DOI:
10.1039/D2RA05030G
(Paper)
RSC Adv., 2022,
12, 32061-32069
Characterization and antibacterial behavior of an edible konjac glucomannan/soluble black tea powder hybrid film with ultraviolet absorption
Received
11th August 2022
, Accepted 26th October 2022
First published on 9th November 2022
Abstract
In this study, a KGM/SBTP film was prepared by a blending method using KGM and a soluble black tea film (SBTP) as substrates, and its hygroscopicity, thermal properties, light barrier properties, microstructure, and bacteriostatic properties were evaluated. The results confirmed that compared with the control group, with the increase in the SBTP content, the transmittance of the film in the ultraviolet region significantly reduced, and the water barrier property and thermal stability were improved. Fourier transform infrared spectroscopy (FTIR) and scanning electron microscopy (SEM) results indicated that the tea polyphenols interacted with the film substrate. SEM also showed that the structure of the KGM/SBTP films was smooth and flat, and all samples showed no fracture. In addition, the KGM/SBTP mixed membrane had obvious concentration-dependent antibacterial activity. When the concentration of SBTP was 0.9%, the inhibition zones against Staphylococcus aureus and Escherichia coli were 12.30 ± 0.20 mm and 12.05 ± 0.47 mm, respectively.
1. Introduction
Recently, food packaging safety has recently received increasing attention, and non-degradable and potentially toxic additives are harmful to people's health. Active food packaging has emerged as a novel approach to improving and maintaining food freshness, as well as reducing waste and chemicals used in packaging.1
Today, non-biodegradable plastics or plastics obtained from non-renewable sources are extremely polluting the environment. Scientists are looking for biodegradable, eco-friendly biomaterials that can replace plastic. The joint efforts of materials science, organic chemistry, physics and other disciplines have made the development of biomaterials possible. Among them, thin film materials with bacteriostatic properties have become a research hotspot because of their good application prospects in food packaging.2 Bio-based materials made from natural polysaccharides have environmental friendliness properties.3 For example, konjac glucomannan (KGM) has attracted considerable attention owing to its abundant resources, easy availability and low cost. The application research of konjac glucomannan has also been widely used in cosmetics, medicine and other fields, including food and food additives.4 KGM also has applications in packaging because of its excellent biocompatibility, gelation, film-forming ability and biodegradability. Therefore, konjac glucomannan-based (KGM-based) films are new alternative materials for solving the white problem.5 However, pure KGM films have several disadvantages, such as their moisture resistance, stability, antioxidant and antibacterial activities are poor.6 Therefore, they are easily damaged and become obstacles in the manufacture of multifunctional food packaging materials. Studies have shown that some of the beneficial properties of KGM films do not necessarily lead to an increase in bioactivity and that the utility of KGM-based films is mainly enhanced by the addition of functional fillers. For example, plant essential oils, plant polyphenols and other natural active substances or nanoparticles are usually used to produce active films.7,8 Recently, researchers have used anthocyanins in smart product packaging. Owing to their high content of polyphenolic compounds, they exhibit excellent antibacterial and antioxidant activity.9
Black tea contains thousands of biologically active substances, such as polyphenols, amino acids, volatile compounds, and alkaloids, which have pharmacological properties.10 Black tea has become a hot topic in the field of nutrition and medical research in Western countries because of its key therapeutic properties, particularly theaflavins (TFS) and thearubigins (TRS), which are more stable and have direct effects on organisms in other plants.11 Furthermore, the polysaccharides extracted from black tea exhibited the highest DPPH and hydroxyl radical scavenging activities.12 Although the physicochemical and antibacterial properties of green tea powder and polysaccharide blend films have been investigated, there are few studies on alternatives to black tea powder. Gao et al. studied polysaccharide films formed by pectin, chitosan, and tea polyphenols, which showed significant sustained-release, antioxidant, and preservative activities, and these activities were proportional to their concentrations.13 Yan et al. developed a new composite film by mixing different ratios and concentrations of KGM and Pullulan and found that it improved the quality of strawberries during storage.14 In this study, SBTP is introduced into KGM-based blended films for the first time, and its effects on the physical properties, structure and antibacterial functions of the films are investigated, and finally different functional requirements are achieved.
