DOI:
10.1039/D2RA02389J
(Review Article)
RSC Adv., 2022,
12, 29197-29213
Antimicrobial activities and mechanisms of extract and components of herbs in East Asia
Received
13th April 2022
, Accepted 3rd October 2022
First published on 12th October 2022
Abstract
Antibacterial drugs face increasing challenges due to drug resistance and adverse reactions, which has created a pressing need for the discovery and development of novel antibacterial drugs. Herbs have played an important role in the treatment of infectious diseases. This review aims to summarize, analyze and evaluate the antibacterial activities and mechanisms of components from popular herbs in East Asia. In this review, we have searched and summarized the scientific papers published during the past twenty-year period from electronic databases such as PubMed, ScienceDirect, and Web of Science. These herbs and their components, including alkaloids, flavonoids, essential oils, terpenes, organic acids, coumarins and lignans, display potential antimicrobial effects. Herbal medicine formulas (HMFs) usually show stronger antibacterial activity than single herbs. Herbs and HMFs bring forth antibacterial activities by damaging cell membranes and walls, inhibiting nucleic acid and protein synthesis, and increasing intracellular osmotic pressure. These herbs and their components can be developed as potential and promising novel antibacterial herbal products.
Introduction
Antimicrobial drugs derived from microbial or chemical products play important roles in the fight against pathogens. However, with wide usage around the world, they have resulted in widespread drug resistance and side effects. For example, a sharp increase in the proportion and absolute number of bacteria resistant to various chemical antibacterial agents has occurred over the past decade.1 Five-sixths of WHO regions have reported international drug resistance rates of 3rd gen. cephalosporins against Escherichia coli above 50 percent.2 The increase in untreatable infections caused by the rapid emergence of multi-drug resistant and pan-drug-resistant bacteria necessitate the discovery and development of new antibacterial agents. However, the development of new antimicrobial drugs is becoming more and more difficult and costly.
Herbs have been widely used to treat bacterial infections thousands of years ago owing to multicomponent synergistic antibacterial activity. At present, 65% to 80% of people in developing countries use botanical drugs for antimicrobial treatment.3 For example, about 900 years ago, the traditional Chinese medicine (TCM) Coptis chinensis Franch. was used to treat acute bacillary dysentery. Herb-derived products have historically been crucial in the development of antibacterial agents. Berberine extracted from Coptis chinensis Franch., Phellodendri chinensis C.K.Schneid. and other herbs shows significant effects against intestinal bacterial infection, and has been developed as an antibacterial agent. Tanshinone, an extract of Salvia miltiorrhiza Bunge, was developed into an oral drug for acne caused by Cutibacterium acnes. Compared with chemical antimicrobial products, herbs display less drug resistance, fewer side effects as well as reversal of antibiotic resistance when combined with antibiotics.
There have been extensive reports on antimicrobial activity, as well as the spectrum and mechanisms of action of antibacterial herbal components. The scientific papers cited in this review were extracted from the electronic databases such as PubMed, ScienceDirect, Web of Science, EBSCO OVID and Wiley Online Library. The terms used to perform the searches involved in keywords including “antimicrobial activities”, “antibacterial mechanism”, “antibacterial components”, “herbs” or “herbal medicine formulas”. “AND” or “OR” operators were used depending on the combination of terms. Through identifying and summarizing a large number of contributions already reported in the past two decades, this review illustrates pharmacodynamic substance basis and antibacterial effects of herbs in East Asia, and antibacterial mechanisms of their phytochemicals (e.g., berberine, matrine, baicalein, galangin, pogostone, apigenin, oridonin, cynaroside, 3,5-di-O-caffeoylquinic acid, 4,5-di-O-caffeoylquinic acid, (+)-pinoresinol, lariciresinol, (−)-olivil-9-O-β-D-glucopyranoside, glochidioboside, and (+)-medioresinol) so as to provide new ideas for the discovery, development and application of antibacterial herbs.
Antibacterial herbs and their components
Alkaloids
Alkaloids are commonly found in plants with complex and diverse structures. Alkaloids comprise a group of nitrogen-containing organic compounds that possess significant antimicrobial effects. Alkaloids are one of the most important active ingredients in herbs. Furthermore, they have inspired the development of several antimicrobial drugs, such as synthesis of quinine to quinolones and the structural alteration from azomycin to metronidazole.4
Coptis chinensis Franch. The dried rhizome of Coptis chinensis Franch is often used to treat vomiting, diarrhoea, high fever and jaundice.5 Berberine (Fig. 1), an isoquinoline alkaloid, is isolated from Coptis chinensis Franch and other herbs. Berberine is a NorA substrate that accumulates in bacterial cells, leading to DNA damage by binding both single- and double-stranded DNA.6 Berberine is a substrate of multi-drug resistance (MDR) efflux pumps for Gram-negative bacteria. And MDR inhibitors can remarkably increase the antibacterial efficacy of berberine.7 The activity of berberine against Gram-positive bacteria occurred primarily through the cell division protein Filamenting temperature-sensitive mutant Z (FtsZ).8 Berberine hydrochloride exhibited moderate to strong activity against S. aureus, E. coli, P. aeruginosa9 and multidrug resistant E. coli.10 It displays antifungal effects against four pathogenic dermatophytes, Trichophyton mentagrophytes, Trichophyton rubrum, Microsporum canis, and Microsporum gypseum.11 It affects the integrity of C. albicans cell wall, leading to mitochondrial dysfunction in fungi, which further results in increased reactive oxygen species and upregulation of oxidative stress.12
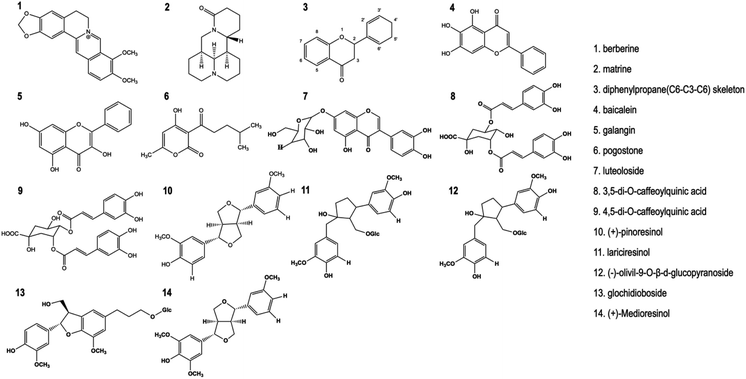 |
| Fig. 1 Chemical structure of antimicrobial herbal components. | |
Apart from berberine, magnoflorine is also a quaternary aporphine alkaloid derived from Coptis chinensis Franch. Magnoflorine exhibited an inhibitory effect against Candida strains with a minimum inhibitory concentration (MIC) of 50 μg mL−1.13 Magnoflorine could cause damage of cell wall of Candida albicans by inhibiting α-glucosidase activity. And it could inhibit the biofilm formation of C. albicans. Most toxicity studies have indicated that magnoflorine is not toxic to most cells.14
Coptis chinensis Franch exhibited strong antimicrobial activity, but its toxicity cannot be ignored. Some studies15 indicated that the toxic constituents of Coptis chinensis Franch are alkaloids, e.g., berberine. The Coptis chinensis Franch extract rich in alkaloid was more toxic than its total extract of.15 The medial lethal dose (LD50) of berberine, coptisine, palmatine and epiberberine derived from Coptis chinensis Franch, were determined as 713.57, 852.12, 1533.68 and 1360 mg kg−1, respectively. And berberine showed the highest cytotoxicity toward HepG2 and 3T3-L1 cells among the four alkaloids, while palmatine showed the lowest.16
It was reported that berberine had an inhibitory effect on human eag-related gene (hERG) channel, resulting in a long QT syndrome, which was the main cause of sudden death.17 Besides, the alkaloids in Coptis chinensis Franch exhibited a strong inhibitory effect on acetylcholinesterase (AChE).18,19 Furthermore, Berberine caused mitochondrial dysfunction, which may be associated to organ toxicity.20 These studies indicates that the toxic mechanisms of alkaloids from Coptis chinensis Franch may be complex. And further study on toxicity of alkaloids is clearly necessary.
Flavonoids
Flavonoids are a group of plant polyphenols commonly found in plants and are extensively used in traditional herbal medicine. Most flavonoids are structurally based on the parent compound, which has a diphenylpropane (C6–C3–C6) skeleton (Fig. 1). Flavonoids display antibacterial activity primarily due to the flavonoids–membrane interaction that is related to their chemical structure, particularly the number and positions of methoxyl and hydroxyl groups.21 Antimicrobial mechanism of flavonoids may include four aspects, i.e., cell membrane damage,22 inhibition of nucleic acid synthesis (caused by topoisomerase inhibition23), inhibition of energy metabolism (caused by NADH-cytochrome c reductase inhibition24) and inhibition of cell wall synthesis.25
Scutellaria baicalensis Georgi. Baicalein (5,6,7-trihydroxyflavone, Fig. 1) is an effective bactericide isolated from Scutellaria baicalensis Georgi, which is widely used as an herb to treat vomiting, nausea, diarrhoea and jaundice in Asia.26 Baicalein can cure bacterial infection by destroying its biofilms, inhibiting biofilm formation27 and protein synthesis, influencing bacterial membrane penetrability, and inhibiting the activities of succinate dehydrogenase, malate dehydrogenase and DNA topoisomerase I and II.28 Baicalein can enhance the effects of ampicillin and gentamicin against oral bacteria (fractional inhibitory concentration index, FICI < 0.375–0.5 and fractional bactericidal concentration index, FBCI < 0.5).29 Baicalein also exhibits synergy with ceftazidime against Streptococcus pyogenes30 and cefotaxime against K. pneumoniae.31 Baicalein inhibits the activity of penicillinase to enhance the antibacterial effects of penicillins in a dose-dependent manner.32 It significantly reverses the resistance of methicillin-resistant Staphylococcus aureus (MRSA) to ciprofloxacin by inhibiting the efflux pump of NorA in vitro.33 It reduces the production of alpha-hemolysin and staphylococcal enterotoxin A in S. aureus, inhibits biofilm formation, and downregulats the quorum sensing system regulators (agrA, RNAIII, and sarA in S. aureus) by inhibiting the transcription of quorum sensing-regulated genes and the translation of quorum sensing-signalling molecules.34 For fungi, baicalin promotes apoptosis in C. albicans by inhibiting the activity of succinate dehydrogenase and Ca2+–Mg2+ ATPase, increasing the concentration of cytoplasm Ca2+ and subsequently damaging the ultrastructure.35
Glycine max (L.) Merr. Soy isoflavones, which can be extracted from Glycine max (L.) Merr., inhibited proliferation of L. monocytogenes and E. coli.36 The structure of isoflavones may be a factor in determining their antibacterial efficacy. C-5 and C-7 hydroxyl groups were very important for anti-MRSA and anti-S. aureus activity, and the removal or rearrangement of the prenyl group at C-6 decreased antimicrobial activity.37 Dhayakaran et al.38 found that isoflavones might alter or prevent the movement of Listeria flagella to impede its adherence. Soy isoflavones might also prevent nucleic acid synthesis by affecting topoisomerase I and II or by inhibiting topoisomerase IV.39
Sophora flavescens Aiton. Cha et al.40 evaluated antibacterial activities of sophora flavanone G extracted from Sophora flavescens Aiton against 10 clinical isolates of methicillin-resistant S. aureus MRSA (MICs ranged from 0.5 to 8 μg mL−1). Tsuchiya and Iinuma41 suggested that sophora flavanone G exhibited antibacterial effects by reducing the fluidity of the outer and inner layers of cellular membranes. Besides, sophora flavanone B isolated from the roots of Desmodium caudatum (Thunb.) DC. exhibited antimicrobial activity against MRSA (MIC, 15.6–31.25 μg mL−1).42
Alpinia officinarum Hance. Galangin (Fig. 1) from Alpinia officinarum Hance inhibits sixteen 4-quinolone resistant S. aureus strains (MICs, 50 μg mL−1).43 There is no cross-resistance between 4-quinolones and galangin and the antibacterial mechanism of galangin may be related to the topoisomerase IV enzyme. Galangin inhibited S. aureus (MIC, 32 μg mL−1)44 and exhibited marked inhibitory activity against penicillinase and β-lactamase.45 This inhibitory activity is due to bacterial cell membrane damage by galangin, which might occur via three mechanisms: inhibition of protein synthesis, effects on penicillin-binding protein 2a and interaction with penicillinase.
