DOI:
10.1039/D2RA04938D
(Paper)
RSC Adv., 2022,
12, 26673-26679
Divergent copper-catalyzed syntheses of 3-carboxylpyrroles and 3-cyanofurans from O-acetyl oximes and β-ketoesters/nitriles†
Received
7th August 2022
, Accepted 13th September 2022
First published on 21st September 2022
Abstract
The reaction between O-acetyl oximes and β-ketoesters/nitriles catalyzed by copper generated polysubstituted pyrroles and furans, respectively, under redox–neutral reaction conditions. Using this protocol, pyrroles or furans could be obtained simply by choosing an appropriate active methylene compound. Although both transformations occur essentially under the same reaction conditions, control experiments indicated that they follow different mechanistic pathways.
Introduction
Redox-active oxime esters 1 are versatile building blocks used in a myriad of synthetic transformations beyond the classical reactions.1 This group of substrates can be effectively activated by transition metals under mild conditions with chemo- and regioselectivity.2 Copper represents a gentle, abundant, and inexpensive reaction mediator between oxime esters and a variety of components, forming the basis of a noteworthy arsenal of synthetic procedures,3 including annulations.2c,4 N–C–C and N–C–C–C synthons are commonly provided by oxime esters, facilitating the formation of medicinally important N-containing heterocyclic compounds. For instance, the reaction of oxime esters 1 with active methylene compounds 2 in the presence of a copper catalyst affords structurally diverse pyridines or fused-pyridines 3 through a [4 + 2] cyclization, with the organocopper species A as a central intermediate (Scheme 1a).5 In these reactions, the nucleophilic character of either 2 or A typically triggers the desired transformation.6 To the best of our knowledge, only one synthetic method is known to give a different type of product employing oxime esters as substrates. This process utilizes the readily oxidized N-protected α-aminomalonate 4 to generate 3-sulfonamido-4-pyrrolin-2-ones 5 and has as a key step the nucleophilic attack of species B on the in situ formed N-protected imine (Scheme 1b).7,8 Despite these previous protocols, there has been a notable lack of investigations into other common reaction intermediates derived from oxime esters with active methylene compounds. It is possible that such intermediates may modify the reaction course, allowing the synthesis of distinct products.
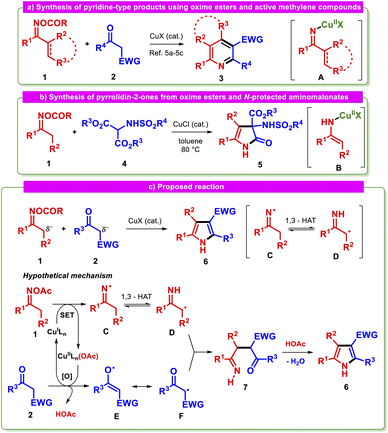 |
| Scheme 1 (a and b) Previously reported reactions between oxime esters and active methylene compounds. (c) Designed reaction and its proposed mechanism. | |
Iminyl radicals derived from the reduction of the N–O bond in oxime esters via single-electron transfer (SET) have recently attracted renewed interest because of the development of alternative syntheses with milder conditions,9 as well as their proclivity to form carbon-centered radicals,10 e.g., α-iminyl radicals form via a 1,3-hydrogen atom transfer process (1,3-HAT).10b,11 Based on our interest in metal-mediated reactions using oxime esters,12 we initially explored the possibility of preparing remarkable heterocycles such as pyrroles 6 starting from oxime esters 1 and active methylene compounds 2 through reaction pathways involving the iminyl radicals C and D (Scheme 1c).
We hypothesized that this transformation could be accomplished if the copper catalyst were to play a dual role in the reaction, acting as both an oxime ester reductant and as an oxidant for the active methylene compound. This would allow us to simultaneously access crucial radicals D and F, which would be expected to undergo selective radical/radical cross-coupling13 if species F behaves as a persistent radical. The planned strategy aimed to alter the nucleophilic character of the carbon atoms which are predicted to be bound to each other in the key step. Beyond the critical C–C bond formation stage, the success of the tandem reaction will depend on fulfilling the following requirements: (a) selective formation of the iminyl radical C, (b) transformation of such an intermediate to the α-iminyl species D, and (c) oxidation of the active methylene compound 2. The synthetic value of the proposed methodology derives from the importance of pyrroles as the cores of many natural products, including drugs and agrochemicals.14
Results and discussion