2. Materials and methods
2.1. Materials
Soluble black tea powder (tea polyphenol ≥ 25%, caffeine ≥ 4.5%) purchased from Damin Food (Zhang zhou) Co., Ltd, was of food grade. KGM was purchased from Hubei Yizhi Konjac Industrial Co., Ltd, was of analytical grade. Glycerin and other reagents were of analytical grade and were obtained from commercial channels (China). All the solutions used in the experiments were prepared using ultrapure water through a Millipore (Millipore, Milford, MA, USA) Milli-Q water purification system.
2.2. Preparation of film solutions and film formation
A 1% (W/V) solution of KGM was prepared and stirred at room temperature for 3 hours. Next, glycerol (0.2, W/V) was added to the KGM solution, and then SBTP was added to the KGM solution to obtain different concentrations of SBTP (0%, 0.3%, 0.6%, and 0.9% W/V). The mixed solution was stirred for 2 h and filtered through 200 mesh nylon to remove impurities. To remove air bubbles, the filtered solution was centrifuged in a centrifuge at 5000 rpm for 15 min. Then, 80 g of the film-forming solution was poured into a flat-bottomed plastic tray (16 cm × 12 cm) and placed horizontally for 2 h. Finally, the solution was placed in a 50 °C oven with vacuum for 24 h for drying. The finished film was stored in a constant temperature and humidity device (25 °C, 53% relative humidity) for 48 h.
2.3. Color measurement
The CIE colorimetric system was used for color determination, and color parameters were measured using a colorimeter (Minolta Co. Ltd). The results were represented as L*, a*, and b*, representing brightness, red and green, and yellow and blue, respectively. Instrumental measurements were standardized on white and black calibration plates. Each sample is measured 5 times in parallel, and the calculation formula for Whiteness is given as follows:
2.4. Hygroscopic properties
The blend films were cut to a size of 40 mm × 40 mm and placed in Petri dishes. It was then put into a vacuum drying oven (60 °C) to reach a constant weight, and the resulting mass was the weight (W0) of the edible composite dry film. The blend films were placed in a temperature (25 °C, 100%) and relative humidity environment and weighed periodically over approximately 4 days to obtain their final weights (W1). Hygroscopicity is calculated as follows:15
where W is hygroscopicity, W0 is the initial sample weight (g), and W1 is the final weight (g). Three parallel determinations for each sample were averaged.
2.5. Light transmittance and transparency values
The films were cut into 10 mm × 25 mm rectangles and placed in cuvettes, and the transmission spectra of the films in the 200–800 nm range were measured using a UV-vis spectrophotometer (PerkinElmer, USA).
2.6. FT-IR analysis
The FT-IR spectra of the films were recorded using an FT-IR spectrometer (PerkinElmer, USA) from 4000 to 400 cm−1 with a resolution of 4 cm−1.
2.7. Thermal analysis
KGM/SBTP composite films were performed using TGA (SDT-Q600, TA Instruments, USA) with a sample weight of approximately 7–13 mg, from 20 to 550 °C at a rate of 10 °C min−1 under flowing nitrogen.
2.8. Film microstructure
The microstructure of KGM/SBTP films was observed using a scanning electron microscope SEM (Hitachi S-4800, Japan). The dried samples were mounted on copper supports and sputter-coated with gold and observed under an acceleration voltage of 3.0 kV of SEM.
2.9. Antibacterial performance
The antibacterial activity of the composite films was tested using diffusion method. The test strains were Staphylococcus aureus and Escherichia coli. SBTP films were first perforated using a punch (6 mm) and then placed in separate Petri dishes containing different bacteria and incubated for 24 h at 37 °C. The diameter of the inhibition zone around the discs was measured using a micrometer and each test was repeated three times.
2.10. Statistical analysis
Statistical analysis and graphing were performed using Origin 2021 software. Data were statistically analyzed with the statistical software SPSS 22 using the Duncan's multiple range test at the P < 0.05 level of significance. All data are expressed as mean ± standard deviation (SD).
3. Results and discussion
3.1. Analysis of film whiteness
The color of edible films has become a major choice for consumer acceptance. It can be observed from Table 1 that as the SBTP concentration increased, the L* value decreased probably because the internal structure network of the blend film became tighter, and the transmittance of the system weakened. The larger a* value indicated that the heating process clearly affected the color characteristics of the films, and the colorless film turned yellow and dark brown after heat treatment.16 The color represented by the L*, a*, and b* values changed significantly at 0.3% SBTP concentration, with a small change from 0.6 to 0.9%, which was consistent with the change in the Whiteness of the film (Fig. 1). The darker color may be due to the higher ash content of KGM and the browning of impurities, or the Maillard reaction between the amino group in SBTP and the carbonyl group in the KGM molecule.
