DOI:
10.1039/D2CE01291J
(Paper)
CrystEngComm, 2023,
25, 114-121
Structure and assembly mechanism of a centipede-shaped high-nuclear Dy14Cu12 heterometallic nanocluster†
Received
18th September 2022
, Accepted 14th November 2022
First published on 14th November 2022
Abstract
The assembly studies of high-nuclear clusters have received much interest in recent years due to their targeted synthesis for some special usages. A Schiff base ligand 2-[N-(2-hydroxynaphthylidene)amino]-2-(hydroxymethyl)propane-1,3-diol (H4L) was thus prepared in this work and was allowed to react with [Cu2(OAc)4(H2O)2] and Dy(NO)3·6H2O, giving a high-nuclear 3d–4f heterometallic Cu12Dy14 nanocluster (1) with the formula [(C2H5)3NH]4[Cu12Dy14O(OH)16(H2L)8(HL)4(HL′)4(OAc)10](NO3)4(OAc)2·6CH3CN·5EtOH·1.5H2O (H3L′ = 1,1,1-tris-(hydroxymethyl)methanamine). It features a centipede-shaped structure constructed from eight Dy3Cu cubanes sharing the Dy(III) vertices. Its assembly mechanism was explored through time-dependent high-resolution electrospray ionization mass spectrometry (HRESI-MS). This revealed that the hierarchical assembly of 1 undergoes two possible routes: H4L → DyCuL → Dy2CuL → Dy3Cu2L2 → Dy4Cu2L2 → Dy4Cu3L3 → Dy5Cu4L4→ Dy14Cu12L12L′4 and H4L → DyCuL → DyCu2L → Dy2Cu2L2 → Dy2Cu3L4 → Dy3Cu4L4 → Dy5Cu4L4 → Dy14Cu12L12L′4. These results not only provide solid evidence for understanding the assembly mechanism of high-nuclear 3d–4f clusters, but also help to give some hints for designing and preparing new high-nuclear clusters.
Introduction
Lanthanide-containing high-nuclear clusters are attracting increasing interest due to their aesthetic nano-scaled structures and their potential applications in fields such as catalysis, information storage, optical devices, and bioactivity.1–7 However, reasonable design and assembly of these clusters are still a huge challenge due to diverse coordination configurations of lanthanide ions and their variable self-assembly pathways. The common method is to assemble metal ions into the anticipated structures using well-designed organic ligands with a special geometry and size and multiple coordination groups which contribute a significant role in the construction of high-nuclear heterometallic clusters.8–10 In the past decades, some well-designed hydroxyl- or carboxylato-containing ligands were employed to build high-nuclear 4f and 3d–4f clusters, such as Gd104,11 Ln60,12 Dy72,13 La20Ni30,14 Gd40Ni44,15 Ni36Gd102,16 Cu24Ln6,17 Cu9Dy2,18 Gd30Co12,19 Cu36Ln24,20 Mn8Ln8,21 Ln24Zn4,22 Fe10Ln10,23 and Fe16Ln4.24 Most of them are homometallic lanthanide clusters, and heterometallic Ln–M clusters (M = Co, Ni, Mn). However, high-nuclear Cu(II)–Dy(III) clusters were rarely reported. It is well known that the reported 4f and 3d–4f high-nuclear clusters present versatile aesthetic structures such as cage,25,26 ring,27,28 linear,29–32 prism,33–35 pancake,36–38 dumbbell39–42 and sphere motifs.43–45 However, 3d–4f high-nuclear clusters with sheet-like skeletons are difficult to form probably due to the limitations from stability. We thus take this challenge to develop high-nuclear Cu(II)–Dy(III) clusters with sheet-like skeletons.
In synthesizing high-nuclear clusters, one usually pays attention to the final products, but ignores their assembling processes and mechanisms. Now, more and more high-nuclear clusters were prepared and applied in a wide field, which requires their precise synthesis under control.46–49 As we all know, the assembling process of high-nuclear clusters is extremely complicated with the assembling mechanism difficult to be clearly detected through routine techniques such as NMR, IR and single crystal X-ray diffraction analysis. Lately, ESI-MS technology has been proved to be an effective method in exploring the assembling mechanism through detecting the intermediates that emerged in the formation process of clusters. However, related studies on the assembly mechanisms of 3d–4f heterometallic complexes are still very limited.47,50–53 Thus, we aimed to investigate the assembling mechanism of the targeted clusters through detecting the intermediates in their formation processes using high resolution ESI-MS.
One of the important tasks for the construction of high-nuclear clusters is the selection of linking atoms or groups which are responsible for gathering metal ions together to achieve a high-nuclear core, as well as for tuning the intermetallic interactions.27,54,55 In this sense, the hydroxyl group is a nice selection, which has strong bridging ability for both lanthanide and transition metal ions and can tune intermetallic interactions through different bridging modes and M–O–M bridging angles. Besides, it is necessary to tune the type and strength of the ligand field around metal ions using appropriate coordination atoms, as well as to control the dimensionality of the clusters through the use of some non-coordinating groups. Taking an overall consideration of these factors, we aimed to design polyhydroxyl multidentate bridging ligands containing also N atoms, which can encapsulate both lanthanide and transition metal ions in their multiple coordination pockets. Thus we designed the ligand 2-[N-(2-hydroxynaphthylidene)amino]-2-(hydroxymethyl)propane-1,3-diol (H4L) to achieve the targeted clusters.
In this study, we successfully obtained a centipede-shaped Dy14Cu12 cluster from the reaction of H4L with Co(OAc)2·4H2O and Dy(NO)3·6H2O. Its possible formation mechanism was investigated using a time-dependent HRESI-MS technique. As far as we know, it is the first time to track the formation process of a high-nuclear heterometallic Cu(II)–Dy(III) cluster with a proposed assembly mechanism.
Experimental
Starting materials
All reagents (analytical grade) in this work were used directly without purification. 2-[N-(2-hydroxynaphthylidene)amino]-2-(hydroxymethyl)propane-1,3-diol was prepared by following the literature method.56 Details for all materials and characterization such as ESI-MS measurements and single-crystal X-ray crystallography are shown in the ESI.† The synthetic route of cluster 1 is shown in Scheme 1.