To test our hypothesis, we began by investigating the reaction between O-acetyl oxime 1a and methyl acetoacetate (2a). The highest yield was obtained with the conditions shown in the reaction scheme embedded in Table 1, which afforded the pyrrole 6a15 in 56% isolated yield.16
Table 1 Optimization of reaction conditionsa

|
Entry |
Deviation from the standard conditions |
Yieldb (%) |
Reaction conditions: 1a (75 μmol), 2a (2 equiv.), CuCN (10 mol%), dtbbpy (15 mol%) in 1 mL THF (0.075 M) at 100 °C for 36 h. NMR yield. 1a (0.15 mmol scale). Isolated yield. NR = no reaction. |
1 |
None |
65% (56%)c,d |
2 |
Other CuI and CuII salts |
<36% |
3 |
0.037 M/0.05 M |
38%/47% |
4 |
Li2CO3, Na2CO3, K2CO3, Et3N |
<45% |
5 |
3 Å, 4 Å, 5 Å MS |
<48% |
6 |
Na2S2O3/Na2SO3/NaHSO3 |
30%/49%/0% |
7 |
Mn(OAc)3, CAN, DCP, TBHP |
<48% |
8 |
R = tBu, Ph, C6H4-pCF3, C6F5 |
<44% |
9 |
Without Cu |
NR |
During the optimization phase, the importance of using CuCN as the copper source became clear since other salts did not furnish comparable results (entry 2). Modification of the reaction concentration, and the addition of Brønsted bases or molecular sieves did not boost the yield either (entries 3–5). The major product observed in most of the experiments was propiophenone (8), which can form from reactive intermediates A or B. To avoid this undesired product, several reductants11b,17 and oxidants were added to the reaction (entries 6, 7). Unfortunately, none of these additives hindered the formation of propiophenone, demonstrating that the inhibition of parasitic pathways is not straightforward. Reactions using O-acyl oximes were ineffective at producing the pyrrole 6a in higher yields (entry 8). We also corroborated that Cu is essential for the reaction (entry 9). As a final remark, during the optimization we often observed the presence of pyrrole 9, which may indicate the participation of the α-iminyl radical D in this transformation.11c
We proceeded to test the reaction with a set of aryl alkyl O-acetyl oximes to determine its scope (Table 2). Electron-donating groups at p- and m-positions gave the expected pyrroles 6a–6c and 6h in good yields; a slightly diminished yield was observed when either halogens or electron-withdrawing substituents were present at the p- and m-positions (6d–6f). Other β-ketoesters were also tolerated although ethyl isobutyrylacetate and ethyl benzoylacetate reacted to form pyrroles but less efficiently (6k, 6l). Notably, 4,5-diaryl pyrroles were also synthesized in moderate yields (6m, 6n); such compounds have shown important bioactivities,14a for example atorvastatin acts as a lipid-lowering agent.18 Other substrates with a larger alkyl side chain that might undergo a 1,5-HAT process10a,b gave the products 6o and 6p. Lastly, a cyclooctanone oxime derivate afforded the fused pyrrole 6q in 34% yield. Unfortunately, the O-acetyl oximes 1 in which R1 was an alkyl chain only formed the parent ketones. This class of substrates likely followed the pathway towards undesired species A and B.
Table 2 Substrate scope with some oxime esters (synthesis of pyrroles 6a–6q)
1 (0.15 mmol), 2 (0.3 mmol), CuCN (10 mol%), dtbbpy (15 mol%) in 2 mL THF (0.075 M) at 100 °C for 36 h. Isolated yield. |
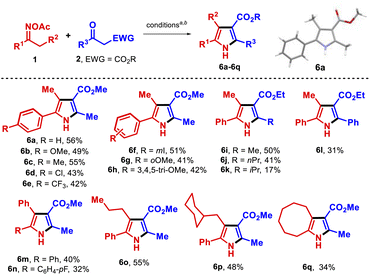 |
Next, we focused on the effect of using another active methylene compound, such as a β-ketonitrile, instead of a β-ketoester. When O-acetyl oxime 1a was reacted with benzoylacetonitrile (2b), 3-cyanofuran 10a (45% isolated yield) was obtained instead of the expected 3-cyanopyrrole (Table 3). The product was preliminarily identified using spectroscopic data, and was unambiguously confirmed by single-crystal X-ray diffraction analysis (CCDC 2155771†).19 Surprisingly, the regioselectivity of the reaction also changed, in that the substituents at the 4- and 5-positions were transposed compared to pyrroles 6. Remarkably, 10a was obtained in higher yield using only 1.1 equiv. of 2b (70% isolated yield), although propiophenone 8 was still observed as the main byproduct. To our knowledge, this represents the first synthesis of furans from oxime esters.20 3-Cyanofurans are useful blocks for synthesizing complex molecules21 and have shown interesting UV-absorbing properties;22 thus, the development of novel protocols to access those heterocycles is an attractive goal.23 When the transformation was applied to the formerly synthesized oximes 1 and others oxime esters, these molecules reacted with 2b to provide a wide range of furans in a more efficient fashion than was observed in the corresponding syntheses of pyrroles described above (Table 3).