Table 1 Color determination value of composite filma
SBTP concentration |
0% |
0.1% |
0.3% |
0.6% |
0.9% |
All measurements were performed at 25 °C and 53% RH. Data were displayed as means ± SD. Significant differences p ≤ 0.05. |
L* |
37.82 ± 1.70a |
35.87 ± 1.01a |
30.44 ± 1.01b |
27.59 ± 1.45bc |
24.86 ± 3.41c |
a* |
−0.11 ± 0.05d |
1.63 ± 0.06c |
4.82 ± 1.06b |
7.01 ± 0.65a |
7.07 ± 1.00a |
b* |
−0.37 ± 0.07c |
7.20 ± 0.11b |
10.40 ± 0.36a |
6.29 ± 0.70b |
6.68 ± 0.88b |
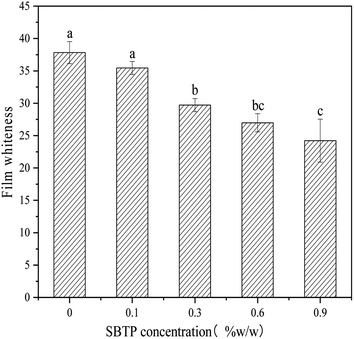 |
| Fig. 1 The Whiteness of KGM/SBTP composite Films. | |
3.2. Water absorption behavior
Water solubility is a key characteristic of edible films. When the film was eaten with food, proper water solubility was beneficial for expanding its application range. In other food applications, the insolubility of the film increased the water resistance of the package and prevented the film from disintegrating in the process of covering wet food surfaces, thereby improving product integrity.17,18 Fig. 2a shows the hygroscopicity of KGM/SBTP blends, particularly for pure KGM films. The hygroscopicity of the blends increased significantly with the storage time, probably because KGM is a typical natural hydrocolloid. Its independent and substantial presence in pure KGM membranes provided additional binding sites for water molecules. It can be observed from Fig. 2b that the overall water absorption rate and adsorption amount of the previous film are presented in three stages. In the first stage (0–21 h), the moisture absorption rate of all blend films was the largest, and the surface of KGM/SBTP blend films quickly absorbed moisture and began to swell. At this time, the content of SBTP had little effect on the hygroscopic ability of the film. In the second stage (21–80 h), the moisture absorption capacity and moisture absorption rate of films with different SBTP contents increased slowly with time. When the SBTP content changed from 0.3% to 0.9%, the hygroscopic ability of the film was obviously weakened. In the third stage, the hygroscopic capacity reached saturation in about 80 h, indicating that the hygroscopic capacity had basically been lost.
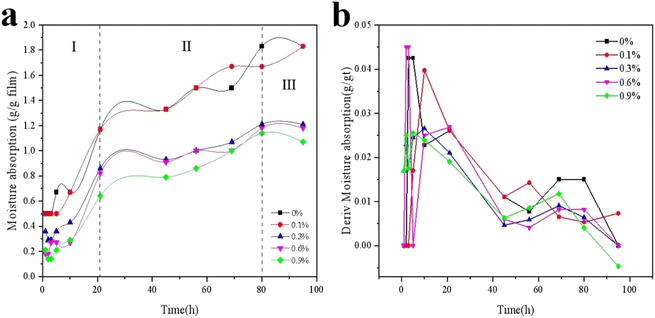 |
| Fig. 2 Water absorption behavior (a) and differential adsorption content (b) of KGM/SBTP composite films at 100% RH and 25 °C. | |
3.3. Light transmittance analysis
Hybrid biofilms are ideal for packaging applications. Fig. 3a shows that compared with the pure KGM film, the addition of SBTP to the KGM-based edible film reduced the transmittance of all wavelengths, which could indicate a synergistic effect between the two substances in the composite film. In particular, with the SBTP concentrations of 0.6 and 0.9%, the transmittance declined to almost zero in the UV range from 200 to 380 nm. The low transmittance of UV radiation has been highly valued; it effectively protected food from oxidative deterioration caused by UV light, discoloration, loss of nutrients and off-flavors.19 Relatively, packaging with high transparency in the visible light region was required because it provided consumers with visual control over the condition of the goods. The requirements for the spectral characteristics of packaging materials were quite complex.20 As can be observed from Fig. 3b, the high concentration of SBTP films in the visible light region had lower transparency, which may be due to the high content of polyphenols in SBTP. Simultaneously, films with lower transmittance were suitable for storing light-sensitive foodstuffs and had practical applications.21
