DOI:
10.1039/D2RA04872H
(Paper)
RSC Adv., 2022,
12, 25154-25162
One-pot thiol-free synthetic approach to sulfides, and sulfoxides selectively†
Received
5th August 2022
, Accepted 25th August 2022
First published on 5th September 2022
Abstract
A facile and efficient thiol-free one-pot method for direct synthesis of sulfides and sulfoxides under green conditions without using any metal catalyst is reported. For this purpose, we used benzyl bromides as starting materials in the presence of potassium thioacetate (PTA) and Oxone® which are low-cost, and readily accessible chemicals. This method is highly compatible with a variety of functional groups and delivered a series of sulfides, bis-sulfides, and sulfoxides in good yields. The selective formation of sulfoxides over sulfones is discussed via a mechanism.
Introduction
Organosulfur compounds constitute a significant part of organic synthesis1 because they are useful in medicinal chemistry,2 materials science,3 and natural product syntheses.4 Thioethers (sulfides) have found attractive applications in the pharma industry.5 For example, Montelukast (Singulair) is a drug prescribed for allergies and asthma.6 Ranitidine is a well-known drug utilized in histamine-2 blockers7 (Fig. 1). Additionally, benzyl sulfides are used as near infra-red (NIR) fluorescent probes for targeted cancer imaging.8 Given the versatility in the utilization of organosulfur compounds, searching for a better and more sustainable synthetic method seems like a necessity.
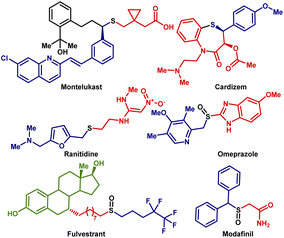 |
| Fig. 1 Representative biologically active organosulfur compounds. | |
A common strategy to prepare organosulfur compounds, especially sulphides, is to use thiols as starting materials.9 The synthesis of sulfides from thiols is promoted by the ease of their availability and high reactivity.10 However, thiols often restrict the direct synthesis of desired sulfides due to inherent disadvantages such as awfully irritating smell and side effects concerning health.11
Our group has been engaged in the design of organosulfur compounds using a green reagent such as rongalite.12 Some of these sulfur compounds are reported as useful synthons in C–C bond formation and metathesis.13 Generally, thioacetate anions are reacted with halides or alcohol derivatives to prepare thioacetates14 which are purified first. Then, in the next step, the thioacetates are converted to thiols.15 Afterwards, the thiols are transformed further to desired unsymmetrical sulfides.9 We questioned whether an easier, shorter, and odorless protocol can be realized for the preparation of sulfides. Gratifyingly, here we present a synthetic strategy that combines the aforementioned three steps in one-pot. Our methodology converts benzyl halides into unsymmetrical sulfides via a one-pot operation. This strategy avoids the formation of byproducts, usage of transition metal catalysts, and toxic solvents. The reaction time is short and the purification is docile. The substrate scope is quite large and the yields are good to excellent. The advantages of this methodology are; avoiding the usage of thiols hence environmentally benign, reducing the number of steps in overall sequence, and employing inexpensive reagents. A library of benzyl sulfides is easily prepared with this strategy.
Several odorless reagents such as Na2S, rongalite, NaHS, KSCN, potassium ethylxanthate, and potassium thioacetate are available for the preparation of sulfur-based compounds. By utilizing sodium sulfide/rongalite, only symmetrical sulfides16/sulfones17 are prepared from halides and these reagents are further explored.18 Sodium hydrosulfide (NaHS), highly hygroscopic and difficult to handle, is a source for HS− which leads to the formation of thiols and hence adding one more step towards preparing sulfides.19 KSCN leads to thiocyanate products as well as isothiocyanate products.20 Potassium ethyl xanthate leads to sulfides in two steps under heating conditions and several byproducts were observed as reported by Fochi et al.21 Therefore, in light of these limitations, we have chosen potassium thioacetate as our reagent due to its capacity of preparing versatile sulfides without involving thiols in a single-pot at room temperature as explored in this manuscript.
Seminal existing pathways for sulfide preparation involving benzyl and alkenyl/alkyl moieties are presented in Fig. 2.15c,22 It is observed from the literature that synthetic strategies for Csp3–S–Csp3 type of sulfides/sulfoxides are much less explored as compared to Csp2–S–Csp2 and Csp2–S–Csp3 sulfides23/sulfoxides24 despite having significant applications (Fig. 1). Our work focuses on the less explored area of unsymmetrical Csp3–S–Csp3 hybridized organosulfur derivatives. The main theme of our strategy involves a one-pot operation and thus avoiding three steps used for converting bromides to sulfides through thioacetates and thiols. Instead, we directly obtain diverse sulfides from halides via a straightforward route in benign solvents such as methanol or water. The application of our method is demonstrated by preparing sulfide derivatives containing the core units of biologically active compounds and to our delight, the unsymmetrical sulfides were obtained in good yields.
