DOI:
10.1039/D2SC06003E
(Edge Article)
Chem. Sci., 2022,
13, 14387-14394
anti-Selective synthesis of β-boryl-α-amino acid derivatives by Cu-catalysed borylamination of α,β-unsaturated esters†
Received
31st October 2022
, Accepted 23rd November 2022
First published on 24th November 2022
Abstract
A copper-catalysed regio- and diastereoselective borylamination of α,β-unsaturated esters with B2pin2 and hydroxylamines has been developed to deliver acyclic β-boryl-α-amino acid derivatives with high anti-diastereoselectivity (up to >99
:
1), which is difficult to obtain by the established methods. A chiral phosphoramidite ligand also successfully induces the enantioselectivity, giving the optically active β-borylated α-amino acids. The products can be stereospecifically transformed into β-functionalised α-amino acids, which are of potent interest in medicinal chemistry.
Introduction
Unnatural α-amino acids are key structures for the synthesis of modified peptide drugs to improve their activities and stabilities.1 In this context, the β-boryl-α-amino acids have received considerable attention since these compounds can be of high potential in the peptidemimetic strategy2 and easily transformed to natural/unnatural β-functionalised α-amino acids such as β-hydroxy-α-amino acids, which are frequently found in drugs and bioactive molecules.3 Thus, the development of efficient and stereoselective synthetic methods for their preparation is of importance not only in synthetic chemistry but also in biological and pharmaceutical research fields. The synthesis of the most simple β-borylalanine (AlaB; Scheme 1a, left) has been well developed by Curtius rearrangement,4a substitution reaction with boron electrophile,4b boron conjugate addition,4c C–H borylation,4d and decarboxylative borylation.4e On the other hand, the more sterically hindered β-disubstituted derivatives5 are still challenging synthetic targets despite the fact that such sterically congested α-amino acid derivatives are promising building blocks in the preparation of modified peptides (Scheme 1a, middle, β-disubstituted-type).1 In particular, there are a few examples of the diastereoselective synthesis of acyclic derivatives. Cho developed the copper-catalysed stereoselective addition of 1,1-diborylalkanes to α-imino esters to form the corresponding anti-β-boryl-α-amino acids (Scheme 1b).5h While a variety of α-imino esters could be employed, only the Me-substituted 1,1-diborylalkane was used in almost cases, which largely limited the substituent pattern at the β-position. On the other hand, Li reported the borylcopper-mediated borylprotonation of the α-dehydroalanine with B2pin2 and proton sources (alcohols) for preparation of the disubstituted-type β-boryl-α-amino acids (Scheme 1c).5b This method showed the remarkably high syn-diastereoselectivity. Such a stereochemical control is proposed to be induced by a strong interaction between the boron and oxygen in the β-borylated O-bound copper enolate intermediate, which regulates the molecular conformation.6 Accordingly, the subsequent protonation with alcohols proceeds on the less sterically hindered H side, giving the syn-product selectively. The obtained syn-isomer can be delivered to the β-hydroxy-α-amino acid with natural threonine-type relative stereochemistry. However, just one example was demonstrated, and the generality of this process thus still remains unclear. Furthermore, the synthesis of the most sterically demanding trisubstituted β-boryl-α-amino acids (Scheme 1a, right, β-trisubstituted-type) has not been reported yet, except for a somewhat specialised cyclic derivative.7
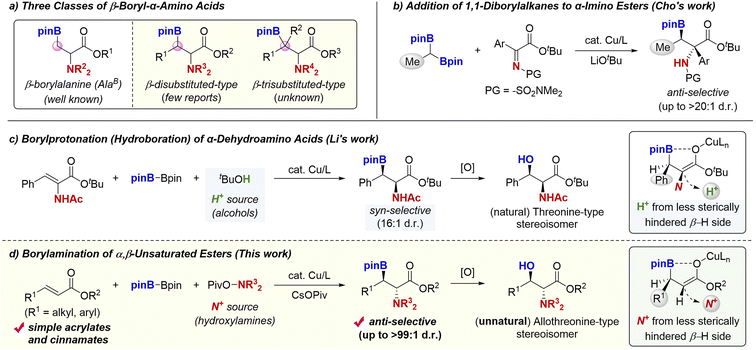 |
| Scheme 1 β-Boryl-α-amino acids: classification and their synthetic strategies. | |
Herein, we report a general and catalytic way to the acyclic anti-β-boryl-α-amino acids; a copper-catalysed borylamination8,9 of the α,β-unsaturated carboxylic acid derivatives with B2pin2 and hydroxylamines (Scheme 1d).10 Analogous to Li's proposal (Scheme 1c), the conformationally regulated β-borylated O-bound copper enolate intermediate undergoes the face-selective C–N bond formation with the hydroxylamine11 on the more sterically accessible H side, en route to the anti-β-boryl-α-amino acid (up to >99
:
1 d.r). The anti-isomer can be transformed to the unnatural allothreonine-type β-hydroxy-α-amino acids of higher value. Additional synthetic advantages of this method include (1) the ready availability of starting materials, α,β-unsaturated esters, (2) accommodation of versatile aromatic and aliphatic substituents at the β-position, and (3) the successful use of β,β-disubstituted acrylates, thus leading to the most bulky trisubstituted β-boryl-α-amino acids. Moreover, an appropriate chiral phosphine ligand makes the reaction enantioselective, affording optically active β-borylated α-amino acid derivatives. The follow-up stereospecific transformations of the Bpin moiety delivered the enantioenriched unnatural α-amino acids with versatile functionalities at the β-position. Although the related copper-catalysed boron conjugate addition of α,β-unsaturated carbonyls was well studied,12 the tandem α-functionalisation of the copper enolate intermediate still remains underdeveloped, except for the classical aldol-type processes.13