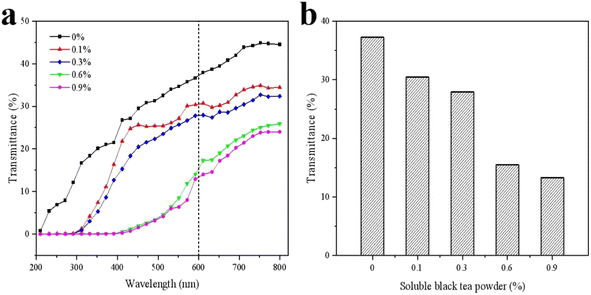 |
| Fig. 3 Transmittance (a) and 600 nm (b) of KGM/SGTP mixed films from 200 to 800 nm. | |
3.4. Fourier transform infrared (FTIR) analysis
Fig. 4 illustrates that FTIR spectroscopy was used to characterize the functional groups of KGM/SBTP blend films to study the interaction between the matrix molecules of the hybrid film samples. In the fingerprint region (800–1250 cm−1), all samples exhibited typical bands and peaks as the characteristic of polysaccharides. The peak at about 3309 cm−1 corresponds to the stretching vibration of O–H. The peak at 2921 cm−1 was attributed to the C–H group stretching vibration.22A A polysaccharide hydration vibration absorption peak was found at 1643 cm−1, and 1023 cm−1 was the variable-angle vibration absorption peak of the alcohol hydroxyl group.23 As the concentration of SBTP in the hybrid film increased, the peaks in the range of 1510–1650 cm−1 were biased towards larger wavenumbers, which marked the N–H and amide I band, probably because of the trace amount of protein contained in SBTP. The C–C stretching vibration at 1100–1500 cm−1 was due to the presence of tea polyphenols, which was also reported in our previous study. The shift of the broadband around 3309 cm−1 to lower wavenumbers was mainly attributed to the formation of intermolecular hydrogen bonds.24 The research showed that the strong hydrogen bond between phenolic compounds and polysaccharides reduced the original chemical bond force constants between polysaccharides, resulting in the absorption frequency moving in the direction of a low wavenumber.25
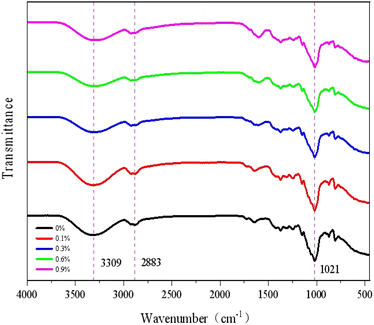 |
| Fig. 4 FT-IR spectra of KGM/SBTP composite films. | |
3.5. Thermal properties analysis
TG was used to study the thermal decomposition of the samples. The first phase (20–130 °C) was due to the loss of free and bound water (physical removal of water). The second stage (130–400 °C) was associated with thermal degradation or carbohydrate polymer degradation of the film samples.26 When the temperature was 450 °C, the retained mass reached 22.34%, 21.66%, 26.70%, 31.51% and 33.81% respectively. As can be observed from Fig. 5a, the initial decomposition temperature of the KGM/SBTP film was higher, indicating that the material had good thermal stability, which was consistent with the tighter network structure of the film and the enhanced hydrogen bond interaction. The area size and shift of the endothermic weight loss peak in the previous DTG were related to weight loss (Fig. 5b). In the range of 250–350 °C, with the increase in the SBTP concentration, the weight loss gradually decreased and was accompanied by the migration of the endothermic peak.27 The maximum degradation temperature of KGM/SBTP blend film was 311.8 °C, 290.2 °C, 285.1 °C, 264.7 °C and 261.5 °C, which also indicated that the material had good thermal stability. A higher degradation rate indicated higher thermal sensitivity.28
