DOI:
10.1039/D2RA05289J
(Paper)
RSC Adv., 2022,
12, 29214-29222
Mechanism of calcium in melatonin enhancement of functional substance-phenolic acid in germinated hulless barley†
Received
23rd August 2022
, Accepted 6th October 2022
First published on 13th October 2022
Abstract
Phenolic acid is a physiologically active substance that has a variety of effects on humans. Barley sprouts are often used as food ingredients to enrich phenolic acids and to further produce functional foods rich in phenolic acids. In this study, the mechanism of Ca2+ involvement in regulating phenolic acid biosynthesis and plant growth in barley by melatonin (MT) under NaCl stress was investigated. According to the studies, MT (25 μM) increased total calcium content, induced Ca2+ burst, and up-regulated the gene expression of calcium-regulated protein-dependent protein kinase and calcium-binding protein transcription-activating protease in NaCl-stressed (60 mM) barley. Exogenous MT and its combined CaCl2 (0.4 mM) significantly promoted phenolic acid biosynthesis by increasing the activity of C4H and PAL, and induced gene expression of PAL and F5H. The addition of exogenous CaCl2 and MT caused systemic tolerance in NaCl-stressed barley, as determined by a decrease in the fluorescence intensity of hydrogen peroxide and oxygen radical anions as well as an enhancement in the antioxidant enzyme, thus significantly increasing sprout length and fresh weight. In addition, combined use of MT with Ca2+ antagonists (lanthanum chloride or ethylene glycol tetraacetic acid), impaired all impacts as mentioned above. These findings imply that Ca2+ participated in MT-induced phenolic acid biosynthesis and growth improvement in NaCl-stressed barley.
1. Introduction
Barley is among the most significant cereal crops worldwide and contains a variety of bioactive substances, along with phenolic acids, tocopherols, and folic acid.1 Among them, phenolic acids have various physiological impacts on the body, including antioxidant, anticancer, and antibacterial.2 Because the human body is unable to manufacture phenolic acids, they must be obtained from the diet,3 the development of functional foods rich in phenolic acids has received much attention from scholars. Studies have shown that environmental stimuli, especially salt stress, can significantly increase the phenolic acid content in barley, but also cause inhibition of barley growth.4
Melatonin (MT), as a plant hormone, is efficient in regulating development of plants5 as well as the detrimental effects of abiotic stress on plants.6 MT has been discovered in recent years to have a significant regulatory function in forming secondary metabolites in plants. Exogenous MT treatment could enhance levels of flavonoids, carotenoids, and isoflavones in citrus,7 tomato,8 and soybean,9 respectively. Previously, we also found that MT considerably stimulated the biosynthesis of phenolic acids in barley under salt stress10 and effectively improved plant stress resistance. These MT effects are frequently linked to higher antioxidant enzyme activity and transcriptional activation of relevant secondary metabolite genes.11 However, MT-related growth, defense responses, and metabolite synthesis of plants are complex, and the signaling pathway that MT regulates these responses is poorly understood.
Ca2+ has a vital role in biological signaling as a second messenger. Plant responses to environmental stimuli, such as NaCl stress, include a spike in Ca2+ levels that activates Ca2+ signaling networks and receptors.12 Ca2+ signaling alleviates plant injury through proline accumulation, enhancement of antioxidant enzyme activity, and regulation of ion homeostasis in tissues.13 By promoting the flavonoid signaling system's gene expression, the calcium signaling route has also been shown to be essential for the anthocyanin production in grapes.14 Notably, an amount of recent studies have revealed that MT can exert a regulatory effect on the calcium signaling pathway in plants. MT induced the relative genes expression involved in Ca2+ signaling in plants.15 Meanwhile, combined MT and Ca2+ treatment could promote photosynthesis and salt tolerance of Dracocephalum kotschyi,16,17 improve postharvest quality of cassava,12 and enhances resilience against arsenic toxicity of Vicia faba.18 The only two studies showed that MT induced the synthetic of apigenin, luteolin, and rosmarinic acid in NaCl-stressed Dracocephalum kotschyi19 and astaxanthin in Haematococcus pluvialis,20 respectively, dependent on Ca2+/CaM signaling. To determine if Ca2+ is involved in the MT-induced production of specific metabolites, more research is required. In particular, the role of Ca2+ in the MT-induced phenolic acid enrichment and growth improvement in NaCl-stressed barley remains to be further elucidated.
Lanthanum chloride (LaCl3) and ethylene glycol tetraacetic acid (EGTA) are Ca2+ plasmalemma channel blockers and Ca2+ chelators, respectively. LaCl3 inhibits the release of extracellular calcium stores by blocking the opening of Ca2+ plasmalemma channel, and attenuates Ca2+ flux on protoplasts, cytoplasmic membranes, and tonoplast vesicles. Therefore, the effects of MT combined with exogenous CaCl2 and its antagonist (LaCl3 and EGTA) treatment on growth characteristics, phenolic acid, and calcium content, activities, as well as gene expressions of critical enzymes required for the synthesis of phenolic acids and Ca2+ signaling metabolic pathways in barley were investigated. This made it possible to learn more about the intrinsic function of Ca2+ signaling involved in MT-induced phenolic acid biosynthesis and growth improvement in germinated barley under NaCl stress.