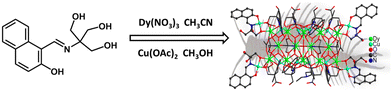 |
| Scheme 1 Schematic diagram for the synthesis of cluster 1. | |
Synthesis of the cluster
Cluster 1 was obtained from the solvothermal reaction of 2-[N-(2-hydroxynaphthylidene)amino]-2-(hydroxymethyl)propane-1,3-diol (0.1 mmol, 0.0105 g) with Dy(NO3)3·6H2O (0.1 mmol, 0.0456 g) and [Cu2(OAc)4(H2O)2] (0.1 mmol, 0.0398 g) at 80 °C for 3 d in the presence of trimethylamine (40 μL) in an evacuated 20 cm-long sealed Pyrex tube using ethanol (1 mL) and acetonitrile (1 mL) as solvents. Cluster 1 crystallized into green crystals with a yield of 24% [calculated from Dy(III)]. Anal. calcd. for C266H377Cu12Dy14N30O119.5: C, 35.72; N, 4.70; H, 4.25%. Found: C, 35.42; N, 4.45; H, 4.44%. IR (KBr, cm−1; Fig. S1†): 3398(s), 1614(s), 1542(s), 1394(s), 1252(m), 1188(w), 1142(m), 1035(m), 829(m), 739(m), 675(w), 579(m), 451(w).
Results and discussion
Crystal structures of the cluster [(C2H5)3NH]4[Cu12Dy14O(OH)16(H2L)8(HL)4(HL′)4(OAc)10](NO3)4(OAc)2·6CH3CN·5EtOH·1.5H2O
Single-crystal diffraction analysis revealed that the title cluster crystallized in a triclinic crystal system with a space group of P
(Table S1†), which consists of fourteen DyIII ions, twelve CuII ions, one O2−, sixteen OH−, eight (H2L)2−, four (HL)3−, four (HL′)2− and eight OAc− in the coordination unit of 1 (Fig. 1a). The detailed crystallographic parameters, bond length and bond angles for the cluster are given in Tables S8 and S9 in the ESI,† respectively. All CuII centers exhibit a planar square geometry completed by three O and one N atom from one Schiff base ligand by ignoring weak Cu⋯O bonds. According to the analysis of SHAPE software (Tables S1–S7†),57 the fourteen DyIII ions present six different coordination configurations as shown in Fig. S5.† Dy1 is nine-coordinated in monocapped square antiprism. Dy5 and Dy7 are seven-coordinated in monocapped trigonal prism and pentagonal bipyramid, respectively. The other DyIII ions are all eight-coordinated in biaugmented trigonal prism for Dy2, square antiprism for Dy4, and triangular dodecahedron for Dy3 and Dy6. The Dy–O and Cu–O/N bond distances range from 2.2075(4) to 2.8545(4) Å and 1.885(5) to 1.993(3) Å, respectively, which are consistent with the values reported in the literature.58–60 The angles of O–Dy–O and O–Cu–O/N are in the range of 51.1(17) to 167.9(4)° and 83.9(2) to 175.1(2)°, respectively. As shown in Fig. S6,† the H4L ligand presents two deprotonated forms (H2L)2− and (HL)3− with two different bridging modes μ2-η1:η3 and μ3-η1:η1:η3 for (H2L)2− and one bridging mode μ4-η1:η1:η2:η3 for (HL)3−. As shown in Fig. 1b, fourteen DyIII ions and twelve CuII ions were linked by forty one O atoms to form a hitherto unknown {Dy14Cu12O41} skeleton. Interestingly, the title cationic cluster [Cu12Dy14O(OH)16(H2L)8(HL)4(HL′)4(OAc)8]2+ exhibits a vertex-sharing octa-cubane structure with a length of approximately 3.70 nm, a width of around 1.69 nm, and a thickness of about 2.20 nm (Fig. S4†), which looks like a “centipede” in appearance. To the best of our knowledge, 1 is the first nanocluster with a centipede-like structure although some DyIII–CuII heterometallic nanoclusters CuLn,61 Cu2Ln2,62 Cu4Ln,63 Cu5Ln2,63 Cu9Ln264 and Cu24Ln665 with different kinds of skeletons were reported.
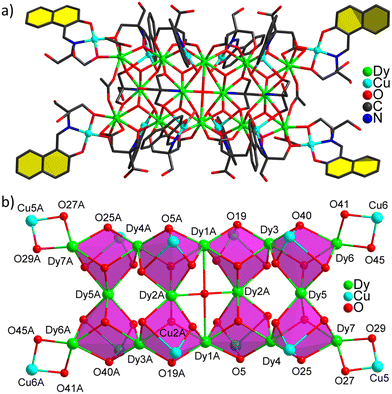 |
| Fig. 1 The molecular structure (a) and skeleton (b) of 1 with selected atoms labeled. Hydrogen atoms are omitted for clarity. | |
Assembly mechanism analysis of the cluster
It is usually difficult to track and explore the self-assembly process of high-nuclear clusters. There are only a few heterometallic complexes reported on the assembly mechanism. In the previous work of our group, the assembly mechanisms of a series of clusters Dy10,66 Dy12,67 Dy2Co868 and Dy4Co8/Dy4Ni869 were clearly studied. Based on these previous research studies, the self-assembly process of the title cluster of Dy14Cu12 was tracked by analyzing the species formed in the reaction at different reaction times through HRESI-MS. The same amount of reaction solution was taken out from parallel experiments at irregular intervals and immediately diluted with chromatographic methanol. Their HRESI-MS spectra were subsequently recorded in anionic and cationic modes and analyzed, which showed us the change of intermediates and their abundance with the reaction time.
