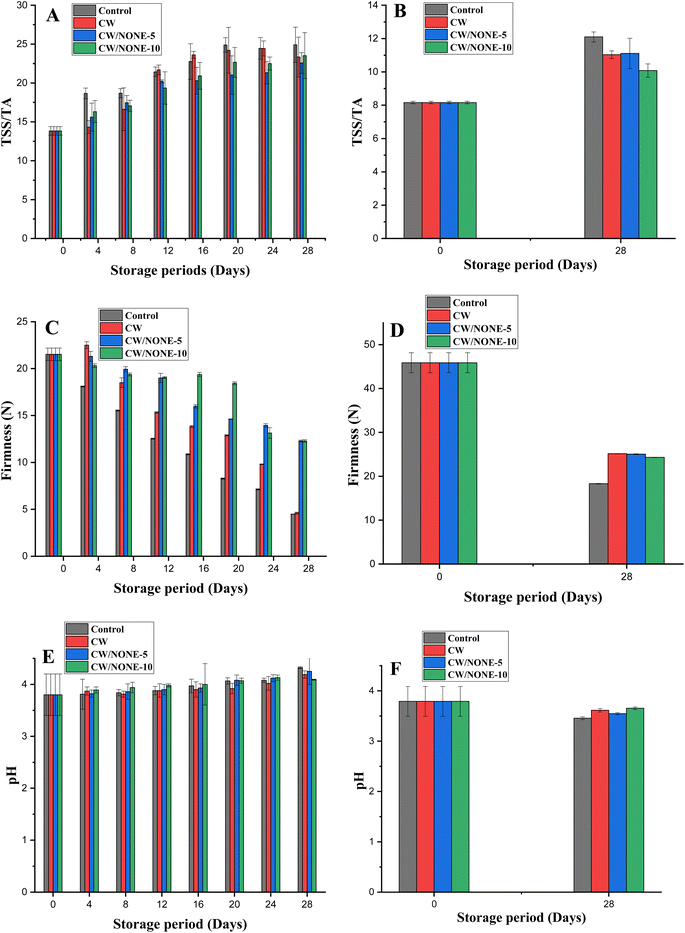 |
| Fig. 4 TSS/TA (maturity index) of treated Khasi mandarin (A) and sweet lemon (B); firmness of treated Khasi mandarin (C) and sweet lemon (D); and pH of Khasi mandarin (E) and sweet lemon (F). | |
Fruit firmness.
Fruit firmness is an important factor that determines the freshness of fruits and consumer acceptability. The fruit firmness of the coated and uncoated fruits decreased during storage but the coated fruits retained significantly higher firmness compared to the control/uncoated sample, which could be due to reduced enzymatic degradation as a result of coating that limits oxygen availability (Fig. 4C and D). The loss of tissue water and the turgor pressure are also responsible for reduced fruit firmness.48 Initially, the Khasi mandarin and the sweet lemon had firmness values of 21.52 N and 45.89 N, and by the end of storage the control sample rapidly lost firmness to 4.49 and 18.32 N, respectively (Table S1†). In the case of Khasi mandarin, the CW coated sample exhibits the highest firmness value (22.5 N) during the first 4 days of storage and rapidly declines by the end of the storage period (4.61 N), while the CW/NONE-5 and CW/NONE-10 coatings comparatively maintained the fruit firmness i.e., 12.28 N until the end of the storage. It can be concluded that the prepared CW/NONE-5 and CW/NONE-10 coatings significantly maintained the firmness of the citrus fruits up to 28 days of storage. In the case of sweet lemon, despite the reduction in firmness of the control sample from 45.89 N to 18.32 N, the coated samples maintained the fruit firmness between 24 and 25 N during the 28 days of storage. The retained firmness in the coated fruits might be due to reduced microbial decay, slow respiration, and reduced water loss. Other studies have also reported such retention of fruit firmness in carnauba wax coated plums,49 grape berries,50 and eggplants.51
pH.
