Correlations between color, textural properties and ripening of the North American pawpaw (Asimina triloba) fruit
Received
30th August 2022
, Accepted 14th January 2023
First published on 23rd January 2023
Abstract
The North American pawpaw (Asimina triloba [L.] Dunal) fruit is the largest edible fruit native to the United States. Over the years, the fruit has remained underutilized with limited published data on the properties of the fruit. In this study, the color of the skin and the pulp of the fruit, as well as the textural properties of unripe and ripe fruit harvested from wild trees were evaluated. The results show statistically significant differences in the textural properties of the unripe and ripe fruits. The ripe fruits had a hardness of 2.2 ± 0.5 kg-force, similar to the hardness of green ripe mangoes but harder than green ripe bananas, and the unripe fruits had a hardness of 68.2 ± 10.9 kg-force. Also, there were strong negative correlations between the fruit skin color a* values and the hardness (r = −0.87), chewiness (r = −0.86), and cohesiveness ratio (r = −0.73), and a strongly positive correlation with total soluble solids (r = 0.90). The skin hue angles had strong positive correlations with hardness (r = 0.86), cohesiveness ratio (r = 0.74) and chewiness (r = 0.86), and a strongly negative correlation with total soluble solids (r = −0.91). The fruit skin color a* values (degree of greenness), skin hue angle and total soluble solids content can be used as non-invasive indicators of pawpaw ripeness. The correlations established in this study provide new insights; farmers could use commercially available portable color and near-infrared Brix meters to determine the maturity of pawpaw fruits. These findings will help farmers and processors to harvest and process pawpaw fruits at the right time to minimize postharvest losses.
1. Introduction
The North American pawpaw (Asimina triloba [L.] Dunal) is a unique member of the Annonaceae family that grows in the temperate region of the world. The fruit of the pawpaw tree is known to be the largest edible fruit native to the United States. The pawpaw fruit has remained underutilized primarily due to its short shelf life, and the darkening of the skin after harvesting.1,2 To date, the fruit has not been commercialized or standardized as a horticultural crop. Several cultivars of the North American pawpaw have recently been selected for their excellent fruit characteristics,3 however, no objective ripeness indicators have been developed, such as those established for fruits like banana, mango, and apple.4–6
Unlike climacteric fruits like banana which can be harvested unripe and allowed to ripen over time, pawpaw fruits are best harvested when ripe. Unripe pawpaw fruits have been found to remain unripe even after comingling with ripe pawpaw fruits, suggesting a low sensitivity to ethylene.7 To harvest pawpaw fruits, the pawpaw tree is given a gentle shake to allow ripe fruits to fall by themselves to the ground. During ripening, the fruit peduncle (portion linking the fruit to the stem of the tree) softens leading to the fall of fruit from the tree when mature. Further, the ripening of the fruit has been reported to be characterized by a loss of the green color intensity, reduction in the hardness, and increases in the soluble solids content and volatile aroma compounds.7,8 However, the correlations between noninvasive ripeness indicators like color and invasive indicators like texture and soluble solids content have not been studied to confirm the general notion that color is not a good indicator of pawpaw fruit ripeness.
This research aims to investigate the textural properties and the color of both ripe and unripe pawpaw fruits, and to test the correlations between the color, textural properties, and total soluble solids content to gain insights on the potential use of noninvasive indicators for pawpaw fruit ripeness. These insights into the color and textural properties of ripening pawpaw fruits will be helpful to identify attributes to monitor fruit ripeness to prevent fruit loss as interest in the crop increases.
2. Materials and methods
2.1 Fruit samples
Ripe and unripe pawpaw fruits were harvested from eight-year-old seedling trees of wild origin growing in a butterfly garden near Eckles Hall on the University of Missouri campus, Columbia, Missouri. In August 2021, ripe pawpaw fruits were harvested by gently shaking the branches of the trees, while nearly mature but still unripe fruits were plucked from the same trees on the same day. The fruits were immediately brought to the laboratory for analysis.
2.2 Fruit color
Fruit color was measured according to the method described by Nambi and others9 using the Hunter LAB color meter (Chroma Meter CR-410, Konica Minolta). The analyses were carried out with 13–17 fruits each for unripe and ripe fruits. Five readings for each pawpaw skin and five readings for each pawpaw pulp were read at five different places on each fruit. The averages for the recordings were used to calculate the total color difference (ΔE or Delta E), chroma, and hue angle using the equations below where, L* is the degree of lightness to darkness, a* is the degree of redness to greenness, b* is the degree of yellowness to blueness, the subscripts f and i denote final (ripe fruits) and initial (unripe fruits) value. |  | (1) |
|  | (2) |
| 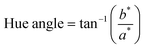 | (3) |
Hue angle values were corrected for the respective quadrants, where 0°/360° = red, 90° = yellow, 180° = green and 270° = blue as described by McLellan and others.10
2.3 Total soluble solids
Total soluble solids (TSS) content was measured according to the AOAC Official Method 932.14C11 using a digital refractometer (HI96800, Hanna Instruments) at ∼25 °C. Thirteen ripe fruits were used for this analysis. A sample of the ripe pulp was placed in the sample well of the refractometer. The total soluble solids measurements were taken in triplicates for each fruit and recorded as degree Brix. The total soluble solids content of the unripe fruits was not measured because the pulp was hard.