Curcuma longa L. Curcumin (diferuloylmethane) is a natural polyphenolic flavonoid isolated from the rhizome of Curcuma longa L., which reduces the MICs of oxacillin, ampicillin, ciprofloxacin and norfloxacin against specific MRSA strains, and inhibits the growth of P. aeruginosa biofilms (MIC, 16 μg mL−1)46 and 65 H. pylori strains in vitro (MICs, 5–50 μg mL−1). Curcumin is an effective therapeutic agent against H. pylori infection due to inhibition of NF-κB activation and H. pylori-induced motogenic response. Curcumin induces kinks in the filaments of B. subtilis and E. coli, indicating that it inhibits bacterial cytokinesis. In addition, formation of the FtsZ and the activity of GTPase in bacteria are strongly inhibited by curcumin.47 Curcumin showed synergism with polymyxins in the treatment of bacterial infections and could ameliorated colistin-induced neurotoxicity and nephrotoxicity.48
Pogostemon cablin (Blanco) Benth. Pogostemon cablin (Blanco) Benth. is a valuable herbal medicine that is commonly used to treat colds, fever, vomiting, nausea and diarrhoea. Pogostone (Fig. 1) from Pogostemon cablin (Blanco) Benth. remarkably inhibits all C. albicans strains (MICs, 12–97 μg mL−1; minimal fungicidal concentration (MFC), 49–97 μg mL−1)49 and all fluconazole-resistant C. albicans strains (MICs, 3.1–50 μg mL−1).50 The antimicrobial activity is associated with the length and functional group of side chains from 3′ position of the pyranoid ketone ring. The functional groups, such as electron withdrawing groups, increase antibacterial activity of pogostone derivatives, while the electron donating group weakens the activity.51 Furthermore, antibacterial activity vanished when the terminal side chain was linked to phenyl benzene. Oral and topical administration of pogostone significantly reduced vaginal fungal load in a vulvovaginal candidiasis mice model. The excellent antibacterial effect observed in vivo was due to favorable oral absorption and bioavailability of pogostone.
Essential oils and terpenes
Most aromatic plants contain antibacterial ingredients composed of dozens of compounds, e.g., alcohols, ketones, aldehydes, phenols, ethers, and lipids.52 Terpenoid structures are very common in plant-derived essential oils and have been recognized to exhibit antimicrobial activities for decades. Because the antibacterial effects of essential oils are mediated by a complex coordination mechanism, the antibacterial mechanism remains unclear. The antimicrobial effects of essential oils may be relevant to cell membrane damage caused due their composition and cytotoxic effects.53 In fungal pathogens, essential oil forms membrane potential on cell wall, which destroys ATP assembly and leads to cell wall damage. Essential oil can also break down mitochondrial membrane and interfere with the electron transport system (ETS) pathway.52
Houttuynia cordata Thunb. The fresh or dried aerial portion of Houttuynia cordata Thunb. is often used to treat lung abscess, cough and skin infections.5 Methyl nonyl ketone, β-myrcene and bornyl acetate are the most abundant components in Houttuynia cordata Thunb essential oil. The essential oil from the above-ground portion of the plant exhibited strong activity against S. aureus (MIC, 0.25 μL mL−1) and Sarcina ureae (MIC, 0.0625 μL mL−1).54 Additional essential oils from different anatomical regions and species exhibited antibacterial effects as well (MICs, 0.0625 to 4.0 μL mL−1). In vivo experiments showed that Houttuynia cordata Thunb water extract was beneficial in treating murine salmonellosis infection.55 Houttuynin, one of the primary active components, effectively inhibits P. aeruginosa biofilm dispersion.56 Ultrasonically nebulized Houttuynia cordata Thunb can remarkably attenuate inflammation and inhibit the colonization of Gram-negative bacilli in the respiratory tract in patients after pneumonectomy.57 Remarkably, Houttuynia cordata Thunb aerosol inhalation cures catarrhal pharyngitis.
Agastache rugosa (Fisch. & C.A.Mey.) Kuntze. Essential oil extracted from the leaf of Agastache rugosa (Fisch. & C.A.Mey.) Kuntze exerted antibacterial effects against E. coli (MIC, 9.4 μg mL−1), and essential oil from the flower inhibited S. aureus (MIC, 21 μg mL−1) potentially through inhibiting biofilm activity.58 Compared to penicillin, in some cases, these oils exhibited the same type of antibacterial activity, while in other cases these oils exhibited stronger activity than standard reference antibiotics (i.e., penicillin, gentamycin sulfate injection).
Cinnamomum cassia (L.) J.Presl. Combination of Cinnamon bark (CB) essential oil decreases MICs of piperacillin against a strain of β-lactamase-producing E. coli,59 indicating that CB essential oil decreases the use of antibiotics to reduce their adverse effects, likely reversing β-lactam antibiotic resistance.60 CB essential oil possesses antibacterial activity against Gram-negative bacteria, including Proteus spp. (MIC, 1.5 μL mL−1), K. pneumonia (MIC, 1.5 μL mL−1), Yersinia enterocolitica (MIC, 6.25 μL mL−1) and E. coli (MIC, 12.5 μL mL−1) by inhibiting biofilm formation.61
Allium sativum. L. Allium sativum L. (Garlic) extracts possess broad antibacterial spectrum and antifungal activity. Garlic essential oils were reported to exert considerable antimicrobial activity against S. aureus, P. aeruginosa, S. epidermidis, Salmonella enteritidis, L. monocytogenes, Aspergillus niger, Penicillium cyclopium and Fusarium oxysporum 62. The strong antimicrobial activity exhibited by garlic essential oils is primarily related to the chemical composition of sulfides such as allicin. The disulfide bonds in these compounds contribute to the antibacterial activity. These sulfides destroy microbial cells by reacting with the sulfhydryl groups (SH) in cellular proteins to produce disulfides.63 Allicin possess broad-spectrum antimicrobial activity against Gram-positive and Gram-negative bacteria, as well as fungi. Multiple antibiotic resistant bacterial strains, such as MRSA and other multidrug-resistant enterotoxicogenic strains of Enterococcus, E. coli, Shigella dysenteriae, S. flexneri, and S. sonnei are sensitive to allicin.64 Pure allicin exerted antifungal activity in vitro against Candida, Cryptococcus, Trichophyton, Epidermophyton, and Microsporum (MICs, 1.57–6.25 μg mL−1).65 1 mg allicin possesses antibiotic activity equivalent to that of 15 IU penicillin.66 Due to the rapid reaction between thiosulfonates and thiol groups, the primary antimicrobial effect of allicin is due to inhibition of thiol-containing microbial enzymes.67
Andrographis paniculata (Burm.f.) Nees. Andrographolide from the leaves of Andrographis paniculata (Burm.f.) Nees, exhibited broad spectrum growth inhibition activity against multiple bacteria by upregulating human β-defensin-2 induced through p38 MAP Kinase (MAPK) and NF-κB pathways in human lung epithelial cells.68 Andrographolide exhibited broad spectrum growth inhibition against E. coli, K. pneumonia, B. subtilis, S. aureus (MICs, 50–100 μg mL−1), Mycobacterium smegmatis, P. aeruginosa and Streptococcus thermophilus.69 Andrographolide increases susceptibility of P. aeruginosa to antibiotics (e.g., cefpirome, ceftazidine and chloramphenicol) and reduces expression levels of the MexAB-OprM efflux pump.70
Patrinia scabiosifolia Link Patrinia scabiosaefolia. Link was first recorded in the “Shennong's Herbal Classic of Materia Medica.” It is commonly used to treat appendicitis, carbuncle sores and lung abscesses. This medicinal herb inhibits proliferation in AmpC β-lactamase-producing bacteria.71 Oleanolic acid plays an important role in the antimicrobial actions of the herb, which has been illustrated against many human bacterial pathogens, e.g., S. pneumonia (MIC, 16 μg mL−1), methicillin-sensitive Staphylococcus aureus (MSSA) (MIC, 8 μg mL−1), MRSA (MIC, 64 μg mL−1), B. subtilis (MIC, 8 μg mL−1), Enterococcus faecalis (MIC, 6.25–8.00 μg mL−1) and E. faecium (MIC, 8 μg mL−1).72 The antimicrobial abilities of oleanolic acid are mediated by affecting efflux pumps and inducing stress responses. Grudniak et al.73 found that treatment of E. coli with oleanolic acid altered the synthesis of DnaK, which induced a heat-shock response. Kurek et al. verified that oleanolic acid affects the bacterial cell wall by inhibiting peptidoglycan turnover in L. monocytogenes.74
Rabdosia rubescens (Hemsl.) H.Hara. Rabdosia rubescens (Hemsl.) H.Hara. has been used as a tea drink for more than 1100 years. The antibacterial effects of ethanol extracts from the herb against S. aureus may occur through disruption of the cell wall and leakage of cellular contents. Oridonin, a bioactive ent-kaurane diterpenoidis, is one of the primary antibacterial active ingredients in Rabdosia rubescens (Hemsl.) H.Hara. and exhibits antibacterial activity against S. aureus, MRSA, β-lactamase-producing S. aureus (MIC is 3.125, 6.25, and 6.25 μg per disc, respectively)75 and Mycobacterium phlei (MIC, 16 μg mL−1).76 The antimycobacterial activity of oridonin might be significantly increased by introduction of a trans-cinnamic moiety. The C-1 substituents of oridonin may affect its antibacterial activity.
Thymus mongolicus (Ronniger) Ronniger. Thymol is the primary monoterpene phenol in essential oils isolated from Lamiaceae family plants (e.g., Thymus mongolicus (Ronniger) Ronniger and other plants). Thymol possesses antimicrobial activity against Gram-positive bacteria (e.g., S. aureus, S. epidermidis, B. cereus) and Gram-negative bacteria (e.g., E. coli, P. aeruginosa, Enterococcus faecalis, Vibrio parahaemolyticus, Vibrio alginolyticus and Salmonella typhimurium) with MICs of 32–64 μg mL−1.77 Furthermore, thymol possesses concentration-dependent inhibitory activity against ethidium bromide cell efflux. In addition, thymol exerts significant anti-biofilm activity.78
Salvia miltiorrhiza Bunge. Cryptotanshinone and dihydrotanshinone I from Salvia miltiorrhiza Bunge showed broad antibacterial activity against a wide spectrum of Gram-positive bacteria79 by inhibiting the action of topoisomerase I and generating superoxide radicals in B. subtilis lysates, which non-selectively inhibit DNA, RNA, and protein synthesis. Cryptotanshinone and dihydrotanshinone I inhibit A. tumefaciens, E. coli, P. lachrymans, R. solanacearum, X. vesicatoria, B. subtilis, S. aureus, S. haemolyticus and M. oryzae (MICs, 6.25 μg mL−1 to 100 μg mL−1).80 Tanshinone from Salvia miltiorrhiza Bunge is safe and effective against acne. Neither resistance nor obvious side effects were observed in the clinical trial.81
Apium graveolens L. Sedanolide from Apium graveolens L. seeds inhibits Candida parapsilasis and C. albicans.51 Apium graveolens L. essential oils, consisting of indenolide, neosebactam and phytadiene, exhibited antifungal activity against Candida albicans, Candida tropicalis, Candida krusei, Candida guilliermondii, Candida parapsilosis, Cryptococcus neoformans, Trichophyton rubrum, Trichophyton mentagrophytes, T. mentagrophytes var. interdigitale, Trichophyton verrucosum, Microsporum canis, Microsporum gypseum, Epidermophyton floccosum, Aspergillus niger, Aspergillus fumigatus and Aspergillus flavus (MICs, 0.04–0.64 μL mL−1).82 Apigenin is a flavonoid that is abundant in A. graveolens; the MICs of apigenin against Gram-positive and Gram-negative strains ranged from 32.5 to 62.5 μg mL−1. Apigenin significantly reduces the MIC of ampicillin (from 800 μg mL−1 to 107 μg mL−1), and ceftriaxone (from 58 μg mL−1 to 2.6 μg mL−1) against MRSA.83 Apigenin inhibited DNA gyrase, leading to the quinolone resistance mutation gyrA (Ser84Leu).84 Liu et al.