2. Materials and methods
2.1 Plant material and treatments
Barley seeds should be soaked in distilled water for 6 h at 25 °C, then put them in a germination box and germinate for 6 days at 25 °C in the dark. Different treatments were used:
(1) N: 60 mM NaCl; (2) NM: 60 mM NaCl plus 20 μM MT; (3) NC: 60 mM NaCl plus 0.4 mM CaCl2; (4) NMC: 60 mM NaCl plus 20 μM MT plus 0.4 mM CaCl2; (5) NL: 60 mM NaCl plus 5 mM LaCl3; (6) NML: 60 mM NaCl plus 20 μM MT plus 5 mM LaCl3; (7) NE: 60 mM NaCl plus 0.4 mM EGTA; (8) NME: 60 mM NaCl plus 20 μM MT plus 0.4 mM EGTA. The concentrations of NaCl, MT, CaCl2, LaCl3 and EGTA were selected according to the previous experiment. Random samples of barley sprouts from different treatments were collected at 4 and 6 days after germination for biochemical examination. NaCl and CaCl2 were procured from Macklin (Shanghai, China), MT, LaCl3 and EGTA were procured from Sigma-Aldrich Co. (St. Louis, USA). All other chemicals and reagents used were analytical grade.
2.2 Sprout length and fresh weight
According to Yin et al. (2022b),9 a sample of thirty sprouts were selected to measure sprout length and fresh weight.
2.3 Total phenolic acid
The Folin-technique Ciocalteu's was used to calculate the total phenolic acid content.3
2.4 Intracellular free calcium, H2O2 and O2˙−
The intracellular free calcium, H2O2 and O2˙− fluorescence staining of 4 day-old germinated barley according to the method of Yin et al.9
2.5 Total calcium
According to the method of Callicott et al.21 determined the total calcium content.
2.6 Antioxidant enzyme activity
Superoxide dismutase (SOD) activity was measured as described by Kochs et al.22 Catalase (CAT) and peroxidase (POD) activities were measured as described by Wang et al.23
2.7 Phenylpropanoid metabolism-related enzyme activity
Phenylalanine ammonia lyase (PAL) and cinnamic acid 4-hydroxylase (C4H) activities were determined according to the method of Ma et al.24
2.8 Gene expression assay
Total RNA extraction, reverse transcription, and fluorescence quantitative PCR analysis refer to the method of Yin et al.25 The primers used were listed in ESI Table S1.† Using the 2−ΔΔCt technique, relative gene expression levels were determined.
2.9 Statistical analysis
The data were all reported as mean ± standard deviation, and each experiment was run in triplicate. Using Tukey's multiple-range test, variables from three replicates were compared, and a p-value of 0.05 was deemed significant.
3. Results
3.1 The total phenolic acid content of barley sprouts
Decreasing phenolic acid content in barley sprouts with increasing germination time (Fig. 1). Compared with NaCl stress, at the same germination time, exogenous MT, CaCl2 and the combination of both significantly increased phenolic acid content in sprout (p < 0.05), and the MT–CaCl2 treatment resulted in the greatest phenolic acid content, 1.13 and 1.11 times that of the NaCl–MT treatment. However, the addition of LaCl3 and EGTA reversed MT-induced phenolic acid synthesis. The above results suggest that exogenous MT was used to increase the total phenolic acid content of barley sprouts through the synthesis of the Ca2+ pathway.
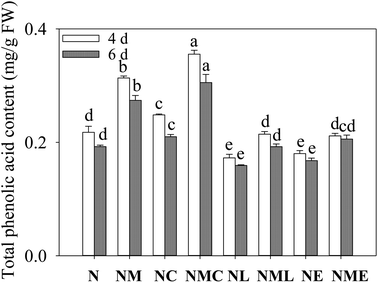 |
| Fig. 1 Effect of MT–Ca2+ on the total phenolic acid content of barley sprout under NaCl stress. Different letters indicate the significant differences in indicators among treatments at the same germination time using Tukey's test (p < 0.05). N: NaCl; NM: NaCl + MT; NC: NaCl + CaCl2; NMC: NaCl + MT + CaCl2; NL: NaCl + LaCl3; NML: NaCl + MT + LaCl3; NE: NaCl + EGTA; (8) NME: NaCl + MT + EGTA. | |
3.2 The physiology and biochemistry of barley sprouts
Exogenous MT, CaCl2 and MT–CaCl2 promoted the length and fresh weight of barley sprouts under NaCl stress at the same growth time (Fig. 2I–III, p < 0.05). EGTA also promoted sprouts length of barley under NaCl stress, however, the addition of LaCl3 not only inhibited the physiological status (Fig. 2I–III) of barley sprouts under NaCl stress but also reversed the alleviating effect of NaCl–MT treatment.
