Partial nitrification/ANAMMOX (PN/A) membrane aerated biofilm reactor (MABR) under a low C/N ratio and metagenomics identification†
Received
28th September 2022
, Accepted 27th October 2022
First published on 28th November 2022
Abstract
The PN/A MABR has attracted much attention as a promising low-cost nitrogen removal technology with a lower carbon footprint. This study explored the minimum C/N ratio of the PN/A MABR. It was found that low lumen pressure (2–5 kPa) was beneficial for a MABR to achieve a partial nitrification process. Also, the ANAMMOX process can be coupled with partial nitrification in the MABR under low C/N conditions (<1.5). The total nitrogen removal efficiency (TNRE) can be seriously affected by DO under a low C/N ratio, even with minor fluctuations. When the C/N ratio was 3–4, the PN/A MABR can achieve a higher TNRE and the effluent water quality was more stable. The pH difference (ΔpH) between the influent and the effluent can reflect the influence of C/N and dissolved oxygen (DO) on the PN/A process. ΔpH was negatively correlated with the C/N ratio and positively correlated with DO. This study also found that Nitrosomonas, Nitrospira, Denitratisoma, Thauera, Candidatus Kuenenia, and Candidatus Brocadia played important roles in the synergy of nitrogen removal in the PN/A MABR by microbial population structure analysis using 16S rRNA and metagenomic sequencing identification techniques. The research results laid an application foundation for low C/N ratio sewage nitrogen removal and provided a technical reference for wastewater treatment plants (WWTPs) that treat municipal wastewater with a PN/A MABR.
Water impact
This study investigated the operation of a PN/A MABR at low C/N ratios and explored the case of a C/N ratio of 0. The ANAMMOX process can effectively be coupled with partial nitrification and denitrification processes in the MABR. It was also found that DO has a serious influence on the total nitrogen removal efficiency in the reactor under the condition of low C/N ratios (<1.5). This work provided insights and a reference for low-cost nitrogen removal with a PN/A MABR.
|
1. Introduction
The MABR has attracted more and more attention because it has many advantages, such as accurate aeration, simple operation, and lower costs.1,2 Air is pumped into gas permeable membranes and the oxygen flux can be adjusted accurately by means of the flow rate and lumen pressure.3 Microorganisms efficiently obtain oxygen and space by membranes, forming aerobic, anoxic, and anaerobic microenvironments according to the DO concentration gradient from the substratum to the biofilm surface.4 Ammonia-oxidizing bacteria (AOB), ammonia-oxidizing archaea (AOA), ANAMMOX bacteria, nitrite-oxidizing bacteria (NOB), and denitrifying bacteria (DB) can keep in close touch with extracellular polymeric substances (EPSs) and quorum sensing (QS) factors to synergize nitrogen removal in a MABR.5–7 The substrates diffuse from the wastewater to the biofilm, and oxygen diffuses from the hollow membrane to the biofilm, forming a counter-diffusion phenomenon in the MABR, which can improve the nitrogen removal efficiency.8–10
In the recent 20 years, the ANAMMOX process as the most economical and efficient nitrogen removal process has been widely studied. It can use nitrite to oxidize ammonium directly into dinitrogen gas without organics and aeration.11,12 This process can contribute to low-carbon emissions for WWTPs.10 However, mainstream PN/A is rarely successfully applied in engineering.13 Nitrite accumulation14,15 and ANAMMOX bacteria enrichment and retention16 are the pivotal technical bottlenecks. AOB can convert ammonia into nitrite, but NOB can easily further oxidize nitrite into nitrate. So the PN/A process requires suppressing NOB activity and reducing the abundance of NOB to minimalize the competition for nitrite and increase the nitrite accumulation rate (NAR).17 Further, in different reactors and under different DO conditions, the specific growth rates of AOB and NOB are different.18 For example, in membrane bioreactors, the maximum specific growth rates of AOB and NOB are 0.63 d−1 and 0.56 d−1, respectively, when DO is greater than 1.5 mg L−1. However, the maximum specific growth rates of AOB and NOB are 0.50 d−1 and 0.14 d−1, respectively.19 This indicates that the accurate control of DO is of great importance for the enrichment of AOB and the inhibition of NOB. A MABR can effectively control the oxygen flux, realize nitrite accumulation, and provide the necessary space for ANAMMOX bacteria.3 So, integrating MABR technology with the PN/A process could amplify their respective advantages for sewage treatment.14
Wastewater carbon pretreatment involves the flocculation, adsorption, and degradation of organics.20,21 The organic carbon should be captured or transferred as much as possible to reduce its impact on autotrophic nitrogen removal in the case of ensuring the carbon demand of subsequent biochemical processes.22,23 Maximizing carbon pretreatment was also conducive to resource recovery24 and energy conversion.25 At present, studies on wastewater carbon pretreatment are mainly focused on coagulation precipitation,26 biological flocculation,27 hybrid pretreatment,28 and bioreactors.30 Ritigala treated swine wastewater with magnetic coagulation, and the removal rates of NH4+–N and COD were 7.82% and 40.25%, respectively.29 Capodici used a sequencing batch reactor (SBR) for the PN process and 40% COD was utilized for denitrification, and the nitrite accumulation rate reached 71.43%.30 Through the above analysis, partial COD in sewage can be removed by pretreatment. This resulted in C/N reduction to varying degrees, depending on different pretreatment processes. A question arises: what is the minimum C/N ratio for a PN/A MABR?
In order to explore the minimum C/N ratio of a PN/A MABR, this work studied the start-up and operation of PN/A MABR feeding low C/N ratio (<1.5) artificial wastewater. The operation of the reactor was analyzed by means of ΔpH. 16S rRNA and metagenomics were also conducted for microbial identification and analysis.