Table 3 Substrate scope with aryl alkyl oxime esters (synthesis of furans 10a–10ab)
1 (0.15 mmol), 2b (1.1 equiv.), CuCN (10 mol%), dtbbpy (15 mol%) in 2 mL THF (0.075 M) at 100 °C for 36 h. Isolated yield. |
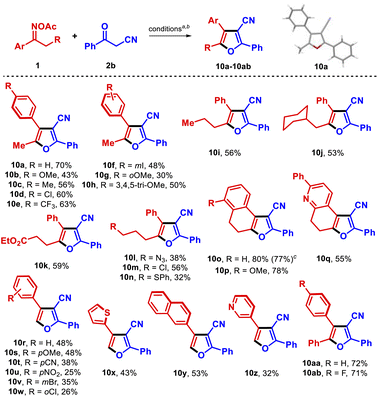 |
All but one of the substituted propiophenone oxime derivatives reacted as intended to generate furans in good yields (10b–10f, 10h); the exception was the O-methoxy substituted substrate, which gave poorer results due to steric constraints (10g). Substrates with an extended aliphatic side chain reacted well (10i, 10j), even those bearing more sensitive functionalities such as ester, azido, chloro, and thioether groups (10k–10n). Those functional groups have the potential to be exploited for the further derivatization of the products. α-Tetralone oximes produced the tricyclic products 10o and 10p in high yield, with the nucleus of these compounds resembling the natural product laevigatin.24 The tetrahydroquinolinone oxime derivative efficiently led to dihydrofuroquinoline 10q in 55% yield. The robustness of the method was demonstrated by isolating 10o in 77% yield from a 1.5 mmol scale reaction. In addition, acetophenone O-acetyl oximes and related compounds were transformed into the respective 2,3,4-trisubstituted furans 10r–10z, although in somewhat inferior yields. These low yields can be attributed to a slower radical isomerization rate in these substrates arising from the lack of an electron-rich alkyl substituent in the α-iminyl radical intermediate. Furthermore, in the reactions with substrates bearing either electronegative or electron-withdrawing substituents, hydrolysis of the O-acetyl moiety was observed. Pleasingly, the use of α-phenylacetophenones as substrates allowed the smooth synthesis of the significant triaryl-3-cyanofurans 10aa and 10ab.
The methodology was next applied to the group of dialkyl oximes 1aa (Table 4). Furans 10ac–10ag were successfully obtained under the optimized conditions in good yields. Interestingly, the reaction of 2,6-dimethylheptan-4-one oxime ester with benzoylacetonitrile also afforded the alkene 12, likely through condensation between the transient imine 11 and benzoylacetonitrile (2b). In principle, such a side product might be involved in the reaction pathway as one of the transient intermediates. Particularly, oxime ester 1ab yielded a 10
:
1 mixture of furans 10ah and 10ai owing to the existence of two distinct α-methylenes. In the cases of the phenoxyacetone and 3-phenoxybutan-2-one oxime derivatives 1ac and 1ad, the furan products 10aj–10ak were found not to contain the phenoxy moiety, while the reaction of the 1-(phenylthio)propan-2-one oxime ester 1ae with benzoylacetonitrile afforded an inseparable mixture of products 10aj and 10al.
Table 4 Substrate scope with dialkyl oxime esters (synthesis of furans 10ac–10al)
1aa (0.15 mmol), 2b (1.1 equiv.), CuCN (10 mol%), dtbbpy (15 mol%) in 2 mL THF (0.075 M) at 100 °C for 36 h. Isolated yield. Inseparable mixture. |
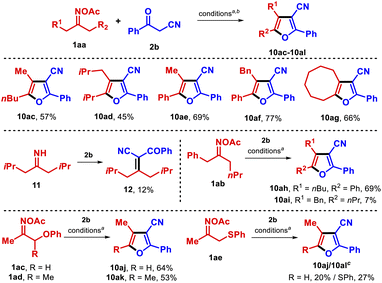 |
We also explored the products obtained when different acylacetonitriles were used as reactants (Table 5). Furans 10am–10as were obtained in good yields, with the presence of electron-withdrawing substituents on the acylacetonitrile having a positive influence on the transformation (10ao–10aq). Notably, reaction of aliphatic propionylacetonitrile with 1a afforded the furan 10at in 66% yield. 2-Pyrenyl-3-cyanofuran 10au was also synthesized and exhibited fluorescent features under UV-light. Lastly, beyond the model oxime ester 1a, other substrates successfully reacted with substituted benzoylacetonitriles to produce furans 10av and 10aw.
Table 5 Substrate scope with diverse acylacetonitriles (synthesis of furans 10am–10aw)
1 (0.15 mmol), 2ba–2bi (1.1 equiv.), CuCN (10 mol%), dtbbpy (15 mol%) in 2 mL THF (0.075 M) at 100 °C for 36 h. Isolated yield. |
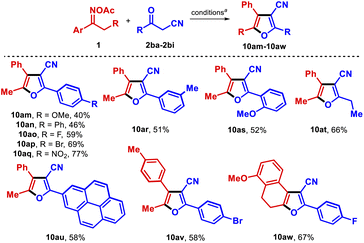 |
Finally, derivatization of the furan-3-carbonitrile 10o was achieved by transforming this furan into the carboxamide 13 in high yield. Additionally, the chloro functionality in compound 10m was successfully substituted by potassium phthalimide, yielding compound 14 in 73% yield (Scheme 2).