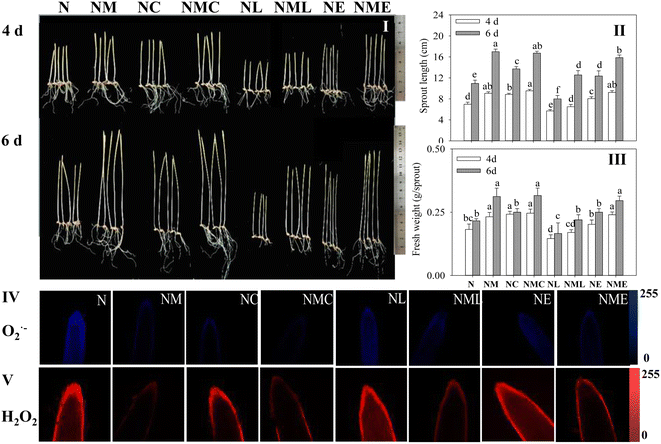 |
| Fig. 2 Effects of MT–Ca2+ on the (I) growth performance, (II) sprout length, (III) fresh weight, and root tip staining of (IV) O2˙− and (V) H2O2 of barley sprout under NaCl stress. The scale length is 100 μm. Different letters indicate the significant differences in indicators among treatments at the same germination time using Tukey's test (p < 0.05). N: NaCl; NM: NaCl + MT; NC: NaCl + CaCl2; NMC: NaCl + MT + CaCl2; NL: NaCl + LaCl3; NML: NaCl + MT + LaCl3; NE: NaCl + EGTA; (8) NME: NaCl + MT + EGTA. | |
MT and MT–CaCl2 groups significantly attenuated blue (O2˙−) and red (H2O2) (Fig. 2IV and V) fluorescence intensities, as well as distributions, compared to NaCl treatment alone, which suggests that MT–CaCl2 reduces the extent of cell membrane damage. LaCl3 and EGTA dramatically increased the fluorescence intensity of H2O2 and O2˙− in the root tips of sprouts treated with NaCl combined with MT, indicating that the Ca2+ inhibitor enhanced the cumulation of reactive oxygen species (ROS) in the cells.
3.3 The antioxidant enzyme activity of barley sprouts
MT significantly increased the activity of antioxidant enzyme in sprouts under NaCl treatment (Fig. 3I–III), and the activities of POD, SOD and CAT increased by 37%, 69.3% and 97.5% on the fourth day, and 34.3%, 72.4% and 114.4% on the sixth day. CaCl2 addition significantly increased POD activity by 10.7% and 10.2% compared to NaCl–MT treatment (Fig. 3I). However, the addition of LaCl3 and EGTA reversed the effects of MT-induced salt stress on POD, SOD and CAT activities in barley, and the inhibitory effect of LaCl3 was greater than that of EGTA.
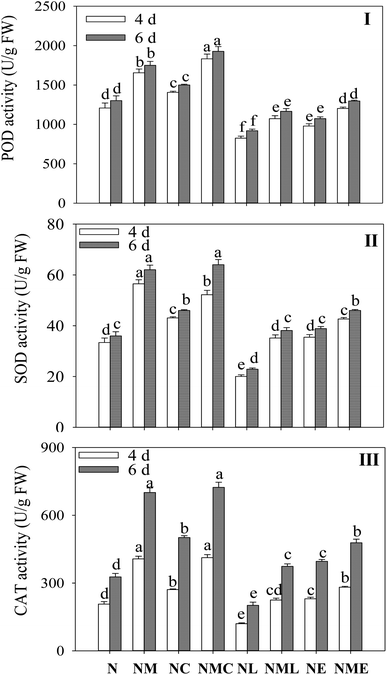 |
| Fig. 3 Effects of MT–Ca2+ on the activities of (I) POD, (II) SOD and (III) CAT of barley sprout under NaCl stress. Different letters indicate the significant differences in indicators among treatments at the same germination time using Tukey's test (p < 0.05). N: NaCl; NM: NaCl + MT; NC: NaCl + CaCl2; NMC: NaCl + MT + CaCl2; NL: NaCl + LaCl3; NML: NaCl + MT + LaCl3; NE: NaCl + EGTA; (8) NME: NaCl + MT + EGTA. | |
3.4 Effect of MT on Ca2+ metabolism of barley sprouts
The addition of exogenous MT considerably raised the total calcium concentration in the sprouts compared to the NaCl treatment alone (Fig. 4I, p < 0.05) and rose by 41.5% and 81.9%. Furthermore, the intensity of green (Ca2+) fluorescence in the root tip obviously increased (Fig. 4II). CaCl2 addition resulted in 2.05- and 1.56-fold higher total calcium content in sprouts than the NaCl plus MT treatment (Fig. 4I), as well as enhanced fluorescent staining (green) and expanded distribution of root tips (Fig. 4II). In contrast, EGTA and LaCl3 addition significantly inhibited the total calcium content in the sprouts, and the inhibition effect of LaCl3 was significantly greater than that of EGTA (Fig. 4I). The findings suggest that MT can cause Ca2+ spikes in cell root tips.