2. Materials and methods
2.1 Materials and equipment
A cylindrical plexiglass container (height 900 mm, diameter 150 mm, and effective volume 15 L) was used. The device had an MABR integrated membrane module. The MABR integrated membrane module was composed of 150 membrane filaments (Hydroking Sci. & Tech. Ltd., China), and the MABR membrane filaments were oxygen-permeable hollow fiber membranes (wall thickness 197 μm, outer diameter 770 μm, and length 1.5 m). So, the specific surface area in this reactor was 38.5 m2 m−3. The inlet end of the integrated membrane module was connected to an air compressor by polyurethane (PU) tubes. Pressure regulators and pressure gauges were installed on the air inlet line. Another end of the integrated membrane module was a vent. Simulated wastewater was pumped into the reactor from the bottom. A magnetic drive pump was used to promote mass transfer in the reactor. Then, the effluent left the reactor via an overflow orifice (Fig. 1).
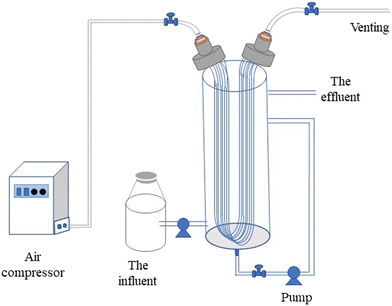 |
| Fig. 1 Schematic diagram of the MABR system in the study. | |
2.2 PN/A MABR start-up
The activated sludge from the aeration tank at Nankai University was used as an inoculant. ANAMMOX bacteria were also inoculated after the MABR biofilm had formed and the effluent nitrite accumulation rate exceeded 85%. The reactor was at room temperature. The influent flow rate was 0.63 L h−1, the hydraulic retention time was about 24 h, and the aeration pressure ranged from 1 to 5 kPa. The cycle ratio was 150–200%. The pH fluctuated between 7.71 and 8.61. The influent contained 50 mg L−1 NH4+–N (provided by NH4Cl), and the concentration of COD (CH3COONa, 0–200 mg L−1) was different. Microelements were referenced.31 The average substrate concentration and C/N ratio in the influent are shown in Table 1.
Table 1 Average substrate concentration in the influent
|
1–7 days |
8–51 days |
52–70 days |
71–105 days |
Average NH4+–N mg L−1 |
45.75 |
45.71 |
49.70 |
52.19 |
Average COD mg L−1 |
64 |
37 |
0 |
173 |
Average C/N |
1.4 |
0.8 |
0 |
3.3 |
2.3 Analytical methods
The detection indexes of the influent and effluent water from the reactor included NH4+–N, NO2−–N, NO3−–N, and COD. Before each test, the sample was filtered using a 0.45 μm filter membrane. Then, these indicators were detected according to Lan's study.32 The pH and DO values were measured using HQ30d (Hach Instruments Inc., USA). The sludge suspension concentration was obtained by the weighing method.33
16S rRNA sequencing and metagenomics were used to analyze the microbial population structure based on Illumina high-throughput sequencing platforms according to prescribed methods. 16S rRNA sequencing mainly included the following steps. Extract the total RNA, and then use 16S rDNA specific primers (338F and 806R) for amplification after reverse transcription, construct a high-throughput sequencing library, and analyze the 16S rDNA V3–V4 region sequence to identify the composition of prokaryotic microorganisms.34 Metagenomics was performed using the paired-end method to construct small fragment libraries for sequencing. Information on gene function and species composition was obtained through biological information analysis.35
2.4 Calculation methods
NAR (%) in the MABR was calculated using eqn (1):36 | NAR = NO2,e−–N/(NO2,e−–N + NO3,e−–N) | (1) |
where NO2,e−–N and NO3,e−–N are the effluent NO2−–N and NO3−–N concentrations, respectively (mg L−1).
TNRE (%) was calculated according to eqn (2):
| TNRE = (NH4,i+–N–NH4,e+–N–NO2,e−–N–NO3,e−–N)/NH4,i+–N | (2) |
where NH
4,i+–N and NH
4,e+–N are the influent NH
4+–N and effluent NH
4+–N concentrations, respectively (mg L
−1).
ΔpH between the influent and effluent was also calculated:
where pH
inf is the pH of the influent water and pH
eff is the pH of the effluent water in this reactor. ΔpH is the difference between pH
inf and pH
eff.
3. Results and discussion
3.1 Performance of nitrogen removal
Before the establishment of PN/A, the MABR membrane filaments were immersed in activated sludge for three weeks to form a biofilm rapidly, during which NH4Cl (50 mg NH4+–N L−1) and CH3COONa (50 mg COD L−1) were regularly added. The start-up (days 1 to 20) of the partial nitrification MABR and the shifts of nitrogen and COD concentrations of the PN/A MABR are shown in Fig. 2.