Organic acids
The antibacterial activity of organic acids may depend upon the physiological characteristics of the organism and the physicochemical status of the external environment.85 The bacteriostasis of organic acids is species specific because not all bacteria are affected by organic acids in a similar manner. Bacteria that cannot decrease their intracellular pH accumulate organic acid anions according to their pH gradient across the cell membrane.86 The bacteriostatic action of organic acids involves the following five possible mechanisms: energy competition between active transport of hydrogen ions and normal metabolism of bacteria, inhibition of bacterial cell membrane stability, increased intracellular osmotic pressure, inhibition of biomacromolecule synthesis and induction of antimicrobial peptides in host cells.87
Lonicera japonica Thunb. Lonicera japonica Thunb. (Honeysuckle flower) is the dried flower bud or opening flower of Lonicera japonica Thunb. Cynaroside, 3,5-di-O-caffeoylquinic acid and 4,5-di-ocaffeoylquinic acid from Lonicera japonicae leaves possessed the strongest antimicrobial activities against S. aureus and E. coli.88 Chlorogenic acid inhibits proliferation of S. aureus and disrupts cell membrane permeability.89 Oleanolic acid displayed antimicrobial effects against Mycobacterium tuberculosis (MIC, 25 μg mL−1), M. tuberculosis, streptomycin-, isoniazid-, rifampin-, and ethambutol-resistant strains (MIC, 50 μg mL−1).90 In addition, ursolic acid eliminates M. tuberculosis at 100 μg mL−1, while inhibiting S. mutans and S. sobrinus.91 These antibacterial actions are due to disruption of bacterial membrane integrity, as well as inhibition of protein synthesis and metabolic pathways.92 Oleanolic acid and ursolic acid affect multiple genes involved in S. mutans metabolism to inhibit glycolysis, as well as synthesis of amino acids, fatty acids and peptidoglycans, all of which contribute to its antimicrobial activity.90
Glycyrrhiza glabra L. Glycyrrhizic acid from Glycyrrhiza glabra L. enhanced the antibacterial effects of gentamicin against intrinsically resistant Enterococcus faecium.93 Glycyrrhizic acid at a subinhibitory concentration of 2.4 mM decreased the MIC of gentamicin in intrinsically resistant E. faecium strains to 6.25%, and low concentrations of glycyrrhizic acid (18 μM) increased the susceptibility of some E. faecium to gentamicin. 18β-glycyrrhetinic acid isolated from the root of the herb displays bactericidal activity against MRSA at high concentrations, reducing virulence gene expression in S. aureus at sublethal doses.94
Portulaca oleracea L. Portulaca oleracea L. is the dry aerial portion of the plant and is one of the most widely used medicinal plants according to WHO. Portulaca oleracea L. exhibits antimicrobial activity against N. gonorrhoea, S. aureus, E. coli and B. subtilis.95 Two active ingredients, linoleic and oleic acids isolated from the herb, exhibit antibacterial activity against MRSA when combined with erythromycin.96 The mechanism of their antimicrobial activity is potentially due to inhibition of bacterial cell efflux pumps.97
Rheum palmatum L. Rheum palmatum L. extracts inhibit Gram-negative and -positive bacterial strains.98 The major active components include five hydroxyanthraquinones, namely, rhein, aloe-emodin, emodin, chrysophanol and physcion.99 Rhein possesses the greatest antibacterial activity against Helicobacter pylori (MIC, 50 μg mL−1) and Porphyromonas gingivalis (MIC, 2.5 μg mL−1), and rhein decreases the expression of vital virulence factor genes in P. gingivalis.100 Rhein also significantly increases the antibacterial activity of amoxicillin against H. pylori. Rhein increase cell permeability of E. coli and Salmonella, causing leakage of cell contents and eventually leading to bacterial cell death.101
Polysaccharides
Polysaccharides are carbohydrate polymers in which at least 10 monosaccharides are linked by glycosidic bonds. In particular, oligosaccharides derived from Cordyceps sinensis (BerK.) Sacc., Astragalus mongholicus Bunge, Taraxacum mongolicum Hand.-Mazz. and Rosa laevigata Michx. have attracted considerable attention in recent years. Despite accumulating research on polysaccharides from herbs and mounting evidence suggesting that polysaccharides possess significant pharmacological effects, the underlying molecular mechanisms of polysaccharide antibacterial properties remain ill-defined.102
Cordyceps cicadae. Polysaccharides from Cordyceps cicadae (C. cicadae) display broad spectrum activity against E. coli, Klebsiella pneumonia, Vivrio cholera, P. aeruginosa, Vibrio alginolyticus, S. aureus, Vibrio parahaemolyticus and Streptococcus pneumonia, demonstrating maximum activity against V. parahaemolyticus.103 Zhang et al.104 extracted a water-soluble polysaccharide from C. cicadae, demonstrating that it exhibited antibacterial activity against E. coli, S. aureus, B. subtilis, Salmonella paratyphi and P. aeruginosa. C. cicadae polysaccharides exert their bactericidal effects through destruction of the bacterial cell wall and membrane, increasing cell permeability and resulting in structural lesions and release of cellular components.
Rosa laevigata Michx. Crude ethanol extracts from Rosa laevigata Michx. exhibited anti-MRSA activity.105 Polysaccharides extracted from the herb exhibit significant inhibitory effects against E. coli, Paratyphoid bacillus and S. aureus.106 At concentrations of 10–15 mg mL−1, the inhibitory effects of polysaccharide against S. aureus, P. bacillus and Saccharomyces cerevisiae were stronger than that of streptomycin (50 ppm).
Coumarins
Coumarin compounds that contain 1,2-benzopyrone structures are considered potential antibacterial agents due to their structural similarity to quinolone. They display excellent inhibitory activity against MRSA and methicillin-resistant Staphylococcus epidermidis (MRSE), arousing significant interest in the field of pharmacochemistry. Some natural coumarin compounds, such as novobiocin, chlorobiocin and coumermycin A1, have been used as a new type of antibiotic to treat infections caused by Gram-positive bacteria. Other natural coumarin compounds are waiting to be developed, such as decursinol angelate and decursin, isolated from Angelica gigas Nakai exhibit significant activity against B. subtilis with MICs of 50 μg mL−1 and 12 μg mL−1, respectively.107
Angelica dahurica (Hoffm.) Benth. & Hook.f. ex Franch. & Sav. 5-Methoxy-8-hydroxypsoralen isolated from the medicinal herb is lethal to Streptococcus iniae.108 Imperatorin, another coumarin extracted from the herb, possesses clear antibacterial activity. Imperatorin significantly inhibits the production of alpha-hemolysin (Hla) in S. aureus by reducing transcriptional levels of the gene encoding Hla and its accessory gene regulator. Furthermore, imperatorin prevented A549 epithelial cell injury induced by Hla in a co-culture system.109
Fraxini Cortex. Fraxini Cortex is a commonly used herbal medicine that was first recorded in the Shennong's Herbal Classic of Materia Medica. It is composed of dry branch or bark material from Fraxinus rhynchophylla Hance, Fraxinus chinensis Roxb., Fraxinus szaboana Lingelsh. and Fraxinus stylosa Lingelsh. Fraxini Cortex is used to treat enteritis, excessive leucorrhoea, chronic bronchitis, bacterial dysentery and other disorders.5 Liu et al.110 found that five coumarin monomers from Fraxinus cortex extracts displayed significant inhibitory and bactericidal effects against E. coli, S. aureus, and P. aeruginosa. The antibacterial activity of those five coumarins from strongest to weakest is fraxetin, aesculetin, aesculin, fraxin and 6,7-dichomethoxyl-8-hydroxycoumarin. Coumarins, such as aesculin, significantly inhibit the growth of E. coli in animal organs.111 Fraxetin displayed antibacterial activity against E. coli (MIC, 40 μg mL−1). The mechanism whereby this occurs might be due to altered permeability of the bacterial cell membrane, inhibition of bacterial soluble protein synthesis and elimination of bacterial plasmids.112 Fraxetin inhibits the activity of S. aureus by increasing cell membrane permeability, inhibiting bacterial DNA and RNA synthesis, and decreasing topoisomerase I and II the activity.113
Lignans
Lignans are a class of secondary plant metabolite that are produced by the oxidative dimerization of two phenylpropanoid units. Although the molecular skeleton of lignans is only comprised of two phenylpropane (C6–C3) units, lignans exhibit enormous structural diversity.114 However, current research on the antimicrobial constituents of lignans is still very limited. Lignans, e.g., forsythin and forsythoside, were found in Forsythia suspensa (Thunb.) Vahl as possible antibacterial agents.
Schisandra chinensis (Turcz.) Baill. Schisandra chinensis extract displays inhibitory effects against E. coli, B. subtilis, Salmonella and S. aureus115 as well as ciprofloxacin-resistant E. coli. The primary antibacterial components of the plant are lignans, namely, schisandrin and schisandrin A. Possible antibacterial effects include destruction of the smooth morphology of the bacterial cell membrane, causing leakage of contents and resulting in metabolic disorders that affect the absorption of carbohydrates and other nutrients.116
Sambucus williamsii Hance. Pinoresinol, lariciresinol, (−)-olivil-9′-O-β-D-glucopyranoside, glochidioboside and (+)-medioresinol isolated from Sambucus williamsii Hance exhibit antifungal effects. Compared to amphotericin B, (−)-olivil-9′-O-β-D-glucopyranoside exhibited favorable antifungal activity against C. albicans by destroying the cell membrane.117 (+)-Pinoresinol, lariciresinol and glochidioboside, also display antifungal effects by damaging the fungal plasma membrane without haemolysis.118 (+)-Medioresinol affects mitochondria and induces reactive oxygen species accumulation in C. albicans cells. Reactive oxygen species induce oxidative stress and increase mitochondrial dysfunction, leading to the release of pro-apoptotic factors.
Magnolia officinalis Rehder & E.H.Wilson. Magnolia officinalis extract (MOE) is rich in lignans, among which magnolol and honokiol are the two major constituents with potent antimicrobial activity against Listeria monocytogenes, Streptococcus faecalis, Escherichia coli, Salmonella, typhimurium, S. aureus, Bacillus anthracis, A. actinomycetemcomitans, S. mutans, and MRSA.119,120 Also, magnolol and honokiol in MOE, which is recently applied for the treatment of human oral diseases, exhibit strong inhibitory effect on oral pathogens such as periodontitis and cariogenic bacteria as well as a relatively low cytotoxic effect against human gingival fibroblasts and epithelial cells.121,122 MIC of honokiol was 10 μg mL−1 for A. actinomycetemcomitans, S. mutans, S. aureus and MRSA. Except S. aureus (MIC, 20 μg mL−1), MIC of magnolol was also 10 μg mL−1 for the microbes mentioned above.119 On top of that, both constituents exhibit stable antimicrobial effect over a wide range of temperatures and pH against the above microbes. Magnolol and honokiol bring forth antimicrobial activity by damaging microbial cell walls or membranes, resulting in increased permeability of cell membrane, which leads to the loss of intracellular components and inhibitory effect on microbial growth.123 Besides, magnolol and honokiol could also interact with cell membrane enzymes and proteins, leading to the impairment of cell structure and changes in cell morphology. Moreover, magnolol and honokiol inhibit the expression of drug-resistance mec gene, which is conducive to reducing the drug-resistance property of MRSA.119
Herbal medicine formulas
The effective ingredients in herbal medicine formulas (HMF) are the material basis for their therapeutic effect. Gegen Qinlian oral liquid exhibit significant antibacterial effects on Enterococcus (MIC, 20.83 μg mL−1), Salmonella (MIC, 41.7 μg mL−1), and Escherichia coli (MIC, 62.5 μg mL−1),124 which is stronger than that of single Pueraria lobata (Willd.) Ohwi, Scutellaria baicalensis Georgi and Coptis chinensis Franch extract. The Chinese compound gallnut (Rhus chinensis Mill., Rheum palmatum L., Scutellaria baicalensis Georgi) shows antimicrobial activity against Aeromonas sobria, Aeromonas caviae, Edwrdsieda tarda, and Flavobacterium columnare. And the effectively antimicrobial compounds of this formula are gallic acid, baicalin and quercetin, among which gallic acid accounts for the highest percentage of 10.47% and quercetin shows moderately antimicrobial activity with a MIC range of 125–312.5 μg mL−1.125 A mixture of Scutellaria baicalensis, Fraxini Cortex, Pulsatilla chinensis (Bunge) Regel and Sophora flavescens Aiton (mixed at a ratio of 1
:
4
:
1
:
2) showed greater antibacterial activity against S. aureus, E. Coli and Salmonella126. It is also noted that the MIC values for HMF (usually >100 μg mL−1) are always higher than those for pure compounds and herb extracts for the most likely reason that HMF are comprised of various single herbs and the antimicrobial components only account for a relatively small percentage. Consequently, a higher concentration for HMF would be needed to ensure the active compounds have an inhibitory or bactericidal effect on pathogens. The detailed antimicrobial HMF commonly used in clinical practice are shown in Table 1.