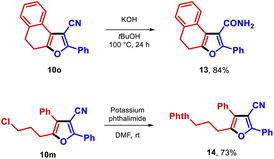 |
| Scheme 2 Derivatization of furans 10o and 10m. | |
We next performed experiments to gain insights into the reaction mechanism (Scheme 3). We found that the addition of an external oxidant such as TEMPO to the reaction of 1a with either methyl acetoacetate (2a) or benzoylacetonitrile (2b) completely suppressed the reaction, and that the presence of the non-oxidizing radical scavenger tert-butylhydroxytoluene (BHT) affected the reactions of 2b and 2a with 1a differently, with the former reaction being less impacted (Scheme 3a). These results were consistent with the proposed radical mechanism via SET to the copper catalyst in the synthesis of pyrroles (Scheme 1c), although it is probable that a somewhat different pathway is operative in the formation of furans 10. Additionally, reaction of the O-acetyl oxime bearing an alkene-tethered motif 1af with 2a or 2b did not yield the expected products 6r and 10ax (Scheme 3b). GC-MS analysis of those reactions suggested the presence of pyrrole 15, which could form via an iminyl radical γ,δ-cyclization.25 Thus, when 1af is used as a reactant, the latter process is apparently more rapid than the desirable 1,3-HAT process. The reaction between 1a and the radical acceptor 16 in the absence of the active methylene compound furnished the pyrroline 1726 in moderate yield (Scheme 3c), thus confirming the formation of an α-iminyl radical during the process. Finally, submission of nitrile 12 to the optimal conditions did not afford 10ad (Scheme 3d), ruling out its participation as an intermediate in the transformation.
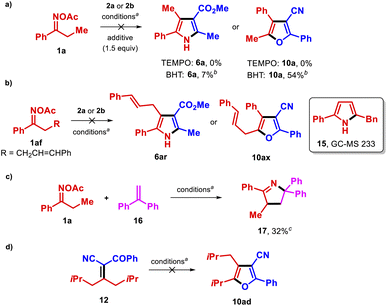 |
| Scheme 3 Control experiments. aCuCN (10 mol%), dtbbpy (15 mol%) in THF (0.075 M) at 100 °C for 36 h. bNMR yield. cIsolated yield. | |
On the basis of these results and literature reports,27 we suggest an alternative mechanistic pathway in the case of furans 10 (Scheme 4). We tentatively propose that a rapid trapping of radical D by the copper species G,27a formed by ligand exchange, gives alkyl-CuIII intermediate H.25 The latter undergoes reductive elimination to create the C–O bond and regenerates the active CuI catalyst.28 Subsequently, the intramolecular nucleophilic addition of C-3 to the imine in intermediate 18 takes place under AcOH catalysis, generating the product.
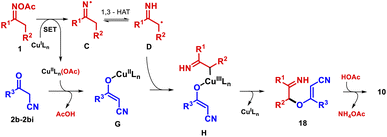 |
| Scheme 4 Alternative mechanism for the synthesis of furans 10. | |
Although β-ketoesters could follow the same mechanism, it seems not to be operational; apparently, the chelation of CuII species by 2a followed by its oxidation is more favorable.
Conclusions
In summary, we have developed two external-oxidant-free synthetic procedures to access alkyl 3-carboxylpyrroles and 3-cyanofurans via copper-mediated cyclization of oxime esters with β-ketoesters and β-ketonitriles, respectively. These protocols are technically identical but mechanistically different. Unlike previously reported synthetic pathways, which have nucleophilic organocopper species as intermediates, the proposed syntheses proceed via radical intermediates. In the procedures described here, the copper catalyst acts as both an oxime ester reductant and as an activator of the active methylene compounds. The preparation of furans was not only more effective but also displayed a broader scope due to the direct participation of the catalyst in the C–O bond formation step. The synthesis of pyrroles was less efficient than that of furans, possibly due to oxidation of the esters and radical–radical cross-coupling being outcompeted by other pathways. Despite the limitations observed in the synthesis of pyrroles, this reaction represents a proof of concept of a strategy that can potentially be used to synthesize other heterocycles. Currently, further studies are underway focused on understanding the divergence in the reaction pathway depending on the active methylene compound used.
Author contributions
W. E. A.-O., A. R. O., O. C.-D., A. R.-S. and A. M.-G. prepared the substrates. W. E. A.-O. and A. R. O. performed and analyzed the experiments. R. O. T.-O. conceived and supervised the project. R. O. T.-O., W. E. A.-O. and A. R. O. wrote the manuscript.
Conflicts of interest
There are no conflicts of interest to declare.
Acknowledgements
We acknowledge CONACYT (FOP16-2021-01, 319783), UC MEXUS-CONACYT Collaborative Research Grant (CN-20-91) and DGAPA-UNAM (grant IA202621) for financial support. A. R. O. (CVU 770510), W. E. A.-O. (CVU 1099675) and O. C.-D. (CVU 1180260) thank CONACYT for graduate studies fellowships. We are also grateful for the technical support provided by Rubén Toscano, Simón Hernández, Diego Otero, Javier Pérez, Carmen García, Ángeles Peña, Elizabeth Huerta, Celia Bustos, Isabel Chávez, Rubén Gaviño, Beatriz Quiroz, Virginia Gómez and Adriana Romo (IQ-UNAM). We thank Prof. José G. López and Prof. Marcos Hernández for their helpful comments and suggestions.