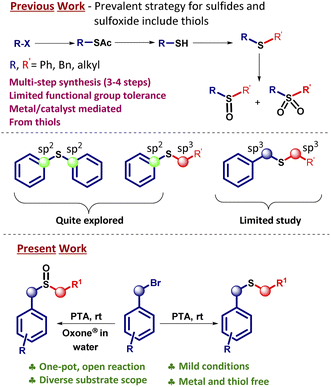 |
| Fig. 2 General overview of the preparation of organosulfur compounds (sulfide, sulfoxide, and sulfone). | |
Other classes of organosulfur compounds include sulfoxides and sulfones. Sulfoxides, along with their biological applications,25 are useful as catalysts and chiral ligands in asymmetric syntheses,26 and hence preparation of sulfoxides is an equally attractive task. Sulfoxides have been prepared extensively from sulfides27 and control is needed to avoid the formation of sulfone.28 To the best of our knowledge, no report is available where halides are directly converted into unsymmetrical Csp3–S–Csp3 hybridized sulfoxides in a single-pot without involving thiols and avoiding four steps of isolation and purification. To this end, the latter part of this article deals with the expansion of our procedure towards the selective preparation of sulfoxides instead of sulfones29 from halides by aqueous Oxone® in the same pot.
Results and discussion
Reaction development and optimization
To realize the one-pot strategy shown in Fig. 2, benzyl bromide was reacted with potassium thioacetate (PTA) in methanol. The reaction proceeds via SN2 type of substitution with thioacetate at the benzylic position. After 2 h, potassium carbonate was added to the same reaction mixture to deprotect –COCH3 group and generate sulfide (S−) nucleophile. Then, sp3 hybridized primary electrophile (R–CH2–X) was added to this reaction mixture and delivered the desired product. Table 1 summarizes the optimization details with respect to the amount of base and solvent used for the conversion of 1 into 2. As we increased the equiv. of the base, we observed a drastic increase in the yield and up to 3 equiv. of potassium carbonate is required (Table 1, entries 1–5) to get maximum yield.
Table 1 Optimization table for the preparation of sulfide 2a
If the addition of halide is exempted after transferring K2CO3 in the reaction pot, then, compound 3 is obtained as a sole product. We also found that non-polar and aprotic solvents such as dichloroethane, dichloromethane, toluene, and diethyl ether are not suitable.
Alternatively, another polar protic solvent i.e. water was tested to check the feasibility of the reaction. The reaction is facile in MeOH
:
water (1
:
1) combination as well as in water (Table 1, entry 6 and 7). However, the addition of water led to a slight reduction in the overall yield of the reaction as compared to the other optimized conditions (Table 1, entry 7).
Later on, we attempted sp2 hybridized, and sp3 hybridized secondary and tertiary electrophiles to deliver the desired compound 4. In each case, we failed to detect the expected sulfide 4. Instead, we observed a common product 3 in good yield. The possibility of secondary nucleophilic substitution reaction at a less hindered sp3 hybridized center leads to the formation of unsymmetrical sulfide 4 (Scheme 1). So, it was concluded that this one-pot methodology is useful with reactive electrophiles such as primary alkyl halide to generate various sulfide derivatives.
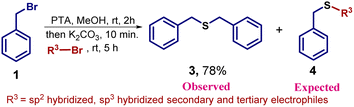 |
| Scheme 1 Attempts to prepare unsymmetrical sulfide 4. | |
Substrate scope
Having identified the optimal reaction conditions, the attention was turned towards establishing the generality of this transformation. To this end, benzyl bromide was reacted with several unsaturated halides and to our delight, the desired unsymmetrical sulfides were obtained in good to excellent yields. Noticeably, the aliphatic chain length of the halide has an inverse relationship with respect to the yield. As the number of methylene units (–CH2) increases in the electrophile, a slight decrease in the yield was observed. After the preparation of unsymmetrical sulfides containing unsaturated aliphatic side chains, the attention was shifted towards the synthesis of unsymmetrical sulfides containing saturated alkane side chains. In this regard, various substituted benzyl bromides were treated with alkyl bromides of varying chain lengths to prepare unsymmetrical sulfides containing benzylic and aliphatic moieties (Scheme 2).