Table 1 Herbal medicine formulas with antimicrobial activity described during the past twenty-year perioda
HMF |
Formula composition |
Antimicrobial spectrum |
Ref. |
HMF: herbal medicine formulas. MIC: minimal inhibit concentration. ESBLs: extended spectrum beta-lactamases. MRSA: methicillin-resistant Staphylococcus aureus. MSSA: methicillin-sensitive Staphylococcus aureus. |
Gegen Qinlian oral liquid |
Puerariae Radix, Scutellaria baicalensis Georgi, Coptis chinensis Franch. |
Enterococcus (MIC: 20.83 μg mL−1), Salmonella (MIC: 41.7 μg mL−1), Escherichia coli (MIC: 62.5 μg mL−1) |
127 |
Pudilan oral liquid |
Taraxacum mongolicum Hand.-Mazz., Scutellaria baicalensis Georgi, Isatis tinctoria L., Corydalis bungeana Turcz. |
Staphylococcus aureus, Escherichia coli, Pseudomonas aeruginosa, C. albicans |
128 |
Sanhuang tablets |
Rheum palmatum L., Scutellaria baicalensis Georgi, Coptis chinensis Franch. |
Hemolytic Streptococcus B, Staphylococcus aureus, Escherichia coli, Pseudomonas aeruginosa, Bacillus subtilis, Candida albicans |
|
Qingkailing injection |
Cholic acid, mother of pearl, swine deoxycholic acid, baicali, Gardenia jasminoides J.Ellis, Cornus officinalis Siebold & Zucc., Isatis tinctoria L., Lonicera japonica Thunb. |
ESBLs-producing Klebsiella pneumonia |
129 |
Shuanghuanglian powder-injection |
Lonicera japonica Thunb., Forsythia suspensa (Thunb.) Vahl, Scutellaria baicalensis Georgi |
Escherichia coli, Staphylococcus aureus, Streptococcus pneumoniae, ESBLs-producing Klebsiella pneumonia extensively drug resistant Acinetobacter baumannii, pan-resistant Klebsiella pneumoniae |
130 |
131 |
Coptis chinensis injection |
Phellodendri Chinensis C.K.Schneid., Coptis chinensis Franch., Scutellaria baicalensis Georgi, Gardenia jasminoides J.Ellis |
ESBLs-producing Klebsiella pneumonia, MRSA |
132 |
Musk-Coptis injection |
Phellodendri Chinensis C.K.Schneid., Coptis chinensis Franch., Scutellaria baicalensis Georgi, Gardenia jasminoides J.Ellis |
ESBLs-producing Klebsiella pneumonia, MRSA |
|
Zhili powder |
Scutellaria baicalensis Georgi, Isatis tinctoria L., Taraxacum mongolicum Hand.-Mazz., Forsythia suspensa (Thunb.) Vahl |
Escherichia coli, Staphylococcus aureus, Salmonella |
133 |
Forsythia powder |
Forsythia suspensa (Thunb.) Vahl, Lonicera japonica Thunb., Platycodon grandiflorus (Jacq.) A.DC., Mentha canadensis L., Lophatherum gracile Brongn., Glycyrrhiza uralensis Fisch., Nepeta cataria L., Sojae Semen Praeparatum, Arctium lappa L. |
MRSA, MSSA |
134 |
Jinhuang power |
Aucklandia lappa DC., Coptis chinensis Franch., Scutellaria baicalensis Georgi, Phellodendri Chinensis C.K.Schneid., Rheum palmatum L., Curcuma longa L., Angelica dahurica (Hoffm.) Benth. & Hook.f. ex Franch. & Sav., Trichosanthes kirilowii Maxim., Magnolia officinalis Rehder & E.H.Wilson, Citrus reticulata Blanco, Atractylodes lancea (Thunb.) DC., Arisaema heterophyllum Blume, Pinellia ternata (Thunb.) Makino, Glycyrrhizae, Bletilla striata (Thunb.) Rchb.f. |
MRSA ATCC 43300, Staphylococcus aureus, Staphylococcus epidermidis, Pseudomonas maltophilia |
135 |
Ramuli Cinnamomi Decoction |
Arctium lappa L., Paeonia lactiflora Pall., Glycyrrhiza uralensis Fisch., Zingiber officinale Roscoe, Ziziphus jujuba Mill. |
MRSA, MSSA |
|
Xiao Chaihu Decoction |
Bupleurum scorzonerifolium Willd.,Pinellia ternata (Thunb.) Makino, Glycyrrhiza uralensis Fisch., Scutellaria baicalensis Georgi, Zingiber officinale Roscoe, Ziziphus jujuba Mill. |
Escherichia coli, Staphylococcus aureus, Streptococcus pneumoniae |
136 |
Modified Xiaochaihutang |
Bupleurum scorzonerifolium Willd., Codonopsis pilosula (Franch.) Nannf., Coptis chinensis Franch., Zingiber oj-jicinale Rosc., Poria, Atractylodes macrocephala Koidz. |
H. pylori |
137 |
Pulsatillae Decoction |
Pulsatilla chinensis (Bunge) Regel, Coptis chinensis Franch., Phellodendri Chinensis C.K.Schneid., Fraxini Cortex |
Staphylococcus aureus, Staphylococcus epidermidis, Shigella flexneri, Shigella sonnei |
|
Formulas 1 |
Astragalus mongholicus Bunge, Salvia miltiorrhiza Bunge, Forsythia suspensa (Thunb.) Vahl, Carthamus tinctorius L. |
Staphylococcus aureus, Streptococcus agalactiae |
138 |
Formulas 2 |
Coptis chinensis Franch., Salvia miltiorrhiza Bunge, Forsythia suspensa (Thunb.) Vahl, Carthamus tinctorius L. |
Escherichia coli, Staphylococcus aureus, Streptococcus agalactiae |
|
Formulas 3 |
Astragalus mongholicus Bunge, Salvia miltiorrhiza Bunge, Coptis chinensis Franch., Carthamus tinctorius L. |
Escherichia coli, Staphylococcus aureus, Streptococcus agalactiae |
|
Formulas 4 |
Scutellaria baicalensis Georgi, Isatis tinctoria L., Taraxacum mongolicum Hand.-Mazz., Forsythia suspensa (Thunb.) Vahl |
Escherichia coli, Staphylococcus aureus, Salmonella |
139 |
The synergistic or additive effect of HMF and chemical antimicrobial agents has been embodied in clinical practice. For example, Pudilan oral liquid combined with azithromycin shows synergistic effects in treating paediatric pneumonia.140 This compound also works in synergy with metronidazole and amoxicillin when treating H. pylori infection.138 Combinations of Shuanghuanglian preparations and chemical antimicrobial agents (e.g., amikacin, minocycline, piperacillin/tazobactam and cefoperazone/sulbactam) exhibit synergistic or additive antimicrobial effects against extensively drug resistant Acinetobacter baumannii.139 Combinations of Shuanghuanglian preparations and imipenem showed synergistic effects on Klebsiella pneumoniae.141
Antimicrobial activity and mechanisms of herbal components
Flavonoids are the most promising antimicrobial agents, which display favorable antibacterial activity. Most of alkaloids exhibit relatively weaker antibacterial effect, however, berberine displays strong antimicrobial activities. Many terpenes and partial essential oils display strong antimicrobial activity. Organic acids, e.g., chlorogenic acid from Lonicera japonica Thunb. show exploitable antimicrobial activity. According to Kuete and Efferth,142 antibacterial activity parameters are given for herb extracts and pure compounds. For extracts, it is estimated that they're significantly active if their MIC values ≤ 100 μg mL−1, moderately active if 100 < MIC ≤ 625 μg mL−1 and weakly active if MIC > 625 μg mL−1; for pure compounds, they're significantly active if their MIC values ≤ 10 μg mL−1, moderately active if 10 < MIC ≤ 100 μg mL−1 and weakly active if MIC > 100 μg mL−1. The antimicrobial herb extracts and their compounds described in the past two decades are summarized in Table 2.
Table 2 Herbal extracts and compounds with antimicrobial activity described during the past twenty-year perioda
Herbal medicine |
Extract/compound |
Bacteria |
Antimicrobial activity (MIC) |
Ref. |
MIC: minimal inhibit concentration. ETEC: enterotoxigenic Escherichia coli. MRSA: methicillin-resistant Staphylococcus aureus. MSSA: methicillin sensitive Staphylococcus aureus. |
Alkaloids |
Coptis chinensis Franch. |
Berberine hydrochloride |
Candida albicans |
160 μg mL−1 |
143 |
Berberine |
ETEC |
1.75 to 1.96 μM |
10,12 |
Trichophyton mentagrophytes |
250.4 μg mL−1 |
Trichophyton rubrum |
125 μg mL−1 |
Microsporum canis |
62 μg mL−1 |
Microsporum gypseum |
125 μg mL−1 |
Sophora flavescens aiton |
Alkaloid extract |
P. aeruginosa |
62.5 μg mL−1 |
144 |
B. subtilis |
62.5 μg mL−1 |
Delphinium cashmerianum Royle |
Ethyl acetate extract |
S. pneumonia |
6.25 μg mL−1 |
145 |
K. pneumoniae |
25 μg mL−1 |
C. albicans |
50 μg mL−1 |
Methanol extracts |
S. pneumonia |
25 μg mL−1 |
N. mucosa |
50 μg mL−1 |
![[thin space (1/6-em)]](https://www.rsc.org/images/entities/char_2009.gif) |
Flavonoids |
Curcuma longa L. |
Curcumin |
MRSA |
125–250 μg mL |
42,146 |
Aqueous extract |
S. epidermis ATCC 12228 |
4 × 103 μg mL−1 |
147 |
S. aureus ATCC 25923 |
6 × 103 μg mL−1 |
K. pneumoniae ATCC 10031 |
1.6 × 104 μg mL−1 |
E. coli ATCC 25922 |
4 × 103 μg mL−1 |
Scutellaria baicalensis Georgi |
Baicalein |
S. anginosus |
MIC50s, 20–160 μg mL−1 |
30 |
S. gordonii |
MIC90s, 80–320 μg mL−1 |
P. intermedia |
Portulaca oleracea L. |
Flavonoids |
E. coli |
313 μg mL−1 |
148 |
S. aureus |
156 μg mL−1 |
Viola philippica Cav. |
|
Streptococcus galactostasis |
39 μg mL−1 |
Staphylococcus aureus |
78 μg mL−1 |
Escherichia coli |
156 μg mL−1 |
Streptococcus agalactiae |
313 μg mL−1 |
Streptococcus mammae |
625 μg mL−1 |
Salmonella |
1250 μg mL−1 |
Trollius chinensis Bunge |
Orientin |
S. aureus |
25 μg mL−1 |
149 |
S. epidermidis |
25 μg mL−1 |
Total flavonoids |
S. epidermidis |
80 μg mL−1 |
150 |
S. aureus |
80 μg mL−1 |
Shigella dysenteriae |
160 μg mL−1 |
S. pyogenes beta |
310 μg mL−1 |
S. paratyphi A |
620 μg mL−1 |
Croton betaceus Baill. |
Hexane fraction |
Enterococcus faecalis |
1.0 mg mL−1 |
151 |
Streptococcus mutans |
1.0 mg mL−1 |
Candida albicans |
1.0 mg mL−1 |
Croton lundianus (Didr.) Müll.Arg. |
Hexane fraction |
Streptococcus mutans |
1.0 mg mL−1 |
151 |
Cullen corylifolium (L.) Medik. |
Phenolic extract of seeds |
C. difficile |
8 μg mL−1 |
152 |
Isobavachalcone |
C. difficile |
4 μg mL−1 |
![[thin space (1/6-em)]](https://www.rsc.org/images/entities/char_2009.gif) |
Essential oils and terpenes |
Allium sativum L. |
Essential oil |
S. aureus |
100 μg mL−1 |
62,70,153 |
P. aeruginosa |
S. epidermidis |
Salmomella Enteritidis |
L. monocytogenes |
Elephantopus scaber L. |
Scabertopin |
S. aureus |
80 μg mL−1 |
154 |
S. epidermidi |
80 μg mL−1 |
Trichophyton mentagrophytes |
80 μg mL−1 |
Isoscabertopin |
S. aureus |
160 μg mL−1 |
S. epidermidi |
160 μg mL−1 |
Trichophyton mentagrophytes |
80 μg mL−1 |
Deoxyelephantopin |
S. aureus |
160 μg mL−1 |
S. epidermidi |
160 μg mL−1 |
Trichophyton rubrum |
80 μg mL−1 |
Trichophyton mentagrophytes |
80 μg mL−1 |
Microsporum canis |
80 μg mL−1 |
Isodeoxyelephantopin |
Trichophyton rubrum |
80 μg mL−1 |
Trichophyton mentagrophytes |
80 μg mL−1 |
Microsporum canis |