Results and discussion
Our optimisation studies commenced with β-monosubstituted unsaturated ester 1a, B2pin2 (2.5 equiv.), and O-pivaloyl-N,N-dibenzylhydroxylamine (2a-Piv; 1.5 equiv.) as model substrates (Table 1). The initial screening of ligands in 1,4-dioxane at room temperature in the presence of a Cu(OAc)2·H2O catalyst (12 mol%) and a CsOPiv base (3.0 equiv.) revealed that the monodentate phosphine ligands were more effective than bidentate ones: PPh3 showed better performance than dppbz, dppe, and Xantphos (entries 1–4). On the other hand, the diastereomeric ratio (d.r.) was uniformly high (94
:
6 to 97
:
3 anti/syn), thus suggesting that the nature of ligands on the copper gives negligible impact on the face selection in the amination step (Scheme 1d). More electron-withdrawing P(3,4,5-F3C6H2)3 slightly improved the yield (entry 6). The CsOPiv base was critical to suppress the competitive protonation of the copper enolate intermediate: Cs2CO3 and NaOtBu afforded the hydroborylated 4a as the major product (entries 7 and 8). The absence of base also resulted in no product formation, and only the undesired 4a was formed in 23% yield along with 68% recovery of 1a (entry 9). Additional investigation of ligands identified P(3,5-F2C6H3)3 to be best (entry 10). Among copper catalyst precursors we tested, Cu(OAc)2 anhydrate further increased the yield (entries 11 and 12). Finally, with a reduced catalyst loading (10 mol%) and toluene solvent instead of 1,4-dioxane, the desired product was isolated in 74% yield as the single anti-isomer (entry 13; see the ESI for more detailed optimisation studies†).14,15
Table 1 Optimisation studies for copper-catalysed borylamination of α,β-unsaturated ester 1a with B2pin2 and hydroxylamine 2a-Piva

|
Entry |
Cu cat. (mol%) |
Ligand (mol%) |
Base |
Solvent |
Yield of 3aa (%), d.rb |
Yield of 4a (%)b |
Conditions: 1a (0.25 mmol), B2pin2 (0.63 mmol), 2a-Piv (0.38 mmol), Cu(OAc)2·H2O, ligand, base (0.75 mmol), solvent (1.0 mL), RT, 18 h, N2.
Estimated by 1H NMR based on 0.25 mmol with 1-methylnaphthalene as the internal standard. The diastereomeric ratio (d.r.) is determined in the crude mixture. Isolated yield is in parentheses.
|
1 |
Cu(OAc)2·H2O (12) |
dppbz (12) |
CsOPiv |
1,4-dioxane |
16, 94 : 6 |
49 |
2 |
Cu(OAc)2·H2O (12) |
dppe (12) |
CsOPiv |
1,4-dioxane |
31, 96 : 4 |
54 |
3 |
Cu(OAc)2·H2O (12) |
Xantphos (12) |
CsOPiv |
1,4-dioxane |
0, — |
18 |
4 |
Cu(OAc)2·H2O (12) |
PPh3 (24) |
CsOPiv |
1,4-dioxane |
65, 97 : 3 |
29 |
5 |
Cu(OAc)2·H2O (12) |
P(4-MeOC6H4)3 (24) |
CsOPiv |
1,4-dioxane |
51, 97 : 3 |
38 |
6 |
Cu(OAc)2·H2O (12) |
P(3,4,5-F3C6H2)3 (24) |
CsOPiv |
1,4-dioxane |
68, 98 : 2 |
25 |
7 |
Cu(OAc)2·H2O (12) |
P(3,4,5-F3C6H2)3 (24) |
Cs2CO3 |
1,4-dioxane |
40, 97 : 3 |
59 |
8 |
Cu(OAc)2·H2O (12) |
P(3,4,5-F3C6H2)3 (24) |
NaOtBu |
1,4-dioxane |
22, 97 : 3 |
78 |
9 |
Cu(OAc)2·H2O (12) |
P(3,4,5-F3C6H2)3 (24) |
None |
1,4-dioxane |
0, — |
23 |
10 |
Cu(OAc)2·H2O (12) |
P(3,5-F2C6H3)3 (24) |
CsOPiv |
1,4-dioxane |
76, 96 : 4 |
21 |
11 |
Cu(OAc)2 (12) |
P(3,5-F2C6H3)3 (24) |
CsOPiv |
1,4-dioxane |
80, 97 : 3 |
18 |
12 |
CuCl (12) |
P(3,5-F2C6H3)3 (24) |
CsOPiv |
1,4-dioxane |
78, 97 : 3 |
21 |
13 |
Cu(OAc)2 (10) |
P(3,5-F2C6H3)3 (20) |
CsOPiv |
Toluene |
80 (74), >99 : 1 |
20 |
With conditions of entry 13 in Table 1, we examined the generality of the copper-catalysed borylamination reaction (Scheme 2). Both simple crotonate and γ-branched acrylates underwent the reaction smoothly to form the desired products 3ba–da in good yields with high anti-diastereoselectivity. The reaction was tolerant of versatile functional groups including alkyl halide, ether, ester, acetal, nitrile, and phthalimide moieties to afford the corresponding β-boryl-α-amino acids 3ea–la in moderate to good yields as the single anti-diastereomers. Additionally, the electronically diverse cinnamates could also be employed; the electron-donating methoxy and electron-withdrawing bromo, chloro, fluoro, and trifluoromethyl groups all were tolerated to afford the corresponding β-boryl-α-amino acid derivatives 3ma–ra in good yields with the exclusive anti-selectivity. The heteroaromatic thiophene and pyridine substrates were also adopted to deliver the targeted α-amino acids 3sa–ta. Notably, the α-amino acid containing the gem-boryl-silyl structure was also prepared from the β-silyl acrylate (3ua). The cyclobutenecarboxylate ester was successfully converted to the α,α-disubstituted β-boryl-α-amino acid 3va. Furthermore, the copper catalyst was applicable to the α,β-unsaturated amide to give the β-boryl-α-amino amide 3wa. The relative stereochemistry of 3ba was confirmed by X-ray analysis (CCDC 2206174),† and others were assigned by analogy.