The acid content of fruits decreases over time as a result of oxidation of organic acids during ripening.52 From Fig. 4E and F, it can be seen that the pH of the coated fruits slightly increased during storage in the case of both Khasi mandarin and sweet lemon. The control Khasi mandarin and sweet lemon recorded the highest increases in pH from 3.80 to 4.32 and from 3.79 to 4.05, respectively, on 28 days of storage (Table S1†). Similar results i.e., an increase in pH during storage were also reported for carnauba wax coated pomegranate fruit47 and papaya,53 which keep their pH levels stable, which may be the reason for the insignificant pH changes. The fruit ripening, inherent organic acid contents and several other factors including the biochemical state of the fruit, slower rate of respiration, etc., might have contributed to the pH change.54
Decay index.
The decay values of the treated Khasi mandarin and sweet lemon during storage are given in Table 3, and visual appearances of whole and half-cut Khasi mandarin and sweet lemon during storage under ambient conditions are presented in Fig. 5 and 6, respectively. In the case of the Khasi Mandarin, the control fruit showed signs of decay after 16 days, but the coated fruit showed signs of decay after 20 days of storage (Fig. 5A). By the end of the storage, the control Khasi mandarin showed the highest decay index (4.16%), whereas the CW/NONE-10 coated fruits had the least decay index (2.66%). In the case of sweet lemon, both control and pristine CW coated fruits showed signs of decay after 16 days of storage; however, CW/NONE-5, and CW/NONE-10 coated fruits showed the first signs of decay after day-20 (Fig. 6A). All the coated sweet lemons show the same decay index (1.2%), whereas the control shows the highest decay index (3.8%) by the end of 28 days of storage (Table 3). Several similar studies were also reported on carnauba wax-based coatings, which significantly reduced decay in coated fruits. For instance, carnauba wax coating decreased brown rot and Rhizopus rot by 4% and 9%, respectively.55 Also, decay in Indian jujube fruits was reduced by coating the fruit with a carnauba wax emulsion.45 Addition of NONEs in CW coating induced a decline in the decay rate of the coated fruits because of the strong antimicrobial nature of the prepared NONEs.