80 μg mL−1 |
Houttuynia cordata Thunb. |
Ethanol extract |
MRSA |
110–1760 μg mL−1 |
155 |
MSSA |
110–1760 μg mL−1 |
Dendranthema morifolium (Ramat.) Tzvelev |
Essential oil |
Streptococci species |
200–800 μg mL−1 |
156 |
streptococci ratti and obligate anaerobic bacteria such as F. nucleatum, P. intermedia, and P. gingivalis |
100–200 μg mL−1 |
Andrographis paniculata (Burm.f.) Nees |
Andrographolide |
E. coli |
50 μg mL−1 |
69 |
K. pneumonia |
100 μg mL−1 |
B. subtilis |
100 μg mL−1 |
S. aureus |
100 μg mL−1 |
Mycobacterium smegmatis |
200 μg mL−1 |
P. aeruginosa |
200 μg mL−1 |
Streptococcus thermophilus |
350 μg m−1 |
Patrinia scabiosifolia Link |
Oleanolic acid |
P. aeruginosa |
256 μg mL−1 |
72 |
Thymus mongolicus (Ronniger) Ronniger |
Essential oil |
E. coli |
350 mL/L |
87,157 |
Aspergillus niger |
S. aureus |
Saccharomyces cerevisiae |
Aspergillus flavus |
Mentha suaveolens ehrh. |
Essential oil |
S. xylosus |
14.4 μL mL−1 |
158 |
Ocimum basilicum L. |
Ocimum basilicum L. oil |
S. typhimurium |
0.009–23.48 μg mL−1 |
159 |
Trachyspermum ammi (L.) Sprague |
Essential oil extracted from seeds |
K. pneumoniae |
250 ppm |
160 |
E. coli |
100 ppm |
S. aureus |
Allium cepa L. |
Onion oil |
S. aureus |
12 μg mL−1 |
161 |
Lonicera japonica Thunb. |
Ursolic acid |
Streptococcus mutans |
2 μg mL−1 |
91 |
Streptococcus sobrinus |
4 μg mL−1 |
Salvia miltiorrhiza Bunge |
Cryptotanshinone |
A. tumefaciens |
12.5 μg mL−1 |
80 |
E. coli |
12.5 μg mL−1 |
P. lachrymans |
12.5 μg mL−1 |
R. solanacearum |
12.5 μg mL−1 |
X. vesicatoria |
6.25 μg mL−1 |
B. subtilis |
25 μg mL−1 |
S. aureus |
100 μg mL−1 |
S. haemolyticus |
50 μg mL−1 |
M. oryzae |
6.25 μg mL−1 |
Dihydrotanshinone I |
A. tumefaciens |
6.25 μg mL−1 |
80 |
E. coli |
25 μg mL−1 |
P. lachrymans |
12.5 μg mL−1 |
R. solanacearum |
12.5 μg mL−1 |
X. vesicatoria |
12.5 μg mL−1 |
B. subtilis |
25 μg mL−1 |
S. aureus |
100 μg mL−1 |
S. haemolyticus |
50 μg mL−1 |
M. oryzae |
3.13 μg mL−1 |
Apium graveolens L. |
Sedanolide |
Candida parapsilasis |
100 μg mL−1 |
162 |
C. albicans |
100 μg mL−1 |
Thymol |
E. coli |
0.04–0.64 μL mL−1 |
67,77 |
P. aeruginosa |
Enterococcus feacalis |
Vibrio paraheamolyticus |
Vibrio alginolyticus |
Salmonella typhimurium |
Taraxacum mongolicum Hand.-Mazz. |
Hexane extract |
S. aureus |
200 μg mL−1 |
163 |
E. coli |
400 μg mL−1 |
K. pneumoniae |
800 μg mL−1 |
Origanum vulgare L. |
Essential oils |
S. aureus |
0.015 μL mL−1 |
164 |
Sporothrix schenckii |
62–500 μg mL−1 |
Sporothrix brasiliensis |
125–250 μg mL−1 |
O.vulgare extracts |
S. aureus |
62.5–125 μg mL−1 |
Staphylococcus epidermidis |
M. luteus |
Bacillus subtilis |
Enterococcus feacalis |
K. pneumoniae |
Blumea balsamifera DC. |
Essential oils |
S. aureus |
9.77 μg mL−1 |
165 |
Seriphidium herba-alba (asso) Y.R.Ling |
Total extract |
S. aureus |
125–500 μg per disc |
166 |
B. subtilis |
E. coli |
F. solani |
62.5–500 μg per disc |
C. albicans |
1,3,8-Trihydroxyeudesm-4-en-7α,11βH-12,6α-olide |
B. subtilis |
25–50 μg per disc |
S. aureus |
C. albicans |
Benzoic acid p-(b-D-glucopyranosyloxy)-methyl ester |
B. subtilis |
25–50 μg per disc |
S. aureus |
Satureja hortensis L. |
Volatile oils |
Aerococcus viridans |
680 μg mL−1 |
167 |
Eubacterium lentum |
80 μg mL−1 |
Pantoea spp. |
170 μg mL−1 |
Actinomyces naeslundii |
340 μg mL−1 |
Staphylococcus sciuri |
340 μg mL−1 |
Anethum graveolens L. |
Volatile oils |
Streptococcus intermedius |
710 μg mL−1 |
![[thin space (1/6-em)]](https://www.rsc.org/images/entities/char_2009.gif) |
Organic acids |
Rhus c hinensis Mill. |
Gallic acid |
P. aeruginosa |
500 μg mL−1 |
168 |
E. coli |
1500 μg mL−1 |
S. aureus |
1750 μg mL−1 |
L. monocytogenes |
2000 μg mL−1 |
![[thin space (1/6-em)]](https://www.rsc.org/images/entities/char_2009.gif) |
Polysaccharides |
Cordyceps cicadae |
Polysaccharide |
E. coli |
100 μg mL−1 |
104 |
Polygonatum odoratum (Mill.) Druce |
Raw P. sibiricum polysaccharide |
E. coli |
1230 μg mL−1 |
169 |
B. subtilis |
980 μg mL−1 |
S. aureus |
1310 μg mL−1 |
Processing P. sibiricum polysaccharide |
E. coli |
670 μg mL−1 |
B. subtilis |
1160 μg mL−1 |
S. aureus |
740 μg mL−1 |
![[thin space (1/6-em)]](https://www.rsc.org/images/entities/char_2009.gif) |
Coumarins |
Cajanus cajan (L.) Millsp. |
Cajanuslactone |
S. aureus ATCC 6538 |
31 μg mL−1 |
170 |
![[thin space (1/6-em)]](https://www.rsc.org/images/entities/char_2009.gif) |
Lignans |
Schisandra chinensis (Turcz.) Baill. |
S. chinensis extract |
Ciprofloxacin-resistant E. coli |
15.63 μg mL−1 |
115 |
Forsythia suspensa (Thunb.) Vahl |
Forsythin |
P. aeruginosa |
512 μg mL−1 |
171 |
Extract |
S. aureus |
MIC90, 980 μg mL−1 |
172 |
S. epidermidis |
MIC90, 244 μg mL−1 |
The antimicrobial mechanisms of herbal components involve damage to the cell membrane and wall, inhibition of nucleic acid and protein synthesis (e.g., inhibition of DNA topoisomerase I, II and IV), inhibition of energy metabolism (e.g., inhibition of NADH-cytochrome c reductase, succinate dehydrogenase and malate dehydrogenase), inhibition of bacterial efflux pumps, and increased intracellular osmotic pressure. Organic acids also achieve their antimicrobial activities by increasing intracellular pH. In general, damage to cell membranes is the most common antimicrobial pathway. The antimicrobial mechanisms of herbal components were shown in Fig. 2.
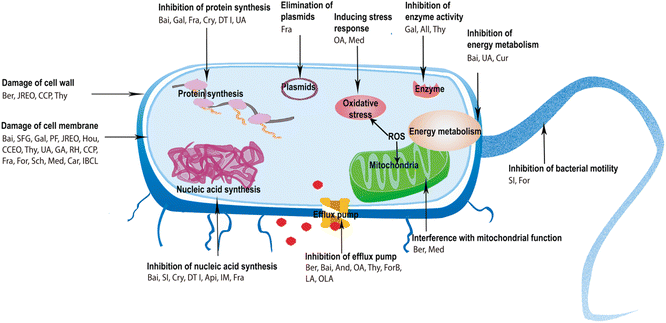 |
| Fig. 2 Antimicrobial mechanisms of herbal components. All, allicin; And, Andrographolide; Api, apigenin; Bai, baicalein; Ber, Berberine; Car, carvacrol; CCEO, Cinnamomum camphora (L.) Presl. essential oil; CCP, Cordyceps cicadae polysaccharide; Cry, Cryptotanshinone; Cur, Curcumin; DTI, dihydrotanshinone I; For, forsythin; ForB, forsythoside B; Fra, fraxetin; GA, gallic acid; Gal, Galangin; Hou, Houttuynin; IBCL, isobavachalcone; IM, imperatorin; JREO, Juniperus rigida essential oil; LA, linoleic acid; Med, (+)-medioresinol; OA, oleanolic acid; OLA, oleic acid; PF, purslane flavonoids; RH, rhein; Sch, schizandin; SFG, sophoraflavanone G; SI,Soy isoflavones; Thy, thymol; UA, ursolic acid. | |
Conclusions and perspectives
The increasing occurrence of dangerous infections caused by resistant bacteria has made the exploration of new molecules and chemical entities an urgent topic in the medical field on a global scale. Compared to synthetic chemistry, herbs provide greater structural diversity, and offer more opportunities for identifying novel antimicrobial compounds which are the most consistently successful source of drugs. Herbs display excellent antibacterial effect due to their safety, effectiveness, antimicrobial synergism and decreased drug resistance based on their multi-component, multi-drug target.85 Besides, plant secondary metabolites include heterogeneous categories of naturally existing compounds, which have been investigated since the 1850s and developed as effective drugs applied to treat diverse diseases.4,173 Several studies have explored the potential of various secondary metabolites against microbial infections in vivo without affecting the beneficial microbes in the gastrointestinal tracts. And secondary metabolites can exert a synergistical or additive effect with less efficient antimicrobial agents combating pathogens including MDRs.174 In order to find the novel antibacterial agents for combating multidrug-resistant microbes, future studies should also put an emphasis on developing the medicinal botanicals which generate a rich diversity of secondary metabolites.175
Combination of herbs and chemical antimicrobial agents for the treatment of infectious diseases is popular in clinical practice in China because of their synergistic or additive effects. Some herbal extracts or components enhanced the antibacterial activity of antimicrobial agents against sensitive and multidrug-resistant microorganisms when combined with antibiotics.176,177 The synergistic or additive action was possibly related to multiple compounds in herbal extracts jointly acting on multiple sites and targets of bacteria by damaging the bacteria cell wall and membrane,178 facilitating drug entry, inhibiting drug efflux pumps179 and enzymes that invalidate chemical antimicrobial agents (such as penicillinase and β-lactamase). Meanwhile, some research technologies, such as new omics technologies and network pharmacology, become an asset for finding the most effective combinations among antimicrobial herbs or in combination with currently available synthetic antibiotics.180 This provides new avenues for the prevention and treatment of infectious disease.
It should be noted that herbs exhibit different antibacterial activity due to different species, producing areas, harvest seasons, medicinal parts, extraction, separation, and purification process. Compared to chemical antibacterial agents, herbal products show low efficiency of bacteriostasis and sterilization, poor antibacterial specificity, and incompatibility, as well as trust issues among doctors and patients. For some herbal components with antibacterial potential, their activity can be improved by structural modification.
Undoubtedly, as extensively occurring natural resources, medicinal phytochemicals play an important role in future discoveries of new drugs, but only a small percentage of them have been studied. There is still a need to screen and identify more small molecular compounds with potent bioactivity for drug discovery researchers. On top of that, one of the difficulties for future studies on numerous phytochemicals is to find more effective and appropriate forms of drug administration conducive to releasing active compounds at the target site in infectious human bodies. Another difficulty is to develop better methods used to precisely determine the botanical compounds with antimicrobial activity in plant extracts or herbal medicine formulas, which involve in complex components.