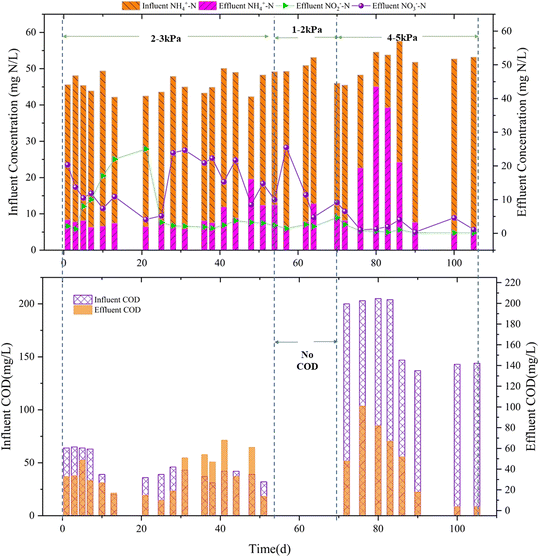 |
| Fig. 2 The shifts of inorganic nitrogen and COD concentrations in the MABR. | |
The average influent NH4+–N and COD concentrations were 45.72 mg L−1 and 43.88 mg L−1, respectively, forming a C/N ratio of less than 1.44 in the first 51 days (Fig. 2). The NO2−–N concentration gradually increased and reached 25 mg L−1 on day 21 by controlling the lumen pressure (Pg) to within 2 kPa to 3 kPa, and NAR was 85.91%. Chen et al. also controlled the MABR lumen pressure to suppress NOB activity and achieved more than 84% NAR.37 Different Pg values made the oxygen penetration depth different in MABR biofilms. Wang et al. found that when Pg was 2 kPa, the oxygen penetration depth was 112 μm.38 In other words, the thickness of the aerobic layer where nitrification occurred was 112 μm in Wang's study. The nitrification layer was at the substratum and was the first to come into contact with the oxygen from hollow membranes in the MABR;39 therefore, Pg directly affected the nitrification process. Further, Regmi et al. recently found that the oxygen half-saturation coefficient of NOB (0.16 mg O2 L−1) was smaller than that of AOB (1.16 mg O2 L−1).39 Wu et al. had similar findings.40 According to Wang's and Wu's studies even if the aeration pressure was 2 kPa, the DO (3.0–3.5 mg L−1) at the MABR biofilm substratum was still greater than the oxygen half-saturation coefficients of AOB and NOB,38 and the oxygen uptake rate (OUR) of AOB was higher than that of NOB,40 which was conducive to AOB reproduction and NO2−–N accumulation.38 Also, in this study, Pg was 2–3 kPa (Fig. 2), which may be the reason for NO2−–N accumulation at this stage. When Pg was less than 2 kPa, the DO at the biofilm substratum may not be very small, because the biofilm substratum was directly in contact with the air from the hollow membrane.37,38 However, a much higher lumen pressure was not suitable for low C/N ratio wastewater because when the lumen pressure was higher, the biofilm of the MABR was thicker, which affected the mass transfer efficiency and TNRE.41,42
After inoculation with ANAMMOX bacteria (day 23), the NH4+–N removal efficiency reached 96.89%, and the TNRE reached 73.26%. Afterwards, due to air compressor replacement, the DO of the reactor suddenly increased to 0.59–0.78 mg O2 L−1, resulting in a high concentration of NO3−–N in the effluent, which affected the TNRE. Under a low C/N ratio (<1), NO3−–N may not be easy to decrease because common heterotrophic microorganisms also use organics, resulting in inadequate denitrification,43 let alone at low concentrations of 31–46 mg COD L−1 in the stage. After reducing the lumen pressure, the reactor was operated for another 23 days, and the NO3−–N concentration only decreased to 8.5–14.8 mg L−1 from 23.9–24.7 mg L−1.
In theory, partial nitrification and ANAMMOX processes can degrade nitrogen with little organic carbon, which is also an advantage of the PN/A process.31 In order to study the performance of the reactor under extreme C/N ratio conditions, from day 52 to day 70, the reactor was fed without any organics and the average influent NH4+–N concentration was 49.70 mg L−1. The average NH4+–N removal efficiency was 89.07% with the highest at 96.55%, as shown in Fig. 2. When the DO in the reactor was 0.32 mg L−1 and the pH was 8.01, the TNRE was 70.56%. At this stage, the DO in the reactor had a particularly significant effect on TNRE, even though it fluctuated in a small range. For example, when the DO was raised from 0.55 mg L−1 to 0.75 mg L−1 in the reactor, the TNRE decreased from 57.74% to 41.89%. The oxygen in the reactor was originally used for the nitrification process and aerobic heterotrophic process. Since the organic matter was reduced to 0, the oxygen in the reactor was mainly used for the nitrification process,44 resulting in the DO rising to 0.75 mg L−1 and the pH difference fluctuating from 0 to 0.47 (Fig. 3). A high DO suppresses the activity of anaerobic bacteria, including ANAMMOX bacteria and DB.10 Hence, the weakening of the denitrification and ANAMMOX processes affected the TNRE.