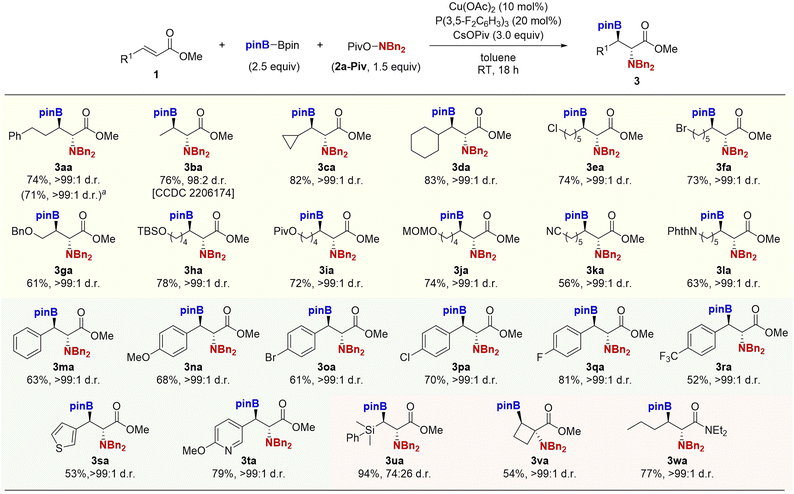 |
| Scheme 2 Copper-catalysed borylamination of various α,β-unsaturated esters 1 with B2pin2 and N,N-dibenzylhydroxylamine 2a-Piv. Conditions: 1 (0.25 mmol), B2pin2 (0.63 mmol), 2a-Piv (0.38 mmol), Cu(OAc)2 (0.025 mmol), P(3,5-F2C6H3)3 (0.050 mmol), CsOPiv (0.75 mmol), toluene (1.0 mL), RT, 18 h, N2. Isolated yields are given. aOn a 1.0 mmol scale. | |
We next investigated the scope of the hydroxylamines (Scheme 3). While the pivalate leaving group was optimal in the case of the N,N-dibenzylamine, other acyclic and cyclic amines required the more sterically hindered and strongly electron-donating o,o-dimethoxybenzoyloxy leaving group for the acceptable reaction efficiency and diastereoselectivity. For example, the borylamination of 1a with O-pivaloyl-N,N-diethylhydroxylamine (2b-Piv) resulted in 43% yield of 3ba with 88
:
12 d.r. Less sterically hindered benzoyl-type 2b-Bz largely dropped the diastereomeric ratio. Introduction of electron-donating groups improved the stereoselectivity (2b-Mes, 2b-MeO, and 2b-NMe2), with 2b-(MeO)2 proving to be best (65% 1H NMR yield, 90
:
10 d.r). The observed trend was consistent with our proposal in Scheme 1d, where the anti-selectivity is generally induced by the steric repulsion between the substituent at the β-position and amino electrophile. Moreover, the electron-donating substituent can suppress the direct reaction of the borylcopper with the hydroxylamine to avoid its unproductive decomposition. The modified leaving group was also effective for N-benzyl-N-methylamine, N,N-diallylamine, piperidine, morpholine, and thiomorpholine to deliver the targeted β-boryl-α-amino acids 3ac–ag in 41–85% yields with synthetically useful diastereomeric ratios (80
:
20–95
:
5 anti/syn). The positive effects of o,o-dimethoxybenzoyl group were more remarkable in the reaction with relatively small cyclic amines such as piperidine (3ae). A similar beneficial effect of the o,o-dimethoxy substitution was reported in the nickel-catalysed carboamination reaction of alkenes developed by Engle.16
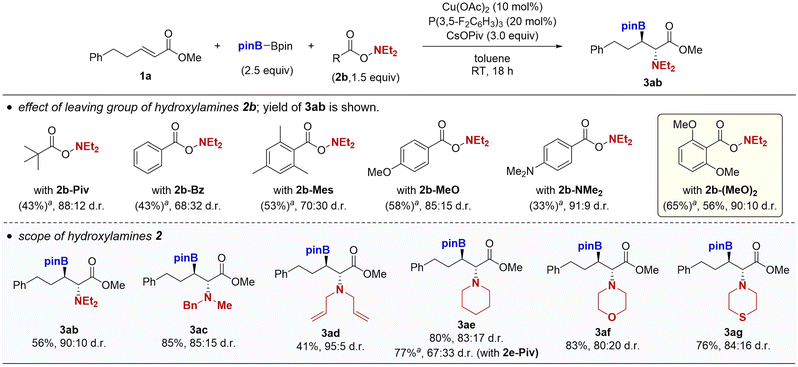 |
| Scheme 3 Copper-catalysed borylamination of α,β-unsaturated esters 1a with B2pin2 and various hydroxylamines 2. Conditions: 1a (0.25 mmol), B2pin2 (0.63 mmol), 2 (0.38 mmol), Cu(OAc)2 (0.025 mmol), P(3,5-F2C6H3)3 (0.050 mmol), CsOPiv (0.75 mmol), toluene (1.0 mL), RT, 18 h, N2. Isolated yields are given. aNMR yield. | |
The aforementioned success prompted us to attempt the borylamination of β,β-disubstituted α,β-unsaturated esters (Scheme 4). This is highly challenging because the rate of 1,4-addition of borylcopper species to the sterically congested β,β-disubstituted unsaturated esters is much slower than that of β-monosubstituted ones to predominantly decompose the hydroxylamine by the direct reaction.17 Actually, in the reaction of the β-methylcinnamate 1A and piperidine derivative 2e-(MeO)2, the Cu(OAc)2/P(3,5-F2C6H3)3 catalyst system did not provide the target product 3Ae at all, even with the assistance of the modified o,o-dimethoxybenzoyloxy leaving group. Thus, we again performed optimisation studies. After extensive re-screening of various catalysts and ligands, the combination of a cationic copper salt, Cu(CH3CN)4PF6 and TMS-modified dppe ligand18 was found to dramatically promote the reaction to give the trisubstituted β-boryl-α-amino acids 3Ae in 86% yield (see the ESI† for details). Although the diastereomeric ratio was moderate (72
:
28 anti/syn),19 to the best of our knowledge, this is the first successful example of preparation of acyclic β-boryl-α-amino acid derivatives with the tetra-substituted carbon centre at the β-position. The newly developed copper catalysis was applicable to other β-methylcinnamates (3Be–Ee) and acrylates (3Fe–He). As the hydroxylamine coupling partner, morpholine (3Af), N-benzyl-N-methylamine (3Ac), and N,N-diethylamine (3Ab) also worked well to furnish the corresponding trisubstituted β-boryl-α-amino acids in moderate to good yields.