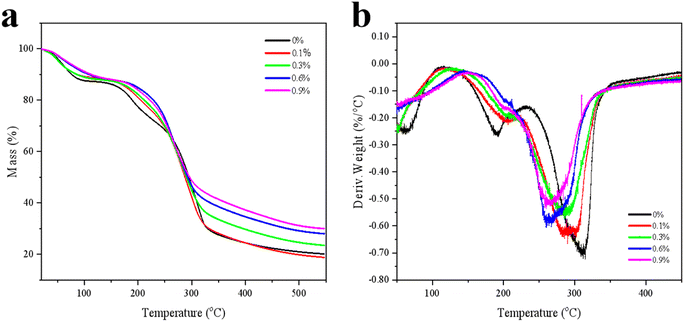 |
| Fig. 5 Effect of SBTP concentration on the TGA (a) and DTG (b) of KGM/SBTP hybrid films. | |
3.6. SEM analysis
The microstructure was investigated by employing scanning electron microscopy (SEM), as shown in Fig. 6. The surfaces of pure KGM film and films with 0.1 and 0.3% SBTP content were relatively smooth and homogeneous, respectively. However, when the SBTP concentration was 0.6%, there were more punctate protrusions on the surface of the film. At a maximum SBTP concentration of 0.9%, these punctate protrusions linked into a ring surrounding the small particulate matter, and a certain trend towards phase separation was observed. This might be due to the obvious interaction and adhesion at the interface between SBTP and KGM chains, as well as the aggregation of high concentrations of SBTP in the KGM matrix.29 However, no fracture occurred in all samples, indicating that SBTP and KGM had high miscibility. Therefore, there was an interaction between the two water-soluble polymers, which resulted in different miscibility states and microstructures of the mixtures.30
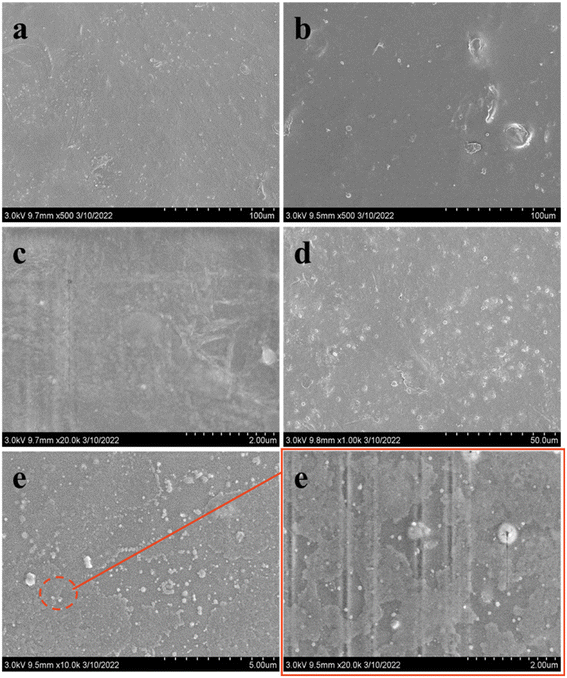 |
| Fig. 6 SEM images of KGM/SBTP films, SBTP content (a 0%, b 0.1%, c 0.3%, d 0.6% and e 0.9%). | |
3.7. Antimicrobial activities of KGM/SBTP composite films
Antibacterial property is an important requirement for food packaging materials. Tea polyphenols have good antibacterial, antioxidant and anticancer effects, so they have attracted considerable attention as a green food additive. In addition, its antibacterial effect is more stable than that of other natural antibacterial ingredients. Theaflavins in black tea have broad-spectrum antibacterial properties and have been shown to be effective both in vitro and in vivo.31 We used the diffusion method to study the antibacterial bioactivity of KGM/SBTP blend films. The results are shown in Fig. 7. Pure KGM film had no antibacterial activity against Escherichia coli and Staphylococcus aureus.32 The zones of inhibition for E. coli and S. aureus were 6.96 ± 0.37 mm and 8.76 ± 0.45 mm for the films with 0.3% SBTP content, respectively. When the SBTP content reached 0.9%, the values increased to 12.05 ± 0.47 mm and 12.30 ± 0.20 mm. Therefore, the antibacterial activity of KGM/SBTP films largely depended on the concentration of SBTP. As the SBTP content increased, the antibacterial activity of the KGM/SBTP film against the two kinds of bacteria was more obvious, and the size of the inhibition zone also increased significantly. Black tea had a stronger inhibitory effect on Gram-positive bacteria, which may be due to the various compositions and structures of the cell walls of the two bacteria. Black tea was not as bacteriostatic as green tea because of its lower polyphenol content. Furthermore, Cui et al. showed that S. aureus was more sensitive to polyphenols than E. coli.33