In the future, study on the antibacterial effect of herbs should also attach importance on the mechanisms of action, pharmacodynamic material basis (i.e., medicinal composition), pharmacokinetics and synergistic action (e.g., herb–herb interaction, herb–chemical antibacterial agent interaction), as well as novel product development (e.g., novel drug delivery systems). The screening and purification of antimicrobial ingredients from herbs become necessary for precise pharmacodynamic evaluation and quality control. Supramolecular self-assembly and self-delivery strategy (nanoparticles and nanofibers)181 can be used to construct herbal nano-antimicrobial agents for the treatment of bacterial infections. The above studies can be conducted at the molecular, subcellular, cellular tissues, organs and system level, especially utlize some modern research methods such as molecular pharmacology, cell pharmacology, network pharmacology, quantitative pharmacology, chronopharmacology, sampling techniques, in silico high-throughput screening (HTS), -omics technologies, synergy studies and metabonomics.175,182,183
Author contributions
Jingru Liang: investigation, writing – original draft. Xuan Huang: investigation, writing – original draft. Guo Ma: conceptualization, writing – review & editing, project administration, funding acquisition.
Conflicts of interest
There are no conflicts to declare.
Abbreviations
AChE | acetylcholinesterase |
ASS | acid sodium salt |
CB | Cinnamon bark |
CL | Curcuma longa |
ETS | electron transport system |
FBCI | fractional bactericidal concentration index |
FICI | fractional inhibitory concentration index |
FtsZ | Filamenting temperature-sensitive mutant Z |
hERG | human eag-related gene |
Hla | alpha-hemolysin |
HMF | herbal medicine formulas |
LD50 | medial lethal dose |
MAPK | MAP Kinase |
MDR | multi-drug resistance |
MFC | minimal fungicidal concentration |
MIC | minimum inhibitory concentration |
MOE | Magnolia officinalis extract |
MRSA | methicillin-resistant Staphylococcus aureus |
MRSE | methicillin-resistant Staphylococcus epidermidis |
MSSA | methicillin-sensitive Staphylococcus aureus |
TCM | traditional Chinese medicine |
WHO | World Health Organization |
Acknowledgements
Thanks for the library of Fudan University, which provides literature databases for us to complete this review. This work was supported by National Natural Science Funds of China [grant numbers 82074109, 81873078, 81374051]; Science and Research Program of Shanghai Municipal Health Commission [grant number 201740094]; Science and Research Program of Traditional Chinese Medicine of Shanghai Municipal Health Commission [grant number 2018YP001]; MHHFDU-SPFDU Joint Research Fund [grant numbers RO-MY201707, 2021MHJC11]; Key Innovative Team of Shanghai Top-Level University Capacity Building in Clinical Pharmacy and Regulatory Science at Shanghai Medical College, Fudan University [grant number HJW-R-2019-66-19].
References
- I. Roca, M. Akova, F. Baquero, J. Carlet, M. Cavaleri, S. Coenen, J. Cohen, D. Findlay, I. Gyssens, O. E. Heuer, G. Kahlmeter, H. Kruse, R. Laxminarayan, E. Liébana, L. López-Cerero, A. MacGowan, M. Martins, J. Rodríguez-Baño, J. M. Rolain, C. Segovia, B. Sigauque, E. Tacconelli, E. Wellington and J. Vila, New Microbes New Infect., 2015, 6, 22–29 CrossRef.
- WHO, Antimicrobial Resistance: Global Report on Surveillance, 2014 Search PubMed.
- Y. Y. Zhou, Q. Cheng, K. Liu, L. Lu and J. Zhu, Chin. J. Antibiot., 2018, 43, 5–11 Search PubMed.
- M. G. Moloney, Trends Pharmacol. Sci., 2016, 37, 689–701 CrossRef CAS PubMed.
- C. P. Commission, Pharmacopoeia of China, China Medical Science and Technology Press, Beijing, 2020 Search PubMed.
- M. Tillhon, L. M. Guamán Ortiz, P. Lombardi and A. I. Scovassi, Biochem. Pharmacol., 2012, 84, 1260–1267 CrossRef CAS PubMed.
- M. Wang, Z. F. Liu, H. Tang and B. A. Chen, Chin. J. Nat. Med., 2018, 16, 561–571 CAS.
- N. Sun, F. Y. Chan, Y. J. Lu, M. A. Neves, H. K. Lui, Y. Wang, K. Y. Chow, K. F. Chan, S. C. Yan, Y. C. Leung, R. Abagyan, T. H. Chan and K. Y. Wong, PLoS One, 2014, 9, e97514 CrossRef.
- G. Pandey, S. Khatoon, M. M. Pandey and A. K. S. Rawat, J. Ayurveda Integr. Med., 2018, 9, 169–176 CrossRef PubMed.
- S. Bandyopadhyay, P. H. Patra, A. Mahanti, D. K. Mondal, P. Dandapat, S. Bandyopadhyay, I. Samanta, C. Lodh, A. K. Bera, D. Bhattacharyya, M. Sarkar and K. K. Baruah, Asian Pac. J. Trop. Med., 2013, 6, 315–319 CrossRef CAS PubMed.
- S. Dhamgaye, F. Devaux, P. Vandeputte, N. K. Khandelwal, D. Sanglard, G. Mukhopadhyay and R. Prasad, PLoS One, 2014, 9, e104554 CrossRef.
- H. Mahmoudvand, S. A. Ayatollahi Mousavi, A. Sepahvand, F. Sharififar, B. Ezatpour, F. Gorohi, E. Saedi Dezaki and S. Jahanbakhsh, ISRN Pharmacol., 2014, 2014, 602436 CrossRef PubMed.
- J. Kim, T. Ha Quang Bao, Y. K. Shin and K. Y. Kim, World J. Microbiol. Biotechnol., 2018, 34, 167 CrossRef PubMed.
- T. Xu, T. Kuang, H. Du, Q. Li, T. Feng, Y. Zhang and G. Fan, Pharmacol. Res., 2020, 152, 104632 CrossRef CAS.
- B. L. Ma, Y. M. Ma, R. Shi, T. M. Wang, N. Zhang, C. H. Wang and Y. Yang, J. Ethnopharmacol., 2010, 128, 357–364 CrossRef.
- J. Wang, L. Wang, G. H. Lou, H. R. Zeng, J. Hu, Q. W. Huang, W. Peng and X. B. Yang, Pharm. Biol., 2019, 57, 193–225 CrossRef PubMed.
- A. Schramm, I. Baburin, S. Hering and M. Hamburger, Planta Med., 2011, 77, 692–697 CrossRef PubMed.
- H. A. Jung, B. S. Min, T. Yokozawa, J. H. Lee, Y. S. Kim and J. S. Choi, Biol. Pharm. Bull., 2009, 32, 1433–1438 CrossRef PubMed.
- H. T. Xiao, J. Peng, Y. Liang, J. Yang, X. Bai, X. Y. Hao, F. M. Yang and Q. Y. Sun, Nat. Prod. Res., 2011, 25, 1418–1422 CrossRef PubMed.
- C. V. Pereira, N. G. Machado and P. J. Oliveira, Toxicol. Sci., 2008, 105, 408–417 CrossRef PubMed.
- T. Wu, M. He, X. Zang, Y. Zhou, T. Qiu, S. Pan and X. Xu, Biochim. Biophys. Acta, 2013, 1828, 2751–2756 CrossRef PubMed.
- S. Selvaraj, S. Krishnaswamy, V. Devashya, S. Sethuraman and U. M. Krishnan, Prog. Lipid Res., 2015, 58, 1–13 CrossRef CAS PubMed.
- Z. P. Xiao, X. D. Wang, P. F. Wang, Y. Zhou, J. W. Zhang, L. Zhang, J. Zhou, S. S. Zhou, H. Ouyang, X. Y. Lin, M. Mustapa, A. Reyinbaike and H. L. Zhu, Eur. J. Med. Chem., 2014, 80, 92–100 CrossRef CAS PubMed.
- N. Chinnam, P. K. Dadi, S. A. Sabri, M. Ahmad, M. A. Kabir and Z. Ahmad, Int. J. Biol. Macromol., 2010, 46, 478–486 CrossRef CAS.
- D. Wu, Y. Kong, C. Han, J. Chen, L. Hu, H. Jiang and X. Shen, Int. J. Antimicrob. Agents, 2008, 32, 421–426 CrossRef CAS PubMed.
- L. Chen, X. Zhang, X. Peng, Y. Yang and H. Yu, Microb. Pathog., 2019, 132, 59–65 CrossRef CAS PubMed.
- Y. Chen, T. Liu, K. Wang, C. Hou, S. Cai, Y. Huang, Z. Du, H. Huang, J. Kong and Y. Chen, PLoS One, 2016, 11, e0153468 CrossRef PubMed.
- B. Y. Yun, L. Zhou, K. P. Xie, Y. J. Wang and M. J. Xie, Yaoxue Xuebao, 2012, 47, 1587–1592 CAS.
- E. J. Jang, S. M. Cha, S. M. Choi and J. D. Cha, Arch. Oral Biol., 2014, 59, 1233–1241 CrossRef PubMed.
- S. Siriwong, T. Pimchan and W. Naknarong, Trop. J. Pharm. Res., 2015, 14, 641–648 CrossRef.
- W. Cai, Y. Fu, W. Zhang, X. Chen, J. Zhao, W. Song, Y. Li, Y. Huang, Z. Wu, R. Sun, C. Dong and F. Zhang, BMC Microbiol., 2016, 16, 181 CrossRef PubMed.
- Z. L. Wang, S. Wang, Y. Kuang, Z. M. Hu, X. Qiao and M. Ye, Pharm. Biol., 2018, 56, 465–484 CrossRef PubMed.
- B. C. Chan, M. Ip, C. B. Lau, S. L. Lui, C. Jolivalt, C. Ganem-Elbaz, M. Litaudon, N. E. Reiner, H. Gong, R. H. See, K. P. Fung and P. C. Leung, J. Ethnopharmacol., 2011, 137, 767–773 CrossRef.
- J. Luo, J. L. Kong, B. Y. Dong, H. Huang, K. Wang, L. H. Wu, C. C. Hou, Y. Liang, B. Li and Y. Q. Chen, Drug Des., Dev. Ther., 2016, 10, 183–203 CrossRef.
- S. Yang, Y. Fu, X. Wu, Z. Zhou, J. Xu, X. Zeng, N. Kuang and Y. Zeng, Biochem. Biophys. Res. Commun., 2014, 451, 36–41 CrossRef.
- R. Dhayakaran, S. Neethirajan and X. Weng, Biochem. Biophys. Rep., 2016, 6, 149–157 Search PubMed.
- A. P. Mukne, V. Viswanathan and A. G. Phadatare, Pharmacogn. Rev., 2011, 5, 13–18 CrossRef.
- R. P. N. Albert Dhayakaran, J. S. Xue and J. Shi, LWT--Food Sci. Technol., 2015, 63, 859–865 CrossRef.
- Q. Wang, H. Wang and M. Xie, Arch. Microbiol., 2010, 192, 893–898 CrossRef.
- J. D. Cha, S. E. Moon, J. Y. Kim, E. K. Jung and Y. S. Lee, Phytother. Res., 2009, 23, 1326–1331 CrossRef.
- H. Tsuchiya and M. Iinuma, Phytomedicine, 2000, 7, 161–165 CrossRef.
- S. H. Mun, O. H. Kang, D. K. Joung, S. B. Kim, Y. S. Seo, J. G. Choi, Y. S. Lee, S. W. Cha, Y. S. Ahn, S. H. Han and D. Y. Kwon, Evidence-Based Complementary Altern. Med., 2013, 2013, 823794 Search PubMed.
- T. P. Cushnie and A. J. Lamb, Phytomedicine, 2006, 13, 187–191 CrossRef PubMed.
- J. Ouyang, F. Sun, W. Feng, Y. Xie, L. Ren and Y. Chen, Chemotherapy, 2018, 63, 20–28 CrossRef PubMed.
- G. Eumkeb, S. Sakdarat and S. Siriwong, Phytomedicine, 2010, 18, 40–45 CrossRef.
- M. Karaman, F. Fırıncı, Z. Arıkan Ayyıldız and I. H. Bahar, Mikrobiyol. Bul., 2013, 47, 192–194 CrossRef.
- D. Praditya, L. Kirchhoff, J. Brüning, H. Rachmawati, J. Steinmann and E. Steinmann, Front. Microbiol., 2019, 10, 912 CrossRef PubMed.
- C. Dai, Y. Wang, G. Sharma, J. Shen, T. Velkov and X. Xiao, Antioxid., 2020, 9, 506 CrossRef.
- Y. Y. Yi, J. J. He, J. Q. Su, S. Z. Kong, J. Y. Su, Y. C. Li, S. H. Huang, C. W. Li, X. P. Lai and Z. R. Su, Fitoterapia, 2013, 84, 135–139 CrossRef.