Table 3 Percent decay index (DI%) of the Khasi mandarin and sweet lemon stored for 28 days under ambient conditionsa
Fruit |
Treatments |
Day-16 |
Day-20 |
Day-24 |
Day-28 |
The values are expressed as means ± SD of triplicate assays, and the values with different superscripts indicate that they are significantly different (p < 0.05).
|
Khasi mandarin |
Uncoated |
2.16 ± 0.16a |
3 ± 0.28a |
3.83 ± 0.16a |
4.16 ± 0.16a |
CW |
0b |
2.33 ± 0.16a,b |
2.66 ± 0.16b |
3.66 ± 0.33a,b |
CW/NONE-5 |
0b |
1.66 ± 0.33b |
2.16 ± 0.33b |
3.16 ± 0.16b,c |
CW/NONE-10 |
0b |
1.66 ± 0.16b |
1.5 ± 0.28b |
2.66 ± 0.16c |
Sweet lemon |
Uncoated |
2.3 ± 0.33a |
2.9 ± 0.13a |
3.4 ± 0.2a |
3.8 ± 0.13a |
CW |
1.1 ± 0.1b |
1.2 ± 0.1b |
1.2 ± 0.1b |
1.2 ± 0.1b |
CW/NONE-5 |
0c |
1.1 ± 0.1b |
1.2 ± 0.1b |
1.2 ± 0.1b |
CW/NONE-10 |
0c |
1.1 ± 0.1b |
1.2 ± 0.1b |
1.2 ± 0.1b |
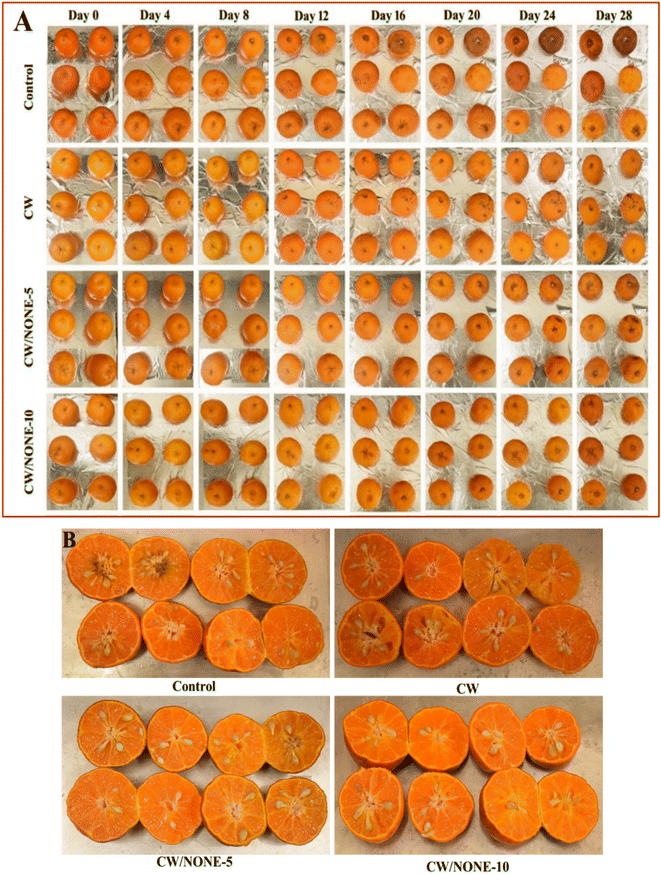 |
| Fig. 5 Visual appearance of (A) whole and (B) half-cut Khasi mandarin during 28 days of storage under ambient conditions. | |
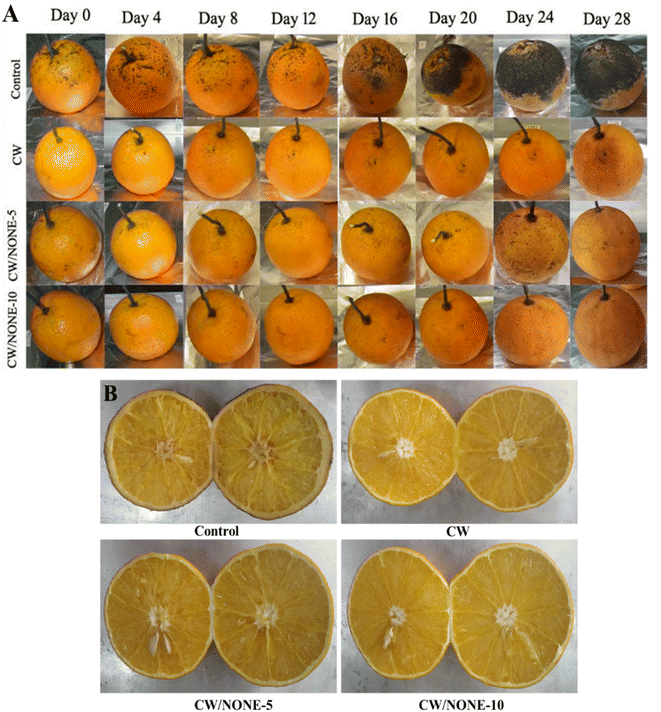 |
| Fig. 6 Visual appearance of whole (A) and half-cut (B) sweet lemon during 28 days of storage under ambient conditions. | |
Conclusion
In this study, edible and active coatings from carnauba wax and neem oil nanoemulsions were developed and applied on citrus fruits for extending their postharvest life. Oil-in-water (O/W) nanoemulsions of neem oil (NONEs) were prepared that showed strong antibacterial activities and thus incorporated as an active agent in developing CW-based coatings. The developed coatings were applied on fresh whole citrus fruits i.e., Khasi mandarin and sweet lemon, and the results showed that the weight loss, TSS, TA, TSS/TA, and firmness of the coated/treated fruits were maintained during 28 days of storage under ambient conditions. Coatings containing a 5% NONE (CW/NONE-5) and 10% NONE (CW/NONE-10) were highly effective in reducing the decay of the fruits and extended their shelf life by up to at least three weeks. Findings of this study indicate that the developed plant wax-based active coatings could be used as an edible and sustainable alternative to conventional/synthetic wax-based coatings for extension of the postharvest life of citrus fruits.