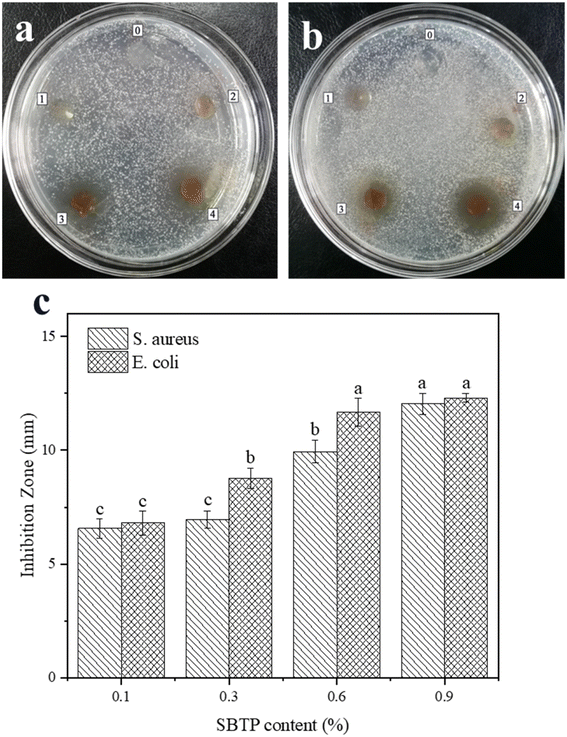 |
| Fig. 7 Antibacterial effects of KGM and SBTP composite film against Staphylococcusaureus (a) and Escherichia coli (b) and the size of the inhibition zone (c). 0 pure KGM film, 1–4 KGM/SBTP films with SBTP 0.1%, 0.3%, 0.6% and 0.9%. Different letters represent significant differences in bacterial species and antibacterial areas under the same SBTP concentration in the hybrid films. | |
4. Conclusions
In this study, a konjac glucomannan-based edible film (KGM) was loaded with various concentrations of SBTP. Based on the physicochemical properties, as the SBTP concentration increases, the mixed film can meet the needs of food light protection and consumers for the appearance and color of the edible film, and its thermal stability and hygroscopicity were regulated. When the concentration of SBTP was 0.9%, there was no damage or fracture, except for the appearance of band-like imprints, and it also showed good antibacterial activity against Gram-positive bacteria. In conclusion, the increase in the SBTP concentration significantly improved the physical properties and antibacterial activity of the KGM-based films. Therefore, the developed KGM/SBTP edible film has great potential as a multifunctional food bioactive packaging material.
Conflicts of interest
No conflict of interest exists in the submission of this manuscript, and manuscript is approved by all authors for publication. All the authors listed have approved the manuscript that is enclosed.
Acknowledgements
This work was financially supported by the National Natural Science Foundation of China (Grant No. U2004160 and No. 31701647), Natural Science Foundation of Henan province (Grant No. 162300410229), University Student Scientific Research Projects of Xinyang Normal University (Grant No. 2021-DXS-114), Innovation and Entrepreneurship Training Programs for College students in Henan Province (Grant No. S202110477040), and Nanhu Scholars Program for Young Scholars of XYNU. This research was also kindly supported by the Analysis & Testing Center of Xinyang Normal University.
References
- J. T. Sánchez, A. V. García, A. Martínez-Abad, F. Vilaplana, A. Jiménez and M. C. Garrigós, Physicochemical and functional properties of active fish gelatin-based edible films added with Aloe vera gel, Foods, 2020, 9, 1248 CrossRef PubMed.
- X. Li, Z. Ren, R. Wang, L. Liu, J. Zhang, F. Ma, M. Z. H. Khan, D. Zhao and X. Liu, Characterization and antibacterial activity of edible films based on carboxymethyl cellulose, Dioscorea opposita mucilage, glycerol and ZnO nanoparticles, Food Chem., 2021, 349, 129208 CrossRef CAS PubMed.
- A. C. Boccia, G. Scavia, I. Schizzi and L. Conzatti, Biobased Cryogels from Enzymatically Oxidized Starch: Functionalized Materials as Carriers of Active Molecules, Molecules, 2020, 25, 2557 CrossRef CAS.
- Z. Gao, R. Su, R. Huang, W. Qi and Z. He, Glucomannan-mediated facile synthesis of gold nanoparticles for catalytic reduction of 4-nitrophenol, Nanoscale Res. Lett., 2014, 9, 1–8 CrossRef CAS PubMed.