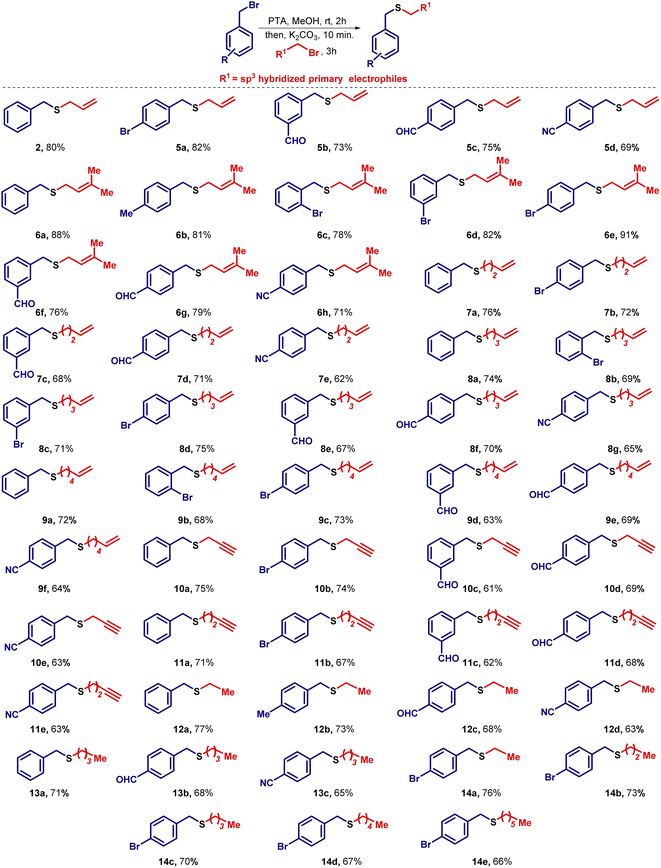 |
| Scheme 2 Synthesis of unsymmetrical sulfides containing unsaturated and saturated aliphatic side chains. Reaction conditions: compound 1 (0.87 mmol, 1 equiv.), PTA (0.87 mmol, 1 equiv.), MeOH (10 ml), K2CO3 (2.61 mmol, 3 equiv.), R1–CH2–Br (0.87 mmol, 1 equiv.). All reactions are performed at room temperature. | |
During the expansion of substrate scope, we observed that unsubstituted aromatic systems gave higher yields of the desired sulfides as compared to substituted aromatic systems (–EWG and –EDG). Additionally, we observed better yields when –Br is present in the benzylic system at different positions like ortho-, meta-, and para-positions, on the same sequence. Further, we also noticed slightly lower yields due to the presence of the –m effect group present in the aromatic system (–CHO, –CN group). In conclusion, the presence of an electron donating group in the benzylic system enhances the nucleophilicity of the sulfide ion, and hence favors relatively higher yields, whereas the presence of an electron withdrawing group decreases the nucleophilicity of sulfide ion and as a result, a slight reduction in the yields was observed. To study the role of chain length on the yields of sulfides, we kept the benzylic system constant and treated it with alkyl bromides of different chain lengths. We found that no drastic change in the final yields of the sulfides 9 was observed. Evidently, a slight decrease in the yields was observed as we go lower to higher carbon chains of the electrophiles. It is worth mentioning that if the series of sulfides 6b–6h would have been prepared from thiols, the required thiol would be 3-methyl-2-butene-1-thiol which has a skunky beer smell.30 This reason may be attributed to why these sulfides, nonetheless quite simple, have not been prepared before. The efficacy of this methodology is realized in the easy retrieval of such sulfides without utilizing smelly thiols (Scheme 2).
Afterward, we tried a similar strategy with benzylic systems as electrophiles and prepared symmetrical (15a–f) and unsymmetrical (16–18) sulfides containing dibenzylic moieties in good yields (Scheme 3). Higher yields were observed here as compared to when aliphatic systems as electrophiles were employed for the preparation of sulfides (Scheme 2). Next, we turned towards the preparation of bisbenzylthio systems. In this regard, several dibromides were successfully converted into bis(benzylthio) derivatives (19–24) in good to excellent yields (Scheme 4). The single-crystal structures for 20a and 21b are presented in Fig. 3.