Notes and references
-
(a) P. W. Neber and A. V. Friedolsheim, Über eine neue Art der Umlagerung von Oximen, Justus Liebigs Ann. Chem., 1926, 449, 109–134 CrossRef CAS
;
(b) C. O'Brien, The Rearrangement of Ketoxime O-Sulfonates to Amino Ketones (The Neber Rearrangement), Chem. Rev., 1964, 64, 81–89 CrossRef
;
(c) W. F. Berkowitz, The Neber Rearrangement, Org. React., 2012, 78, 321–410 CAS
;
(d) E. Beckmann, Zur Kenntniss der Isonitrosoverbindungen, Chem. Ber., 1886, 19, 988–993 CrossRef
;
(e) B. Jones, Kinetics and Mechanism of the Beckmann Rearrangement, Chem. Rev., 1944, 35, 335–350 CrossRef CAS
. -
(a) For reviews: D. S. Bolotin, N. A. Bokach, M. Ya. Demakova and V. Yu. Kukushkin, Metal-Involving Synthesis and Reactions of Oximes, Chem. Rev., 2017, 117, 13039–13122 CrossRef CAS PubMed
;
(b) H. Huang, X. Ji, W. Wu and H. Jiang, Transition Metal-Catalyzed C–H Functionalization of N-Oxyenamine Internal Oxidants, Chem. Soc. Rev., 2015, 44, 1155–1171 RSC
;
(c) H. Huang, J. Cai and G.-J. Deng, O-Acyl Oximes: Versatile Building Blocks for N-Heterocycle Formation in Recent Transition Metal Catalysis, Org. Biomol. Chem., 2016, 14, 1519–1530 RSC
;
(d) J. Li, Y. Hu, D. Zhang, Q. Liu, Y. Dong and H. Liu, Transition Metal-Catalyzed Reactions Involving Oximes, Adv. Synth. Catal., 2017, 359, 710–771 CrossRef CAS
;
(e) K. A. Rykaczewski, E. R. Wearing, D. E. Blackmun and C. S. Schindler, Reactivity of Oximes for Diverse Methodologies and Synthetic Applications, Nat. Synth., 2022, 1, 24–36 CrossRef
. -
(a) Y.-N. Zheng, H. Zheng, T. Li and W.-T. Wei, Recent Advances in Copper-Catalyzed C−N Bond Formation Involving N-Centered Radicals, ChemSusChem, 2021, 14, 5340–5358 CrossRef CAS PubMed
;
(b) L. Lei, C. Li and D. Mo, Recent Advances in Copper-Catalyzed N-O Cleavage Strategy, Chin. J. Org. Chem., 2019, 39, 2989–3012 CrossRef CAS
. -
(a) C. Chen, J. Zhao, X. Shi, L. Liu, Y.-P. Zhu, W. Sun and B. Zhu, Recent Advances in Cyclization Reactions of Unsaturated Oxime Esters (Ethers): Synthesis of Versatile Functionalized Nitrogen-Containing Scaffolds, Org. Chem. Front., 2020, 7, 1948–1969 RSC
;
(b) N. A. Bokach, D. S. Bolotin and V. Y. Kukushkin, in Synthetic Approaches to Nonaromatic Nitrogen Heterocycles, ed. A. M. M. M. F. Phillips, Wiley, Hoboken, 1st edn, 2020, ch. 16, pp. 501–532 Search PubMed
;
(c) J. C. Walton, Synthetic Strategies for 5- and 6-Membered Ring Azaheterocycles Facilitated by Iminyl Radicals, Molecules, 2016, 21, 660 CrossRef PubMed
. -
(a) H. Jiang, J. Yang, X. Tang and W. Wu, Divergent Syntheses of Isoquinolines and Indolo[1,2-a]quinazolines by Copper-Catalyzed Cascade Annulation from 2-Haloaryloxime Acetates with Active Methylene Compounds and Indoles, J. Org. Chem., 2016, 81, 2053–2061 CrossRef CAS PubMed
;
(b) L. Zhang, J. Duan, G. Xu, X. Ding, Y. Mao, B. Rong, N. Zhu, Z. Fang, Z. Li and K. Guo, Copper-Catalyzed N–O Cleavage of α,β-Unsaturated Ketoxime Acetates toward Structurally Diverse Pyridines, J. Org. Chem., 2020, 85, 2532–2542 CrossRef CAS PubMed
;
(c) B. Rong, G. Xu, H. Yan, S. Zhang, Q. Wu, N. Zhu, Z. Fang, J. Duan and K. Guo, Synthesis of Benzofuro- and Benzothieno[2,3-c]pyridines via Copper-Catalyzed [4 + 2] Annulation of Ketoxime Acetates with Acetoacetanilide, Org. Chem. Front., 2021, 8, 2939–2943 RSC