- Y. Ni, Y. Liu, W. Zhang, S. Shi, W. Zhu, R. Wang, L. Zhang, L. Chen, J. Sun and J. Pang, Advanced konjac glucomannan-based films in food packaging: Classification, preparation, formation mechanism and function, LWT-Food Sci. Technol., 2021, 152, 112338 CrossRef CAS.
- N. Zhou, L. Wang, P. You, L. Wang, R. Mu and J. Pang, Preparation of pH-sensitive food packaging film based on konjac glucomannan and hydroxypropyl methyl cellulose incorporated with mulberry extract, Int. J. Biol. Macromol., 2021, 172, 515–523 CrossRef CAS PubMed.
- W. Zhang and J.-W. Rhim, Recent progress in konjac glucomannan-based active food packaging films and property enhancement strategies, Food Hydrocolloids, 2022, 128, 107572 CrossRef CAS.
- W. Xu, Z. Li, H. Sun, S. Zheng, H. Li, D. Luo, Y. Li, M. Wang and Y. Wang, High Internal-Phase Pickering Emulsions Stabilized by Xanthan Gum/Lysozyme Nanoparticles: Rheological and Microstructural Perspective, Front. Nutr., 2021, 8, 744234 CrossRef PubMed.
- P. You, L. Wang, N. Zhou, Y. Yang and J. Pang, A pH-intelligent response fish packaging film: Konjac glucomannan/carboxymethyl cellulose/blackcurrant anthocyanin antibacterial composite film, Int. J. Biol. Macromol., 2022, 204, 386–396 CrossRef CAS PubMed.
- B. N. Singh, Prateeksha, A. Rawat, R. Bhagat and B. Singh, Black tea: Phytochemicals, cancer chemoprevention, and clinical studies, Crit. Rev. Food Sci. Nutr., 2017, 57, 1394–1410 CrossRef CAS.
- S. Li, C.-Y. Lo, M.-H. Pan, C.-S. Lai and C.-T. Ho, Black tea: chemical analysis and stability, Food Funct., 2013, 4, 10–18 RSC.
- L. Xu, Y. Chen, Z. Chen, X. Gao, C. Wang, P. Panichayupakaranant and H. Chen, Ultrafiltration isolation, physicochemical characterization, and antidiabetic activities analysis of polysaccharides from green tea, oolong tea, and black tea, J. Food Sci., 2020, 85, 4025–4032 CrossRef CAS PubMed.
- H.-X. Gao, Z. He, Q. Sun, Q. He and W.-C. Zeng, A functional polysaccharide film forming by pectin, chitosan, and tea polyphenols, Carbohydr. Polym., 2019, 215, 1–7 CrossRef CAS PubMed.
- Y. Yan, S. Duan, H. Zhang, Y. Liu, C. Li, B. Hu, A. Liu, D. Wu, J. He and W. Wu, Preparation and characterization of Konjac glucomannan and pullulan composite films for strawberry preservation, Carbohydr. Polym., 2020, 243, 116446 CrossRef CAS PubMed.
- S. D. Saoji, S. C. Atram, P. W. Dhore, P. S. Deole, N. A. Raut and V. S. Dave, Influence of the Component Excipients
on the Quality and Functionality of a Transdermal Film Formulation, AAPS PharmSciTech, 2015, 16, 1344–1356 CrossRef CAS PubMed.
- A. Etxabide, P. A. Kilmartin, J. I. Maté and J. Gómez-Estaca, Characterization of glucose-crosslinked gelatin films reinforced with chitin nanowhiskers for active packaging development, LWT-Food Sci. Technol., 2022, 154, 112833 CrossRef CAS.
- H. H. Gahruie, A. Mirzapour, F. Ghiasi, M. H. Eskandari, M. Moosavi-Nasab and S. M. H. Hosseini, Development and characterization of gelatin and Persian gum composite edible films through complex coacervation, LWT-Food Sci. Technol., 2022, 153, 112422 CrossRef.
- H. H. Gahruie, M. Mostaghimi, F. Ghiasi, S. Tavakoli, M. Naseri and S. M. H. Hosseini, The effects of fatty acids chain length on the techno-functional properties of basil seed gum-based edible films, Int. J. Biol. Macromol., 2020, 160, 245–251 CrossRef.