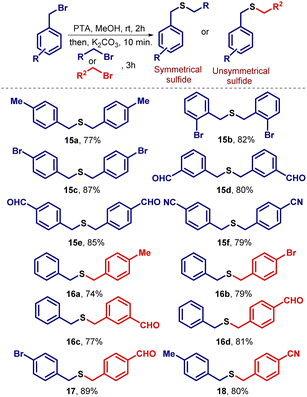 |
| Scheme 3 Synthesis of symmetrical and unsymmetrical sulfides containing aromatic rings. Reaction conditions: compound 1 (0.87 mmol, 1 equiv.), PTA (0.87 mmol, 1 equiv.), MeOH (10 ml), K2CO3 (2.61 mmol, 3 equiv.), R/R2–CH2–Br (0.87 mmol, 1 equiv.). All reactions are performed at room temperature. | |
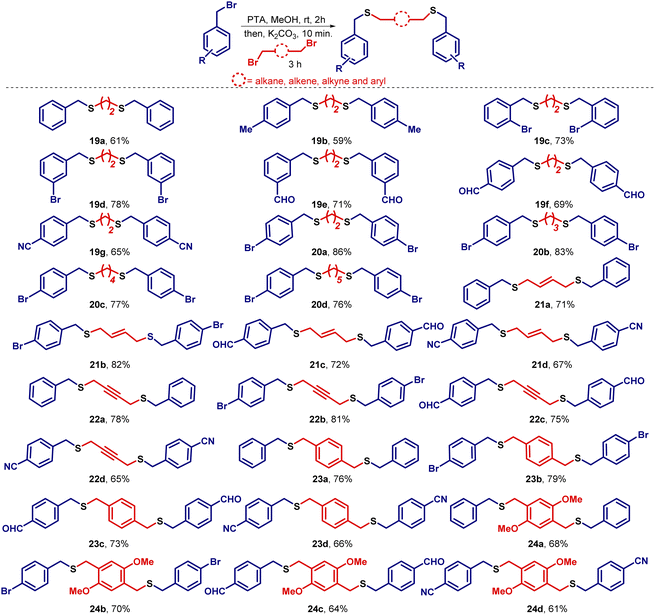 |
| Scheme 4 Synthesis of saturated and unsaturated bridges containing dithia molecules. Conditions: compound 1 (0.87 mmol, 1 equiv.), PTA (0.87 mmol, 1 equiv.), MeOH (10 ml), K2CO3 (2.61 mmol, 3 equiv.), dibromo derivative (0.44 mmol, 0.5 equiv.). All reactions are performed at room temperature. | |
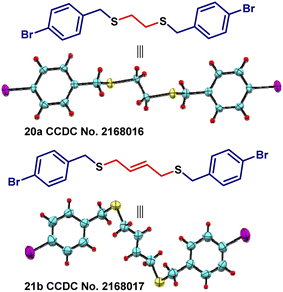 |
| Fig. 3 Single-crystal structures of 20a and 21b (shown at 50% thermal ellipsoids). | |
Sulfoxide preparation
To prepare sulfoxide and sulfone derivatives via a single-pot operation starting with benzyl bromide 1, we tried the oxidation sequence with Oxone® (Scheme 5). In this regard, we used Oxone® in water and after careful monitoring of the reaction mixture by thin-layer chromatography and by NMR data, we found the selective formation of sulfoxides in a one-pot operation. By using different equivalents of Oxone® (0.5 to 1.5 equiv.), we noticed a mixture of compounds 2, 25 (trace amount), and 26a were formed. By increasing the equivalents of Oxone® from 1.5 to 2.2 equiv., the formation of compound 26a was increased and complete conversion of sulfide into sulfoxide 26a was noticed. Earlier reports28c state that if the equivalent of Oxone® used is more than 1.5, then conversion to sulfone predominates however, in our case, even if Oxone® content was increased up to 3.0 equiv. sulfoxide was the only product obtained. This study led us to the conclusion that a controlled and easy one-pot reaction selectively provides sulfoxides as a major product directly from halides. In contrast, when we isolated and purified the sulfide 2, then subjected to oxidation with Oxone® (3 equiv.) in water
:
methanol (1
:
1), sulfone 25 was found to be the major product (Scheme 5).