Conflicts of interest
The authors declare no competing interest.
Acknowledgements
SK and AM are very much thankful to the Department of Biotechnology (DBT), Government of India for the research funding (sanction letter vide No. BT/PR39789/NER/95/1664/2020).
References
-
A. Iqbal, R. A. Khera, M. A. Hanif, M. A. Ayub and A. M. Al-Sadi, in Medicinal Plants of South Asia, Elsevier, 2020, pp. 617–630 Search PubMed.
- S. M. B. Hashemi, A. M. Khaneghah, F. J. Barba, Z. Nemati, S. S. Shokofti and F. Alizadeh, J. Funct. Foods, 2017, 38, 409–414 CrossRef CAS.
- S. Brahma, R. K. Nath, K. K. Roy, R. Sarma and P. Ahmed, Int. J. Curr. Microbiol. Appl. Sci., 2020, 9, 81–85 CrossRef.
- L. C. De, Int. J. Agric. Sci. Res., 2017, 7, 325–342 Search PubMed.
- Y. Cheng, Y. Lin, H. Cao and Z. Li, Microorganisms, 2020, 8, 449 CrossRef CAS PubMed.
- O. Alegbeleye, O. A. Odeyemi, M. Strateva and D. Stratev, Appl. Food Res., 2022, 2, 100122 CrossRef CAS.
- I. H. Ahmed and A.-B. A. Abu-Goukh, J. Agric. Sci., 2021, 51, 59–72 Search PubMed.
- H. Wang, N. Xu, Y. Peng and X. Zhang, J. Sci. Food Agric., 2022, 102, 4949–4954 CrossRef CAS.
- I. B. Basumatary, A. Mukherjee, V. Katiyar, S. Kumar and J. Dutta, Coatings, 2021, 11, 1366 CrossRef CAS.
- I. B. Basumatary, A. Mukherjee, V. Katiyar, J. Dutta and S. Kumar, LWT, 2022, 168, 113940 CrossRef CAS.
- C. A. S. de Freitas, P. H. M. de Sousa, D. J. Soares, J. Y. G. da Silva, S. R. Benjamin and M. I. F. Guedes, Food Chem., 2019, 291, 38–48 CrossRef CAS PubMed.
- M. M. Gutiérrez-Pacheco, L. A. Ortega-Ramírez, B. A. Silva-Espinoza, M. R. Cruz-Valenzuela, G. A. González-Aguilar, J. Lizardi-Mendoza, R. Miranda and J. F. Ayala-Zavala, Coatings, 2020, 10, 614 CrossRef.
- S. Singh, P. Khemariya, A. Rai, A. C. Rai, T. K. Koley and B. Singh, LWT, 2016, 74, 420–426 CrossRef CAS.
- M. D. Ferreira, D. S. Corrêa, M. Miranda, T. L. Ohashi, L. Pilon and P. C. Spricigo, Revista Iberoamericana de Tecnología Postcosecha, 2015, 16, 199–209 Search PubMed.
- M. Y. Won and S. C. Min, Food Sci. Biotechnol., 2018, 27, 1649–1658 CrossRef CAS PubMed.
- E. Motamedi, J. Nasiri, T. R. Malidarreh, S. Kalantari, M. R. Naghavi and M. Safari, Sci. Hortic., 2018, 240, 170–178 CrossRef CAS.