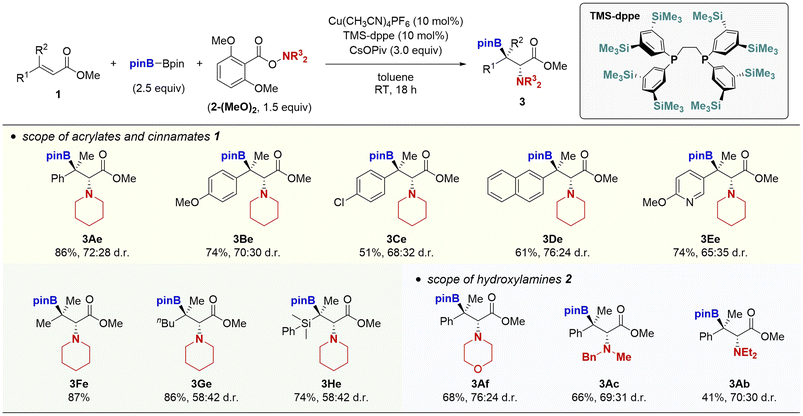 |
| Scheme 4 Copper-catalysed borylamination of β,β-disubstituted cinnamates and acrylates. Conditions: 1 (0.25 mmol), B2pin2 (0.63 mmol), 2-(MeO)2 (0.38 mmol), Cu(CH3CN)4PF6 (0.025 mmol), TMS-dppe (0.025 mmol), CsOPiv (0.75 mmol), toluene (1.0 mL), RT, 18 h, N2. Isolated yields are given. | |
We next turned attention to the diastereo- and enantioselective borylamination. Our initial attempts of common chiral bidentate ligands, such as Quinox P*, Ph-BPE, SEGPHOS, BINAP, and Josiphos led to no product formation or low enantioselectivity. On the other hand, several chiral monodentate phosphoramidite ligands were found to be good candidates. In particular, TADDOL-based piperidine phosphoramidite L20 successfully induced the high enantioselectivity as well as diastereoselectivity in the reaction of tBu ester 1a-OtBu and O-(4-MeO)benzoyl-N,N-dibenzylhydroxylamine (2a-OMe) to furnish 3aa-OtBu in 74% yield with 95
:
5 d.r. and 91
:
9 e.r. (Scheme 5a). The (2R,3R) absolute configuration was determined by comparison of retention time in chiral HPLC analysis with the known compound after the oxidative derivatisation (see the ESI† for details). The asymmetric catalysis was compatible with the alkyl chloride, alkyl bromide, silyl ether, pivaloyl ester, acetal, and nitrile functionalities, and the functionalised β-boryl-α-amino acids 3ea-OtBu, 3fa-OtBu, 3ha-OtBu, 3ia-OtBu, 3ja-OtBu, and 3ka-OtBu were prepared in good yields with 89
:
11 to 90
:
10 e.r. Cinnamate 1m-OtBu was also applicable to the enantioselective borylamination with synthetically acceptable enantioselectivity. In addition to 2a-OMe, N-benzyl-N-methylamine 2c-(OMe)2 and morpholine 2f-(OMe)2 were viable to produce 3ac-OtBu and 3af-OtBu with good enantiomeric ratios. Moreover, single recrystallisation from Et2O/hexane afforded the optically pure β-boryl-α-amino acids (3aa-OtBu and 3ba-OtBu) with adjacent two stereocentres (>99
:
1 d.r. and >99
:
1 e.r).