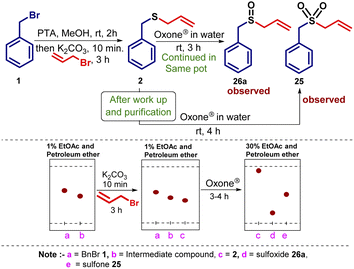 |
| Scheme 5 TLC behavior during the selective formation of sulfoxides. Conditions: compound 1 (0.87 mmol, 1 equiv.), PTA (0.87 mmol, 1 equiv.), MeOH (10 ml), K2CO3 (2.61 mmol, 3 equiv.), allylbromide (0.87 mmol, 1 equiv.). Oxone® (1.91 mmol, 2.2 equiv.) in 10 ml H2O. All reactions are performed at room temperature. | |
Substrate scope of sulfoxides
Having optimized the conditions to prepare sulfoxide in one-pot, we explored the substrate scope and studied other sulfoxides. Fortunately, we obtained diverse sulfoxides starting from benzyl bromides in a single step. Various unsymmetrical sulfoxides (26–33) prepared are included in Scheme 6. Benzyl sulfoxides (32 and 33) were obtained in good to excellent yields. A similar trend like sulfides was observed with respect to yield as discussed previously (Scheme 2).
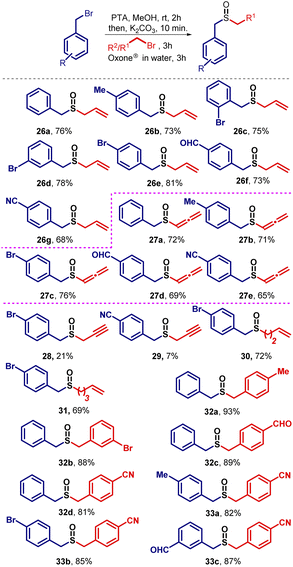 |
| Scheme 6 One-pot preparation of sulfoxides. Conditions: compound 1 (0.87 mmol, 1 equiv.), PTA (0.87 mmol, 1 equiv.), MeOH (10 ml), K2CO3 (2.61 mmol, 3 equiv.), R2/R1–CH2–Br (0.87 mmol, 1 equiv.). Oxone® (1.91 mmol, 2.2 equiv.) in 10 ml H2O. All reactions are performed at room temperature. | |
Allenes are useful synthons for several critical transformations.31 Therefore, the preparation of such sulfoxides is a worthwhile exercise. There are reports which deal with sulfide allenyl derivatives.32 However, sulfoxide allenyl derivatives are quite limited and were prepared via a multi-step synthetic protocol.33 With this methodology, they are prepared quite easily. Allenyl sulfoxides were observed when the propargyl bromides were used as electrophiles. The expected sulfoxides 28 and 29 having propargyl group as a side chain were only isolated as minor products when the aromatic ring in benzyl bromide was substituted with a –Br and –CN group at para-position respectively (Scheme 6).
Mechanistic studies
Later on, we were concerned about the mechanism of sulfoxide formation instead of sulfone (Scheme 7) even if the equivalents of Oxone® were increased from the reported methods.27c In the first step, during the formation of intermediate A, the liberation of KBr is proposed. Here, KBr is playing a prominent role when we added Oxone® to generate sulfoxides. In the presence of KBr and Oxone®, the formation of potassium sulfate and hypobromous acid (HOBr) takes place. From HOBr, bromine moiety is attached to sulfur atom and forms intermediate B containing S–Br bond which leads to the formation of intermediate C by reacting with water. Based on the mechanism as previously reported, we suggest that the formation of sulfur bromo bond is a key factor to reduce the reactivity of sulfur atom thus inhibiting further oxidation.34 Because of this bond formation, only one oxygen atom can be attached to the sulfur atom and hence the formation of the sulfoxides (26–33) predominates in a one-pot reaction.
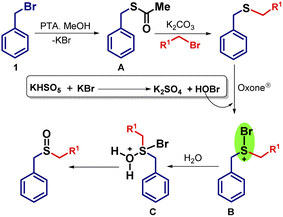 |
| Scheme 7 Plausible mechanism for the formation of sulfoxides from benzyl halides in one-pot containing oxone–KBr combination. | |
Gram scale synthesis
The methodology is tested for the gram-scale synthesis of sulfide and sulfoxide to demonstrate the synthetic utility of the developed reaction. When 1.5 g of benzyl bromide was used for gram-scale synthesis under the optimized conditions, then 1.15 g (80%) sulfide 2 and 1.20 g (76%) sulfoxide 26a were obtained respectively, hence showing the scalability of our one-pot operation (Scheme 8).
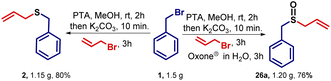 |
| Scheme 8 Gram-scale synthesis of sulfide 2 and sulfoxide 26a in one-pot. | |
Late stage functionalization
Given the importance of sulfide (thioether) in pharmaceuticals we speculated that combining the sulfide core with natural products or biologically active compounds will be a useful exercise as these products could be further useful in medicinal chemistry. Therefore, we carried out the preparation of sulfide derivatives starting with biologically active compounds by late-stage functionalization. After having bromoderivatives of citronellol, thymol, vitamin E (tocopherol), and estrone (refer ESI page S34†), we adopted the optimized conditions to prepare interesting sulfides 34 which are useful substrates for biological properties. Various derivatives prepared are listed in Scheme 9.