- L. Susmita Devi, S. Kalita, A. Mukherjee and S. Kumar, Trends Food Sci. Technol., 2022, 129, 296–305 CrossRef CAS.
- C. Chen, X. Peng, R. Zeng, M. Chen, C. Wan and J. Chen, Sci. Hortic., 2016, 202, 41–48 CrossRef CAS.
- R. Passafiume, I. Tinebra, R. Gaglio, L. Settanni, G. Sortino, A. Allegra and V. Farina, Coatings, 2022, 12, 664 CrossRef CAS.
- M. D. J. Jhalegar, R. R. Sharma and D. Singh, J. Food Sci. Technol., 2015, 52, 2229–2237 CrossRef PubMed.
- E. Osman Mohamed Ali, N. A. Shakil, V. S. Rana, D. J. Sarkar, S. Majumder, P. Kaushik, B. B. Singh and J. Kumar, Ind. Crops Prod., 2017, 108, 379–387 CrossRef CAS.
- P. Wen, M.-H. Zong, R. J. Linhardt, K. Feng and H. Wu, Trends Food Sci. Technol., 2017, 70, 56–68 CrossRef CAS.
- S. Kumar, N. Singh, L. S. Devi, S. Kumar, M. Kamle, P. Kumar and A. Mukherjee, J. Agric. Food Res., 2022, 7, 100254 Search PubMed.
- P. de Castro e Silva, L. A. S. Pereira, A. M. T. Lago, M. Valquíria, É. M. de Rezende, G. R. Carvalho, J. E. Oliveira and J. M. Marconcini, Food Biophys., 2019, 14, 456–466 CrossRef.
- F. Khorram, A. Ramezanian and S. M. H. Hosseini, Sci. Hortic., 2017, 225, 22–28 CrossRef CAS.
- F. Nourozi and M. Sayyari, Sci. Hortic., 2020, 262, 109041 CrossRef CAS.
- A. Perdones, L. Sánchez-González, A. Chiralt and M. Vargas, Postharvest Biol. Technol., 2012, 70, 32–41 CrossRef CAS.
- I. B. Basumatary, A. Mukherjee, V. Katiyar, S. Kumar and J. Dutta, Coatings, 2021, 11, 1366 CrossRef CAS.
- S. N. Liew, U. Utra, A. K. Alias, T. B. Tan, C. P. Tan and N. S. Yussof, LWT, 2020, 128, 109388 CrossRef CAS.
- U. Luesakul, S. Puthong, K. Sansanaphongpricha and N. Muangsin, Carbohydr. Polym., 2020, 230, 115625 CrossRef CAS PubMed.
- S. Kheradmandnia, E. Vasheghani-Farahani, M. Nosrati and F. Atyabi, Nanomed.: Nanotechnol. Biol. Med., 2010, 6, 753–759 CrossRef CAS PubMed.
- M. Koroleva, T. Nagovitsina and E. Yurtov, Phys. Chem. Chem. Phys., 2018, 20, 10369–10377 RSC.
- M. I. Guerra-Rosas, J. Morales-Castro, L. A. Ochoa-Martínez, L. Salvia-Trujillo and O. Martín-Belloso, Food Hydrocolloids, 2016, 52, 438–446 CrossRef CAS.
- F. Rinaldi, P. N. Hanieh, C. Longhi, S. Carradori, D. Secci, G. Zengin, M. G. Ammendolia, E. Mattia, E. Del Favero and C. Marianecci, J. Enzyme Inhib. Med. Chem., 2017, 32, 1265–1273 CrossRef CAS PubMed.
- M. I. Guerra-Rosas, J. Morales-Castro, M. A. Cubero-Márquez, L. Salvia-Trujillo and O. Martín-Belloso, Food Control, 2017, 77, 131–138 CrossRef CAS.
- M. Jaworska, E. Sikora and J. Ogonowski, Chem. Eng. Technol., 2015, 38, 1469–1476 CrossRef CAS.