- Y. C. Li, H. C. Liang, H. M. Chen, L. R. Tan, Y. Y. Yi, Z. Qin, W. M. Zhang, D. W. Wu, C. W. Li, R. F. Lin, Z. R. Su and X. P. Lai, Phytomedicine, 2012, 20, 77–83 CrossRef PubMed.
- Z. W. Tang, C. Peng, M. Dai and B. Han, Fitoterapia, 2015, 106, 41–45 CrossRef.
- S. Tariq, S. Wani, W. Rasool, K. Shafi, M. A. Bhat, A. Prabhakar, A. H. Shalla and M. A. Rather, Microb. Pathog., 2019, 134, 103580 CrossRef PubMed.
- A. Brochot, A. Guilbot, L. Haddioui and C. Roques, Microbiologyopen, 2017, 6, e00459 CrossRef.
- H. Lu, X. Wu, Y. Liang and J. Zhang, Chem. Pharm. Bull., 2006, 54, 936–940 CrossRef PubMed.
- G. S. Kim, D. H. Kim, J. J. Lim, J. J. Lee, D. Y. Han, W. M. Lee, W. C. Jung, W. G. Min, C. G. Won, M. H. Rhee, H. J. Lee and S. Kim, Biol. Pharm. Bull., 2008, 31, 2012–2017 CrossRef PubMed.
- T. Wang, W. Huang, Q. Duan, J. Wang, H. Cheng, J. Shao, F. Li and D. Wu, Mol. Biol. Rep., 2019, 46, 471–477 CrossRef PubMed.
- Y. L. Ma, Z. F. Han, J. R. Wang, L. M. Zhang, L. J. Qin, Y. Wang and C. X. Yang, Zhongcaoyao, 2001, 32, 334–337 Search PubMed.
- G. Haiyan, H. Lijuan, L. Shaoyu, Z. Chen and M. A. Ashraf, Saudi J. Biol. Sci., 2016, 23, 524–530 CrossRef PubMed.
- P. S. Yap, S. H. Lim, C. P. Hu and B. C. Yiap, Phytomedicine, 2013, 20, 710–713 CrossRef.
- R. Naveed, I. Hussain, A. Tawab, M. Tariq, M. Rahman, S. Hameed, M. S. Mahmood, A. B. Siddique and M. Iqbal, BMC Complementary Altern. Med., 2013, 13, 265 CrossRef PubMed.
- N. G. Vasconcelos, J. Croda and S. Simionatto, Microb. Pathog., 2018, 120, 198–203 CrossRef PubMed.
- S. M. Razavi Rohani, M. Moradi, T. Mehdizadeh, S. S. Saei-Dehkordi and M. W. Griffiths, LWT--Food Sci. Technol., 2011, 44, 2260–2265 CrossRef.
- P. Putnik, D. Gabrić, S. Roohinejad, F. J. Barba, D. Granato, K. Mallikarjunan, J. M. Lorenzo and D. Bursać Kovačević, Food Chem., 2019, 276, 680–691 CrossRef PubMed.
- S. Ankri and D. Mirelman, Microbes Infect., 1999, 1, 125–129 CrossRef.
- Y. Yamada and K. Azuma, Antimicrob. Agents Chemother., 1977, 11, 743–749 CrossRef CAS PubMed.
- J. Han, L. Lawson, G. Han and P. Han, Anal. Biochem., 1995, 225, 157–160 CrossRef CAS PubMed.
- A. Marchese, R. Barbieri, A. Sanches-Silva, M. Daglia, S. F. Nabavi, N. J. Jafari and S. M. Nabavi, Trends Food Sci. Technol., 2016, 52, 49–56 CrossRef CAS.
- Z. J. Shao, X. W. Zheng, T. Feng, J. Huang, J. Chen, Y. Y. Wu, L. M. Zhou, W. W. Tu and H. Li, Can. J. Physiol. Pharmacol., 2012, 90, 647–653 CrossRef CAS PubMed.
- M. Arifullah, N. D. Namsa, M. Mandal, K. K. Chiruvella, P. Vikrama and G. R. Gopal, Asian Pac. J. Trop. Biomed., 2013, 3, 604–610 CrossRef.
- C. M. Wu, J. L. Cao, M. H. Zheng, Y. Ou, L. Zhang, X. Q. Zhu and J. X. Song, J. Int. Med. Res., 2008, 36, 178–186 CrossRef CAS PubMed.
- D. M. Liu, J. C. Bi, H. Q. Qie, P. Jiao and P. L. Yan, J. Tradit. Chin. Med., 2008, 6, 654–655 Search PubMed.
- J. A. Jesus, J. H. Lago, M. D. Laurenti, E. S. Yamamoto and L. F. Passero, Evidence-Based Complementary Altern. Med., 2015, 2015, 620472 Search PubMed.
- A. M. Grudniak, A. Kurek, J. Szarlak and K. I. Wolska, Curr. Microbiol., 2011, 62, 1331–1336 CrossRef CAS PubMed.
- A. Kurek, A. M. Grudniak, M. Szwed, A. Klicka, L. Samluk, K. I. Wolska, W. Janiszowska and M. Popowska, Antonie van Leeuwenhoek, 2010, 97, 61–68 CrossRef CAS PubMed.
- G. S. Li, W. Zhang, T. Peng and M. J. Guo, Mod. Tradit. Chin. Med. Mater. Med. World Sci. Technol., 2014, 16, 610–613 Search PubMed.
- S. Xu, D. Li, L. Pei, H. Yao, C. Wang, H. Cai, H. Yao, X. Wu and J. Xu, Bioorg. Med. Chem. Lett., 2014, 24, 2811–2814 CrossRef CAS.
- H. Miladi, T. Zmantar, Y. Chaabouni, K. Fedhila, A. Bakhrouf, K. Mahdouani and K. Chaieb, Microb. Pathog., 2016, 99, 95–100 CrossRef CAS PubMed.
- H. Miladi, T. Zmantar, B. Kouidhi, Y. M. A. Al Qurashi, A. Bakhrouf, Y. Chaabouni, K. Mahdouani and K. Chaieb, Microb. Pathog., 2017, 112, 156–163 CrossRef CAS PubMed.
- D. S. Lee, S. H. Lee, J. G. Noh and S. D. Hong, Biosci., Biotechnol., Biochem., 1999, 63, 2236–2239 CrossRef CAS PubMed.
- J. Zhao, J. Lou, Y. Mou, P. Li, J. Wu and L. Zhou, Molecules, 2011, 16, 2259–2267 CrossRef CAS PubMed.
- W. Chen and Q. Z. Liu, Chin. J. Drug. Appl. Monit., 2007, 6, 38–39 Search PubMed.
- B. Marongiu, A. Piras, S. Porcedda, D. Falconieri, A. Maxia, M. A. Frau, M. J. Gonçalves, C. Cavaleiro and L. Salgueiro, Nat. Prod. Res., 2013, 27, 1521–1527 CrossRef CAS PubMed.
- K. Akilandeswari and K. Ruckmani, Cell. Mol. Biol., 2016, 62, 74–82 CrossRef CAS PubMed.
- Y. Morimoto, T. Baba, T. Sasaki and K. Hiramatsu, Int. J. Antimicrob. Agents, 2015, 46, 666–673 CrossRef CAS PubMed.
- S. C. Ricke, Poult. Sci., 2003, 82, 632–639 CrossRef CAS PubMed.
- F. Van Immerseel, J. B. Russell, M. D. Flythe, I. Gantois, L. Timbermont, F. Pasmans, F. Haesebrouck and R. Ducatelle, Avian Pathol., 2006, 35, 182–188 CrossRef CAS PubMed.
- J. Zhang, Z. G. Tian, J. H. Wang and A. R. Wang, Chin. J. Prev. Vet. Med., 2011, 3, 323–328 Search PubMed.
- J. Xiong, S. Li, W. Wang, Y. Hong, K. Tang and Q. Luo, Food Chem., 2013, 138, 327–333 CrossRef CAS PubMed.
- G. Li, X. Wang, Y. Xu, B. Zhang and X. Xia, Eur. Food Res. Technol., 2014, 238, 589–596 CrossRef CAS.
- S. N. Park, Y. K. Lim, M. H. Choi, E. Cho, I. S. Bang, J. M. Kim, S. J. Ahn and J. K. Kook, Curr. Microbiol., 2018, 75, 11–19 CrossRef CAS PubMed.
- M. J. Kim, C. Kim, J. Y. Park, Y. Lim, S. N. Park, S. Ahn and J. K. Kook, Int. J. Oral Sci., 2011, 36, 7–11 Search PubMed.
- C. M. Wang, Y. L. Jhan, S. J. Tsai and C. H. Chou, Molecules, 2016, 21 Search PubMed.
- S. Schmidt, K. Heymann, M. F. Melzig, S. Bereswill and M. M. Heimesaat, Planta Med., 2016, 82, 1540–1545 CrossRef CAS PubMed.
- D. R. Long, J. Mead, J. M. Hendricks, M. E. Hardy and J. M. Voyich, Antimicrob. Agents Chemother., 2013, 57, 241–247 CrossRef CAS PubMed.
- Y. Y. Lim and E. P. L. Quah, Food Chem., 2007, 103, 734–740 CrossRef CAS.
- B. C. Chan, X. Q. Han, S. L. Lui, C. W. Wong, T. B. Wang, D. W. Cheung, S. W. Cheng, M. Ip, S. Q. Han, X. S. Yang, C. Jolivalt, C. B. Lau, P. C. Leung and K. P. Fung, J. Pharm. Pharmacol., 2015, 67, 107–116 CrossRef CAS PubMed.
- K. P. Fung, Q. B. Han, M. Ip, X. S. Yang, C. B. Lau and B. C. Chan, Hong Kong Med. J., 2017, 23(Suppl 5), 38–42 Search PubMed.
- M. G. Aly and N. M. Afr, J. Biotechnol., 2011, 10, 12058–12063 Search PubMed.
- J. Wang, H. Zhao, W. Kong, C. Jin, Y. Zhao, Y. Qu and X. Xiao, Phytomedicine, 2010, 17, 684–689 CrossRef CAS PubMed.
- J. Azelmat, J. F. Larente and D. Grenier, Arch. Oral Biol., 2015, 60, 342–346 CrossRef CAS PubMed.
- Y. Y. Hou, Master thesis, Shanghai Ocean University, 2015.
- Y. Wu, X. Wang, B. Shen, L. Kang and E. Fan, Recent Pat. Food, Nutr. Agric., 2013, 5, 57–61 CrossRef CAS PubMed.
- S. K. Sharma, N. Gautam and N. S. Atri, BMC Complementary Altern. Med., 2015, 15, 446 CrossRef PubMed.
- Y. Zhang, Y. T. Wu, W. Zheng, X. X. Han, Y. H. Jiang, P. L. Hu and L. E. Shi, J. Funct. Foods, 2017, 38, 273–279 CrossRef CAS.
- G. Y. Zuo, G. C. Wang, Y. B. Zhao, G. L. Xu, X. Y. Hao, J. Han and Q. Zhao, J. Ethnopharmacol., 2008, 120, 287–290 CrossRef CAS PubMed.
- T. T. Zhang, J. H. Pan, L. W. Nie, B. J. Wu, S. S. Zhao and Y. Yang, J. Biol., 2005, 22, 41–42 Search PubMed.
- S. Lee, D. S. Shin, J. S. Kim, K. B. Oh and S. S. Kang, Arch. Pharmacal Res., 2003, 26, 449–452 CrossRef CAS PubMed.
- Q. Xie, Rec. Nat. Prod., 2015, 10, 294–306 Search PubMed.
- P. Ouyang, J. Chen, M. Sun, Z. Yin, J. Lin, H. Fu, G. Shu, C. He, C. Lv, X. Deng, K. Wang, Y. Geng and L. Yin, Antonie van Leeuwenhoek, 2016, 109, 915–922 CrossRef CAS PubMed.
- L. M. Liu, R. H. Wang, L. Chen, Q. Yang, X. G. Weng and J. H. Sun, J. Tradit. Chin. Med., 2009, 16, 39–42 Search PubMed.
- S. H. Duncan, H. J. Flint and C. S. Stewart, FEMS Microbiol. Lett., 1998, 164, 283–288 CrossRef CAS.
- S. Liu, K. P. Xie, D. Zou, L. Zhou and M. J. Xie, Chin. J. Microecol., 2014, 26, 1123–1126 CAS.
- H. Wang, D. Zou, K. Xie and M. Xie, Mol. Med. Rep., 2014, 10, 2341–2345 CrossRef CAS.