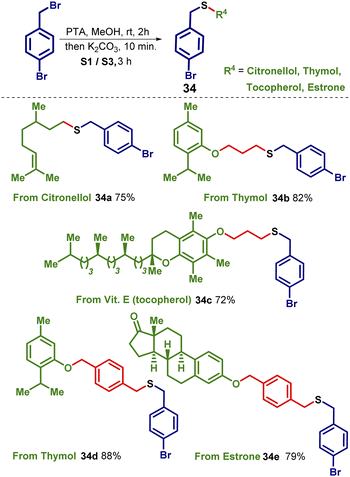 |
| Scheme 9 Late-stage functionalization of biologically active compounds into sulfides. | |
Conclusions
To summarize, a wide range of sulfur-containing scaffolds are prepared efficiently. The preparation of sulfides/sulfoxides from halides that usually requires three to four steps has been achieved in a one-pot operation. This methodology involves a thiol-free path and hence much greener and odorless than the earlier reported methods. Potassium thioacetate has acted as an efficient surrogate for unsymmetrical Csp3–S–Csp3 type sulfides which are not much explored yet. Numerous benzylic sulfides and sulfoxides containing alkyl, alkenyl, alkynyl, and phenyl moieties are prepared and their structures are unambiguously established by spectral data. Selective preparation of sulfoxides and sulfone is also realized. Allenyl sulfoxides were prepared via facile one-pot operation which can be further utilized in several transformations. A series of sulfides containing biologically active scaffolds were prepared by late-stage functionalization.
Conflicts of interest
There are no conflicts to declare.
Acknowledgements
S. K. thanks Dr P. N. Pandey (Managing Director) Penam Laboratories Ltd. for financial support. N. K. G. thanks the Council of Scientific & Industrial Research (CSIR), New Delhi for the award of a research fellowship. S. A. thanks University Grants Commission (UGC), New Delhi, India for the award of a research fellowship. The authors thank Mr Sandeep Pal for his help in the purification of some compounds.
Notes and references
-
(a) R. Zhang, H. Ding, X. Pu, Z. Qian and Y. Xiao, Recent advances in the synthesis of sulfides, sulfoxides and sulfones via C–S bond construction from non-halide substrates, Catalyst, 2020, 10, 1339 CrossRef CAS;
(b) D. Kaiser, I. Klose, R. Oost, J. Neuhaus and N. Maulide, Bond-forming and -breaking reaction at sulfur (IV): sulfoxides, sulfonium salts, sulfur ylides, and sulfinate salts, Chem. Rev., 2019, 119, 8701–8780 CrossRef CAS PubMed;
(c) W. Guo, K. Tao, W. Tan, M. Zhao, L. Zheng and X. Fan, Recent advances in photocatalytic C–S/P–S bond formation via the generation of sulfur centered radicals and functionalization, Org. Chem. Front., 2019, 6, 2048–2066 RSC;
(d) J. Li, S. Yang, W. Wu and H. Jiang, Recent developments in palladium-catalyzed C–S bond formation, Org. Chem. Front., 2020, 7, 1395–1417 RSC.
-
(a) E. A. Ilardi, E. Vitaku and J. T. Njardarson, Data-mining for sulfur and fluorine: an evaluation of pharmaceuticals to reveal opportunities for drug design and discovery, J. Med. Chem., 2014, 57, 2832–2842 CrossRef CAS PubMed;
(b) M. Feng, B. Tang, S. Liang and X. Jiang, Sulfur containing scaffolds in drugs: synthesis and application in medicinal chemistry, Curr. Top. Med. Chem., 2016, 16, 1200–1216 CrossRef CAS PubMed;
(c) K. A. Scott and J. T. Njardarson, Analysis of US FDA-approved drugs containing sulfur atoms, Top. Curr. Chem., 2018, 376, 5 CrossRef PubMed;
(d) A. S. Surur, L. Schulig and A. Link, Interconnection of sulfides and sulfoxide in medicinal chemistry, Arch. Pharm. Chem. Life Sci., 2019, 352, 1800248 Search PubMed.