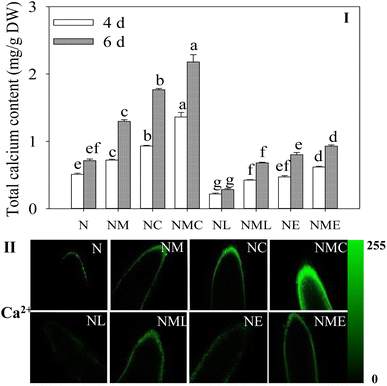 |
| Fig. 4 Effect of MT on Ca2+ metabolism of barley sprouts under NaCl stress. (I) Total calcium content and root tip staining of (II) intracellular free calcium. The scale length is 100 μm. Different letters indicate the significant differences in indicators among treatments at the same germination time using Tukey's test (p < 0.05). N: NaCl; NM: NaCl + MT; NC: NaCl + CaCl2; NMC: NaCl + MT + CaCl2; NL: NaCl + LaCl3; NML: NaCl + MT + LaCl3; NE: NaCl + EGTA; (8) NME: NaCl + MT + EGTA. | |
3.5 The expression of calcium target protein genes of barley sprouts
Exogenous MT substantially increased CaMT expression in 6 day-old barley sprouts exposed to NaCl (p < 0.05), and this increase was 2.43 times more than that of NaCl treatment (Fig. 5V). In 6 day-old barley sprouts, CaCl2 addition significantly induces the levels of Ca2+-ATP, CaMK1, CaMK2 and CDPK (Fig. 5I–IV, p < 0.05), which was 2.9-, 3.6-, 12.1- and 13-fold greater than that of the NaCl–MT treatment, respectively. Similarly, the addition of EGTA and LaCl3 significantly up-regulated the expression of Ca2+-ATP, CaMK1, CaMK2 and CDPK on the sixth day compared to the NaCl plus MT treatment (p < 0.05).
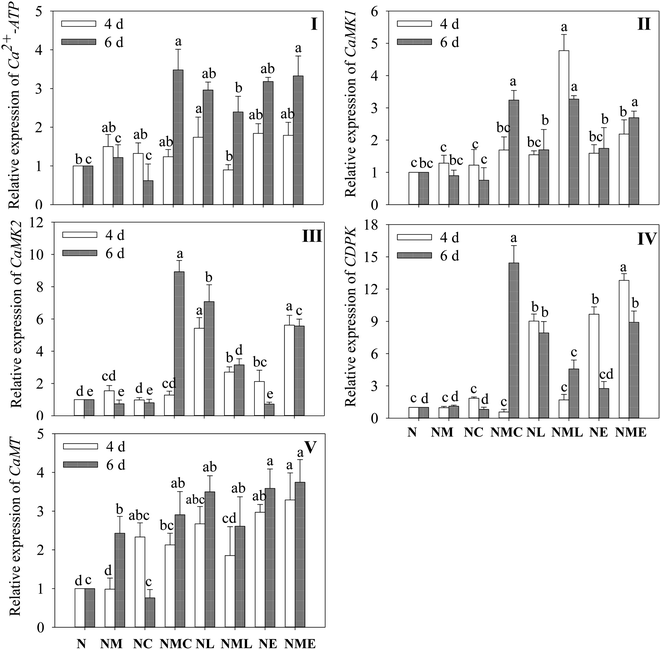 |
| Fig. 5 Effect of MT–Ca2+ on the gene expression of Ca2+-ATP (I), CaMK1 (II), CaMK2 (III), CDPK (IV), and CaMT (V) of barley sprouts. The gene expression in germinating barley treated with NaCl was used as the control. Different letters indicate the significant differences in indicators among treatments at the same germination time using Tukey's test (p < 0.05). N: NaCl; NM: NaCl + MT; NC: NaCl + CaCl2; NMC: NaCl + MT + CaCl2; NL: NaCl + LaCl3; NML: NaCl + MT + LaCl3; NE: NaCl + EGTA; (8) NME: NaCl + MT + EGTA. | |
3.6 The expression of phenolic acid metabolizing enzymes and related genes of barley sprouts
As shown in Fig. 6I and II, exogenous MT, CaCl2 and the combination of both markedly enhanced the activity of PAL and C4H in wheat seedlings under NaCl stress (p < 0.05). At 4 d of NaCl stress, PAL and C4H activities in sprouts under MT–CaCl2 treatment were the highest, 1.23 and 1.19 times higher than those in the MT-treated group. The stimulatory effect of MT was markedly reversed under NaCl stress by applying the LaCl3 and EGTA (p < 0.05).
 |
| Fig. 6 Effects of MT–Ca2+ on activity of PAL (I) and C4H (II) and gene expression of PAL (III), 4CL (IV), C4H (V), C3H (VI), F5H (VII), and COMT (VIII) in barley sprouts. The gene expression in germinating barley treated with NaCl was used as the control. Different letters indicate the significant differences in indicators among treatments at the same germination time using Tukey's test (p < 0.05). N: NaCl; NM: NaCl + MT; NC: NaCl + CaCl2; NMC: NaCl + MT + CaCl2; NL: NaCl + LaCl3; NML: NaCl + MT + LaCl3; NE: NaCl + EGTA; (8) NME: NaCl + MT + EGTA. | |
To deeply investigate the mechanism of MT-induced phenolic acid synthesis, we analyzed the gene expression of key enzymes in the phenolic acid synthesis pathway (Fig. 6III–VIII). According to Fig. 6, compared with NaCl treatment, MT addition substantially up-regulated the expressions of PAL, C4H and 4CL. Additionally, the NaCl–MT treatment significantly increased the expression of C3H and COMT in sprouts that were 6 days old (p < 0.05). The application of CaCl2 significantly up-regulated the expression of PAL (Fig. 6III) on germination 4 d and F5H (Fig. 6VII) on germination 6 d, and which was 2.1 and 29.3 times higher than that of NaCl combined with MT treatment, respectively. However, compared with NaCl combined with MT treatment, the addition of EGTA significantly up-regulated the expression of F5H, but did not affect the expression of C4H, C3H, and 4CL. In contrast, LaCl3 treatment suppressed the expression of C4H as well as PAL, C3H, 4CL and COMT for 6 days of germination. The above results indicated that CaCl2 and MT promoted the synthesis of phenolic acids by enhancing the metabolic activity of key enzymes and the expression levels of enzyme genes in barley sprouts under NaCl stress through the interaction relationship.