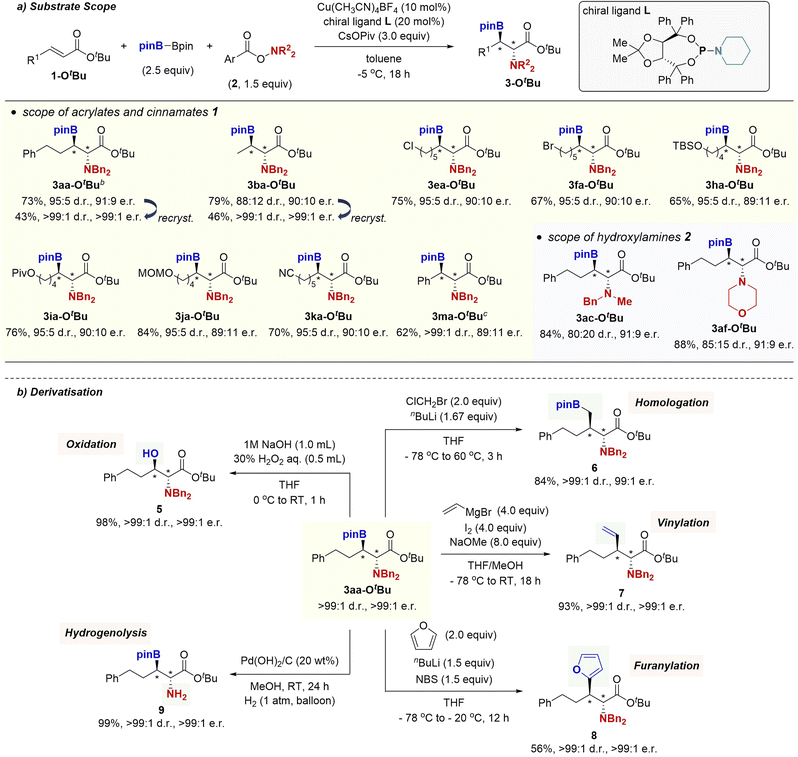 |
| Scheme 5 (a) Copper-catalysed enantioselective borylamination of α,β-unsaturated estersa and (b) derivatisations of 3aa-OtBu. aConditions: 1-OtBu (0.25 mmol), B2pin2 (0.63 mmol), 2 (0.38 mmol), Cu(CH3CN)4BF4 (0.025 mmol), L (0.050 mmol), CsOPiv (0.75 mmol), toluene (1.0 mL), −5 °C, 18 h, N2. Ar = 4-MeOC6H4- (2a-OMe) or 2,6-(MeO)2C6H3- (2c-(OMe)2 and 2f-(OMe)2). Isolated yields are given. bOn a 1.0 mmol scale. cOn a 0.50 mmol scale. | |
To further demonstrate the synthetic utility of the copper-catalysed borylamination, we converted the stereochemically pure β-boryl-α-amino acid 3aa-OtBu into functionalised α-amino acid derivatives based on the established organoboron chemistry (Scheme 5b). The enantioenriched β-hydroxy-α-amino acid 5 with the unnatural anti-configuration could be easily accessed by oxygenation with H2O2. Matteson homologation21 with the in situ-generated LiCH2Cl was also possible to afford optically active 6. Additionally, Zweifel-type olefination22 delivered the vinylation product 7 in 93% yield with complete stereoretention. The coupling with furan could also be conducted under conditions developed by Aggarwal23 to furnish the cross-coupling product 8 in 88% yield without any erosion of the stereochemistry. Furthermore, the hydrogenolysis of N-benzyl groups proceeded without any detectable deborylation to give the primary amine 9 in 78% yield.
Conclusions
We have developed an anti-selective copper-catalysed borylamination of α,β-unsaturated esters with B2pin2 and hydroxylamines to give the corresponding acyclic β-boryl-α-amino acid derivatives with high diastereoselectivity (up to >99
:
1 anti/syn). The use of the amino electrophile is critical to induce the anti-stereochemistry in the acyclic system, which is otherwise difficult to obtain by the reported procedures. Additionally, the originally developed modified dppe-type ligand accommodates the more sterically congested β,β-disubstituted cinnamates and acrylates to form the non-trivial β-trisubstituted derivatives. Furthermore, the enantioselectivity is successfully induced by the appropriate chiral phosphoramidite ligand. The obtained optically active β-boryl-α-amino acid with adjacent two stereocentres can be easily transformed into highly functionalised α-amino acids with the anti-stereochemistry, which demonstrates the synthetic value of our protocol. More detailed mechanistic studies and asymmetric synthesis of the most congested β-trisubstituted-type β-boryl-α-amino acids24 are ongoing in our laboratory.
Data availability
All experimental procedures and spectroscopic data can be found in the ESI.†
Author contributions
S. N. and K. H. conceived the idea. S. N. performed all experiments. K. H. supervised the project. Y. N. supported X-ray analysis. The paper was written by S. N. and K. H. All the authors discussed the results and commented on the manuscript.
Conflicts of interest
There are no conflicts to declare.
Acknowledgements
This work was supported by JSPS KAKENHI Grant Nos JP 22H02077 (Grant-in-Aid for Scientific Research(B), to K. H.), and JP 22J10951 (Grant-in-Aid for JSPS Research Fellow, to S. N.) as well as by JST FOREST Program, Grant Number JPMJFR 211X to K. H. K.H. also acknowledges Toyota Riken Scholar for financial support.
Notes and references
- For selected reviews, see:
(a) Y. Hamada and T. Shioiri, Chem. Rev., 2005, 105, 4441 CrossRef CAS PubMed;
(b) C. Najera and J. M. Sansano, Chem. Rev., 2007, 107, 4584 CrossRef CAS PubMed;
(c) C. T. Walsh, R. V. O'Brien and C. Khosla, Angew. Chem., Int. Ed., 2013, 52, 7098 CrossRef CAS PubMed;
(d) M. A. T. Blaskovich, J. Med. Chem., 2016, 59, 10807 CrossRef CAS PubMed;
(e) A. A. Vinogradov, Y. Yin and H. Suga, J. Am. Chem. Soc., 2019, 141, 4167 CrossRef CAS PubMed;
(f) Y. Ding, J. P. Ting, J. Liu, S. Al-Azzam, P. Pandya and S. Afshar, Amino Acids, 2020, 52, 1207 CrossRef CAS PubMed;
(g) J. B. Hedges and K. S. Ryan, Chem. Rev., 2020, 120, 3161 CrossRef CAS PubMed;
(h) M. Muttenthaler, G. F. King, D. J. Adams and P. F. Alewood, Nat. Rev. Drug Discovery, 2021, 20, 309 CrossRef CAS PubMed.