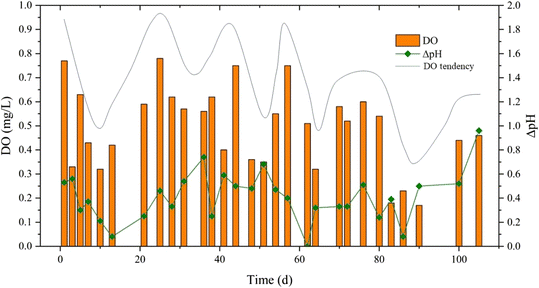 |
| Fig. 3 ΔpH, DO and DO tendency in the PN/A MABR. | |
The aforementioned demonstrated that, on the whole, the reactor had a fine nitrogen removal performance under a low C/N ratio (Fig. 2). Even if the influent C/N was 0, the MABR can also remove most inorganic nitrogen. This may be because Pg (1–2 kPa) reduced the activity of common heterotrophs and the thickness of the biofilm,38 improved the mass transfer efficiency and ensured the partial nitrification process.10,37 In addition, it was also verified that a partial nitrification MABR can entrap ANAMMOX bacteria and realize a PN/A MABR.9
This study has proved that the PN/A MABR can effectively remove nitrogen under a low C/N ratio, but the operating parameters were extremely stringent. In order to further prove that the PN/A MABR can have a better nitrogen removal effect and that the C/N ratio can affect the microbial complexity, the influent C/N ratio was adjusted to 3–4 on days 71 to 105. The TNRE decreased at first, and then increased (Fig. 2). On the one hand, the decrease may be because the microorganisms including the ANAMMOX bacteria had adapted to the low C/N ratio environment.45 On the other hand, it may be because heterotrophic bacteria competed with AOB for oxygen, resulting in insufficient partial nitrification and ANAMMOX, and reduced the TNRE (Fig. 2). Then the microorganisms in the reactor gradually adapted to the higher C/N ratio, and the average TNRE reached 83.65%. Also, Vishnyakova found that a secondary wastewater treatment reactor was beneficial to total nitrogen removal and the activity maintenance of ANAMMOX bacteria when the C/N ratio was greater than 5.46 The abundance of ANAMMOX bacteria cannot be maintained for a long time in a single-stage nitrogen removal process with a high C/N ratio because high concentration of COD can inhibit the ANAMMOX bacteria activity.47 Therefore, the C/N ratio can be regulated to 3–4 to make specific PN/A applications achieve a higher sewage treatment efficiency.
3.2 ΔpH effect
According to the biochemical reactions (eqn (4) and (5)) of AOB and ANAMMOX bacteria, it can be seen that the acid production in the partial nitrification process is greater than the acid consumption in the ANAMMOX process.48,49 This may be the main reason why the effluent pH was lower than the influent pH in the PN/A process (Fig. 3). | NH4+ + 1.5O2 → NO2− + 2H+ + H2O | (4) |
| NH4+ + 1.32NO2− + 0.066HCO3− + 0.13H+ → 1.02N2 + 0.26NO3− + 0.066CH2O0.5N0.15 + 2.03H2O | (5) |
| 0.625CH3COOH + NO3− → 0.5N2 + 1.25CO2 + 0.75H2O + OH− | (6) |
DB utilize organics resulting in varying alkalinities. Reducing 1 mole of nitrate to dinitrogen produces 1 mole of hydroxide eqn (6). The alkali production process was expectedly weaker than the acid production process because there was just a little COD in the reactor. In addition, the three biochemical reactions made the effluent pH different. Therefore, ΔpH can reflect the performance of the PN/A process. When the influent pH remains steady and the C/N ratio increases, the pH (4) is basically unchanged, the process (5) should be slightly lower and the denitrification process (6) should be enhanced.49 The effluent pH should theoretically rise. So, ΔpH should decrease when the C/N ratio increases, and it should be negatively correlated with the C/N ratio in a PN/A MABR.
However, the shift of ΔpH did not adhere strictly to the above analysis. An important reason was that DO also had a profound impact on ΔpH.50 When the DO in the reactor increased, the process (4) was further enhanced, and acid production also increased. The process (5) activity weakened, and the acid consumption was reduced. Meanwhile, the denitrification (6) rate was reduced, and the alkali was also reduced. So, when the DO went up, the pH of the effluent wastewater decreased, resulting in a bigger ΔpH. In Fig. 3, for example, the DO increased from 0.52 to 0.6 mg L−1, and the ΔpH increased from 0.33 to 0.51 on days 72 to 76. It can also be seen from Fig. 3 that the trendline of DO was positively correlated with the change of ΔpH before the replacement of the air compressor (before the 25th day) and after the C/N ratio was adjusted to 3–4 (71–105 days).
3.3 Microbial population structure analysis
In this study, 16S rRNA sequencing identification (on days 1, 15, and 45) and metagenomic identification (on the 95th day) were used to analyze the microbial structure and microbial metabolic pathways.
In the initial biofilm, Ballusus (17.03%), Ferruginibacter (13.3%), and Exiguobacterium (7.44%) were identified, ranking them as the top three. Zhao et al. also found Bacillus (heterotrophic nitrifying and aerobic denitrifying bacteria, HNADB) in an ANAMMOX MABR.14 HNADB can utilize organic carbon for denitrification in an aerobic environment. However, when the reactor was operated to day 15 at a C/N ratio of less than 1.5, Bacillus was not detected again. Ferruginibacter is related to Fe-transformation and was also found in low C/N wastewater.51 NO2−–N gradually accumulated from day 1 to day 15, forming a partial nitrification process. Nitrosomonas played a key role in converting NH4+–N into NO2−–N in the MABR. The relative abundance of Nitrosomonas was 0.8–0.92%, while the relative abundance of Nitrospira (NOB) decreased from 0.53% to 0.32% from day 1 to day 15 (Fig. 4). Moreover, the relative abundances of Denitratisoma and Thauera on day 15 were significantly greater than those in the initial biofilm (Fig. 4). These showed that both partial nitrification and denitrification were enhanced.52,53
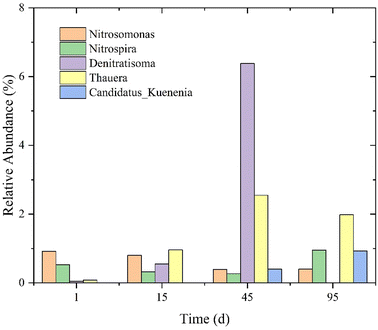 |
| Fig. 4 Shifts in the relative abundances of major microorganisms associated with nitrogen removal. | |
By increasing the C/N ratio to 3–4 in the reactor, the TNRE was increased from 59.02% to 83.66%, and the microbial structure in the reactor was greatly changed. The relative abundance of Flavobacterium ranked first. Meanwhile, the relative abundances of Hydrogenophaga and Acidovorax jumped to 3.36% and 2.82%, respectively. Thauera also entered the top 10 in terms of relative abundance (1.98%) due to the increase in organic matter.54 However, Denitratisoma was not detected on the 95th day. The relative abundances of Nitrospira and Nitrosomonas were still low, being 0.95% and 0.4%, respectively. Candidatus Kuenenia, as ANAMMOX bacteria, can directly convert NH4+–N and NO2−–N into N2, and their relative abundance increased from 0.4% to 0.93%. Candidatus Brocadia were also detected and their relative abundance increased from 0 to 0.65% on the 95th day. Comparing the number of microbial genera, the fourth identification showed that the number of microbial genera (relative abundance >0.01%) on the 95th day was 4–5 times as many as in the first three tests. This may be related to the C/N ratio at different stages.43 The fewer microbial species made the biofilm structure simpler, which was also the reason why the nitrogen removal performance was easily affected by minor condition changes, such as DO, under a low C/N ratio in the MABR (Fig. 3). In this study, after more than 100 days of operation of the PN/A reactor, Nitrosomonas, Thauera, and Candidatus Kuenenia still existed (Fig. 4), which also confirmed that ANAMMOX bacteria can be effectively coupled with AOB and DB.54 In other words, ANAMMOX can effectively be coupled with partial nitrification and denitrification processes at a low C/N ratio in a MABR.