4. Discussion
Barley is considered a good food material for the enrichment of phenolic acids. However, abiotic stress, although able to increase phenolic acids in germinated barley, can inhibit the growth and development.4 Exogenous MT, a novel plant regulator, participates in the biosynthesis of secondary metabolites in plants and is efficient in reducing the negative effects of abiotic stress on them.7,8 Exogenous MT is not only effective in alleviating the growth inhibition of barleys by NaCl stress, but also further promotes phenolic acid biosynthesis under salt stress, according to prior research.10 However, the mechanisms by which MT regulates phenolic acid metabolism and NaCl tolerance in barleys are still unknown. Ca2+, an essential messenger of plant signaling,26 has been shown to interact with MT to activate antioxidant enzyme systems, reduce ROS, and promote secondary metabolite anabolism.27 However, studies on the control of phenolic acid metabolism in barley sprouts by MT–Ca2+ interaction under NaCl stress are few.
Ca2+ is a widely distributed secondary messenger in plant signaling networks.28 Plants' ability to regulate their reactions to their environment and adapt to it depends heavily on the concentration and redistribution of Ca2+. The findings of the current investigation shown that, in comparison to NaCl treatment, the addition of exogenous MT considerably raised Ca2+ fluorescence intensity and increased the total calcium content in cells (Fig. 4I), indicating that MT not only induced Ca2+ relocalization and distribution in root tip cells but also promoted the release of Ca2+ from root tips. We also found that the inhibitory effect of LaCl3 on total calcium was significantly greater than that of EGTA, indicating that Ca2+ transport by MT under NaCl stress was mainly via calcium channels. Environmental stresses induce Ca2+ with different amplitude, temporal and spatial distribution, producing so-called “Ca2+ signaling” with different characteristics. In addition, Ca2+ and CaM can interact in plants to create Ca2+–CaM complexes.29 The addition of CaCl2 in this study significantly increased the relative gene expression of CaMK1, CaMK2, CDPK, and Ca2+-ATP under MT treatment (Fig. 5). Through the activation of flavonoid biosynthesis genes, the Ca2+ signaling pathway has also been demonstrated to be involved in anthocyanin production.30 Salicylic acid-induced31 and abscisic acid32 secondary metabolite synthesis are likewise controlled by the Ca2+ signaling. In the current investigation, CaCl2 addition further greatly enhanced the phenolic acid content in MT-treated seedlings, while the addition of calcium antagonists (LaCl3 and EGTA) completely reversed it (Fig. 1). These findings imply that MT most likely to regulates the amount of extracellular Ca2+ that enters the plasma membrane, thereby increasing the cytosolic Ca2+ level and inducing Ca2+ signaling, further regulating phenolic acid anabolism. To our knowledge, there aren't many research on how MT and Ca2+ interactions affect the synthesis of phenolic chemicals. However, consistent with our hypothesis, methyl jasmonate33 and cytokinin34 have all been reported to promote alkaloid synthesis by increasing cytosolic Ca2+ and thus alkaloid synthesis in cell culture in vitro. Meanwhile, study by Vafadar et al.19 has demonstrated that extracellular Ca2+ influx and Ca2+/CaM complex formation are necessary for the MT-induced accumulation of phenolic compounds in plants.
The accumulation of phenolic acid is closely related to the activity and relative gene expression levels of major enzymes involved in the pathway of phenolic acid synthesis. The activity of PAL, a rate-limiting enzyme for the production of phenols in plants, is correlated with the concentration of phenols.35 The results showed that both exogenous MT alone and its combined CaCl2 treatment significantly enhanced PAL and C4H activity and the corresponding phenolic acid content in NaCl-stressed barley. In contrast, the addition of LaCl3 and EGTA inhibited PAL and C4H activity and reduced phenolic acid enrichment. This suggests that during phenolic acid synthesis in barley seedlings, Ca2+ may act downstream of MT to increase metabolic enzyme activity and thus induce phenolic acid accumulation. This agrees with the findings of Vafadar et al.19 that exogenous MT enhances PAL activity through modulation of Ca2+ signaling and ultimately increases phenolics in salt-stressed Dracocephalum kotschyi. Additionally, the addition of CaCl2 dramatically up-regulated the expression levels of related phenolic acid synthesis genes under NaCl combined with MT treatment. Exogenous MT and CaCl2 promoted phenolic acid enrichment under NaCl stress by inducing expression levels of PAL, COMT, 4CL, and C3H in 6 day-old barley. This is consistent with the findings reported in the literature that exogenous Ca2+ enhanced the expression of PAL and thus the phenolic content of wheat plants in a saline environment.36 It is shown that under NaCl stress, MT acts on Ca2+ signaling to induce the activity (PAL and C4H) and gene expression levels (PAL, C3H, 4CL, and F5H) of enzymes involved in the phenolic acid metabolic pathway, thereby promoting phenolic acid accumulation.