-
(a) K. Takimiya, I. Osaka, T. Mori and M. Nakano, Organic semiconductors based on [1]Benzothieno[3,2-b][1]benzothiophene substructure, Acc. Chem. Res., 2014, 47, 1493–1502 CrossRef CAS PubMed;
(b) A. A. Dar, S. Ali, A. Ghosh, A. T. Khan, A. K. Dwivedi and P. K. Iyer, Synthesis of unsymmetrical sulfides catalysed by n-tetrabutyl-ammonium tribromide: a selective fluorescence probe for mercury ion, Sens. Actuators, B, 2014, 193, 509–514 CrossRef CAS;
(c) B. Xu, X. Pan and J. Zhu, Fabrication of oxidative and pH dual-responsive photonic crystals based on sulfide-containing block copolymers, ACS Appl. Polym. Mater., 2022, 4, 3315–3323 CrossRef CAS.
-
(a) C.-S. Li, A. M. Sarotti, P. Huang, U. T. Dang, J. G. Hurdle, T. P. Kondratyuk, J. M. Pezzuto, J. Turkson and S. Cao, NF-κB inhibitors, unique γ-pyranol-γ-lactams with sulfide and sulfoxide moieties from Hawaiian plant Lycopodiella cernua derived fungus Paraphaeosphaeria neglecta FT462, Sci. Rep., 2017, 7, 10424 CrossRef PubMed;
(b) N. Wang, P. Saidhareddya and X. Jiang, Construction of sulfur-containing moieties in the total synthesis of natural products, Nat. Prod. Rep., 2020, 37, 246–275 RSC.
-
(a) S. Leucht, G. Pitschel-Walz, R. R. Engel and W. Kissling, Amisulpride, an unusual “atypical” antipsychotic: a meta-analysis of randomized controlled trials, Am. J. Psychiatry, 2002, 159, 180–190 CrossRef PubMed;
(b) A. M. Giannetti, H. Wong, G. J. P. Dijkgraaf, E. C. Dueber, D. F. Ortwine, B. J. Bravo, S. E. Gould, E. G. Plise, B. L. Lum, V. Malhi and R. A. Graham, Identification, characterization, and implications of species-dependent plasma protein binding for the oral hedgehog pathway inhibitor vismodegib (GDC-0449), J. Med. Chem., 2011, 54, 2592–2601 CrossRef CAS PubMed;
(c) P. L. McCormack and G. M. Keating, Eletriptan: a review of its use in the acute treatment of migraine, Drugs, 2006, 66, 1129–1149 CrossRef CAS PubMed;
(d) S. R. Turner, J. W. Strohbach, R. A. Tommasi, P. A. Aristoff, P. D. Johnson, H. I. Skulnick, L. A. Dolak, E. R. Seest, P. K. Tomich, M. J. Bohanon, M.-M. Horng, J. C. Lynn, K.-T. Chong, R. R. Hinshaw, K. D. Watenpaugh and M. N. Janakiraman, Tipranavir (PNU-140690): a potent, orally bioavailable nonpeptidic HIV protease inhibitor of the 5, 6-dihydro-4-hydroxy-2-pyrone sulfonamide class, J. Med. Chem., 1998, 41, 3467–3476 CrossRef CAS PubMed;
(e) F. He, L. H. Mai, A. Longeon, B. R. Copp, N. Loaec, A. Bescond, L. Meijer and M.-L. Bourguet-Kondracki, Novel adociaquinone derivatives from the indonesian sponge Xestospongia sp., Mar. Drugs, 2015, 13, 2617–2628 CrossRef CAS PubMed.
- https://www.webmd.com/drugs/2/drug-64858277/singulair-oral/montelukast-oral/details.
- https://www.drugs.com/ranitidine.html.
- R. Wang, J. Chen, J. Gao, J.-A. Chen, G. Xu, T. Zhu, X. Gu, Z. Guo, W.-H. Zhu and C. Zhao, A molecular design strategy toward enzyme-activated probes with near-infrared I and II fluorescence for targeted cancer imaging, Chem. Sci., 2019, 10, 7222–7227 RSC.