As shown in Fig. 2a and S7–S9,† the reaction was monitored for a duration of about 24 h with the HRESI-MS data collected in positive mode at different times. A series of positively charged ion peaks are concentrated in the m/z range of 200–3000, from which ten intermediates (Table S10†) were detected with the maximum m/z value at 2624.00 for Dy5Cu4L4. This revealed that the species in the reaction solution underwent a change from low-nuclear to high-nuclear with the reaction time. The time-dependent change trend of the peak intensity of each intermediate is plotted in Fig. 2b. It showed that the species at the beginning of the reaction (approximately 5 min) are mainly inorganic metal salts (labelled Dy) and organic ligands. Subsequently, the species named DyL′, DyCuL and Dy2CuL (Table S11†) were detected as the kinetic products which increased with the reaction time and reached their maximum at about 45 min for DyL′ and DyCuL and 90 min for Dy2CuL. When the reaction was carried out for 60 minutes, DyCu2L appeared and reached a maximum at about 240 min with a subsequent fast decrease. At a reaction time of 90 min, a new species Dy3Cu2L2 was observed and reached its maximum at about 450 min. The species Dy2Cu2L2 and Dy4Cu2L2 appeared at 180 min and increased with the reaction time to their maxima at about 360 and 600 min, respectively. As the reaction was progressed further, the species Dy4Cu3L3, Dy3Cu4L4 and Dy2Cu3L4 were obviously detected in sequence at about 240, 240, and 360 min, and reached their maximum at 810, 1080 and 600 min, respectively. The species Dy5Cu4L4 was first detected at 600 min and reached maximum at 1080 min. Based on the information on these species and their peak intensity changes with reaction time, two possible assembly routes can presumably be established: H4L → DyCuL → Dy2CuL → Dy3Cu2L2 → Dy4Cu2L2 → Dy4Cu3L3 → Dy5Cu4L4→ Dy14Cu12L12L′4 and H4L → DyCuL → DyCu2L → Dy2Cu2L2 → Dy2Cu3L4 → Dy3Cu4L4 → Dy5Cu4L4 → Dy14Cu12L12L′4 (Fig. 3). There are a few reports on the assembly mechanisms of 3d–4f heterometallic nanoclusters such as Eu24Ti8,70 NiII2DyIII2,71 DyIIIxCoII10−x (x = 2, 4),68 DyIII4MII8 (M = Ni, Co)69 and [DyIII10MnIII4MnII2].72 However, there are no related studies reported on the assembly mechanism of DyIII–CuII heterometallic nanoclusters. Thus, this is the first case clarifying the assembly mechanism of DyIII–CuII heterometallic nanoclusters through HRESI-MS.
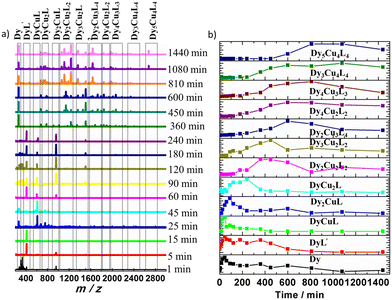 |
| Fig. 2 (a) Time-dependent HRESI-MS for the reaction solution used for tracking the assembly process of 1; (b) HRESI-MS spectral intensity versus time profiles of intermediates during self-assembly of 1. | |
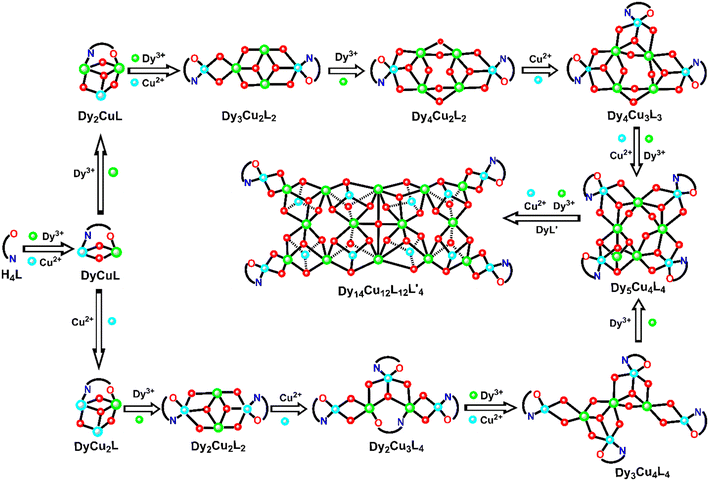 |
| Fig. 3 Possible assembly mechanism for 1. | |
Magnetic properties
A polycrystalline sample of 1 was collected and its direct current magnetic susceptibilities were measured from 2 to 300 K under an external field of 1000 Oe as plotted in Fig. 4a. The χMT value of 1 at room temperature is 201.71 cm3 K mol−1, close to the corresponding theoretical values for fourteen independent DyIII centers (198.38 cm3 K mol−1; 6H15/2, S = 5/2, L = 5, J = 15/2, and g = 4/3)43,73,74 and twelve independent CuII centers (4.50 cm3 K mol−1; S = 1/2, g = 2 and C = 0.375 cm3 K mol−1).53,75 As the temperature is lowered, the χMT value of 1 decreases slowly to a value of 187.72 cm3 K mol−1 at 50 K. The further decreasing temperature of the sample led to an abrupt decrease of χMT of 1, reaching 126.22 cm3 K mol−1 at 2 K. The χMT versus T profile of 1 might be caused by the depopulation of Stark levels and possible antiferromagnetic interactions.