Plants accumulate large amounts of ROS in the body under abiotic stress,37 which can induce a ROS scavenging system of enzymatic antioxidants in plants. Studies have demonstrated the ability of MT to modulate multiple antioxidant enzyme activities through the ROS scavenging system composed of enzymatic antioxidants and improve plant tolerance to a variety of environmental stresses.38 The results demonstrated that MT greatly increased POD, SOD, and CAT activities in sprouts (Fig. 3). The addition of exogenous CaCl2 further increased antioxidant enzyme activities in seedlings, compared with NaCl combined MT treatment, which was inhibited by adding LaCl3 and EGTA. Furthermore, exogenous MT significantly reduced the H2O2 and O2˙− fluorescence intensity in barley root tips under NaCl stress, compared with NaCl stress, and CaCl2 addition further reduced the fluorescence intensity (Fig. 2). Previous studies reported that co-treatment of MT and CaCl2 increased the antioxidant enzyme system and decreased the accumulation of ROS in low temperature-stressed cotton39 and drought-stressed Dalbergia odorifera,40 respectively.
Based on the above results, Fig. 7 shows a possible molecular mechanism by which MT–Ca2+ affects the enhancement of phenolic acid accumulation and growth, and we conclude that (1) exogenous MT induced Ca2+ influx and expression levels of calmodulin-related genes. (2) Ca2+ plays a critical part in MT regulation of activities and gene expression levels of the key enzyme in the phenolic acid synthesis pathway, leading to phenolic acid enrichment. (3) Ca2+ is involved in MT activation of antioxidant enzyme systems and maintenance of ROS homeostasis, thus improving barley growth. However, further in-depth studies are needed to confirm the details of Ca2+ signaling involvement in MT regulates phenolic acid biosynthesis and improves growth.
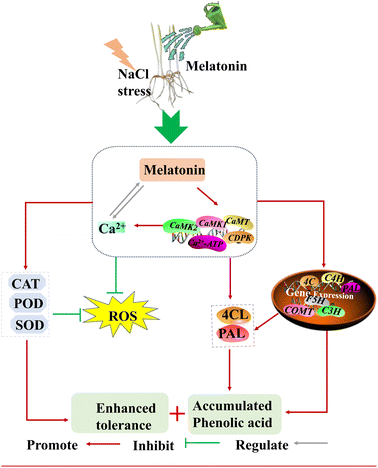 |
| Fig. 7 A simulation of how combined MT and Ca2+ affected the phenolic acid biosynthesis and enhanced tolerance in barley under NaCl stress. CAT: catalase; SOD: superoxide dismutase; POD: peroxidase; PAL: phenylalanine ammonia lyase; 4CL: 4-coumarate-CoA ligase; ROS: reactive oxygen species. | |
Author contributions
Xin Tian: performed experiments and drafted manuscript. Xudong He: reviewed writing, Jinpeng Xu: performed experiments. Zhengfei Yang: reviewed writing. Weiming Fang: reviewed writing. Yongqi Yin: designed study and wrote manuscript.
Conflicts of interest
The authors declare no conflict of interest.
Acknowledgements
The project was financed by China Postdoctoral Science Foundation (2019M651978).
References
- E. Idehen, Y. Tang and S. Sang, Bioactive phytochemicals in barley, J. Food Drug Anal., 2017, 25, 148–161 CrossRef CAS PubMed.
- A. Bento-Silva, V. M. Koistinen, P. Mena, M. R. Bronze, K. Hanhineva, S. Sahlstrm, V. Kitryt, S. Moco and A. M. Aura, Factors affecting intake, metabolism and health benefits of phenolic acids: do we understand individual variability?, Eur. J. Nutr., 2020, 9, 1275–1293 CrossRef PubMed.
- Y. Ma, P. Wang, Z. Chen, Z. Gu and R. Yang, NaCl stress on physio-biochemical metabolism and antioxidant capacity in germinated hulless barley (Hordeum vulgare L.), J. Sci. Food Agric., 2019, 99, 1755–1764 CrossRef CAS PubMed.
- D. Ma, C. Wang, J. Feng and B. Xu, Wheat grain phenolics: a review on composition, bioactivity, and influencing factors, J. Sci. Food Agric., 2021, 101, 6167–6185 CrossRef CAS PubMed.
- C. Sun, L. Liu, L. Wang, B. Li, C. Jin and X. Lin, Melatonin: a master regulator of plant development and stress responses, J. Integr. Plant Biol., 2021, 63, 126–145 CrossRef CAS PubMed.
- T. A. Khan, Q. Fariduddin, F. Nazir and M. Saleem, Melatonin in business with abiotic stresses in plants, Physiol. Mol. Biol. Plants, 2020, 26, 1931–1944 CrossRef CAS PubMed.
- M. Jafari and A. Shahsavar, The effect of foliar application of melatonin on changes in secondary metabolite contents in two citrus species under drought stress conditions, Front. Plant Sci., 2021, 12, 692735 CrossRef PubMed.