- M. Saleem, H. J. Kim, M. S. Ali and Y. S. Lee, Nat. Prod. Rep., 2005, 22, 696–716 RSC.
- S. Y. Yan, S. Q. Lin, J. Fu, G. M. Li, D. J. Wang and R. Z. Wan, Chin. J. Exp. Tradit. Med. Formul., 2014, 20, 142–146 Search PubMed.
- Y. J. Feng, Y. Y. Zhang, R. X. Wang and S. G. Li, Food. Ferment. Ind., 2016, 42, 72–76 CAS.
- H. Choi, J. Lee, Y. S. Chang, E. R. Woo and D. G. Lee, Biochim. Biophys. Acta, 2013, 1828, 2002–2006 CrossRef CAS.
- B. Hwang, J. Lee, Q. H. Liu, E. R. Woo and D. G. Lee, Molecules, 2010, 15, 3507–3516 CrossRef CAS PubMed.
- K. C. Chiu, Y. H. Shih, T. H. Wang, W. C. Lan, P. J. Li, H. S. Jhuang, S. M. Hsia, Y. W. Shen, M. Yuan-Chien Chen and T. M. Shieh, J. Formosan Med. Assoc., 2021, 120, 827–837 CrossRef CAS PubMed.
- Y. J. Hu, J. L. Qiao, X. Zhang and C. R. Ge, J. Food Biochem., 2011, 35, 425–441 CrossRef CAS.
- B. Chang, Y. Lee, Y. Ku, K. Bae and C. Chung, Planta Med., 1998, 64, 367–369 CrossRef CAS PubMed.
- J. Feng, J. Y. Li and X. D. Zhou, Sichuan Daxue Xuebao, Yixueban, 2007, 38, 456–458 CAS.
- Y. Hu, J. Qiao, X. Zhang and C. Ge, J. Sci. Food Agric., 2011, 91, 1050–1056 CrossRef CAS.
- C. S. Zuo, Chin. J. Vet. Med., 2015, 51, 54–56 Search PubMed.
- S. M. Zheng, J. J. Huang, W. U. Qing and S. Sha, Acta Hydrobiol. Sin., 2010, 34, 57–64 CrossRef CAS.
- X. Wang, Y. Z. Cui and T. S. Han, China J. Tradit. Chin. Med. Pharm., 2009, 37, 169–171 Search PubMed.
- D. L. Zhang, D. F. Tang, Q. Zheng, M. Yang, D. Liu and Y. Tang, Zhongcaoyao, 2015, 46, 3771–3778 CAS.
- Y. Chen, L. J. Hu and T. T. Wang, World Chin. Med., 2018, 10, 2449–2452 Search PubMed.
- X. U. Peng, China Mod. Med., 2018, 33, 65–67 Search PubMed.
- P. Xu, China Mod. Med., 2013, 9, 84–85 Search PubMed.
- D. M. Ma, Q. C. Tao and H. W. Qi, Lab. Med., 2018, 9, 1091–1094 Search PubMed.
- X. Y. Liang, H. Y. Zhu, X. M. Liu and M. Pan, Lab. Med., 2015, 3, 261–264 Search PubMed.
- J. Feng, Y. H. Wei, S. X. Zhou, L. L. Jiang and L. Wang, Chinese J. Vet. Drug, 2017, 51, 30–35 Search PubMed.
- Y. F. Luan, X. S. Kong, J. Wang and Y. Q. Zhang, J. Tradit. Chin. Med., 2011, 11, 810–812 Search PubMed.
- X. Y. Zhu, C. Peng and M. Dai, J. Sichuan Tradit. Chin. Med., 2014, 4, 62–65 Search PubMed.
- C. J. Yuan, F. G. Lu and Y. W. Zhu, J. Tradit. Chin. Med., 2005, 10, 60–61 Search PubMed.
- X. Chen, L. Hu, H. Wu, W. Liu, S. Chen, A. Zhou and Y. Liu, Evidence-Based Complementary Altern. Med., 2018, 2018, 6810369 Search PubMed.
- X. Y. Zhi, E. H. Cui, Y. P. Fan, W. R. Ma, Y. Y. Xu, L. Z. X. Suo and X. P. Song, Xibei Nongye Xuebao, 2014, 23, 114–119 Search PubMed.
- X. Wang, Y. Z. Cui, H. Shao and T. S. Han, J. Northeast Agric., 2011, 42, 115–118 CrossRef CAS.
- L. J. Hu, W. Liu, H. H. Wu, L. Li and A. J. Zhou, Mod. Chin. Med., 2016, 18, 307–311 Search PubMed.
- D. M. Ma, Q. C. Tao, D. K. Jiang and Y. Y. Zhang, Internet J. Lab. Med., 2020, 9, 1052–1055+1059 Search PubMed.
- V. Kuete and T. Efferth, Front. Pharmacol., 2010, 1, 123 Search PubMed.
- Y. Liu, Y. Zhao, D. L. Guo, W. W. Liu and Y. X. Liu, Chin. Herb. Med., 2017, 9, 353–357 CrossRef.
- N. Küükboyaci, S. Zkan, N. Adigüzel and F. Tosun, Turk. J. Biol., 2011, 35, 379–385 Search PubMed.
- B. A. Bhat, W. R. Mir, B. A. Sheikh, M. A. Rather, T. U. H. Dar and M. A. Mir, J. Ethnopharmacol., 2022, 291, 115046 CrossRef CAS PubMed.
- R. De, P. Kundu, S. Swarnakar, T. Ramamurthy, A. Chowdhury, G. B. Nair and A. K. Mukhopadhyay, Antimicrob. Agents Chemother., 2009, 53, 1592–1597 CrossRef CAS PubMed.
- N. Niamsa and C. Sittiwet, J. Toxicol. Pharmacol., 2009, 4, 173–177 CrossRef.
- G. N. Chen, F. L. Sun, Y. R. Yan and M. M. Liu, ChemBioEng., 2015, 32, 34–37 CAS.
- M. Miski, A. Ulubelen, C. Johansson and T. J. Mabry, J. Nat. Prod., 1983, 46, 874–875 CrossRef CAS PubMed.
- P. Liu, G. H. Chen, S. H. Deng, Y. L. Liu and J. M. Tong, Chin. J. Exp. Tradit. Med. Formul., 2013, 19, 207–210 CAS.
- R. F. H. André, P. G. S. Erasmo, L. Francisca, S. G. C. Anabela, R. Alyne and C. L. Eliana, LWT--Food Sci. Technol., 2021, 139, 110521 CrossRef.
- X. W. Liu, Y. J. Yang, Z. Qin, S. H. Li, L. X. Bai, W. B. Ge and J. Y. Li, Front. Pharmacol., 2022, 13, 914188 CrossRef CAS PubMed.
- N. Benkeblia, LWT--Food Sci. Technol., 2004, 37, 263–268 CrossRef CAS.
- S. Y. Liu, M. X. Zhang, Y. H. Wu and X. H. Yang, J. Northeast Norm. Univ., 2011, 43, 93–96 CAS.
- Y. Sekita, K. Murakami, H. Yumoto, H. Mizuguchi, T. Amoh, S. Ogino, T. Matsuo, Y. Miyake, H. Fukui and Y. Kashiwada, Biosci., Biotechnol., Biochem., 2016, 80, 1205–1213 CrossRef CAS PubMed.
- J. Eun-Kyung, J. Bacteriol. Virol., 2009, 39, 61–69 CrossRef.
- M. Omidbeygi, M. Barzegar, Z. Hamidi and H. Naghdibadi, Food Control, 2007, 18, 1518–1523 CrossRef CAS.
- G. L. Petretto, F. Fancello, S. Zara, M. Foddai, N. P. Mangia, M. L. Sanna, E. A. Omer, L. Menghini, M. Chessa and G. Pintore, J. Food Sci., 2014, 79, M369–M377 CrossRef CAS PubMed.
- D. Beatovic, D. Krstic-Milosevic and S. Trifunovic, Rec. Nat. Prod., 2015, 9, 62–75 Search PubMed.
- M. Hassanshahian, Z. Bayat, S. Saeidi and Y. Shiri, Int. J. Biomed. Adv. Res., 2014, 2, 18–24 CAS.
- A. M. Mahmoud, R. M. A. El-Baky, A. B. F. Ahmed and G. F. M. Gad, Am. J. Microbiol. Res., 2016, 4, 16–25 CAS.
- R. A. Momin and M. G. Nair, J. Agric. Food Chem., 2001, 49, 142–145 CrossRef CAS PubMed.
- K. Díaz, L. Espinoza, A. Madrid, L. Pizarro and R. Chamy, Evidence-Based Complementary Altern. Med., 2018, 2018, 2706417 Search PubMed.
- S. Soltani, A. Shakeri, M. Iranshahi and M. Boozari, Iran. J. Pharm. Res., 2021, 20, 268–285 CAS.
- H. Yang, Y. Gao, L. Long, Y. Cai, J. Liao, J. Peng and L. Wang, Arch. Microbiol., 2021, 203, 3981–3988 CrossRef CAS PubMed.
- T. A. Mohamed, A. A. Abd El Aty, A. A. Shahat, N. S. Abdel-Azim, K. A. Shams, A. A. Elshamy, M. M. Ahmed, S. H. H. Youns, T. M. El-Wassimy, S. A. El-Toumy and M. F. Hegazy, Nat. Prod. Res., 2021, 35, 1959–1967 CrossRef CAS PubMed.
- M. Popa, L. Mărutescu, E. Oprea, C. Bleotu, C. Kamerzan, M. C. Chifiriuc and G. Grădisteanu Pircalabioru, Antibiot., 2020, 9, 428 CrossRef CAS PubMed.
- A. Borges, C. Ferreira, M. J. Saavedra and M. Simões, Microb. Drug Resist., 2013, 19, 256–265 CrossRef CAS PubMed.
- G. H. Cao, Z. D. Li, R. H. Zhao, Q. R. Zhang, J. B. Li, Z. W. He, K. Kang and S. He, Food. Sci. Technol., 2017, 42, 202–206 Search PubMed.
- Y. Kong, Y. J. Fu, Y. G. Zu, F. R. Chang, Y. H. Chen, X. L. Liu and H. M. Schiebel, Food Chem., 2010, 121, 1150–1155 CrossRef CAS.
- Y. M. Wang and H. J. Cheng, Mod. Chin. Med., 2013, 15, 950–953 Search PubMed.
- Z. X. Li, X. H. Wang, J. H. Zhao, J. F. Yang and X. Wang, J Tradit Chin Med, 2007, 24, 328–331 Search PubMed.
- J. N. Kabera, E. Semana, A. R. Mussa and X. He, J. Pharm. Pharmacol., 2014, 2, 377–392 Search PubMed.
- K. S. Allemailem, J. Pharm. BioAllied Sci., 2021, 13, 155–162 CAS.
- U. Anand, N. Jacobo-Herrera, A. Altemimi and N. Lakhssassi, Metabolites, 2019, 9, 258 CrossRef CAS PubMed.
- M. F. Haroun and R. S. Al-Kayali, Iran. J. Basic Med. Sci., 2016, 19, 1193–1200 Search PubMed.
- M. Park, L. Horn, V. Lappi, D. Boxrud, C. Hedberg and B. Jeon, Pathogens, 2022, 11 Search PubMed.
- S. Hossain, M. Yousaf, Y. Liu, D. Chang and X. Zhou, Front. Pharmacol., 2022, 13, 876183 CrossRef CAS PubMed.
- J. E. Lan, X. J. Li, X. F. Zhu, Z. L. Sun, J. M. He, M. Zloh, S. Gibbons and Q. Mu, Nat. Prod. Res., 2021, 35, 1881–1886 CrossRef CAS PubMed.
- F. J. Álvarez-Martínez, E. Barrajón-Catalán, M. Herranz-López and V. Micol, Phytomedicine, 2021, 90, 153626 CrossRef PubMed.
- T. Li, P. Wang, W. Guo, X. Huang, X. Tian, G. Wu, B. Xu, F. Li, C. Yan, X. J. Liang and H. Lei, ACS Nano, 2019, 13, 6770–6781 CrossRef CAS PubMed.
- M. O. Osungunna, J. Microbiol., Biotechnol. Food Sci., 2020, 9, 727–735 CrossRef CAS.
- F. J. Álvarez-Martínez, E. Barrajón-Catalán and V. Micol, Biomedicines, 2020, 8, 405 CrossRef PubMed.
Footnote |
† These authors contributed equally to this article. |
|
This journal is © The Royal Society of Chemistry 2022 |
Click here to see how this site uses Cookies. View our privacy policy here.