2.4 Texture analyses
Textural properties of the fruits were determined by a texture profile analysis (TPA) following the method described by Yang and others12 with some modifications. Pawpaw fruits were analyzed for their hardness, chewiness, cohesiveness, springiness ratio (or springiness) and resilience ratio using a Texture Analyzer (TA.HDPlus C, Stable Micro Systems) equipped with a 100 kg load cell and connected to the Exponent Connect Software (Stable Micro Systems). A P/75 (3-inch diameter) compression plate was used for the analyses. The texture analyzer was programmed to carry out a texture profile analysis with the following test conditions: pretest speed of 1 mm s−1, test speed of 1 mm s−1, post-test speed of 1 mm s−1, trigger force of 5 g, compression distance of 10 mm and a time of 5 s between compressions. The analyses were carried out with 13–17 fruits each for unripe and ripe fruits of similar size and shape. An illustration of the texture profile with the variables used to obtain the textural parameters are shown in Fig. 1. Hardness and chewiness were recorded in kilograms of force (kg); cohesiveness and resilience ratios were recorded as percentages (%), and springiness ratio was recorded as a dimensionless ratio. | Chewiness = hardness × cohesiveness × springiness | (4) |
| 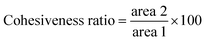 | (5) |
| 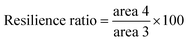 | (6) |
| 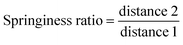 | (7) |
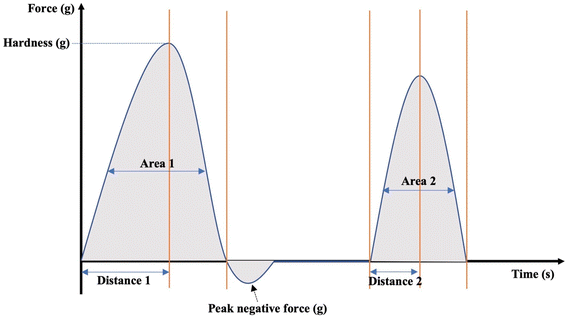 |
| Fig. 1 An illustration of a texture profile from which the hardness, chewiness, cohesiveness ratio, resilience ratio, and springiness ratio are obtained. | |
2.5 Statistical analyses
Student's t-test was used to test for differences in the means of the textural properties and color indices of the unripe and ripe pawpaw fruits. The data were analyzed at a significance level of 0.05 using JMP 14.0.0 software (SAS Institute Inc., Cary, USA). Principal component analysis (PCA), hierarchical cluster analysis (HCA) and Pearson's correlation analysis were performed using OriginPro 2021 version 9.8.0 software (Origin Lab Inc., Northampton, Massachusetts, USA).
3. Results
3.1 Color of fruit and pulp
The data obtained show that apart from the b* values, there were significant differences in all the color parameters between unripe and ripe fruits (Table 1). The unripe fruits had higher L* values (59.9 ± 3.5) than the ripe fruits (56.2 ± 5.4) (p = 0.0002) indicating the unripe fruits were lighter in color than the ripe fruits. The unripe fruits recorded a high skin a* value (degree of greenness) (−15.2 ± 4.6) whereas the ripe fruits showed some degree of redness (b* value) on the skin (0.9 ± 4.9). The unripe fruits had a slightly higher color saturation (chroma) than the ripe fruits as shown in Table 1 and Fig. 2. Also, the data obtained show that there is a clear visible total color difference (Delta E) in the skin colors of the unripe and ripe fruits (18.4 ± 4.2) (Table 1). The hue angles for the colors of the fruits indicated that the average color of the unripe fruits (118.8° ± 3.9°) lies between yellow (90°) and green (180°) while the average color of the ripe fruits (87.4° ± 9.1°) lies between red (0°) and yellow (90°) (Table 1). The color data for the unripe and ripe pulps show a more significant difference in the lightness (L*) and color saturation (chroma) values of the fruit pulp (Table 1).
Table 1 External fruit (skin) color and pulp color of pawpaw fruits grown in central Missouri, 2021a
|
L* |
a* |
b* |
Chroma |
Delta E |
Hue angle (°) |
All t-tests were two-tailed with 0.05 level of significance.
Delta E values calculated based on difference from unripe samples.
|
Unripe fruit skin |
59.9 ± 3.5 |
−15.2 ± 4.6 |
27.5 ± 3.2 |
31.7 ± 3.4 |
0.0 ± 0.0b |
118.8 ± 3.9 |
Ripe fruit skin |
56.2 ± 5.4 |
0.9 ± 4.9 |
27.8 ± 5.7 |
28.2 ± 5.8 |
18.4 ± 4.2 |
87.4 ± 9.1 |
t-Test |
3.85 |
−15.83 |
−0.27 |
3.52 |
−28.90 |
−10.45 |
p-Value |
0.0002 |
<0.0001 |
0.7863 |
0.0007 |
<0.0001 |
<0.0001 |
Unripe pulp |
76.2 ± 3.9 |
−7.2 ± 1.8 |
24.9 ± 2.9 |
26.0 ± 3.1 |
0.0 ± 0.0b |
106.1 ± 2.9 |
Ripe pulp |
63.0 ± 3.7 |
11.6 ± 2.1 |
50.1 ± 6.3 |
51.5 ± 6.5 |
34.5 ± 5.5 |
77.0 ± 1.8 |
t-Test |
16.42 |
−44.74 |
−23.98 |
−23.50 |
−41.43 |
−28.26 |
p-Value |
<0.0001 |
<0.0001 |
<0.0001 |
<0.0001 |
<0.0001 |
<0.0001 |
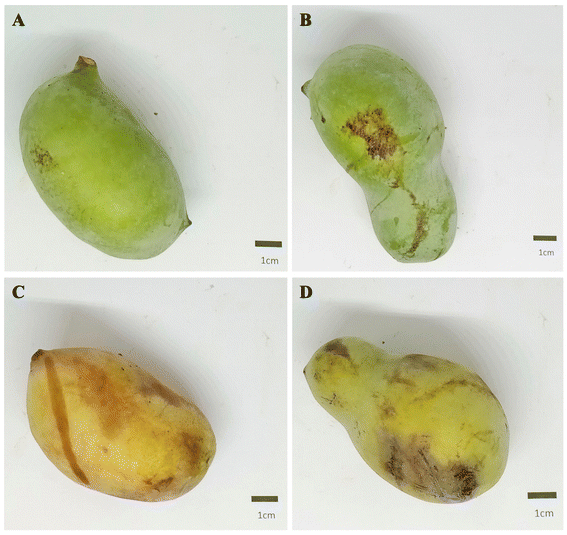 |
| Fig. 2 Images of unripe pawpaw fruits (A) and (B) and ripe pawpaw fruits (C) and (D). | |
3.2 Total soluble solids content
The total soluble solids content (Brix) of the pulp of the ripe fruits was 21.52 ± 0.94. The total soluble solids content of the unripe fruits was not measured due to its hard texture, which made it difficult to determine the total soluble solids content.
3.3 Texture profile of fruits
The hardness of the unripe pawpaw fruits (68.2 ± 10.9 kg-force) was significantly higher than that of the ripe fruits (2.2 ± 0.5 kg-force) (Table 2) as shown in Fig. 3. The data obtained in this study shows that the unripe fruits had a significantly high cohesiveness (56.9 ± 8.1%) compared to the ripe fruits (19.7 ± 2.4%) (Table 2). The unripe fruits had a chewiness of 167.6 ± 20.4 kg-force while the ripe fruits recorded a chewiness of 1.7 ± 0.6 kg-force (Table 2). Unripe fruits (27.4 ± 2.9%) had a significantly higher resilience ratio than the ripe fruits (7.5 ± 1.3%), however, the springiness of the unripe fruits (3.6 ± 0.7) was not statistically different from that of the ripe fruits (3.5 ± 0.2).