- For selected examples of biological activities of AlaB derivatives, see:
(a) J. T. Lee, D. Y. Chen, Z. Yang, A. D. Ramos, J. J. D. Hsieh and M. Bogyo, Bioorg. Med. Chem. Lett., 2009, 19, 5086 CrossRef CAS PubMed;
(b) E. Bassini, S. Gazzotti, F. Sannio, L. L. Presti, J. Sgrignani, J.-D. Docquier, G. Grazioso and A. Silvani, Antibiotics, 2020, 9, 249 CrossRef CAS PubMed;
(c) T. A. Mollner, P. G. Isenegger, B. Josephson, C. Buchanan, L. Lercher, D. Oehlrich, D. F. Hansen, S. Mohammed, A. J. Baldwin, V. Gouverneur and B. G. Davis, Nat. Chem. Biol., 2021, 17, 1245 CrossRef CAS PubMed.
- For selected examples of biological activities of β-hydroxy-α-amino acids, see:
(a) Y. Sugiura, H. Tanaka, Y. Mino, T. Ishida, N. Ota, M. Inoue, K. Nomoto, H. Yoshioka and T. Takemoto, J. Am. Chem. Soc., 1981, 103, 6979 CrossRef CAS;
(b) A. Zampella, M. V. D'Auria, L. G. Paloma, A. Casapullo, L. Minale, C. Debitus and Y. Henin, J. Am. Chem. Soc., 1996, 118, 6202 CrossRef CAS;
(c) K. C. Nicolaou, C. N. C. Boddy, S. Bräse and N. Winssinger, Angew. Chem., Int. Ed., 1999, 38, 2096 CrossRef;
(d) D. S. Goldstein, Cardiovasc. Drug Rev., 2006, 24, 189 CrossRef CAS PubMed;
(e) D. A. Wirtz, K. C. Ludwig, M. Arts, C. E. Marx, S. Krannich, P. Barac, S. Kehraus, M. Josten, B. Henrichfreise, A. Müller, G. M. König, A. J. Peoples, A. Nitti, A. L. Spoering, L. L. Ling, K. Lewis, M. Crüsemann and T. Schneider, Angew. Chem., Int. Ed., 2021, 60, 13579 CrossRef CAS PubMed . Also see ref. 1a,b,d..
-
(a) D. H. Kinder and M. M. Ames, J. Org. Chem., 1987, 52, 2452 CrossRef CAS;
(b) M. N. Kenworthy, J. P. Kilburn and R. J. K. Taylor, Org. Lett., 2004, 6, 19 CrossRef CAS PubMed;
(c) F. Bartoccini, S. Bartolucci, S. Lucarini and G. Piersanti, Eur. J. Org. Chem., 2015, 15, 3352 CrossRef;
(d) J. He, H. Jiang, R. Takise, R.-Y. Zhu, G. Chen, H.-X. Dai, T. G. M. Dhar, J. Shi, H. Zhang, P. T. W. Cheng and J.-Q. Yu, Angew. Chem., Int. Ed., 2016, 55, 785 CrossRef CAS PubMed;
(e) F. Zhu, E. Miller, W. C. Powell, K. Johnson, A. Beggs, G. E. Evenson and M. A. Walczak, Angew. Chem., Int. Ed., 2022, 61, e202207153 CAS.
-
(a) Z.-T. He, Y.-S. Zhao, P. Tian, C.-C. Wang, H.-Q. Dong and G.-Q. Lin, Org. Lett., 2014, 16, 1426 CrossRef CAS PubMed;
(b) J.-B. Xie, S. Lin, S. Qiao and G. Li, Org. Lett., 2016, 18, 3926 CrossRef CAS PubMed;
(c) B. Carboni, M. Ollivault, F. L. Bouguenec, R. Carri and M. Jazotdi, Tetrahedron Lett., 1997, 38, 6665 CrossRef CAS;
(d) A. Lopez-Perez, M. Segler, J. Adrio and J. C. Carretero, J. Org. Chem., 2011, 76, 1945 CrossRef CAS PubMed;
(e) K. Kubota, K. Hayama, H. Iwamoto and H. Ito, Angew. Chem., Int. Ed., 2015, 54, 8809 CrossRef CAS PubMed;
(f) K. Hayama, K. Kubota, H. Iwamoto and H. Ito, Chem. Lett., 2017, 46, 1800 CrossRef CAS;
(g) K. Hayama, R. Kojima, K. Kubota and H. Ito, Org. Lett., 2020, 22, 739 CrossRef CAS PubMed;
(h) J. Kim, M. Shin and S. H. Cho, ACS Catal., 2019, 9, 8503 CrossRef CAS.
- A similar O to B coordination in the β-borylated carbonyl compounds is often proposed to explain the observed stereochemistry
(a) A. Whiting, Tetrahedron Lett., 1991, 32, 1503 CrossRef CAS;
(b) R. J. Mears and A. Whiting, Tetrahedron, 1993, 49, 177 CrossRef CAS;
(c) G. Conole, R. J. Mears, H. De Silva and A. Whiting, J. Chem. Soc., Perkin Trans. 1, 1995, 1825 RSC;
(d) R. J. Mears, H. E. Sailes, J. P. Watts and A. Whiting, J. Chem. Soc., Perkin Trans. 1, 2000, 3250 RSC;
(e) H. E. Sailes, J. P. Watts and A. Whiting, J. Chem. Soc., Perkin Trans. 1, 2000, 3362 RSC;
(f) Y.-J. Zuo, X.-T. Chang, Z.-M. Hao and C.-M. Zhong, Org. Biomol. Chem., 2017, 15, 6323 RSC;
(g) Y.-J. Zuo, Z. Zhong, Y. Fan, X. Li, X. Chen, Y. Chang, R. Song, X. Fu, A. Zhang and C.-M. Zhong, Org. Biomol. Chem., 2018, 16, 9237 RSC;
(h) Y.-P. Bi, H.-M. Wang, H.-Y. Qu, X.-C. Liang, Y. Zhou, X.-Y. Li, D. Xu, M.-H. Shen and H.-D. Xu, Org. Biomol. Chem., 2019, 17, 1542 RSC;
(i) E. M. Larin, J. Loup, I. Polishchuk, R. J. Ross, A. Whyte and M. Lautens, Chem. Sci., 2020, 11, 5716 RSC;
(j) M. Zhan, Z. Ding, S. Du, H. Chen, C. Feng, M. Xu, Z. Liu, M. Zhang, C. Wu, Y. Lan and P. Li, Nat. Commun., 2020, 11, 792 CrossRef CAS PubMed.