- Q. Sun, L. Liu, L. Zhang, H. Lv, Q. He, L. Guo, X. Zhang, H. He, S. Ren, N. Zhang, B. Zhao and Y. D. Guo, Melatonin promotes carotenoid biosynthesis in an ethylene-dependent manner in tomato fruits, Plant Sci., 2020, 298, 110580 CrossRef CAS PubMed.
- Y. Yin, X. Tian, X. He, J. Yang, Z. Yang and W. Fang, Exogenous melatonin stimulated isoflavone biosynthesis in NaCl-stressed germinating soybean (Glycine max L.), Plant Physiol. Biochem., 2022, 185, 123–131 CrossRef CAS PubMed.
- Y. Yin, J. Xu, X. He, Z. Yang, W. Fang and J. Tao, Role of exogenous melatonin involved in phenolic acid metabolism of germinated hulless barley under NaCl stress, Plant Physiol. Biochem., 2022, 170, 14–22 CrossRef CAS PubMed.
- X. Yang, J. Chen, Y. Ma, M. Huang, T. Qiu, H. Bian, N. Han and J. Wang, Function, mechanism, and application of plant melatonin: an update with a focus on the cereal crop, Barley (Hordeum vulgare L.), Antioxidants, 2022, 11, 634 CrossRef CAS PubMed.
- W. Hu, W. Tie, W. Ou, Y. Yan, H. Kong, J. Zuo, X. Ding, Z. Ding, Y. Liu and C. Wu, Crosstalk between calcium and melatonin affects postharvest physiological deterioration and quality loss in cassava, Postharvest Biol. Technol., 2018, 140, 42–49 CrossRef CAS.
- P. Manishankar, N. Wang, P. Koster, A. A. Alatar and J. Kudla, Calcium signaling during salt stress and in the regulation of ion homeostasis, J. Exp. Bot., 2018, 17, 1–17 Search PubMed.
- R. Gollop, S. Even, V. Colova-Tsolova and A. Perl, Expression of the grape dihydroflavonol reductase gene and analysis of its promoter region, J. Exp. Bot., 2002, 53, 1397–1409 CAS.
- J. Liu, S. Shabala, J. Zhang, G. Ma, D. Chen, L. Shabala, F. Zeng, Z. H. Chen, M. Zhou, G. Venkataraman and Q. Zhao, Melatonin improves rice salinity stress tolerance by NADPH oxidase-dependent control of the plasma membrane K+ transporters and K+ homeostasis, Plant, Cell Environ., 2020, 43, 2591–2605 CrossRef CAS PubMed.
- F. Vafadar, R. Amooaghaie, P. Ehsanzadeh, F. Ghanati and R. H. Sajedi, Crosstalk between melatonin and Ca2+/CaM evokes systemic salt tolerance in Dracocephalum kotschyi, J. Plant Physiol., 2020, 252, 153237 CrossRef CAS PubMed.
- F. Vafadar, R. Amooaghaie, P. Ehsanzadeh, F. Ghanati and S. Allakhverdiev, Melatonin improves the photosynthesis in Dracocephalum kotschyi under salinity stress in a Ca2+/CaM-dependent manner, Funct. Plant Biol., 2021, 49, 89–101 CrossRef PubMed.
- M. H. Siddiqui, S. Alamri, M. N. Khan, F. J. Corpas, A. A. Al-Amri, Q. D. Alsubaie, H. M. Ali, H. M. Kalaji and P. Ahmad, Melatonin and calcium function synergistically to promote the resilience through ROS metabolism under arsenic-induced stress, J. Hazard. Mater., 2020, 398, 122882 CrossRef CAS PubMed.
- F. Vafadar, R. Amooaghaie, P. Ehsanzadeh, M. Ghanadian, M. Talebi and F. Ghanati, Melatonin and calcium modulate the production of rosmarinic acid, luteolin, and apigenin in Dracocephalum kotschyi under salinity stress, Phytochemistry, 2020, 177, 112422 CrossRef CAS PubMed.
- J. Cui, C. Yu, D.-B. Zhong, Y. Zhao and X. Yu, Melatonin and calcium act synergistically to enhance the coproduction of astaxanthin and lipids in Haematococcus pluvialis under nitrogen deficiency and high light conditions, Bioresour. Technol., 2020, 305, 123069 CrossRef CAS PubMed.
- R. H. Callicott and P. W. Carr, Concurrent determination of total serum calcium and magnesium by thermometric titration with ethylenediaminetetraacetate, Clin. Chem., 1976, 22, 1084–1088 CrossRef CAS.
- G. Kochs and H. Grisebach, Enzymic synthesis of isoflavones, Eur. J. Biochem., 1986, 155, 311–318 CrossRef CAS PubMed.
- Y. S. Wang, S. P. Tian and Y. Xu, Effects of high oxygen concentration on pro- and anti-oxidant enzymes in peach fruits during postharvest periods, Food Chem., 2005, 91, 99–104 CrossRef CAS.
- Y. Ma, P. Wang, T. Zhou, Z. J. Chen, Z. X. Gu and R. Q. Yang, Role of Ca2+ in phenolic compound metabolism of barley (Hordeum vulgare L.) sprouts under NaCl stress, J. Sci. Food Agric., 2019, 99, 5176–5186 CrossRef CAS PubMed.