Table 2 Textural properties of unripe and ripe pawpaw fruits grown in central Missouri, 2021a
|
Hardness (kg-force) |
Chewiness (kg-force) |
Cohesiveness ratio (%) |
Resilience ratio (%) |
Springiness ratio |
All t-tests were two-tailed with 0.05 level of significance.
|
Unripe fruit |
68.2 ± 10.9 |
167.6 ± 20.4 |
56.9 ± 8.1 |
27.4 ± 2.9 |
3.6 ± 0.7 |
Ripe fruit |
2.2 ± 0.5 |
1.7 ± 0.6 |
19.7 ± 2.4 |
7.5 ± 1.3 |
3.5 ± 0.2 |
t-Test |
10.12 |
12.66 |
7.69 |
3.14 |
1.49 |
p-Value |
<0.0001 |
<0.0001 |
<0.0001 |
0.004 |
0.148 |
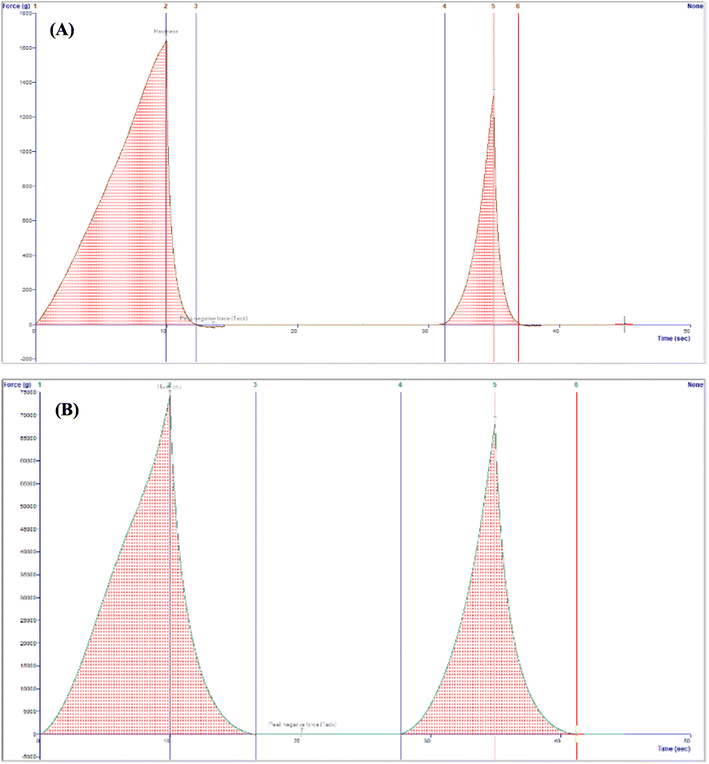 |
| Fig. 3 Texture profiles of (A) ripe pawpaw fruit and (B) unripe pawpaw fruit. | |
3.4 Pearson's correlation analysis
From the correlation plot and correlation matrix (Fig. 4 and Table 3), statistically significant correlations between the skin and pulp color parameters and the textural properties were found. Most of the stronger correlations were between the pulp color and the textural properties. The correlation matrix shows that there is a strong negative correlation between pawpaw fruit hardness and the skin a* values (r = −0.87, p ≤ 0.01), and fruit hardness and skin total color difference (r = −0.87, p ≤ 0.01), and a strong positive correlation between fruit hardness and skin hue angle (r = 0.86, p ≤ 0.01). Also, the cohesiveness ratio had strong negative correlations with the skin a* value (r = −0.73, p ≤ 0.01) and the skin total color difference (r = −0.84, p ≤ 0.01) but a strong correlation with skin hue angle (r = 0.74, p ≤ 0.01). Similarly, chewiness had a strong negative correlation with the skin a* value (r = −0.86, p ≤ 0.01) and the skin total color difference (r = −0.88, p ≤ 0.01), and a strong positive correlation with the skin hue angle (r = 0.86, p ≤ 0.01). Total soluble solids had a strong positive correlation with the skin a* value (r = 0.90, p ≤ 0.01), skin hue angle (r = 0.86, p ≤ 0.01), and the skin total color difference (r = 0.95, p ≤ 0.01).