- M. R. Becker, E. R. Wearing and C. S. Schindler, Nat. Chem., 2020, 12, 898 CrossRef CAS PubMed.
- For contributions from our group, see:
(a) N. Matsuda, K. Hirano, T. Satoh and M. Miura, J. Am. Chem. Soc., 2013, 135, 4934 CrossRef CAS PubMed;
(b) R. Sakae, K. Hirano and M. Miura, J. Am. Chem. Soc., 2015, 137, 6460 CrossRef CAS PubMed;
(c) R. Sakae, K. Hirano, T. Satoh and M. Miura, Angew. Chem., Int. Ed., 2015, 54, 613 CAS;
(d) D. Nishikawa, K. Hirano and M. Miura, Org. Lett., 2016, 18, 4856 CrossRef CAS PubMed;
(e) K. Kato, K. Hirano and M. Miura, Angew. Chem., Int. Ed., 2016, 55, 14400 CrossRef CAS PubMed.
- For contributions from other groups, see:
(a) A. Parra, L. Amenos, M. Guisan-Ceinos, A. Lopez, L. L. G. Ruano and M. Tortosa, J. Am. Chem. Soc., 2014, 136, 15833 CrossRef CAS PubMed;
(b) C.-H. Yang, Y.-S. Zhang, W.-W. Fan, G.-Q. Liu and Y.-M. Li, Angew. Chem., Int. Ed., 2015, 54, 12636 CrossRef CAS PubMed;
(c) H.-C. Jiang, X.-Y. Tang and M. Shi, Chem. Commun., 2016, 52, 5273 RSC.
- For seminal work on electrophilic amination with hydroxylamines, see:
(a) H. Tsutsui, Y. Hayashi and K. Narasaka, Chem. Lett., 1997, 26, 317 CrossRef;
(b) A. M. Berman and J. S. Johnson, J. Am. Chem. Soc., 2004, 126, 5680 CrossRef CAS PubMed;
(c) S. Liu and L. S. Liebeskind, J. Am. Chem. Soc., 2008, 130, 6918 CrossRef CAS PubMed ; representative reviews;
(d) E. Erdik and M. Ay, Chem. Rev., 1989, 89, 1947 CrossRef CAS;
(e) K. Narasaka and M. Kitamura, Eur. J. Org. Chem., 2005, 4505 CrossRef CAS;
(f) E. Ciganek, Org. React., 2008, 72, 1 Search PubMed;
(g) T. J. Barker and E. R. Jarvo, Synthesis, 2011, 24, 3954 Search PubMed;
(h) M. Corpet and C. Gosmini, Synthesis, 2014, 46, 2258 CrossRef CAS;
(i) M. T. Pirnot, Y.-M. Wang and S. L. Buchwald, Angew. Chem., Int. Ed., 2016, 55, 48 CrossRef CAS PubMed;
(j) X. Dong, Q. Liu, Y. Dong and H. Liu, Chem. – Eur. J., 2017, 23, 2481 CrossRef CAS PubMed;
(k) M. T. Pirnot, Y.-M. Wang and S. L. Buchwald, Angew. Chem., Int. Ed., 2016, 55, 48 CrossRef CAS PubMed;
(l) R. Y. Liu and S. L. Buchwald, Acc. Chem. Res., 2020, 53, 1229 CrossRef CAS PubMed;
(m) K. Hirano and M. Miura, J. Am. Chem. Soc., 2022, 144, 648 CrossRef CAS PubMed.
- For recent computational studies on the electrophilic amination of organocopper species with the hydroxylamine, see:
(a) S. Tobisch, Chem. – Eur. J., 2016, 22, 8290 CrossRef CAS PubMed;
(b) S. Tobisch, Chem. – Eur. J., 2017, 23, 17800 CrossRef CAS PubMed;
(c) S. Tobisch, Chem. Sci., 2017, 8, 4410 RSC.
- For selected examples, see:
(a) J.-E. Lee and J. Yun, Angew. Chem., Int. Ed., 2008, 120, 151 CrossRef;
(b) X. Feng and J. Yun, Chem. Commun., 2009, 45, 6577 RSC;
(c) H.-S. Sim, X. Feng and J. Yun, Chem. – Eur. J., 2009, 15, 1939 CrossRef CAS PubMed;
(d) W. J. Fleming, H. Müller-Bunz, V. Lillo, E. Fernandez and P. J. Guiry, Org. Biomol. Chem., 2009, 7, 2520 RSC;
(e) J. A. Schiffner, K. Müther and M. Oestreich, Angew. Chem., Int. Ed., 2010, 49, 1194 CrossRef CAS PubMed;
(f) L. Mantilli and C. Mazet, ChemCatChem, 2010, 2, 501 CrossRef CAS;
(g) J. K. Park, H. H. Lackey, M. D. Rexford, K. Kovnir, M. Shatruk and D. T. McQuade, Org. Lett., 2010, 12, 5008 CrossRef CAS PubMed.