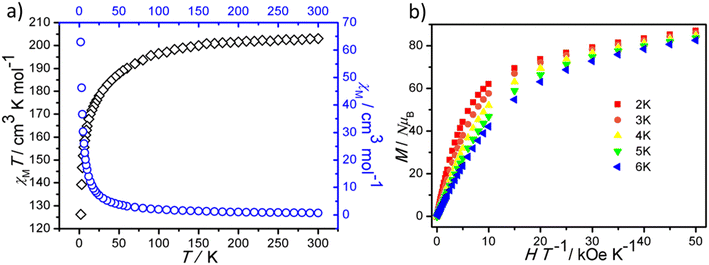 |
| Fig. 4 Plots of χM and χMT versus T (a), M vs. H T−1 (b) for the cluster. | |
The field-dependent magnetization of 1 was measured in the temperature range of 2.0–6.0 K and its M vs. HT−1 curves (Fig. 4b) and M vs. H curves are shown in Fig. S10.† The magnetization of 1 has a sharp increase in the low field range with a subsequent much slower increase in the high field range, reaching a value of 27.78 NμB at 70 kOe and 1.8 K.51,76 The M vs. H T−1 isotherms collected at different temperatures are not superimposed, which revealed possible anisotropy and/or low energy states for 1. The magnetic hysteresis loops at 2 K for 1 were not apparent, which is shown in Fig. S11.†
In order to investigate the presence of slow magnetic relaxation behaviors in 1, the variable-frequency alternating current (ac) susceptibilities were obtained at a temperature range of 2–5 K and an applied dc field of 0 Oe with an oscillating ac field of 2 Oe, as shown in Fig. S12.† This revealed no obvious temperature- and frequency-dependence of its ac susceptibilities (χ′ and χ′′) with the absence of peaks in χ′ and χ′′ vs. v plots and a nearly zero value of χ′′ in 1, as shown in Fig. S13.† Even when a series of different dc fields were applied for the ac susceptibility measurements of 1 at 2 K (Fig. S14†), no frequency-dependence was found in its χ′ and χ′′ vs. v plots with a nearly zero χ′′ value in 1.
Conclusions
In summary, we prepared a heterometallic coordination cluster [(C2H5)3NH]4[Cu12Dy14O(OH)16(H2L)8(HL)4(HL′)4(OAc)10](NO3)4(OAc)2·6CH3CN·5EtOH·1.5H2O (1) bearing the Schiff base ligand 2-[N-(2-hydroxynaphthylidene)amino]-2-(hydroxymethyl)propane-1,3-diol (H4L), which presents a centipede-shaped structure bearing eight Dy3Cu cubanes sharing the Dy(III) vertices. The time-dependent HRESI-MS analysis revealed a possible formation mechanism with two routes H4L → DyCuL → Dy2CuL → Dy3Cu2L2 → Dy4Cu2L2 → Dy4Cu3L3 → Dy5Cu4L4 → Dy14Cu12L12L′4 and H4L → DyCuL → DyCu2L → Dy2Cu2L2 → Dy2Cu3L4 → Dy3Cu4L4 → Dy5Cu4L4 → Dy14Cu12L12L′4. As far as we know, this is one of the rare examples of exploring the formation mechanism of heterometallic clusters, and it is the first study of the assembly mechanism of Dy–Cu clusters. This will provide an inspiration for designing and synthesizing high-nuclear clusters.
Author contributions
The manuscript was written through contributions of all the authors. All the authors have given approval to the final version of the manuscript.
Conflicts of interest
There are no conflicts of interest to declare.
Acknowledgements
This work was supported by the National Natural Science Foundation of China (grant no. 12064002, 22061004 and 21901050), the Guangxi Natural Science Foundation of China (grant no. 2018GXNSFBA050031, 2020GXNSFAA159132, 2018GXNSFDA281002 and AD20238043), the Guangxi Technology Base and Talent Subject (grant no. GUIKE AD19245002), and the Key Project of Guangxi Normal University (grant no. 2018ZD003).
References
- W. G. Zhu, L. Y. Zhu, L. J. Sun, Y. G. Zhen, H. L. Dong, Z. X. Wei and W. P. Hu, Uncovering the Intramolecular Emission and Tuning the Nonlinear Optical Properties of Organic Materials by Cocrystallization, Angew. Chem., Int. Ed., 2016, 55, 14023–14027 CrossRef CAS PubMed.
- T. K. Ghosh, S. Maity, J. Mayans and A. Ghosh, Family of Isomeric Cu(II)-Ln(III) (Ln = Gd, Tb, and Dy) Complexes Presenting Field-Induced Slow Relaxation of Magnetization Only for the Members Containing Gd(III), Inorg. Chem., 2021, 60, 438–448 CrossRef CAS.
- J. P. Karns, S. V. Eliseeva, C. L. Ward, M. J. Allen, S. Petoud and J. C. Lutter, Near-Infrared Lanthanide-Based Emission from Fused Bis[Ln(III)/Zn(II)-14-metallacrown-5] Coordination Compounds, Inorg. Chem., 2022, 61, 5691–5695 CrossRef CAS.
- I. Koehne, A. Lik, M. Gerstel, C. Bruhn, J. P. Reithmaier, M. Benyoucef and R. Pietschnig, Functionalised phosphonate ester supported lanthanide (Ln = La, Nd, Dy, Er) complexes, Dalton Trans., 2020, 49, 16683–16692 RSC.
- F. Piccinelli, I. Carrasco, C. G. Ma and M. Bettinelli, Systematic Analysis of the Crystal Chemistry and Eu3+ Spectroscopy along the Series of Double Perovskites Ca2LnSbO6 (Ln = La, Eu, Gd, Lu, and Y), Inorg. Chem., 2021, 60, 8259–8266 CrossRef CAS PubMed.
- M. A. Shmelev, M. A. Kiskin, J. K. Voronina, K. A. Babeshkin, N. N. Efimov, E. A. Varaksina, V. M. Korshunov, I. V. Taydakov, N. V. Gogoleva, A. A. Sidorov and I. L. Eremenko, Molecular and Polymer Ln2M2 (Ln = Eu, Gd, Tb, Dy; M = Zn, Cd) Complexes with pentafluorobenzoate Anions: The Role of Temperature and Stacking Effects in the Structure; Magnetic and Luminescent Properties, Materials, 2020, 13, 5689–5710 CrossRef CAS.
- L. Xi, C. Y. Jin, H. W. Song, X. T. Wang, L. C. Li and J. P. Sutter, Supramolecular heptanuclear Ln-Cu complexes involving nitronyl nitroxide biradicals: structure and magnetic behavior, Dalton Trans., 2022, 51, 6955–6963 RSC.