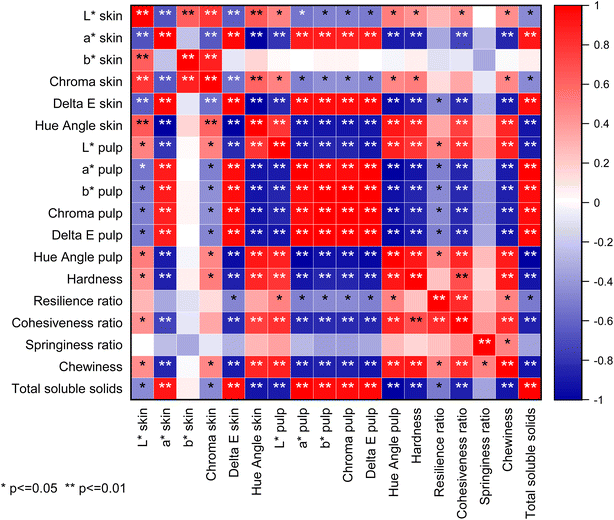 |
| Fig. 4 Correlation plot showing statistical significance for Pearson's correlations among the color, total soluble solids, and textural properties of pawpaw fruit. | |
Table 3 Correlation matrix of Pearson's correlation coefficients (r) for the color, total soluble solids, and textural properties of pawpaw fruits grown in Central Missouri, 2021
|
L* skin |
a* skin |
b* skin |
Chroma skin |
Delta E skin |
Hue angle skin |
L* pulp |
a* pulp |
b* pulp |
Chroma pulp |
Delta E pulp |
Hue angle pulp |
Hardness |
Resilience ratio |
Cohesiveness ratio |
Springiness ratio |
Chewiness |
Total soluble solids |
L* skin |
1.00 |
−0.67 |
0.63 |
0.78 |
−0.62 |
0.65 |
0.49 |
−0.52 |
−0.49 |
−0.49 |
−0.52 |
0.50 |
0.44 |
0.29 |
0.44 |
0.01 |
0.44 |
−0.45 |
a* skin |
−0.67 |
1.00 |
−0.24 |
−0.66 |
0.97 |
−0.99 |
−0.80 |
0.89 |
0.88 |
0.88 |
0.90 |
−0.89 |
−0.87 |
−0.33 |
−0.73 |
−0.24 |
−0.86 |
0.90 |
b* skin |
0.63 |
−0.24 |
1.00 |
0.86 |
−0.10 |
0.18 |
0.03 |
0.01 |
0.04 |
0.04 |
0.02 |
−0.02 |
0.07 |
−0.11 |
−0.09 |
−0.33 |
0.00 |
0.07 |
Chroma skin |
0.78 |
−0.66 |
0.86 |
1.00 |
−0.56 |
0.61 |
0.49 |
−0.48 |
−0.43 |
−0.43 |
−0.47 |
0.47 |
0.52 |
0.15 |
0.36 |
−0.08 |
0.47 |
−0.44 |
Delta E skin |
−0.62 |
0.97 |
−0.10 |
−0.56 |
1.00 |
−0.98 |
−0.84 |
0.95 |
0.95 |
0.95 |
0.96 |
−0.95 |
−0.87 |
−0.47 |
−0.84 |
−0.30 |
−0.88 |
0.95 |
Hue angle skin |
0.65 |
−0.99 |
0.18 |
0.61 |
−0.98 |
1.00 |
0.80 |
−0.90 |
−0.90 |
−0.90 |
−0.91 |
0.90 |
0.86 |
0.34 |
0.74 |
0.28 |
0.86 |
−0.91 |
L* pulp |
0.49 |
−0.80 |
0.03 |
0.49 |
−0.84 |
0.80 |
1.00 |
−0.89 |
−0.84 |
−0.85 |
−0.90 |
0.87 |
0.79 |
0.49 |
0.81 |
0.36 |
0.87 |
−0.89 |
a* pulp |
−0.52 |
0.89 |
0.01 |
−0.48 |
0.95 |
−0.90 |
−0.89 |
1.00 |
0.95 |
0.95 |
0.99 |
−0.99 |
−0.91 |
−0.48 |
−0.85 |
−0.27 |
−0.89 |
0.98 |
b* pulp |
−0.49 |
0.88 |
0.04 |
−0.43 |
0.95 |
−0.90 |
−0.84 |
0.95 |
1.00 |
1.00 |
0.98 |
−0.94 |
−0.85 |
−0.46 |
−0.83 |
−0.37 |
−0.88 |
0.95 |
Chroma pulp |
−0.49 |
0.88 |
0.04 |
−0.43 |
0.95 |
−0.90 |
−0.85 |
0.95 |
1.00 |
1.00 |
0.98 |
−0.94 |
−0.85 |
−0.46 |
−0.83 |
−0.38 |
−0.88 |
0.95 |
Delta E pulp |
−0.52 |
0.90 |
0.02 |
−0.47 |
0.96 |
−0.91 |
−0.90 |
0.99 |
0.98 |
0.98 |
1.00 |
−0.98 |
−0.89 |
−0.48 |
−0.86 |
−0.34 |
−0.90 |
0.98 |
Hue angle pulp |
0.50 |
−0.89 |
−0.02 |
0.47 |
−0.95 |
0.90 |
0.87 |
−0.99 |
−0.94 |
−0.94 |
−0.98 |
1.00 |
0.91 |
0.49 |
0.85 |
0.28 |
0.89 |
−0.99 |
Hardness |
0.44 |
−0.87 |
0.07 |
0.52 |
−0.87 |
0.86 |
0.79 |
−0.91 |
−0.85 |
−0.85 |
−0.89 |
0.91 |
1.00 |
0.26 |
0.69 |
0.17 |
0.90 |
−0.90 |
Resilience ratio |
0.29 |
−0.33 |
−0.11 |
0.15 |
−0.47 |
0.34 |
0.49 |
−0.48 |
−0.46 |
−0.46 |
−0.48 |
0.49 |
0.26 |
1.00 |
0.84 |
0.25 |
0.48 |
−0.50 |
Cohesiveness ratio |
0.44 |
−0.73 |
−0.09 |
0.36 |
−0.84 |
0.74 |
0.81 |
−0.85 |
−0.83 |
−0.83 |
−0.86 |
0.85 |
0.69 |
0.84 |
1.00 |
0.41 |
0.83 |
−0.87 |
Springiness ratio |
0.01 |
−0.24 |
−0.33 |
−0.08 |
−0.30 |
0.28 |
0.36 |
−0.27 |
−0.37 |
−0.38 |
−0.34 |
0.28 |
0.17 |
0.25 |
0.41 |
1.00 |
0.50 |
−0.35 |
Chewiness |
0.44 |
−0.86 |
0.00 |
0.47 |
−0.88 |
0.86 |
0.87 |
−0.89 |
−0.88 |
−0.88 |
−0.90 |
0.89 |
0.90 |
0.48 |
0.83 |
0.50 |
1.00 |
−0.92 |
Total soluble solids |
−0.45 |
0.90 |
0.07 |
−0.44 |
0.95 |
−0.91 |
−0.89 |
0.98 |
0.95 |
0.95 |
0.98 |
−0.99 |
−0.90 |
−0.50 |
−0.87 |
−0.35 |
−0.92 |
1.00 |
3.5 Multivariate analysis
From the PCA results (Fig. 5A), the variance contribution rates of the first, second, and third PCs (principal components) are 70.86%, 13.57%, and 5.76% respectively.