. - Alongside the metal-catalyzed methodologies, other metal-free protocols involving oxime esters and active methylene compounds also afford pyridines:
(a) H. Huang, J. Cai, H. Xie, J. Tan, F. Li and G.-J. Deng, Transition-Metal-Free N–O Reduction of Oximes: A Modular Synthesis of Fluorinated Pyridines, Org. Lett., 2017, 19, 3743–3746 CrossRef CAS PubMed
;
(b) Y. Xia, J. Cai, H. Huang and G.-J. Deng, Synthesis of Polysubstituted Pyridines from Oxime Acetates Using NH4I as a Dual-Function Promoter, Org. Biomol. Chem., 2018, 16, 124–129 RSC
. - C.-B. Miao, A.-Q. Zheng, L.-J. Zhou, X. Lyu and H.-T. Yang, Copper-Catalyzed Annulation of Oxime Acetates with α-Amino Acid Ester Derivatives: Synthesis of 3-Sulfonamido/Imino 4-Pyrrolin-2-ones, Org. Lett., 2020, 22, 3381–3385 CrossRef CAS
. - For a [3 + 2 + 1] annulation involving oxime esters, active methylene compounds and aldehydes to generate pyridines via intermediate B see: H. Jiang, J. Yang, X. Tang, J. Li and W. Wu, Cu-Catalyzed Three-Component Cascade Annulation Reaction: An Entry to Functionalized Pyridines, J. Org. Chem., 2015, 80, 8763–8771 CrossRef CAS PubMed
. -
(a) For reviews: S. Z. Zard, Recent Progress in the Generation and Use of Nitrogen-Centred Radicals, Chem. Soc. Rev., 2008, 37, 1603–1618 RSC
;
(b) M. M. Jackman, Y. Cai and S. L. Castle, Recent Advances in Iminyl Radical Cyclizations, Synthesis, 2017, 49, 1785–1795 CrossRef CAS
;
(c) J. Davies, S. P. Morcillo, J. J. Douglas and D. Leonori, Hydroxylamine Derivatives as Nitrogen-Radical Precursors in Visible-Light Photochemistry, Chem.–Eur. J., 2018, 24, 12154–12163 CrossRef CAS PubMed
;
(d) M. Bera, D. S. Lee and E. J. Cho, Advances in N-Centered Intermediates by Energy Transfer Photocatalysis, Trends Chem., 2021, 3, 877–891 CrossRef CAS
;
(e) K. Kwon, R. T. Simons, M. Nandakumar and J. L. Roizen, Strategies to Generate Nitrogen-Centered Radicals That May Rely on Photoredox Catalysis: Development in Reaction Methodology and Applications in Organic Synthesis, Chem. Rev., 2022, 122, 2353–2428 CrossRef CAS PubMed
. -
(a) For reviews: L. Liu, X.-H. Duan and L.-N. Guo, Recent Advance in Iminyl Radical Triggered C–H and C–C Bond Functionalization of Oxime Esters via 1,5-HAT and β-Carbon Scission, Synthesis, 2021, 53, 4375–4388 CrossRef CAS
;
(b) I. B. Krylov, O. O. Segida, A. S. Budnikov and A. O. Terent'ev, Oxime-Derived Iminyl Radicals in Selective Processes of Hydrogen Atom Transfer and Addition to Carbon-Carbon π-Bonds, Adv. Synth. Catal., 2021, 363, 2502–2528 CrossRef CAS
;
(c) F. Xiao, Y. Guo and Y.-F. Zeng, Recent Developments in Radical Cross-Coupling of Redox-Active Cycloketone Oximes, Adv. Synth. Catal., 2021, 363, 120–143 CrossRef CAS
. - For copper-catalyzed reactions see:
(a) J. Ke, Y. Tang, H. Yi, Y. Li, Y. Cheng, C. Liu and A. Lei, Copper-Catalyzed Radical/Radical Csp3-H/P-H Cross-Coupling: α-Phosphorylation of Aryl Ketone O-Acetyloximes, Angew. Chem., Int. Ed., 2015, 54, 6604–6607 CrossRef CAS PubMed
;
(b) S.-P. Jiang, Y.-T. Su, K.-Q. Liu, Q.-H. Wu and G.-W. Wang, Copper(I)-Catalyzed Heteroannulation of [60]Fullerene with Ketoxime Acetates: Preparation of Novel 1-Fulleropyrrolines, Chem. Commun., 2015, 51, 6548–6551 RSC
;
(c) L. Ran, Z.-H. Ren, Y.-Y. Wang and Z.-H. Guan, Copper-Catalyzed Homocoupling of Ketoxime Carboxylates for Synthesis of Symmetrical Pyrroles, Green Chem., 2014, 16, 112–115 RSC