- H. M. Dong, Z. C. Zhang, H. Y. Li, Z. Y. Liu, E. C. Yang and X. J. Zhao, High-nuclear heterometallic oxime clusters assembled from triangular subunits: solvothermal syntheses, crystal structures and magnetic properties, Dalton Trans., 2018, 47, 169–179 RSC.
- L. D. Lin, R. Ge, J. Zhang, Z. Li, H. Yu, J. H. Liu, X. X. Li, Y. Q. Sun, D. Zhao and S. T. Zheng, Construction of Two High-Nuclear 3d-4d Heterometallic Cluster Organic Frameworks by Introducing a Bifunctional Tripodal Alcohol as a Structure-Directing Agent, Chem. – Asian J., 2019, 14, 1985–1991 CrossRef CAS PubMed.
- J. Zhou, H. Xiao, H. H. Zou and X. Liu, A New Type of Three-Dimensional Hybrid Polymeric Haloplumbate Based on Rare High-Nuclear Heterometallic Clusters, Inorg. Chem., 2018, 57, 12860–12868 CrossRef CAS.
- J. B. Peng, X. J. Kong, Q. C. Zhang, M. Orendac, J. Prokleska, Y. P. Ren, L. S. Long, Z. Zheng and L. S. Zheng, Beauty, symmetry, and magnetocaloric effect-four-shell keplerates with 104 lanthanide atoms, J. Am. Chem. Soc., 2014, 136, 17938–17941 CrossRef CAS PubMed.
- J. Dong, P. Cui, P. F. Shi, P. Cheng and B. Zhao, Ultrastrong Alkali-Resisting Lanthanide-Zeolites Assembled by [Ln60] Nanocages, J. Am. Chem. Soc., 2015, 137, 15988–15991 CrossRef CAS.
- L. Qin, Y. Z. Yu, P. Q. Liao, W. Xue, Z. P. Zheng, X. M. Chen and Y. Z. Zheng, A “Molecular Water Pipe”: A Giant Tubular Cluster {Dy72} Exhibits Fast Proton Transport and Slow Magnetic Relaxation, Adv. Mater., 2016, 28, 10772–10779 CrossRef CAS.
- X. J. Kong, Y. P. Ren, L. S. Long, Z. Zheng, R. B. Huang and L. S. Zheng, A keplerate magnetic cluster featuring an icosidodecahedron of Ni(II) ions encapsulating a dodecahedron of La(III) ions, J. Am. Chem. Soc., 2007, 129, 7016–7017 CrossRef CAS PubMed.
- N. F. Li, Q. F. Lin, X. M. Luo, J. P. Cao and Y. Xu, Cl−-Templated Assembly of Novel Peanut-like Ln40Ni44 Heterometallic Clusters Exhibiting a Large Magnetocaloric Effect, Inorg. Chem., 2019, 58, 10883–10889 CrossRef CAS.
- W. P. Chen, P. Q. Liao, P. B. Jin, L. Zhang, B. K. Ling, S. C. Wang, Y. T. Chan, X. M. Chen and Y. Z. Zheng, The Gigantic {Ni36Gd102} Hexagon: A Sulfate-Templated “Star-of-David” for Photocatalytic CO2 Reduction and Magnetic Cooling, J. Am. Chem. Soc., 2020, 142, 4663–4670 CrossRef CAS.
- G. Xiong, H. Xu, J. Z. Cui, Q. L. Wang and B. Zhao, The multiple core-shell structure in Cu24Ln6 cluster with magnetocaloric effect and slow magnetization relaxation, Dalton Trans., 2014, 43, 5639–5642 RSC.
- I. A. Kuhne, G. E. Kostakis, C. E. Anson and A. K. Powell, An Undecanuclear Ferrimagnetic Cu9Dy2 Single Molecule Magnet Achieved through Ligand Fine-Tuning, Inorg. Chem., 2016, 55, 4072–4074 CrossRef.
- H. J. Lun, L. Xu, X. J. Kong, L. S. Long and L. S. Zheng, A High-Symmetry Double-Shell Gd30Co12 Cluster Exhibiting a Large Magnetocalo ric Effect, Inorg. Chem., 2021, 60, 10079–10083 CrossRef CAS.
- J. D. Leng, J. L. Liu and M. L. Tong, Unique nanoscale {CuII36LnIII24} (Ln = Dy and Gd) metallo-rings, Chem. Commun., 2012, 48, 5286–5288 RSC.
- K. R. Vignesh, S. K. Langley, B. Moubaraki, K. S. Murray and G. Rajaraman, Large Hexadecametallic {Mn(III)-Ln(III)} Wheels: Synthesis, Structural, Magnetic, and Theoretical Characterization, Chem. – Eur. J., 2015, 21, 16364–16369 CrossRef CAS PubMed.
- X. Y. Zheng, S. Q. Wang, W. Tang, G. L. Zhuang, X. J. Kong, Y. P. Ren, L. S. Long and L. S. Zheng, Two nanosized 3d-4f clusters featuring four Ln6 octahedra encapsulating a Zn4 tetrahedron, Chem. Commun., 2015, 51, 10687–10690 RSC.
- A. Baniodeh, Y. Liang, C. E. Anson, N. Magnani, A. K. Powell, A. N. Unterreiner, S. Seyfferle, M. Slota, M. Dressel, L. Bogani and K. Goss, Unraveling the Influence of Lanthanide Ions on Intra- and Inter-Molecular Electronic Processes in Fe10Ln10 Nano-Toruses, Adv. Funct. Mater., 2014, 24, 6280–6290 CrossRef CAS.
- A. Baniodeh, I. J. Hewitt, V. Mereacre, Y. H. Lan, G. Novitchi, C. E. Anson and A. K. Powell, Heterometallic 20-membered {Fe16Ln4} (Ln = Sm, Eu, Gd, Tb, Dy, Ho) metallo-ring aggregates, Dalton Trans., 2011, 40, 4080–4086 RSC.
- Q. Tang, Y. Sun, H. Y. Li, J. Q. Wu, Y. N. Liang and Z. Zhang, Hexanuclear 3d-4f metal-organic cages assembled from a carboxylic acid-functionalized tris-triazamacrocycle for highly selective fluorescent sensing of picric acid, Appl. Organomet. Chem., 2019, 33, 4814–4826 CrossRef.