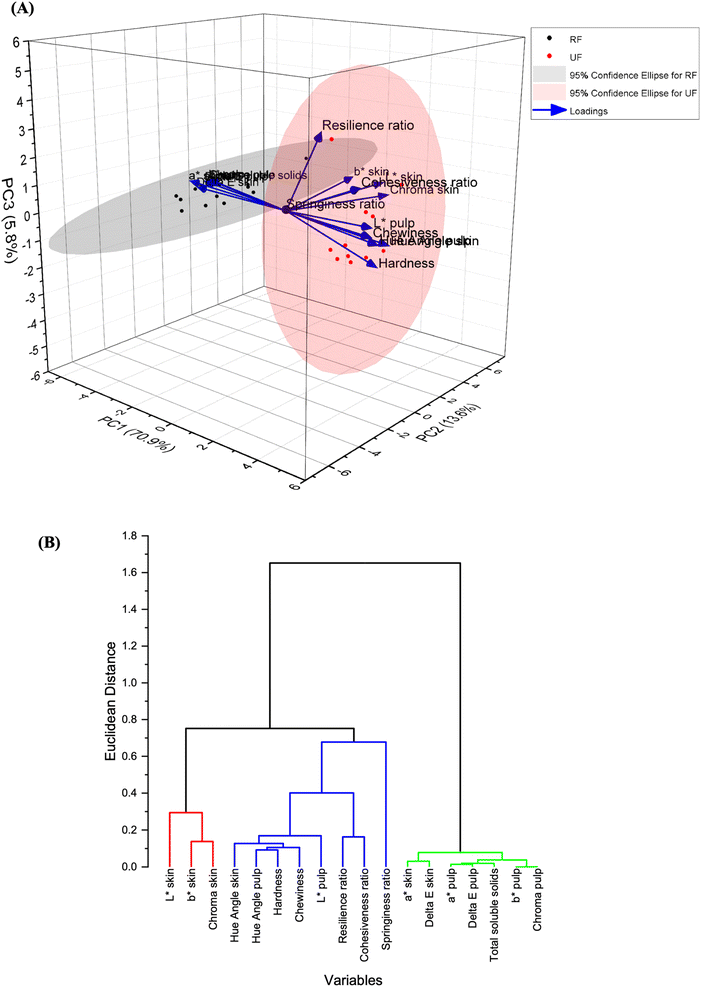 |
| Fig. 5 (A) Principal component analysis biplot and (B) hierarchical cluster analysis dendrogram of the ripe and unripe pawpaw fruits based on their fruit and pulp color, total soluble solids, and textural properties. | |
4. Discussion
4.1 Color of fruit and pulp
The color of the pawpaw fruit and pulp was determined using the Hunter L, a, b color system. The data obtained were used to calculate the chroma, which is an indication of color saturation; total color difference using the unripe color data as the initial data and the ripe color data as the final data; and hue angle, which shows the quadrant the color of the sample lies in within two-dimensional space.10,12 The unripe fruits had higher L* values than the ripe fruits because as pawpaw fruits ripen their skin darkens. Further, the darkening of the skin becomes intense in the first few hours after harvesting if the fruits are bruised. The darkening of the ripe fruits could also be attributed to the method of harvesting, which involved allowing the fruits to fall to the ground, possibly resulting in some mechanical damage thereby affecting the lightness of the ripe fruits. Since negative a* values indicate green color and positive a* values indicate red color, it is clear that the unripe fruits have a greener skin color compared to the ripe fruits. The skin color of the ripe fruits studied was lighter, and greener than those obtained by Adainoo and others13 in a study in which ripe fruits had been frozen for weeks before analyses.
The slightly higher chroma of the unripe fruits may be a result of the darkening in the skin of the ripe fruits from the sustained bruises during harvesting (Fig. 2). In addition, the total color difference (Delta E) shows the degree to which the color of the ripe fruits differs from that of the unripe fruits. Delta E values range from 0 to 100 with a Delta E value of 0 meaning the two colors being compared are mathematically exact and a Delta E value of 100 meaning the widest visible difference between the two colors being compared. A Delta E value lower than 0.3 is not visible to the human eye, whereas a Delta E value of 2 is the minimum for visually detecting the difference between saturated colors.14 Further, the visible total color difference between the unripe and ripe fruits is shown in the hue angle data obtained. However, based on the hue angle data obtained, the variation in the color of the ripe fruits shows that a pawpaw fruit may be ripe and still have a color that lies in the same quadrant as an unripe fruit as shown in Fig. 2D. Therefore, the skin hue angle of the fruit may be used as an index for determining the ripeness of pawpaw fruits, but it may not be a good predictor of pawpaw ripeness for all fruits. Nevertheless, previous studies have noted that as pawpaw fruits ripen, their hue angle decreases and fruits with hue angles of about 100° or lower typically have high total headspace aroma volatile contents which are indicative of ripe fruits.8
Based on the wider differences between the colors of the unripe pulp and ripe pulp compared to that of the unripe and ripe fruit, pulp color might be a better predictor of pawpaw ripeness. However, since determining the color of the pulp is invasive, it may not be a productive option for determining pawpaw ripeness. Nonetheless, the color data obtained in this experiment were consistent with the data obtained for four cultivars of pawpaw analyzed by Mcgrath and Karahadian8 and the data obtained for eight cultivars of pawpaw analyzed by Adainoo and others.13
4.2 Texture profile of fruits
The textural properties of the fruits determined in this study were the hardness, chewiness, cohesiveness, springiness, and resilience ratio of the fruits. Hardness is given by the maximum force of the first compression.15 The hardness indicates how much force must be applied to the fruit to compress it by 10 mm (based on the settings of the texture analyzer used in this study). Fruit hardness is a characteristic that is dependent on the elasticity modulus (the measure of how elastic a material is) and visco-elastic properties of the fruit.16 These physical properties are influenced by the composition of the fruit. For the pawpaw fruits analyzed, the high fiber content may explain the high hardness values for the unripe fruits.17 Studies show that there is a positive linear correlation between hardness and pectin content, and a negative linear correlation between hardness and crude fiber and moisture content.18 However, there are currently no published data on the various polysaccharides in the pawpaw fruit to draw a conclusive inference. The hardness of the ripe wild pawpaw fruits used in this study was higher than the hardness of ripe ‘Shenandoah’ pawpaw fruits reported by Zhang and others19 but lower than that of the tree-ripened pawpaw fruits studied by Mcgrath and Karahadian.8 Additionally, the hardness of the ripe pawpaw fruits was similar to the hardness of freshly harvested green ripe mangoes (2.5 kg-force) but higher than that of freshly harvested green ripe bananas (1.28 kg-force).16,20
Cohesiveness is a measure of the strength of the internal bonds that keep the food sample intact.15,21 In a TPA, cohesiveness is given by the sum of the second area of compression and retraction (A2) divided by the sum of the first area of compression and retraction (A1) expressed as a percentage as shown in the eqn (5) above. The unripe fruits had a higher cohesiveness compared to the ripe fruits possibly because as the fruit ripens, the conversion of the complex sugars into simple sugars loosens up the structure, making the ripe fruits less cohesive than the unripe fruits. In a study conducted with date fruits, it was observed that there was a positive correlation between the arabinoxylan (a polysaccharide mainly found in the cell wall of some plants) concentration and the cohesiveness of the fruits (r = 0.623, p < 0.01), but there was no significant correlation between the total fiber content and the cohesiveness.21 A similar conclusion may be drawn for the unripe pawpaw fruits, however, there is a need for further studies into the various polysaccharides present in the fruit at that stage of maturity to adequately arrive at a similar conclusion. Due to the relatively low cohesiveness of the ripe fruits, it may not be suitable to pack a lot of fruits on top of each other during transportation or at the point of sale as this may result in the deformation of the fruit, making it less appealing to consumers.