3.4 Kegg pathways
The Kyoto Encyclopedia of Genes and Genomes (Kegg) is a comprehensive database of collected genomic data, metabolic pathways, and compound information about organisms.55 There were 22 Kegg pathways in level 2 and 4 Kegg pathways in level 1 in the PN/A MABR (Fig. 5). “Global and overview maps” was 16.29%. “Amino acid metabolism”, “Carbohydrate metabolism” and “Energy metabolism” belong to “Metabolism” (Kegg pathway level 1), and their relative abundances were 5.56%, 5.08%, and 3.26%, respectively (S1). KEGG pathway level 3 showed that the relative abundances of “nitrogen metabolism (KO00910)” and “carbon metabolism (KO01200)” were 0.61% and 3.19%, respectively (S2). This further demonstrated that long-term nitrogen removal can be achieved in a MABR at low C/N ratios. In a MABR, due to the different oxygen utilization efficiencies of different microorganisms, there is often a decreasing concentration gradient from the substratum to the biofilm surface.54 Different microorganisms grow on the outer surface of membrane filaments according to the oxygen concentration gradient, occupying their respective ecological niches and forming biofilms. In addition, they can obtain energy through metabolizing the substrates, including nitrogen, to meet their survival and proliferation needs.38,56
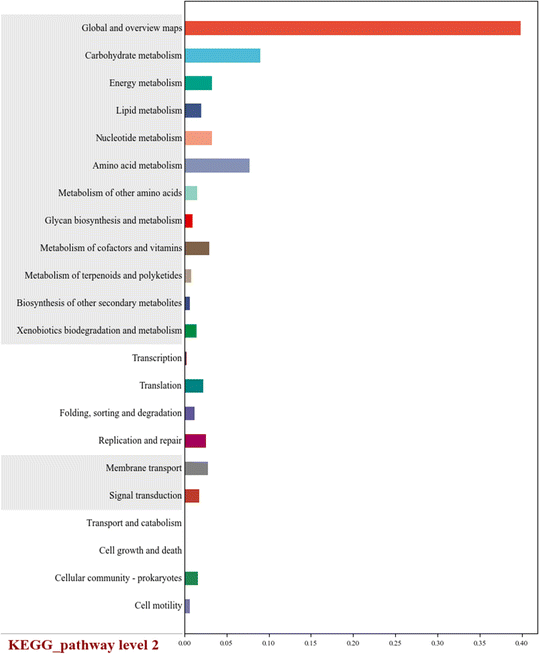 |
| Fig. 5 Kegg metabolic pathway related functional genes at level 2. | |
4. Conclusions
Low lumen pressure (1–5 kPa) was suitable for low C/N ratio (<1.5) sewage treatment to accumulate nitrite (NAR 85.91%) in a MABR, and a PN/A MABR can be achieved by inoculating ANAMMOX bacteria. The total nitrogen removal efficiency can exceed 83.65%, which was seriously affected by DO, especially in the case of a low C/N ratio. With DO less than 0.55 mg L−1, key functional microorganisms can play a better role in nitrogen removal. The ΔpH of the PN/A MABR can be affected by the C/N ratio and DO. Moreover, the ΔpH was negatively correlated with the C/N ratio and positively correlated with DO.
The C/N ratio significantly affected the complexity of the microbial population structure. When the C/N ratio was 3–4, the number of microbial genera in the reactor was 4–5 times as high as when the C/N ratio was <1.5. Heterotrophic denitrifiers (Thauera) can survive at least 16 days when the PN/A MABR was not fed any organic carbon. Also, it was critical for Nitrosomonas, Nitrospira, Denitratisoma, Thauera, Candidatus Kuenenia and Candidatus Brocadia to remove nitrogen synergistically in a PN/A MABR with low cost and little carbon.
Author contributions
Ming Li: investigation, analysis, writing – original draft; Baoan Li: writing – review & editing; Yi Li: writing – review & editing; Zhiye Sun, Jun Liu, Ting Li, Meichao Lan, and Peng Zhang: conceptualization, methodology, validation, supervision, project administration, funding acquisition, project administration.