;
(d) B. Zhao, H.-W. Liang, J. Yang, Z. Yang and Y. Wei, Copper-Catalyzed Intermolecular Cyclization between Oximes and Alkenes: A Facile Access to Spiropyrrolines, ACS Catal., 2017, 7, 5612–5617 CrossRef CAS
. -
(a) R. O. Torres-Ochoa, A. Leclair, Q. Wang and J. Zhu, Iron-Catalysed Remote C(sp3)−H Azidation of O-Acyl Oximes and N-Acyloxy Imidates Enabled by 1,5-Hydrogen Atom Transfer of Iminyl and Imidate Radicals: Synthesis of γ-Azido Ketones and β-Azido Alcohols, Chem.–Eur. J., 2019, 25, 9477–9484 CrossRef CAS PubMed
;
(b) Z. Li, R. O. Torres-Ochoa, Q. Wang and J. Zhu, Functionalization of Remote C(sp3)-H Bonds Enabled by Copper-Catalyzed Coupling of O-Acyloximes with Terminal Alkynes, Nat. Commun., 2020, 11, 403 CrossRef CAS PubMed
;
(c) R. Lavernhe, R. O. Torres-Ochoa, Q. Wang and J. Zhu, Copper-Catalyzed Aza-Sonogashira Cross-Coupling to Form Ynimines: Development and Application to the Synthesis of Heterocycles, Angew. Chem., Int. Ed., 2021, 60, 24028–24033 CrossRef CAS PubMed
. - Z. Zhu, X. Tang, J. Li, X. Li, W. Wu, G. Deng and H. Jiang, Synthesis of Enaminones Via Copper-Catalyzed Decarboxylative Coupling Reaction under Redox-Neutral Conditions, Chem. Commun., 2017, 53, 3228–3231 RSC
. -
(a) For reviews: V. Bhardwaj, D. Gumber, V. Abbot, S. Dhiman and P. Sharma, Pyrrole: A Resourceful Small Molecule in Key Medicinal Hetero-aromatics, RSC Adv., 2015, 5, 15233–15266 RSC
;
(b) C. Bulumulla, R. Gunawardhana, P. L. Gamage, J. T. Miller, R. N. Kularatne, M. C. Biewer and M. C. Stefan, Pyrrole-Containing Semiconducting Materials: Synthesis and Applications in Organic Photovoltaics and Organic Field-Effect Transistors, ACS Appl. Mater. Interfaces, 2020, 12, 32209–32232 CrossRef CAS
;
(c) K. Seipp, L. Geske and T. Opatz, Marine Pyrrole Alkaloids, Mar. Drugs, 2021, 19, 514 CrossRef CAS PubMed
;
(d) M. da C. A. Dias Bianco, D. I. L. Firmino Marinho, L. Villas Boas Hoelz, M. Macedo Bastos and N. Boechat, Pyrroles as Privileged Scaffolds in the Search for New Potential HIV Inhibitors, Pharmaceuticals, 2021, 14, 893 CrossRef
. - Crystallographic data for the compound 6a have been deposited with the CCDC 2177946†.
- For further details, see ESI†.
-
(a) Z.-H. Guan, Z.-Y. Zhang, Z.-H. Ren, Y.-Y. Wang and X. Zhang, Synthesis of Enamides via CuI-Catalyzed Reductive Acylation of Ketoximes with NaHSO3, J. Org. Chem., 2011, 76, 339–341 CrossRef CAS PubMed
;
(b) Z.-H. Ren, Z.-Y. Zhang, B.-Q. Yang, Y.-Y. Wang and Z.-H. Guan, Copper-Catalyzed Coupling of Oxime Acetates with Aldehydes: A New Strategy for Synthesis of Pyridines, Org. Lett., 2011, 13, 5394–5397 CrossRef CAS PubMed
. -
(a) B. D. Roth, C. J. Blankley, A. W. Chucholowski, E. Ferguson, M. L. Hoefle, D. F. Ortwine, R. S. Newton, C. S. Sekerke, D. R. Sliskovic and M. Wilson, Inhibitors of Cholesterol Biosynthesis. 3. Tetrahydro-4-hydroxy-6-[2-(1H-pyrrol-1-yl)ethyl]-2H-pyran 2-one Inhibitors of HMG-CoA Reductase. 2. Effects of Introducing Substituents at Positions Three and Four of the Pyrrole Nucleus, J. Med. Chem., 1991, 34, 357–366 CrossRef CAS PubMed
;
(b) B. D. Roth, The Discovery and Development of Atorvastatin, A Potent Novel Hypolipidemic Agent, Prog. Med. Chem., 2002, 40, 1–22 CrossRef CAS PubMed
. - Crystallographic data for the compound 10a has been deposited with the CCDC 2155771†.