Chewiness is the energy needed to chew the fruit until it is ready to swallow.15 It is given by the product of hardness, cohesiveness, and springiness. While the chewiness values obtained for the ripe pawpaw fruits were within the chewiness range of apples (0.54–1.55 kg-force), the values obtained for the unripe fruits (167.6 ± 20.4 kg-force) were too high above the chewiness range of apples.22 This may be due to the presence of high levels of polysaccharides like pectin and starch which have not yet been converted into soluble sugars in the unripe fruit. In most fruits, the conversion of polysaccharides into soluble sugars during ripening is accompanied by a decrease in the amount of energy needed to chew the fruit until it is ready to swallow.
Resilience ratio is a measure of how a sample fights to return its original height after the first compression in a TPA before the waiting period starts. Results from this study clearly show that the unripe fruits have a higher resilience ratio compared to the ripe fruits. This may have been due to the higher strength of the internal bonds in the unripe fruits as shown in the cohesiveness ratio values. The stronger internal bonds in the unripe fruits may have contributed to the recovery of the original height of the samples after the first TPA compression, whereas the weaker bonds in the ripe fruits resulted in a much lower resilience ratio. These findings are key in the packaging and transportation of pawpaw fruits to avoid deforming the fruits before they reach the target market since resilience of fruits is a key quality index among consumers.23
Springiness is a dimensionless measure of the elasticity of a sample; it is a measure of a sample's ability to return to its original form when the force of deformation is removed. A higher springiness indicates a high sample elasticity. It is determined by the ratio between the residual displacement and maximum displacement.24 The springiness ratios obtained for both unripe and ripe pawpaw fruits in this study were higher than the springiness reported for fruits like fresh blueberries (0.46–0.69), apples (0.88), and bayberry fruits (0.95) but lower than the maximum springiness ratio of date fruits (5.3).12,18,24,25 This relatively high springiness ratio of the pawpaw fruits compared to the fruits mentioned above may be largely due to the differences in fruit cell size and tissue layers.26,27 Further, the data obtained show that the springiness of the unripe and ripe fruits is not very different even though the unripe fruits have a slightly higher springiness ratio value. Studies in other fruits like blueberries show that springiness is often maintained during the early stages of storage after harvesting, while in other fruits like dates, springiness is highest at the moisture content of about 21.5%.18,24 Since both unripe and ripe pawpaw fruits in the present study were not stored for more than 24 hours prior to their analysis, their high springiness may have been maintained by their moisture contents. Future studies should investigate the storage conditions and the moisture contents of pawpaw fruits and how they relate to their springiness ratio and other textural properties to gain deeper insights.
4.3 Correlation analysis of measured quality characteristics
Skin color by itself has generally been known not to be a good indicator of pawpaw fruit ripeness. Farmers typically determine the ripeness of pawpaw fruits by their hardness (or firmness) by touch. However, since the fruits have a thin peel thickness, there is a high potential for breaking the skin through checking the hardness by touch.13 Currently, no research has been published testing the correlations between the skin color, which can be determined noninvasively, the textural properties, which are currently the main indices for assessing pawpaw ripeness, and the total soluble solids content (Brix). Establishing good correlations between these two physical properties and the total soluble solids contents will enable farmers to use commercially available portable devices (like handheld color meters and near-infrared total soluble solids sensors) to noninvasively determine the ripeness of pawpaw fruits. Potentially, future studies could also explore the use of unmanned aerial vehicles (drones) with artificial intelligence systems to monitor farms for pawpaw fruit skin color parameters to ensure prompt harvesting of fruits to prevent postharvest losses as has been done for fruits like strawberries and apples.28,29
Since it has already been established that the textural properties like hardness and cohesiveness ratio of pawpaw fruits are better indicators of ripeness, Pearson's correlation analysis was performed to test the relationship between the color parameters and the textural properties to evaluate which color parameters correlate with the textural properties and may be used as indicators of ripeness. Studies on climacteric fruits like apples and bananas show that specific color parameters like a* values, b* values, and hue angles can be used as indicators of ripeness.6,30 According to Mcgrath and Karahadian,8b* values (degree of yellowness) are good indicators of pawpaw ripeness and not a* values. However, the findings in this study indicate otherwise. From the correlations, it can be inferred that skin a* value (degree of greenness), total color difference, and hue angle are better non-destructive indicators of pawpaw fruit ripeness compared with the other skin color indices and the pulp color parameters which are destructive. Based on this, skin a* value, total color difference, and hue angle of pawpaw fruits may be more useful in predicting the ripeness of pawpaw fruits. Skin lightness (L*) values, b* values (degree of yellowness), and chroma had relatively weak correlations with the textural properties of the pawpaw fruits, hence, these skin color parameters may not be suitable indicators of pawpaw fruit ripeness. There were strong negative and positive correlations between the pulp color parameters and the textural properties, and between the total soluble solids and the textural properties of the fruits as shown in the correlation plot and correlation matrix (Fig. 4 and Table 3). However, since the determination of the pulp color and total soluble solids is destructive, they would not be productive indices for determining fruit ripeness on a large scale. This further shows the need for identification of non-invasive methods to determine the ripeness of underutilized pawpaw fruits.