- Y. Yin, X. Tian, J. Yang, Z. Yang, J. Tao and W. Fang, Melatonin mediates isoflavone accumulation in germinated soybeans (Glycine max L.) under ultraviolet-B stress, Plant Physiol. Biochem., 2022, 175, 23–32 CrossRef CAS PubMed.
- H. Ren, X. Zhao, W. Li, J. Hussain, G. Qi and S. Liu, Calcium signaling in plant programmed cell death, Cells, 2021, 10, 1089 CrossRef CAS PubMed.
- R. Sharif, C. Xie, H. Zhang, M. B. Arnao, M. Ali, Q. Ali, I. Muhammad, A. Shalmani, M. A. Nawaz, P. Chen and Y. Li, Melatonin and its effects on plant systems, Molecules, 2018, 23, 1–10 Search PubMed.
- X. Wang, C. Feng, L. Tian, C. Hou, W. Tian, B. Hu, Q. Zhang, Z. Ren, Q. Niu, J. Song, D. Kong, L. Liu, Y. He, L. Ma, C. Chu, S. Luan and L. Li, A transceptor-channel complex couples nitrate sensing to calcium signaling in Arabidopsis, Mol. Plant, 2021, 14, 774–786 CrossRef CAS PubMed.
- H. Frohnmeyer, L. Loyall, M. R. Blatt and A. Grabov, Millisecond UV-B irradiation evokes prolonged elevation of cytosolic-free Ca2+ and stimulates gene expression in transgenic parsley cell cultures, Plant J., 1999, 20, 109–117 CrossRef CAS PubMed.
- W. P. Xu, H. Peng, T. B. Yang, B. Whitaker, L. H. Huang, J. H. Sun and P. Chen, Effect of calcium on strawberry fruit flavonoid pathway gene expression and anthocyanin accumulation, Plant Physiol. Biochem., 2014, 82, 289–298 CrossRef CAS PubMed.
- H. B. Guo, N. Zhu, M. K. Deyholos, J. Liu, X. R. Zhang and J. E. Dong, Calcium mobilization in salicylic acid-induced salvia miltiorrhiza cell cultures and its effect on the accumulation of rosmarinic acid, Appl. Biochem. Biotechnol., 2015, 175, 2689–2702 CrossRef CAS PubMed.
- I. L. Vighi, R. L. Crizel, E. C. Perin, C. V. Rombaldi and V. Galli, Crosstalk during fruit ripening and stress response among abscisic acid, calcium-dependent protein kinase and phenylpropanoid, Crit. Rev. Plant Sci., 2019, 38, 99–116 CrossRef CAS.
- C. W. Lee-Parsons and S. Ertürk, Ajmalicine production in methyl jasmonate-induced Catharanthus roseus cell cultures depends on Ca2+ level, Plant Cell Rep., 2005, 24, 677–682 CrossRef CAS PubMed.
- J.-M. Mérillon, D. LIU, F. Huguet, J.-C. Chénieux and M. Rideau, Effects of calcium entry blockers and calmodulin inhibitors on cytokinin-enhanced alkaloid accumulation in Catharanthus roseus cell cultures, Plant Physiol. Biochem., 1991, 29, 289–296 Search PubMed.
- H. Yu, D. Li, D. Yang, Z. Xue, J. Li, B. Xing, K. Yan, R. Han and Z. Liang, SmKFB5 protein regulates phenolic acid biosynthesis by controlling the degradation of phenylalanine ammonia-lyase in Salvia miltiorrhiza, J. Exp. Bot., 2021, 72, 4915–4929 CrossRef CAS PubMed.
- B. Zaman, B. Niazi, M. Athar and M. Ahmad, Response of wheat plants to sodium and calcium ion interaction under saline environment, Int. J. Environ. Sci. Technol., 2005, 2, 7–12 CrossRef CAS.
- E. Medina, S. H. Kim, M. Yun and W. G. Choi, Recapitulation of the function and role of ROS generated in response to heat stress in plants, Plants, 2021, 10, 371–384 CrossRef CAS PubMed.
- M. Moustafa-Farag, A. Almoneafy, A. Mahmoud, A. Elkelish, M. B. Arnao, L. Li and S. Ai, Melatonin and its protective role against biotic stress impacts on plants, Biomolecules, 2019, 10, 54–64 CrossRef PubMed.
- Y. Zhang, Y. Fan, C. Rui, H. Zhang, N. Xu, M. Dai, X. Chen, X. Lu, D. Wang, J. Wang, J. Wang, Q. Wang, S. Wang, C. Chen, L. Guo, L. Zhao and W. Ye, Melatonin improves cotton salt tolerance by regulating ROS scavenging system and Ca2+ signal transduction, Front. Plant Sci., 2021, 12, 693690 CrossRef PubMed.
- E.-H. M. Cisse, L.-J. Zhang, Y.-J. Pu, L.-F. Miao, D.-D. Li, J. Zhang and F. Yang, Exogenous Ca2+ associated with melatonin alleviates drought-induced damage in the woody tree Dalbergia odorifera, J. Plant Growth Regul., 2021, 40, 1–16 CrossRef.
|
This journal is © The Royal Society of Chemistry 2022 |
Click here to see how this site uses Cookies. View our privacy policy here.