- R. Lufu, A. Ambaw and U. L. Opara, Sci. Hortic., 2020, 272, 109519 CrossRef.
- M. Miranda, M. R. Marilene De Mori, P. C. Spricigo, L. Pilon, M. C. Mitsuyuki, D. S. Correa and M. D. Ferreira, Heliyon, 2022, e09803 CrossRef CAS PubMed.
- A. S. Nandane, R. K. Dave and T. V. R. Rao, J. Food Sci. Technol., 2017, 54, 1–8 CrossRef CAS PubMed.
- B. Saberi, J. B. Golding, S. Chockchaisawasdee, C. J. Scarlett and C. E. Stathopoulos, Starch – Stärke, 2018, 70, 1700299 CrossRef.
- R. Thakur, P. Pristijono, J. B. Golding, C. E. Stathopoulos, C. J. Scarlett, M. Bowyer, S. P. Singh and Q. V. Vuong, Sci. Hortic., 2018, 237, 59–66 CrossRef CAS.
- A. Khan, M. Azam, J. Shen, M. A. Ghani, A. S. Khan, S. Ahmad, M. A. Iqbal, N. Anjum, J. Zhang, M. A. Anjum, M. J. Jaskani, M. Ayyub and A. Javed, J. Food Meas. Charact., 2021, 15, 1727–1736 CrossRef.
- M. Radi, E. Firouzi, H. Akhavan and S. Amiri, J. Food Qual., 2017, 2017, 9764650 CrossRef.
- W.-S. Jo, H.-Y. Song, N.-B. Song, J.-H. Lee, S. C. Min and K. B. Song, LWT–Food Sci. Technol., 2014, 55, 490–497 CrossRef CAS.
- H. Chen, Z. Sun and H. Yang, Sci. Hortic., 2019, 244, 157–164 CrossRef CAS.
- F. Khorram, A. Ramezanian and S. M. H. Hosseini, J. Food Meas. Charact., 2017, 11, 1827–1833 CrossRef.
- H. Meighani, M. Ghasemnezhad and D. Bakhshi, J. Food Sci. Technol., 2015, 52, 4507–4514 CrossRef CAS PubMed.
- A. C. Paniagua, A. R. East, J. P. Hindmarsh and J. A. Heyes, Postharvest Biol. Technol., 2013, 79, 13–19 CrossRef.
- I.-H. Kim, H. Lee, J. E. Kim, K. B. Song, Y. S. Lee, D. S. Chung and S. C. Min, J. Food Sci., 2013, 78, E1551–E1559 CrossRef CAS PubMed.
- I.-H. Kim, Y. A. Oh, H. Lee, K. B. Song and S. C. Min, LWT–Food Sci. Technol., 2014, 58, 1–10 CrossRef CAS.
- S. Singh, B. Singh and T. Alam, J. Food Sci. Technol., 2019, 56, 4826–4833 CrossRef CAS PubMed.
- M. Cofelice, F. Lopez and F. Cuomo, Foods, 2019, 8, 189 CrossRef CAS PubMed.
- N. M. Zucchini, C. Florencio, M. Miranda, K. R. Borba, F. C. A. Oldoni, J. G. Oliveira Filho, N. S. Bonfim, K. A. Rodrigues, R. M. D. de Oliveira, M. C. Mitsuyuki, S. Z. Hubinger, J. D. Bresolin and M. D. Ferreira, Acta Hortic., 2021, 1325, 199–206 CrossRef.
- P. Jitareerat, S. Paumchai, S. Kanlayanarat and S. Sangchote, N. Z. J. Crop Hortic. Sci., 2007, 35, 211–218 CrossRef CAS.
- F. P. Gonçalves, M. C. Martins, G. J. S. Junior, S. A. Lourenço and L. Amorim, Postharvest Biol. Technol., 2010, 58, 211–217 CrossRef.
|
This journal is © The Royal Society of Chemistry 2023 |
Click here to see how this site uses Cookies. View our privacy policy here.