4.4 Multivariate analysis of measured quality characteristics
The principal component analysis (PCA) was used to reduce the dimensionality of the data matrix and highlight the differences in the quality characteristics of the pawpaw fruits. The total variance contribution rate of the first three principal components (PCs) is 90.20% (more than 85%), hence, most information of the original data i.e., 90.20% of the total variance of the color, total soluble solids, and texture data, can be explained by the first three PCs.31 From the biplot, the ripe fruits are separated from the unripe fruits based on the PCA scores calculated with the color, texture, and total soluble solids data obtained in this study. The extracted eigenvectors for the PCA show that PC1 was mainly contributed to positively by skin hue angle, pulp hue angle, chewiness, pulp L* values, and hardness. On the other hand, PC1 was negatively contributed to by pulp total color difference, skin total color difference, total soluble solids, pulp a* values, and pulp b* values. For PC2, the skin b* values, skin chroma, and skin L* were the main variables that had main positive contributions; whereas springiness ratio, resilience ratio, cohesiveness ratio, and skin a* values were the main variables that negatively contributed to PC2. PC3 was mainly positively contributed to by the resilience ratio, cohesiveness ratio, skin L* values, and springiness ratio, while it was mainly negatively contributed to by the hardness and the skin hue angle. In the PCA biplot, the unripe fruits (UF) appeared mostly on the positive side of PC1 and PC2 while the ripe fruits (RF) appeared mostly on the negative side of PC1, with some on the positive side of PC3. This shows that largely, there are clear differences between ripe and unripe pawpaw fruits based on their textural and color characteristics.
Hierarchical cluster analysis (HCA) was applied using Euclidean distance as a measure of similarity, and the dendrogram showed three distinct clusters, enabling a good classification of the properties to determine the ripeness of pawpaw fruits (Fig. 5B). The skin a*, skin total color difference, pulp a*, pulp total color difference, total soluble solids, pulp b*, pulp chroma, and skin hue angle were grouped in the same cluster, indicating the close relation of these quality indices in showing the ripeness of pawpaw fruits. This confirms the results from the PCA since these same quality indices were closely packed together on the negative side of PC1 in the score plot (Fig. 5A).
5. Conclusion
Pawpaw fruit remains underutilized as a specialty crop, with very little published data on the properties of the cultivated pawpaw fruit and even less data on established objective indicators of ripeness. The data from this study show that unripe and ripe pawpaw fruits have significantly different skin and pulp colors. Unripe pawpaw fruits are much harder and have a higher chewiness than ripe pawpaw fruits. Due to the conversion of complex sugars to simpler sugars that occurs during ripening, the ripe pawpaw fruits have a lower cohesiveness than unripe fruits. As a result, it may not be suitable for ripe pawpaw fruits to be packed on top of each other during transportation. From the correlation analysis, it was found that the skin a* values and hue angles have a strong correlation with the textural properties of the fruits, hence, the skin a* values and hue angles can be used as noninvasive indices of pawpaw ripeness. Potentially, farmers can use commercially available handheld color meter and Brix meters to determine pawpaw fruit ripeness noninvasively based on specific skin color indices. This study was conducted on wild fruit from one location and results may be different for specific cultivars of pawpaw grown in different environments. The findings from this study establish a foundation for further research on better indicators of pawpaw fruit ripeness solutions to prevent pawpaw fruit loss.
Author contributions
Bezalel Adainoo: conceptualization, methodology, investigation, data curation, formal analysis, writing – original draft, visualization, writing – review & editing; Andrew L. Thomas: resources, writing – review & editing; Kiruba Krishnaswamy: conceptualization, funding acquisition, writing – review & editing.
Conflicts of interest
There are no conflicts to declare.
Acknowledgements
This work is supported by the University of Missouri Center for Agroforestry and the USDA/ARS Dale Bumpers Small Farm Research Center, Agreement number 58-6020-0-007 from the USDA Agricultural Research Service. This work is supported by the Food Engineering and Sustainable Technologies (FEAST) lab through (USDA) Hatch Funds (MO-HAFE0003).
References
- K. W. Pomper and D. R. Layne, The North American Pawpaw: Botany and Horticulture, Hortic. Rev., 2010, 31, 349–382 Search PubMed.
- D. Donno, G. L. Beccaro, M. G. Mellano, A. K. I. M. Cerutti and G. Bounous, Chemical Fingerprinting as Nutraceutical Quality Differentiation Tool in Asimina triloba L. Fruit Pulp at Different Ripening Stages: An Old Species for New Health Needs, J. Food Nutr. Res., 2014, 53(1), 81–95 CAS.
-
K. W. Pomper, S. B. Crabtree and J. D. Lowe, Pawpaw Cultivars and Grafted Tree Sources, Kentucky State University, 2009, https://www.kysu.edu/academics/college-acs/school-of-ace/pawpaw/2009-pawpaw-cultivars-and-grafted-tree-sources.php, accessed 2021-04-18 Search PubMed.
- F. M. A. Mazen and A. A. Nashat, Ripeness Classification of Bananas Using an Artificial Neural Network, Arabian J. Sci. Eng., 2019, 44(8), 6901–6910, DOI:10.1007/S13369-018-03695-5.
- K. Ratprakhon, W. Neubauer, K. Riehn, J. Fritsche and S. Rohn, Developing an Automatic Color Determination Procedure for the Quality Assessment of Mangos (Mangifera indica) Using a CCD Camera and Color Standards, Foods, 2020, 9(11), 1709, DOI:10.3390/FOODS9111709.
- C. Lang and T. Hübert, A Colour Ripeness Indicator for Apples, Food Bioprocess Technol., 2012, 5(8), 3244–3249, DOI:10.1007/S11947-011-0694-4/FIGURES/5.
- D. D. Archbold, R. Koslanund and K. W. Pomper, Ripening and Postharvest Storage of Pawpaw, Horttechnology, 2003, 13(3), 439–441, DOI:10.21273/HORTTECH.13.3.0439.
- M. J. Mcgrath and C. Karahadian, Evaluation of Physical, Chemical and Sensory Properties of Pawpaw Fruit (Asimina triloba) as Indicators of Ripeness, J. Agric. Food Chem., 1994, 42, 968–974 CrossRef CAS.
- V. E. Nambi, K. Thangavel, S. Shahir and V. Geetha, Evaluation of Colour Behavior during Ripening of Banganapalli Mango Using CIE-Lab and RGB Colour Coordinates, J. Appl. Hortic., 2015, 17(3), 205–209 CrossRef.
- M. R. McLellan, L. R. Lind and R. W. Kime, Hue Angle Determinations and Statistical Analysis for Multiquadrant Hunter L, a, b Data, J. Food Qual., 1995, 18(3), 235–240, DOI:10.1111/j.1745-4557.1995.tb00377.x.
-
AOAC, Official Methods of Analysis of the Association of Analytical Chemists, Association of Official Analytical Chemists, 2000 Search PubMed.