- G. J. Zhou, W. P. Chen, Y. Z. Yu, L. Qin, T. Han and Y. Z. Zheng, Filling the Missing Links of M3n Prototype 3d-4f and 4f Cyclic Coordination Cages: Syntheses, Structures, and Magnetic Properties of the Ni10Ln5 and the Er3n Wheels, Inorg. Chem., 2017, 56, 12821–12829 CrossRef CAS PubMed.
- I. A. Lutsenko, M. A. Kiskin, K. A. Lysenko, E. M. Zueva, N. N. Efimov, E. A. Ugolkova, Y. V. Maksimov, V. K. Imshennik, M. M. Petrova, A. A. Sidorov and I. L. Eremenko, A new heterometallic pivalate {Fe8Cd} complex as an example of unusual “quot;ferric wheel” molecular self-assembly, Dalton Trans., 2020, 49, 15175–15179 RSC.
- B. B. Yang, L. N. Feng, X. M. Fan, K. X. Zhang, J. H. Yang and B. Liu, Towards a new type of heterometallic system based on a paddle-wheel Ru2 dimer: first results derived from the use of a high spin diruthenium(III) building block, Inorg. Chem. Front., 2017, 4, 1061–1065 RSC.
- N. Dalla-Favera, J. Hamacek, M. Borkovec, D. Jeannerat, G. Ercolani and C. Piguet, Tuneable intramolecular intermetallic interactions as a new tool for programming linear heterometallic 4f-4f complexes, Inorg. Chem., 2007, 46, 9312–9322 CrossRef CAS.
- R. Castaneda and J. L. Brusso, Novel homo and heterometallic linear trinuclear complexes employing N-2-pyrimidylimidoyl-2-pyrimidylamidine ligands, Acta Crystallogr., Sect. A: Found. Adv., 2019, 75, A117 Search PubMed.
- B. Q. Liu, W. H. Liu, C. R. Wang, J. H. Zheng, Y. H. Zhang and J. W. Zhang, Syntheses and properties of two types of linear tetranuclear heterometallic clusters with identical formulas but different structures, Polyhedron, 2021, 194, 114921–114926 CrossRef CAS.
- B. Miroslaw, B. Cristovao and Z. Hnatejko, Heterometallic Zn(II)-Ln(III)-Zn(II) Schiff Base Complexes with Linear or Bent ConformationSynthesis, Crystal Structures, Luminescent and Magnetic Characterization, Molecules, 2018, 23, 1761–1776 CrossRef.
- X. Y. Shen, Y. Y. Zhang, L. Zhang, Y. J. Lin and G. X. Jin, Discrete Rectangles, Prisms, and Heterometallic Cages from a Conjugated Cp*Rh-Based Building Block, Chem. – Eur. J., 2015, 21, 16975–16981 CrossRef CAS PubMed.
- H. T. Pham, C. P. Dang, N. T. Trung and V. T. Ngan, Transformation between Hexagonal Prism and Antiprism of the Singly and Doubly Cr-Doped Ge12 Clusters, J. Phys. Chem. A, 2019, 123, 10721–10729 CrossRef CAS PubMed.
- T. G. Tziotzi, E. K. Andreou, E. Tzanetou, D. A. Kalofolias, D. J. Cutler, M. Weselski, M. Siczek, T. Lis, E. K. Brechin and C. J. Milios, The first amino acid bound manganese-calcium clusters: a {[MnCa]2} methylalanine complex, and a [MnCa] trigonal prism, Dalton Trans., 2020, 49, 10339–10343 RSC.
- H. Bohringer, G. Chon and J. Trumper, The Cosmic Large-Scale Structure in X-rays (CLASSIX) Cluster Survey: II. Unveiling a pancake structure with a 100 Mpc radius in the local Universe, Astron. Astrophys., 2021, 651, 1–7 Search PubMed.
- U. Bong, J. Kim, J. Bang, J. Park, K. J. Han and S. Hahn, 'Defect-irrelevant-winding' no-insulation (RE)Ba2Cu3O7-x pancake coil in conduction-cooling operation, Supercond. Sci. Technol., 2021, 34, 085003 CrossRef.
- M. A. Lemes, N. Mavragani, P. Richardson, Y. X. Zhang, B. Gabidullin, J. L. Brusso, J. O. Moilanen and M. Murugesu, Unprecedented intramolecular pancake bonding in a {Dy2} single-molecule magnet, Inorg. Chem. Front., 2020, 7, 2592–2601 RSC.
- S. P. Ding, Z. Y. Zhang, G. J. Zhou and R. Cao, Synthesis, Structure and Luminescence Properties of Dumbbell-like Silver Clusters, Chin. J. Struct. Chem., 2020, 39, 1824–1834 Search PubMed.
- F. Kuroda, T. Fukazawa and T. Miyake, Ordered and disordered phases in CaCu5-type derived structures: Dumbbell cluster modeling with first-principles calculations, Phys. Rev. Mater., 2021, 5, 124405–124409 CrossRef CAS.
- M. Sato, Clusters formed by dumbbell-like one-patch particles confined in thin systems, Sci. Rep., 2021, 11, 95542–95546 Search PubMed.
- F. Shao, J. J. Zhuang, M. G. Chen, N. Wang, H. Y. Shi, J. P. Tong, G. Luo, J. Tao and L. S. Zheng, Facile and environmentally friendly synthesis of six heterometallic dumbbell-shaped MII5LnIII4 (M = Co, Ni; Ln = Eu, Gd, Dy) clusters as cryogenic magnetic coolants and molecular magnets, Dalton Trans., 2018, 47, 16850–16854 RSC.
- D. M. Brigham, A. S. Ivanov, B. A. Moyer, L. H. Delmau, V. S. Bryantsev and R. J. Ellis, Trefoil-Shaped Outer-Sphere Ion Clusters Mediate Lanthanide(III) Ion Transport with Diglycolamide Ligands, J. Am. Chem. Soc., 2017, 139, 17350–17358 CrossRef CAS PubMed.