- Although the reaction of 4-hydroxycoumarins with oxime esters to give furo[3,2-c]coumarins is known, the use of 2b in lieu of the coumarin represents a significant challenge due to its lower tendency to be oxidized
(a) T. A. To, Y. H. Vo, A. T. Nguyen, A. N. Q. Phan, T. Truong and N. T. S. Phan, A New Route to Substituted Furocoumarins via Copper-Catalyzed Cyclization between 4-Hydroxycoumarins and Ketoximes, Org. Biomol. Chem., 2018, 16, 5086–5089 RSC
;
(b) M. He, Z. Yan, W. Wang, F. Zhu and S. Li, Copper-Catalyzed Radical/Radical Cross-Coupling of Ketoxime Carboxylates with 4-Hydroxycoumarins: A Novel Synthesis of Furo[3,2-c]-coumarins, Tetrahedron Lett., 2018, 59, 3706–3712 CrossRef CAS
;
(c) Q. T. Pham, P. Q. Le, H. V. Dang, H. Q. Ha, H. T. D. Nguyen, T. Truong and T. M. Le, Iodine-Mediated Formal [3 + 2] Annulation for Synthesis of Furocoumarin from Oxime Esters, RSC Adv., 2020, 10, 44332–44338 RSC
. -
(a) I. García-Ventura, M. Flores-Alamo and J. J. García, Carbon–Carbon vs. Carbon–Oxygen Bond Activation in 2- and 3-Furonitriles with Nickel, RSC Adv., 2016, 6, 101259–101266 RSC
;
(b) B. Guo, J. G. de Vries and E. Otten, Hydration of Nitriles Using a Metal–Ligand Cooperative Ruthenium Pincer Catalyst, Chem. Sci., 2019, 10, 10647–10652 RSC
;
(c) M. A. Mansour, D. S. Lasheen, H. M. Gaber and K. A. M. Abouzid, Elaborating Piperazinyl-Furopyrimidine Based Scaffolds as Phosphoinositol-3-kinase Enzyme Alpha (PI3Kα) Inhibitors to Combat Pancreatic Cancer, RSC Adv., 2020, 10, 32103–32112 RSC
. - J.-S. Li, Y. Xue, Z.-W. Li, W.-D. Liu, C.-H. Lu and P.-X. Zhao, An Efficient Access to Fluorescent 2,3,4-Tricyanofurans from α-Cyano Ketones Using DDQ as Maleonitrile Building Block, Synlett, 2013, 24, 2003–2005 CrossRef CAS
. -
(a) P. Liu, M. Lei, L. Ma and L. Hu, An Efficient Synthesis of 2-Aminofuran-3-carbonitriles via Cascade Stetter-γ-Ketonitrile Cyclization Reaction Catalyzed by N-Heterocyclic Carbene, Synlett, 2011, 2011, 1133–1136 CrossRef
;
(b) Z.-L. Wang, H.-L. Li, L.-S. Ge, X.-L. An, Z.-G. Zhang, X. Luo, J. S. Fossey and W.-P. Deng, DDQ-Mediated Oxidative Coupling: An Approach to 2,3-Dicyanofuran (Thiophene), J. Org. Chem., 2014, 79, 1156–1165 CrossRef CAS PubMed
;
(c) L. Wang, X. Liu, M. Wang and J. Liu, Copper(I)-Catalyzed Heterocyclization of α-Acyl-α-alkynyl Ketene Dithioacetals: Synthesis of 3-Cyanofurans, Org. Lett., 2016, 18, 2162–2165 CrossRef CAS PubMed
;
(d) Y. Yu, Y. Chen, W. Wu and H. Jiang, Facile Synthesis of Cyanofurans via Michael-addition/cyclization of Ene–Yne–Ketones with Trimethylsilyl Cyanide, Chem. Commun., 2017, 53, 640–643 RSC
;
(e) J. Zhou, X. Zhu, M. Huang and Y. Wan, SeO2-Mediated One-Pot Synthesis of 3-Cyanofurans from 3-Oxo-3-arylpropanenitriles and Substituted Acetaldehydes, Eur. J. Org. Chem., 2017, 2017, 2317–2321 CrossRef CAS
. - F. Bohlmann and C. Zdero, Natürlich vorkommende Terpen-Derivate, 80. Einige Inhaltsstoffe der Gattung Chromolaena, Chem. Ber., 1977, 110, 487–490 CrossRef CAS
. - A. Faulkner, N. J. Race, J. S. Scott and J. F. Bower, Copper Catalyzed Heck-like Cyclizations of Oxime Esters, Chem. Sci., 2014, 5, 2416–2421 RSC
. - A proposed mechanism for pyrroline 17 is depicted within ESI†.
-
(a) X. Tang, J. Yang, Z. Zhu, M. Zheng, W. Wu and H. Jiang, Access to Thiazole via Copper-Catalyzed [3 + 1 + 1]-Type Condensation Reaction under Redox-Neutral Conditions, J. Org. Chem., 2016, 81, 11461–11466 CrossRef CAS PubMed
;
(b) X. Tang, L. Huang, Y. Xu, J. Yang, W. Wu and H. Jiang, Copper-Catalyzed Coupling of Oxime Acetates with Sodium Sulfinates: An Efficient Synthesis of Sulfone Derivatives, Angew. Chem., Int. Ed., 2014, 53, 4205–4208 CrossRef CAS PubMed
;
(c) C. Zhu, R. Zhu, H. Zeng, F. Chen, C. Liu, W. Wu and H. Jian, Copper-Catalyzed C(sp3)-H/C(sp3)-H Cross-Dehydrogenative Coupling with Internal Oxidants: Synthesis of 2-Trifluoromethyl-Substituted Dihydropyrrol-2-ols, Angew. Chem., Int. Ed., 2017, 56, 13324–13328 CrossRef CAS
. - The key C–O bond formation step in the proposed mechanism for furans 10 is different from the one conjectured for the preparation of furo[3,2-c]coumarins, see ref. 20a and 20b.
Footnotes |
† Electronic supplementary information (ESI) available: Experimental procedures; spectroscopic data and X-ray crystal structure. CCDC 2177946 and 2155771. For ESI and crystallographic data in CIF or other electronic format see https://doi.org/10.1039/d2ra04938d |
‡ These authors contributed equally to this work. |
|
This journal is © The Royal Society of Chemistry 2022 |