- Z. Yang, Y. Zheng, S. Cao, S. Tang, S. Ma and N. Li, Effects of Storage Temperature on Textural Properties of Chinese Bayberry Fruit, J. Texture Stud., 2007, 38(1), 166–177, DOI:10.1111/J.1745-4603.2007.00092.X.
- B. Adainoo, B. Crowell, A. L. Thomas, C.-H. Lin, Z. Cai, P. Byers, M. Gold and K. Krishnaswamy, Physical Characterization of Frozen Fruits from Eight Cultivars of the North American Pawpaw (Asimina triloba), Front. Nutr., 2022, 2393, DOI:10.3389/FNUT.2022.936192.
-
N. N. Bhookya, R. Malmathanraj and P. Palanisamy, Yield Estimation of Chili Crop Using Image Processing Techniques, in 2020 6th International Conference on Advanced Computing and Communication Systems (ICACCS), IEEE, Coimbatore, India, 2020, pp. 200–204 Search PubMed.
-
S. Kasapis and A. Bannikova, Rheology and Food Microstructure, in Advances in Food Rheology and Its Applications, ed. J. Ahmed, Woodhead Publishing, Sawston, England, 2017, pp. 7–46, DOI:10.1016/B978-0-08-100431-9.00002-4.
- X. Huang, R. Lv, S. Wang, J. H. Aheto and C. Dai, Integration of Computer Vision and Colorimetric Sensor Array for Nondestructive Detection of Mango Quality, J. Food Process Eng., 2018, 41(8), e12873, DOI:10.1111/JFPE.12873.
- S.-Y. Park, H.-L. Jang and J.-S. Nam, Comparison of Nutritional Compositions and Physicochemical Properties of Unripe and Ripe Pawpaw (Asimina triloba [L.] Dunal) Fruits Grown in Korea, J. Korean Soc. Food Sci. Nutr., 2022, 51(9), 933–941 CrossRef CAS.
- V. Singh, N. Guizani, A. Al-Alawi, M. Claereboudt and M. S. Rahman, Instrumental Texture Profile Analysis
(TPA) of Date Fruits as a Function of Its Physico-Chemical Properties, Ind. Crops Prod., 2013, 50, 866–873, DOI:10.1016/J.INDCROP.2013.08.039.
- L. Zhang, S. Dai and R. G. Brannan, Effect of High-Pressure Processing, Browning Treatments, and Refrigerated Storage on Sensory Analysis, Color, and Polyphenol Oxidase Activity in Pawpaw (Asimina triloba L.) Pulp, LWT–Food Sci. Technol., 2017, 86, 49–54, DOI:10.1016/j.lwt.2017.07.023.
- M. Soltani, R. Alimardani, M. Omid and I. Karaj, Changes in Physico-Mechanical Properties of Banana Fruit during Ripening Treatment, J. Am. Sci., 2011, 7(5), 14–19 Search PubMed.
- A. Kamal-Eldin, N. George, B. Sobti, N. AlRashidi, S. Ghnimi, A. A. Ali, A. A. M. Andersson, R. Andersson, A. Antony and F. Hamed, Dietary Fiber Components, Microstructure, and Texture of Date Fruits (Phoenix dactylifera, L.), Sci. Rep., 2020, 10(1), 1–11, DOI:10.1038/s41598-020-78713-4.
- R. P. F. Guiné, S. Andrade, A. C. Correia, A. M. Jordão, A. D. Lopes and D. Ferreira, Evaluation of Textural Properties in Apples of Regional Varieties, Int. J. Food Prop., 2011, 14(2), 331–338, DOI:10.1080/10942910903177848.
- A. Lázaro and C. de Lorenzo, Texture Analysis in Melon Landraces through Instrumental and Sensory Methods, Int. J. Food Prop., 2015, 18(7), 1575–1583, DOI:10.1080/10942912.2014.923441/SUPPL_FILE/LJFP_A_923441_SM5992.ZIP.
- C. Li, J. Luo and D. Maclean, A Novel Instrument to Delineate Varietal and Harvest Effects on Blueberry Fruit Texture during Storage, J. Sci. Food Agric., 2011, 91(9), 1653–1658, DOI:10.1002/JSFA.4362.
- S. Najafi Marghmaleki, S. M. H. Mortazavi, H. Saei and A. Mostaan, The Effect of Alginate-Based Edible Coating Enriched with Citric Acid and Ascorbic Acid on Texture, Appearance and Eating Quality of Apple Fresh-Cut, International Journal of Fruit Science, 2020, 21(1), 40–51, DOI:10.1080/15538362.2020.1856018.
- L. Giongo, P. Poncetta, P. Loretti and F. Costa, Texture Profiling of Blueberries (Vaccinium Spp.) during Fruit Development, Ripening and Storage, Postharvest Biol. Technol., 2013, 76, 34–39, DOI:10.1016/J.POSTHARVBIO.2012.09.004.
- P. A. McAtee, I. C. Hallett, J. W. Johnston and R. J. Schaffer, A Rapid Method of Fruit Cell Isolation for Cell Size and Shape Measurements, Plant Methods, 2009, 5(1), 1–7, DOI:10.1186/1746-4811-5-5/FIGURES/5.
- X. Zhou, W. S. Lee, Y. Ampatzidis, Y. Chen, N. Peres and C. Fraisse, Strawberry Maturity Classification from UAV and Near-Ground Imaging Using Deep Learning, Smart Agricultural Technology, 2021, 1, 100001, DOI:10.1016/J.ATECH.2021.100001.
- G. Sun, X. Wang, H. Yang and X. Zhang, A Canopy Information Measurement Method for Modern Standardized Apple Orchards Based on UAV Multimodal Information, Sensors, 2020, 20(10), 2985, DOI:10.3390/S20102985.
- M. Soltani, R. Alimardani and M. Omid, Comparison of Some Chromatic, Mechanical and Chemical Properties of Banana Fruit at Different Stages of Ripeness, Mod. Appl. Sci., 2010, 4(7), 34–41 CrossRef CAS.
- M. Liu, J. Wang, D. Li and M. Wang, Electronic Tongue Coupled with Physicochemical Analysis for the Recognition of Orange Beverages, J. Food Qual., 2012, 35(6), 429–441, DOI:10.1111/JFQ.12004.
|
This journal is © The Royal Society of Chemistry 2023 |
Click here to see how this site uses Cookies. View our privacy policy here.