- I. L. Rasskazov, N. Spegazzini, P. S. Carney and R. Bhargava, Dielectric Sphere Clusters as a Model to Understand Infrared Spectroscopic Imaging Data Recorded from Complex Samples, Anal. Chem., 2017, 89, 10813–10818 CrossRef CAS PubMed.
- W. Vigilante, O. Lopez and J. Fung, Brownian dynamics simulations of sphere clusters in optical tweezers, Opt. Express, 2020, 28, 36131–36146 CrossRef CAS PubMed.
- R. Ge, X. X. Li and S. T. Zheng, Recent advances in polyoxometalate-templated high-nuclear silver clusters, Coord. Chem. Rev., 2021, 435, 213787 CrossRef CAS.
- L. L. Li, B. Cai, D. H. Pang, X. X. Du, X. L. Yin, H. W. Wang, J. Yang, D. C. Li and J. M. Dou, Construction of high-nuclear 4p-4f heterometallic {Ln11Ge12} cluster-organic frameworks with high-sensitivity luminescence sensing of Fe3+ in aqueous solution, CrystEngComm, 2020, 22, 8343–8352 RSC.
- Y. L. Li, H. L. Wang, Z. H. Zhu, J. Li, H. H. Zou and F. P. Liang, A Series of High-Nuclear Gadolinium Cluster Aggregates with a Magnetocaloric Effect Constructed through Two-Component Manipulation, Inorg. Chem., 2021, 60, 16794–16802 CrossRef CAS.
- M. R. Zhang, B. Liu, H. D. Zhang, C. Zhang, J. P. Wang and J. Y. Niu, Synthesis and Mechanism Studies of a High-Nuclear Mn72W48 Cluster, Inorg. Chem., 2020, 59, 13733–13740 CrossRef CAS.
- U. Warzok, L. K. Mahnke and W. Bensch, Soluble Hetero-Polyoxovanadates and Their Solution Chemistry Analyzed by Electrospray Ionization Mass Spectrometry, Chem. – Eur. J., 2019, 25, 1405–1419 CrossRef CAS PubMed.
- M.-H. Du, X.-Y. Zheng, X.-J. Kong, L.-S. Long and L.-S. Zheng, Synthetic Protocol for Assembling Giant Heterometallic Hydroxide Clusters from Building Blocks: Rational Design and Efficient Synthesis, Matter, 2020, 3, 1334–1349 CrossRef.
- V. Chandrasekhar, S. Das, A. Dey, S. Hossain, S. Kundu and E. Colacio, Linear, Edge-Sharing Heterometallic Trinuclear [CoIILnIIICoII] (Ln(III) = GdIII, DyIII, TbIII, and HoIII) Complexes: Slow Relaxation of Magnetization in the DyIII Derivative, Eur. J. Inorg. Chem., 2014, 2014, 397–406 CrossRef CAS.
- S. Yu, Q. H. Zhang, J. R. Zhu, F. L. Wei, D. C. Liu, H. C. Hu, H. H. Zou, Y. N. Liang, F. P. Liang and Z. L. Chen, Two tetranuclear Cu2Ln2 (Ln = Dy, Tb) heterometallic complexes: Structure, solution behavior, and magnetic properties, Appl. Organomet. Chem., 2022, 36, 6557–6564 Search PubMed.
- C. M. Liu, X. Hao and D. Q. Zhang, CO2-fixation into carbonate anions for the construction of 3d-4f cluster complexes with salen-type Schiff base ligands: from molecular magnetic refrigerants to luminescent single-molecule magnets, Appl. Organomet. Chem., 2020, 34, e5893–e5906 CAS.
- Z. Wang, H. F. Su, C. H. Tung, D. Sun and L. S. Zheng, Deciphering synergetic core-shell transformation from [Mo6O22@Ag44] to [Mo8O28@Ag50], Nat. Commun., 2018, 9, 4407 CrossRef PubMed.
- Z. A. K. Khattak, H. A. Younus, N. Ahmad, H. Ullah, S. Suleman, M. S. Hossain, M. Elkadi and F. Verpoort, Highly active dinuclear cobalt complexes for solvent-free cycloaddition of CO2 to epoxides at ambient pressure, Chem. Commun., 2019, 55, 8274–8277 RSC.
- D. Casanova, M. Llunell, P. Alemany and S. Alvarez, The rich stereochemistry of eight-vertex polyhedra: a continuous shape measures study, Chem. – Eur. J., 2005, 11, 1479–1494 CrossRef CAS.
- V. Baskar, K. Gopal, M. Helliwell, F. Tuna, W. Wernsdorfer and R. E. Winpenny, 3d-4f clusters with large spin ground states and SMM behaviour, Dalton Trans., 2010, 39, 4747–4750 RSC.
- F. J. Kettles, V. A. Milway, F. Tuna, R. Valiente, L. H. Thomas, W. Wernsdorfer, S. T. Ochsenbein and M. Murrie, Exchange interactions at the origin of slow relaxation of the magnetization in {TbCu3} and {DyCu3} single-molecule magnets, Inorg. Chem., 2014, 53, 8970–8978 CrossRef CAS PubMed.
- X. Liu, J. You, R. Wang, Z. Ni, F. Han, L. Jin, Z. Ye, Z. Fang and R. Guo, Synthesis and Absorption Properties of Hollow-spherical Dy2Cu2O5 via a Coordination Compound Method with [DyCu(3,4-pdc)2(OAc)(H2O)2]*10.5H2O Precursor, Sci. Rep., 2017, 7, 13085–13095 CrossRef.
- L. Xi, C. Y. Jin, H. W. Song, X. T. Wang, L. C. Li and J. P. Sutter, Supramolecular heptanuclear Ln-Cu complexes involving nitronyl nitroxide biradicals: structure and magnetic behavior, Dalton Trans., 2022, 51, 6955–6963 RSC.