- X. Chen, Q. Long, L. Zhu, L.-X. Lu, L.-N. Sun, L. Pan, L.-J. Lu and W.-R. Yao, A double-switch temperature-sensitive controlled release antioxidant film embedded with lyophilized nanoliposomes encapsulating rosemary essential oils for solid food, Materials, 2019, 12, 4011 CrossRef CAS.
- J. Simona, D. Dani, S. Petr, N. Marcela, T. Jakub and T. Bohuslava, Edible films from carrageenan/orange essential oil/trehalose—structure, optical properties, and antimicrobial activity, Polymers, 2021, 13, 332 CrossRef CAS PubMed.
- A. Sood and C. Saini, Red pomelo peel pectin based edible composite films: Effect of pectin incorporation on mechanical, structural, morphological and thermal properties of composite films, Food Hydrocolloids, 2022, 123, 107135 CrossRef CAS.
- R. M. Viana, N. M. Sá, M. O. Barros, M. de Fátima Borges and H. M. Azeredo, Nanofibrillated bacterial cellulose and pectin edible films added with fruit purees, Carbohydr. Polym., 2018, 196, 27–32 CrossRef CAS PubMed.
- Q. Li, D. Wang, D. Bai, C. Cai, J. Li, C. Yan, S. Zhang, Z. Wu, J. Hao and G. Yu, Photoprotective effect of Astragalus membranaceus polysaccharide on UVA-induced damage in HaCaT cells, PloS one, 2020, 15, e0235515 CrossRef CAS PubMed.
- F. Yang, J. Yang, S. Qiu, W. Xu and Y. Wang, Tannic acid enhanced the physical and oxidative stability of chitin particles stabilized oil in water emulsion, Food Chem., 2021, 346, 128762 CrossRef CAS PubMed.
- M. I. Pinzon, O. R. Garcia and C. C. Villa, The influence of Aloe vera gel incorporation on the physicochemical and mechanical properties of banana starch-chitosan edible films, J. Sci. Food Agric., 2018, 98, 4042–4049 CrossRef CAS PubMed.
- D. Wang, Y. Liu, J. Sun, Z. Sun, F. Liu, L. Du and D. Wang, Fabrication and characterization of gelatin/zein nanofiber films loading perillaldehyde for the preservation of chilled chicken, Foods, 2021, 10, 1277 CrossRef CAS PubMed.
- W. Liu, Z. Ling, C. Huang, C. Lai and Q. Yong, Investigation of galactomannan/deacetylated chitosan nanocomposite films and their anti-bacterial capabilities, Mater. Today Commun., 2022, 30, 103002 CrossRef CAS.
- H. Hashemi Gahruie, A. Mirzapour, F. Ghiasi, M. H. Eskandari, M. Moosavi-Nasab and S. M. H. Hosseini, Development and characterization of gelatin and Persian gum composite edible films through complex coacervation, LWT-Food Sci. Technol., 2022, 153, 112422 CrossRef CAS.
- C. Wu, Y. Li, Y. Du, L. Wang, C. Tong, Y. Hu, J. Pang and Z. Yan, Preparation and characterization of konjac glucomannan-based bionanocomposite film for active food packaging, Food Hydrocolloids, 2019, 89, 682–690 CrossRef CAS.
- J. Lu, X. Wang and C. Xiao, Preparation and characterization of konjac glucomannan/poly (diallydimethylammonium chloride) antibacterial blend films, Carbohydr. Polym., 2008, 73, 427–437 CrossRef CAS.
- Q. Zhang, J. Zhang, J. Zhang, D. Xu, Y. Li, Y. Liu, X. Zhang, R. Zhang, Z. Wu and P. Weng, Antimicrobial effect of tea polyphenols against foodborne pathogens: A review, J. Food Prot., 2021, 84, 1801–1808 CrossRef CAS PubMed.
- J. Lei, L. Zhou, Y. Tang, Y. Luo, T. Duan and W. Zhu, High-strength konjac glucomannan/silver nanowires composite films with antibacterial properties, Materials, 2017, 10, 524 CrossRef PubMed.
- Y. Cui, Y. Oh, J. Lim, M. Youn, I. Lee, H. Pak, W. Park, W. Jo and S. Park, AFM study of the differential inhibitory effects of the green tea polyphenol (−)-epigallocatechin-3-gallate (EGCG) against Gram-positive and Gram-negative bacteria, Food Microbiol., 2012, 29, 80–87 CrossRef CAS PubMed.
|
This journal is © The Royal Society of Chemistry 2022 |
Click here to see how this site uses Cookies. View our privacy policy here.