- For some related papers on copper-catalysed carboboration of α,β-unsaturated carbonyl compounds, see:
(a) A. Welle, V. Cirriez and O. Riant, Tetrahedron, 2012, 68, 3435 CrossRef CAS;
(b) A. R. Burns, G. S. Gonzalez and H. W. Lam, Angew. Chem., Int. Ed., 2012, 51, 10827 CrossRef CAS PubMed;
(c) K. Semba and Y. Nakao, J. Am. Chem. Soc., 2014, 136, 7567 CrossRef CAS PubMed;
(d) J. Sendra, R. Manzano, E. Reyes, J. L. Vicario and E. Fernandez, Angew. Chem., Int. Ed., 2020, 59, 2100 CrossRef CAS PubMed;
(e) K. Nguyen, H. A. Clement, L. Bernier, J. W. Coe, W. Farrell, C. J. Helal, M. R. Reese, N. W. Sach, J. C. Lee and D. G. Hall, ACS Catal., 2021, 11, 404 CrossRef CAS;
(f) G. Dahiya, M. Pappoppula and A. Aponick, Angew. Chem., Int. Ed., 2021, 60, 19604 CrossRef CAS PubMed . Also see ref.6f,g,h,i..
- The monodentate phosphine ligand is believed to accelerate the C–N bond forming step. In our previous work on the copper-catalysed borylamination of unactivated terminal alkenes, styrenes, alkenylboronates, and vinylsilanes, the bidentate phosphine ligands showed better performance than the monodentate ones (see ref. 8). The bidentate phosphine ligands generally promote the addition step of borylcopper species to alkenes, resulting in the suppression of the decomposition of the amino electrophile with the borylcopper. On the other hand, for the activated alkenes, such as acrylates, the corresponding boryl-conjugate addition is more feasible and proceeds efficiently by using either bidentate or monodentate phosphine ligand (see ref. 12). Therefore, under the present copper catalysis, the monodentate phosphine ligand is more critical in the amination step..
- We could not identify the H+ source for the undesired hydroborylated product 4a. The generation of 4a could not be suppressed by the addition of molecular sieves 4A or under rigorously dry reaction conditions using a glovebox filled with nitrogen. Meanwhile, no deuterated 4a–d was observed when the reaction was performed in toluene-d8 under otherwise identical conditions..
- T. Kang, N. Kim, P. T. Cheng, H. Zhang, K. Foo and K. M. Engle, J. Am. Chem. Soc., 2021, 143, 13962 CrossRef CAS PubMed.
- For selected examples of the copper-catalysed hydroboration of β,β-disubstituted α,β-unsaturated carbonyl compounds, see:
(a) I.-H. Chen, L. Yin, W. Itano, M. Kanai and M. Shibasaki, J. Am. Chem. Soc., 2009, 131, 11664 CrossRef CAS PubMed;
(b) X. Feng and J. Yun, Chem. – Eur. J., 2010, 16, 13609 CrossRef CAS PubMed;
(c) J. M. O'Brien, K.-S. Lee and A. H. Hoveyda, J. Am. Chem. Soc., 2010, 132, 10630 CrossRef PubMed;
(d) I.-H. Chen, M. Kanai and M. Shibasaki, Org. Lett., 2010, 12, 4098 CrossRef CAS PubMed;
(e) S. Kobayashi, P. Xu, T. Endo, M. Ueno and T. Kitanosono, Angew. Chem., Int. Ed., 2012, 51, 12763 CrossRef CAS PubMed . Also see ref. 12e,f..
- We do not have an explanation for the exact reason why TMS-dppe showed uniquely higher performance than other dppe-type ligands, but one possibility is the attractive London dispersion, which can accelerate the electrophilic amination step as well as the conjugate addition, see:
(a) D. J. Liptrot and P. P. Power, Nat. Rev. Chem., 2017, 1, 1 CrossRef;
(b) G. Lu, R. Y. Liu, Y. Yang, C. Fang, D. S. Lambrecht, S. L. Buchwald and P. Liu, J. Am. Chem. Soc., 2017, 139, 16548 CrossRef CAS PubMed;
(c) A. A. Thomas, K. Speck, I. Lu, Z. Kevlishvili, P. Liu and S. L. Buchwald, J. Am. Chem. Soc., 2018, 140, 13976 CrossRef CAS PubMed;
(d) Y. Xi, B. Su, X. Qi, S. Pedram, P. Liu and J. F. Hartwig, J. Am. Chem. Soc., 2020, 142, 18213 CrossRef CAS PubMed;
(e) T. Kang, T. G. Erbay, K. L. Xu, G. M. Gallego, A. Burtea, S. K. Nair, R. L. Patman, R. Zhou, S. C. Sutton, I. J. McAlpine, P. Liu and K. M. Engle, ACS Catal., 2020, 10, 13075 CrossRef CAS PubMed.
- The relative stereochemistry of trisubstituted β-boryl-α-amino acids could not be confirmed by the X-ray analysis because all obtained products were less crystalline. Additionally, the products could not be derived to any known compounds. Therefore, the relative stereochemistry was just assigned by the analogy to the disubstituted β-boryl-α-amino acids.
- For a review of TADDOL derivatives, see: D. Seebach, A. K. Beck and A. Heckel, Angew. Chem., Int. Ed., 2001, 40, 92 CrossRef CAS PubMed.
-
(a) H. C. Brown, S. M. Singh and M. V. Rangaishenvi, J. Org. Chem., 1986, 51, 3150 CrossRef CAS;
(b) D. S. Matteson, Chem. Rev., 1989, 89, 1535 CrossRef CAS.
-
(a) R. P. Sonawane, V. Jheengut, C. Rabalakos, R. Larouche-Gauthier, H. K. Scott and V. K. Aggarwal, Angew. Chem., Int. Ed., 2011, 50, 3760 CrossRef CAS PubMed;
(b) R. J. Armstrong and V. K. Aggarwal, Synthesis, 2017, 49, 3323 CrossRef CAS.
- A. Bonet, M. Odachowski, D. Leonori, S. Essafi and V. K. Aggarwal, Nat. Chem., 2014, 6, 584 CrossRef CAS PubMed.
- We preliminarily screened some chiral ligands and found (S,S)-Xyl-BDPP to induce enantioselectivity, but the enantiomeric ratio was just 86
:
14. See the ESI† for more details..
|
This journal is © The Royal Society of Chemistry 2022 |