Conflicts of interest
The authors declare no competing financial interest.
Acknowledgements
This study was funded by Gansu Membrane Science and Technology Research Institute Co. Ltd. “Research and Demonstration of High-efficiency, Low-Carbon and Low-Cost Municipal Wastewater Treatment and Quality Improvement Technology Based on MABR” (No. 21CX6QA129). The authors are grateful for the research collaboration.
References
- M. Ahmar Siddiqui, B. Kumar Biswal, B. Siriweera, G. Chen and D. Wu, Integrated self-forming dynamic membrane (SFDM) and membrane-aerated biofilm reactor (MABR) system enhanced single-stage autotrophic nitrogen removal, Bioresour. Technol., 2021, 102, 291–300 Search PubMed
.
- H. Tian, Y. Hu, X. Xu, M. Hui, Y. Hu, W. Qi and B. Li, Enhanced wastewater treatment with high o-aminophenol concentration by two-stage MABR and its biodegradation mechanism, Bioresour. Technol., 2019, 289, 121649 CrossRef CAS
.
- X. Quan, K. Huang, M. Li, M. Lan and B. Li, Nitrogen removal performance of municipal reverse osmosis concentrate with low C/N ratio by membrane-aerated biofilm reactor, Front. Environ. Sci. Eng., 2018, 12, 5 CrossRef
.
- V. Acevedo Alonso and S. Lackner, Membrane Aerated Biofilm Reactors - How longitudinal gradients influence nitrogen removal - A conceptual study, Water Res., 2019, 166, 115060 CrossRef CAS PubMed
.
- J. Wang, Q. Liu, X. Li, S. Ma, H. Hu, B. Wu and H. Ren, In-situ monitoring AHL-mediated quorum-sensing regulation of the initial phase of wastewater biofilm formation, Environ. Int., 2020, 135, 105326 CrossRef PubMed
.
- Z. Sun, Y. Li, M. Li, N. Wang, J. Liu, H. Guo and B. Li, Steel pickling rinse wastewater treatment by two-stage MABR system: Reactor performance, extracellular polymeric substances (EPS) and microbial community, Chemosphere, 2022, 299, 134402 CrossRef CAS PubMed
.
- J. Zhang, Y. C. Zhang, X. J. Wang, J. Li, R.-X. Zhou, J. Wei and K. Zhang, Effects of substrate shock on release of AHL signals in ANAMMOX granules and properties of granules, Environ. Sci.: Water Res. Technol., 2019, 5(4), 756–768 RSC
.
- R. Nerenberg, The membrane-biofilm reactor (MBfR) as a counter-diffusional biofilm process, Curr. Opin. Biotechnol., 2016, 38, 131–136 CrossRef CAS PubMed
.
- P. Bunse, L. Orschler, S. Agrawal and S. Lackner, Membrane aerated biofilm reactors for mainstream partial nitritation/anammox: Experiences using real municipal wastewater, Water Res.: X, 2020, 9, 100066 CAS
.
- N. Kangwannarakul, C. Wantawin and P. Noophan, Anammox bacteria with attached-growth media for nitrogen removal in wastewater, Clean Technol. Environ., 2017, 20(1), 219–226 CrossRef
.
- C. Ding, F. O. Enyi and L. Adrian, Anaerobic Ammonium Oxidation (Anammox) with Planktonic Cells in a Redox-Stable Semicontinuous Stirred-Tank Reactor, Environ. Sci. Technol., 2018, 52(10), 5671–5681 CrossRef CAS
.
- M. Jia, C. M. Castro-Barros, M. K. H. Winkler and E. I. P. Volcke, Effect of organic matter on the performance and N2O emission of a granular sludge anammox reactor, Environ. Sci.: Water Res. Technol., 2018, 4(7), 1035–1046 RSC
.
- Y. Cao, M. C. van Loosdrecht and G. T. Daigger, Mainstream partial nitritation-Anammox in municipal wastewater treatment: status, bottlenecks, and further studies, Appl. Microbiol. Biotechnol., 2017, 101(4), 1365–1383 CrossRef CAS
.
- B. Zhao, X. Ma, F. Xie, Y. Cui, X. Zhang and X. Yue, Development of simultaneous nitrification-denitrification and anammox and in-situ analysis of microbial structure in a novel plug-flow membrane-aerated sludge blanket, Sci. Total Environ., 2021, 750, 142296 CrossRef CAS PubMed
.
- J. Lu, Y. Hong, Y. Wei, J. D. Gu, J. Wu, Y. Wang and J. G. Lin, Nitrification mainly driven by ammonia-oxidizing bacteria and nitrite-oxidizing bacteria in an Anammox-inoculated wastewater treatment system, AMB Express, 2021, 11(1), 158 CrossRef CAS PubMed
.
- M. Adams, J. Xie, J. Xie, Y. Chang, M. Guo, C. Chen and T. C. Zhang, The effect of carrier addition on Anammox start-up and microbial community: a review, Rev. Environ. Sci. Bio/Technol., 2020, 19(2), 355–368 CrossRef CAS
.
- J. Gu, Q. Yang and Y. Liu, Mainstream Anammox in a novel A-2B process for energy-efficient municipal wastewater treatment with minimized sludge production, Water Res., 2018, 138, 1–6 CrossRef CAS PubMed
.
- F. Fang, B. J. Ni, X. Y. Li, G. P. Sheng and H. Q. Yu, Kinetic analysis on the two-step processes of AOB and NOB in aerobic nitrifying granules, Appl. Microbiol. Biotechnol., 2009, 83(6), 1159–1169 CrossRef CAS
.