- S. Maity, A. Mondal, S. Konar and A. Ghosh, The role of 3d-4f exchange interaction in SMM behaviour and magnetic refrigeration of carbonato bridged CuII2LnIII2 (Ln = Dy, Tb and Gd) complexes of an unsymmetrical N2O4 donor ligand, Dalton Trans., 2019, 48, 15170–15183 RSC.
- P. Richardson, D. I. Alexandropoulos, L. Cunha-Silva, G. Lorusso, M. Evangelisti, J. K. Tang and T. C. Stamatatos, 'All three-in-one': ferromagnetic interactions, single-molecule magnetism and magnetocaloric properties in a new family of [Cu4Ln] (Ln(III) = Gd, Tb, Dy) clusters, Inorg. Chem. Front., 2015, 2, 945–948 RSC.
- I. A. Kuhne, G. E. Kostakis, C. E. Anson and A. K. Powell, An Undecanuclear Ferrimagnetic Cu9Dy2 Single Molecule Magnet Achieved through Ligand Fine-Tuning, Inorg. Chem., 2016, 55, 4072–4074 CrossRef PubMed.
- G. Xiong, H. Xu, J. Z. Cui, Q. L. Wang and B. Zhao, The multiple core-shell structure in Cu24Ln6 cluster with magnetocaloric effect and slow magnetization relaxation, Dalton Trans., 2014, 43, 5639–5642 RSC.
- H. L. Wang, X. F. Ma, J. M. Peng, Z. H. Zhu, B. Li, H. H. Zou and F. P. Liang, Tracking the Stepwise Formation of the Dysprosium Cluster (Dy10) with Multiple Relaxation Behavior, Inorg. Chem., 2019, 58, 9169–9174 CrossRef CAS PubMed.
- X. F. Ma, H. L. Wang, Z. H. Zhu, B. Li, K. Q. Mo, H. H. Zou and F. P. Liang, Formation of nanocluster {Dy12} containing Dy-exclusive vertex-sharing [Dy4(μ3-OH)4] cubanes via simultaneous multitemplate guided and step-by-step assembly, Dalton Trans., 2019, 48, 11338–11344 RSC.
- S. Yu, H. L. Wang, Z. L. Chen, H. H. Zou, H. C. Hu, Z. H. Zhu, D. C. Liu, Y. N. Liang and F. P. Liang, Two Decanuclear Dy(III)xCo(II)(10-x)(x=2, 4) Nanoclusters: Structure, Assembly Mechanism, and Magnetic Properties, Inorg. Chem., 2021, 60, 4904–4914 CrossRef CAS PubMed.
- S. Yu, Q. H. Zhang, Z. L. Chen, H. H. Zou, H. C. Hu, D. C. Liu and F. P. Liang, Structure, assembly mechanism and magnetic properties of heterometallic dodecanuclear nanoclusters (DyIII4MII8)-M(M = Ni, Co), Inorg. Chem. Front., 2021, 8, 5214–5224 RSC.
- H. Zheng, M. H. Du, S. C. Lin, Z. C. Tang, X. J. Kong, L. S. Long and L. S. Zheng, Assembly of a Wheel-Like Eu24Ti8 Cluster under the Guidance of High-Resolution Electrospray Ionization Mass Spectrometry, Angew. Chem., Int. Ed., 2018, 57, 10976–10979 CrossRef CAS.
- K. Q. Mo, Z. H. Zhu, H. L. Wang, X. F. Ma, J. M. Peng, H. H. Zou, J. Bai and F. P. Liang, Substituents lead to differences in the formation of two different butterfly-shaped NiII2DyIII2 clusters: structures and multistep assembly mechanisms, Dalton Trans., 2019, 48, 16641–16649 RSC.
- S. Yu, H. C. Hu, H. H. Zou, D. C. Liu, Y. N. Liang, F. P. Liang and Z. L. Chen, Two Heterometallic Nanoclusters [DyIII4NiII8] and [DyIII10MnIII4MnII2]: Structure, Assembly Mechanism, and Magnetic Properties, Inorg. Chem., 2022, 61, 3655–3663 CrossRef CAS PubMed.
- S. K. Bose, K. Geetharani, S. Sahoo, K. H. K. Reddy, B. Varghese, E. D. Jemmis and S. Ghosh, Synthesis, Characterization, and Electronic Structure of New Type of Heterometallic Boride Clusters, Inorg. Chem., 2011, 50, 9414–9422 CrossRef CAS PubMed.
- V. Das, I. Khan, F. Hussain, M. Sadakane, N. Tsunoji, K. Ichihashi, C. Kato, K. Inoue and S. Nishihara, Single-Molecule Magnetic, Catalytic and Photoluminescence Properties of Heterometallic 3d-4f [Ln{PZn2W10O38(H2O)2}2]11− Tungstophosphate Nanoclusters, Eur. J. Inorg. Chem., 2021, 2021, 3819–3831 CrossRef CAS.
- D. Dermitzaki, O. Bistola, M. Pissas, V. Psycharis, Y. Sanakis and C. P. Raptopoulou, Heptanuclear heterometallic Cu5Ln2(Ln = Gd, Tb) complexes: Synthesis, crystal structures, and magnetic properties studies, Polyhedron, 2018, 150, 47–53 CrossRef CAS.
- P. Kalita, J. Goura, J. M. Herrera, E. Colacio and V. Chandrasekhar, Heterometallic Octanuclear NiII4LnIII4(Ln = Y, Gd, Tb, Dy, Ho, Er) Complexes Containing NiII2LnIII2O4 Distorted Cubane Motifs: Synthesis, Structure, and Magnetic Properties, ACS Omega, 2018, 3, 5202–5211 CrossRef CAS PubMed.
Footnote |
† Electronic supplementary information (ESI) available: Experimental details, crystal data, IR, PXRD, TG, and HRESI-MS data and additional figures for magnetic properties are provided. CCDC 2223068 contains the supplementary crystallographic data for this paper. For ESI and crystallographic data in CIF or other electronic format see DOI: https://doi.org/10.1039/d2ce01291j |
|
This journal is © The Royal Society of Chemistry 2023 |