- M. Soliman and A. Eldyasti, Ammonia-Oxidizing Bacteria (AOB): opportunities and applications-a review, Rev. Environ. Sci. Bio/Technol., 2018, 17(2), 285–321 CrossRef CAS
.
- M. Zhao, F. Wang, Y. Fan, A. Raheem and H. Zhou, Low-temperature alkaline pyrolysis of sewage sludge for enhanced H2 production with in-situ carbon capture, Int. J. Hydrogen Energy, 2019, 44(16), 8020–8027 CrossRef CAS
.
- I. B. S. Poblete, O. Q. F. Araujo and J. L. de Medeiros, Sewage-water treatment with bio-energy production and carbon capture and storage, Chemosphere, 2022, 286, 131763 CrossRef CAS
.
- P. Roots, A. F. Rosenthal, Q. Yuan, Y. Wang, F. Yang, J. A. Kozak and G. F. Wells, Optimization of the carbon to nitrogen ratio for mainstream deammonification and the resulting shift in nitrification from biofilm to suspension, Environ. Sci.: Water Res. Technol., 2020, 6(12), 3415–3427 RSC
.
- T. Kindaichi, T. Awata, Y. Mugimoto, R. Rathnayake, S. Kasahara and H. Satoh, Effects of organic matter in livestock manure digester liquid on microbial community structure and in situ activity of Anammox granules, Chemosphere, 2016, 159, 300–307 CrossRef CAS
.
- Y. Guo, Z. Luo, J. Shen and Y.-Y. Li, The main Anammox-based processes, the involved microbes and the novel process concept from the application perspective, Front. Environ. Sci. Eng., 2022, 16(7), 84 CrossRef CAS
.
- D. Hou, D. Jassby, R. Nerenberg and Z. J. Ren, Hydrophobic Gas Transfer Membranes for Wastewater Treatment and Resource Recovery, Environ. Sci. Technol., 2019, 53(20), 11618–11635 CrossRef CAS
.
- G. Chen, Y. Zhang, X. Wang, F. Chen, L. Lin, Q. Ruan and P. Chiang, Optimizing of operation strategies of the single-stage partial nitrification-Anammox process, J. Cleaner Prod., 2020, 256, 120667 CrossRef CAS
.
- W. Zhang, R. Xiong and G. Wei, Biological flocculation treatment on distillery wastewater and recirculation of wastewater, J. Hazard. Mater., 2009, 172(2–3), 1252–1257 CrossRef CAS PubMed
.
- H. E. Gulsen Akbay, N. Dizge and H. Kumbur, Enhancing biogas production of anaerobic co-digestion of industrial waste and municipal sewage sludge with mechanical, chemical, thermal, and hybrid pretreatment, Bioresour. Technol., 2021, 340, 125688 CrossRef CAS
.
- T. Ritigala, Y. Chen, J. Zheng, H. Demissie, L. Zheng, D. Yu and Y. Wei, Comparison of an integrated short-cut biological nitrogen removal process with magnetic coagulation treating swine wastewater and food waste digestate, Bioresour. Technol., 2021, 329, 124904 CrossRef CAS PubMed
.
- M. Capodici, S. F. Corsino, D. Di Trapani and G. Viviani, Achievement of partial nitrification under different carbon-to-nitrogen ratio and ammonia loading rate for the co-treatment of landfill leachate with municipal wastewater, Biochem. Eng. J., 2019, 149, 107229 CrossRef CAS
.
- M. R. Augusto, P. R. Camiloti and T. S. O. Souza, Fast start-up of the single-stage nitrogen removal using anammox and partial nitritation (SNAP) from conventional activated sludge in a membrane-aerated biofilm reactor, Bioresour. Technol., 2018, 266, 151–157 CrossRef CAS
.
- M. Lan, M. Li, J. Liu, X. Quan, Y. Li and B. Li, Coal chemical reverse osmosis concentrate treatment by membrane-aerated biofilm reactor system, Bioresour. Technol., 2018, 270, 120–128 CrossRef CAS
.
- Z. Cui, J. Wang, H. Zhang, H. H. Ngo, H. Jia, W. Guo, F. Gao, G. Yang and D. Kang, Investigation of backwashing effectiveness in membrane bioreactor (MBR) based on different membrane fouling stages, Bioresour. Technol., 2018, 269, 355–362 CrossRef CAS PubMed
.
- P. Han, U. Klumper, A. Wong, M. Li, J. G. Lin, Z. Quan and J. D. Gu, Assessment of molecular detection of anaerobic ammonium-oxidizing (Anammox) bacteria in different environmental samples using PCR primers based on 16S rRNA and functional genes, Appl. Microbiol. Biotechnol., 2017, 101(20), 7689–7702 CrossRef CAS
.
- S. Ciesielski, K. Czerwionka, D. Sobotka, T. Dulski and J. Makinia, The metagenomic approach to characterization of the microbial community shift during the long-term cultivation of Anammox-enriched granular sludge, J. Appl. Genet., 2018, 59(1), 109–117 CrossRef CAS PubMed
.
- Z. Fan, W. Zeng, Q. Meng, H. Liu, C. Ma and Y. Peng, Achieving partial nitrification, enhanced biological phosphorus removal and in-situ fermentation (PNPRF) in continuous-flow system and mechanism analysis at transcriptional level, Chem. Eng. J., 2022, 428, 131098 CrossRef CAS
.
- R. Chen, S. Cao, L. Zhang and Y. Zhou, NOB suppression strategies in a mainstream membrane aerated biofilm reactor under exceptionally low lumen pressure, Chemosphere, 2022, 290, 133386 CrossRef CAS PubMed
.
- R. Wang, F. Xiao, Y. Wang and Z. Lewandowski, Determining the optimal transmembrane gas pressure for nitrification in membrane-aerated biofilm reactors based on oxygen profile analysis, Appl. Microbiol. Biotechnol., 2016, 100(17), 7699–7711 CrossRef CAS PubMed
.
- P. Regmi, M. W. Miller, B. Holgate, R. Bunce, H. Park, K. Chandran and C. B. Bott, Control of aeration, aerobic SRT and COD input for mainstream nitritation/denitritation, Water Res., 2014, 57, 162–171 CrossRef CAS
.
- J. Wu, Y. Zhang, M. Zhang and Y. Li, Effect of nitrifiers enrichment and diffusion on their oxygen half-saturation value measurements, Biochem. Eng. J., 2017, 123, 110–116 CrossRef CAS
.
- M. Piculell, C. Suarez, C. Li, M. Christensson, F. Persson, M. Wagner and T. Welander, The inhibitory effects of reject water on nitrifying populations grown at different biofilm thickness, Water Res., 2016, 104, 292–302 CrossRef CAS PubMed
.
- C. Sanchez-Huerta, L. Fortunato, T. Leiknes and P. Y. Hong, Influence of biofilm thickness on the removal of thirteen different organic micropollutants via a Membrane Aerated Biofilm Reactor (MABR), J. Hazard. Mater., 2022, 432, 128698 CrossRef CAS PubMed
.
- B. Du, Y. Gu, G. Chen, G. Wang and L. Liu, Flagellar motility mediates early-stage biofilm formation in oligotrophic aquatic environment, Ecotoxicol. Environ. Saf., 2020, 194, 110340 CrossRef CAS
.
- S. Qiu, J. Liu and L. Zhang, Sludge fermentation liquid addition attained advanced nitrogen removal in low C/N ratio municipal wastewater through short-cut nitrification-denitrification and partial Anammox, Front. Environ. Sci. Eng., 2021, 15, 26 CrossRef CAS
.
- X. Xu, B. Ma, W. Lu, D. Feng, Y. Wei, C. Ge and Y. Peng, Effective nitrogen removal in a granule-based partial-denitrification/Anammox reactor treating low C/N sewage, Bioresour. Technol., 2019, 297, 122467 CrossRef
.
- A. V. Vishnyakova, Y. V. Litti and E. A. Botchkova, Changes in Relative Abundance of Microbial Groups Involved in Nitrogen Removal in the Anammox–Partial Nitrification Reactor System at Increase in Ammonium Nitrogen and COD Load, Microbiology, 2020, 89, 205–211 CAS
.
- A. Nejidat, D. Diaz-Reck, N. Massalha, A. Arbiv, A. Dawas, C. Dosoretz and I. Sabbah, Abundance and diversity of Anammox bacteria in a mainstream municipal wastewater treatment plant, Appl. Microbiol. Biotechnol., 2018, 102(15), 6713–6723 CrossRef CAS PubMed
.
- G. Ciudad, R. Gonzalez, C. Bornhardt and C. Antileo, Modes of operation and pH control as enhancement factors for partial nitrification with oxygen transport limitation, Water Res., 2007, 41(20), 4621–4629 CrossRef CAS PubMed
.
- C. Karasuta, X. Wang, X. Zheng, Y. Chen and Z. Chen, Effect of hydraulic retention time on effluent pH in Anammox bioreactors: Characteristics of effluent pH and pH as an indicator of reactor performance, J. Environ. Manage., 2021, 280, 111716 CrossRef CAS PubMed
.
- J. Li, W. Zhu, H. Dong and D. Wang, Performance and kinetics of ANAMMOX granular sludge with pH shock in a sequencing batch reactor, Biodegradation, 2017, 28(4), 245–259 CrossRef CAS PubMed
.
- Y. Shi, T. Liu, H. Yu and X. Quan, Enhancing anoxic denitrification of low C/N ratio wastewater with novel ZVI composite carriers, J. Environ. Sci., 2022, 112, 180–191 CrossRef CAS PubMed
.
- X. Li, M. Y. Lu, Y. Huang, Y. Yuan and Y. Yuan, Influence of seasonal temperature change on autotrophic nitrogen removal for mature landfill leachate treatment with high-ammonia by partial nitrification-Anammox process, J. Environ. Sci., 2021, 102, 291–300 CrossRef CAS
.
- Q. Li, A. Abdelfattah, Y.-Y. Yu, M. I. Hossain and L. Cheng, Assessment of the start-up of the passive aeration simultaneous nitrification and denitrification (PASND) process using activated sludge as a sole seed for low-energy C and N removal, Environ. Sci.: Water Res. Technol., 2022, 8, 1731–1740 CAS
.
- Y. Liu, T. Zhu, S. Ren, T. Zhao, H. Chai, Y. Xu and Y. Liu, Contribution of nitrification and denitrification to nitrous oxide turnovers in membrane-aerated biofilm reactors (MABR): A model-based evaluation, Sci. Total Environ., 2022, 806(3), 151321 CrossRef CAS
.
- M. Kanehisa, S. Goto and S. Kawashima,
et al., The kegg resource for deciphering the genome, Nucleic Acids Res., 2014, 32, 277–280 CrossRef
.
- C. Pellicer-Nacher and B. F. Smets, Structure, composition, and strength of nitrifying membrane-aerated biofilms, Water Res., 2014, 57, 151–161 CrossRef CAS PubMed
.
|
This journal is © The Royal Society of Chemistry 2023 |
Click here to see how this site uses Cookies. View our privacy policy here.