-
(a) C.-C. Han and R. Balakumar, Mild and efficient methods for the conversion of benzylic bromides to benzylic thiols, Tetrahedron Lett., 2006, 47, 8255–8258 CrossRef CAS;
(b) S. Tanaka, P. K. Pradhan, Y. Maegawa and M. Kitamura, Highly efficient catalytic dehydrative S-allylation of thiols and thioic S-acids, Chem. Commun., 2010, 46, 3996–3998 RSC;
(c) M. S. Holzwarth, W. Frey and B. Plietker, Binuclear Fe-complexes as catalysts for the ligand-free regioselective allylic sulfenylation, Chem. Commun., 2011, 47, 11113–11115 RSC;
(d) C. Li, J. Li, C. Tan, W. Wu and H. Jiang, DDQ-mediated regioselective C-S bond formation: efficient access to allylic sulfides, Org. Chem. Front., 2018, 5, 3158–3162 RSC;
(e) F. Ling, T. Liu, C. Xu, J. He, W. Zhang, C. Ling, L. Liu and W. Zhong, Divergent electrolysis for the controllable, coupling of thiols with 1,2-dichloroethane: a mild approach to sulfide and sulfoxide, Green Chem., 2022, 24, 1342–1349 RSC.
- M. Soleiman-Beigia, M. Kazemia, R. Aryan and L. Shiria, TBAOH Mediated: An efficient and simple procedure for alkylation of alcohols, phenols and thiols under neat aqueous conditions, Lett. Org. Chem., 2014, 11, 321–326 CrossRef.
- J. Clayden, N. Greeves and S. Warren, in Organic Chemistry, Oxford University Press, Oxford, 2nd edn, 2012, ch. 1, pp. 3–4 Search PubMed.
-
(a) S. Kotha and A. Chavan, Design and synthesis of benzosultine-sulfone as a o-xylylene precursor via cross-enyne metathesis and rongalite: further expansion to polycyclics via regioselective Diels–Alder reaction, J. Org. Chem., 2010, 75, 4319–4322 CrossRef CAS PubMed;
(b) S. Kotha and P. Khedkhar, Differential reactivity pattern of hybrid o-quinodimethane precursors: strategic expansion to annulated benzocycloalkanes via rongalite, J. Org. Chem., 2009, 74, 5667–5670 CrossRef CAS PubMed.
-
(a) S. Kotha, N. Sreenivasachary, K. Mohanraja and S. Durani, Modification of constrained peptides by ring-closing metathesis reaction, Bioorg. Med. Chem. Lett., 2001, 11, 1421–1423 CrossRef CAS PubMed;
(b) S. Kotha, N. G. Krishna, S. Halder and S. Misra, A synergistic approach to polycyclics via a strategic utilization of Claisen rearrangement and olefin metathesis, Org. Biomol. Chem., 2011, 9, 5597–5624 RSC;
(c) S. Kotha and G. Waghule, Diversity oriented approach to crownophanes by enyne metathesis and Diels–Alder reaction as key steps, J. Org. Chem., 2012, 77, 6314–6318 CrossRef CAS PubMed;
(d) S. Kotha, M. Mesharam, P. Khedkar, S. Benerjee and D. Deodhar, Recent applications of ring-rearrangement metathesis in organic
synthesis, Beilstein J. Org. Chem., 2015, 11, 1833–1864 CrossRef CAS PubMed.
- F. Olivito, P. Costanzo, M. L. Di Gioia, M. Nardi, M. Oliverio and A. Procopio, Efficient synthesis of organic thioacetates in water, Org. Biomol. Chem., 2018, 16, 7753–7759 RSC.
-
(a) B. T. Holmes and A. W. Snow, Aliphatic thioacetate deprotection using catalytic tetrabutylammonium cyanide, Tetrahedron, 2005, 61, 12339–12342 CrossRef CAS;
(b) O. B. Wallace and D. M. Springer, Mild, selective deprotection of thioacetates using sodium thiomethoxide, Tetrahedron Lett., 1998, 39, 2693–2694 CrossRef CAS;
(c) Z. Lian, B. N. Bhawal, P. Yu and B. Morandi, Palladium-catalyzed carbon-sulfur or carbon-phosphorus bond metathesis by reversible arylation, Science, 2017, 356, 1059–1063 CrossRef CAS PubMed.
- B. Czech, S. Quici and S. L. Regen, Convenient synthesis of organic sulfides using impregnated reagents, Synthesis, 1980, 113 CrossRef CAS.
- W. F. Jarvis, M. D. Hoey, A. L. Finocchio and D. C. Dittmer, Organic reactions of reduced species of sulfur dioxide, J. Org. Chem., 1988, 53, 5750–5756 CrossRef CAS.
-
(a) S. Kotha and P. Khedkar, Rongalite: a useful green reagent in organic synthesis, Chem. Rev., 2012, 112, 1650–1680 CrossRef CAS PubMed;
(b) S. Golla, N. Anugu, S. Jalagam and H. P. Kokatla, Rongalite-induced transition-metal and hydride-free reductive aldol reaction: a rapid access to 3,3′-disubstituted oxindoles and its mechanistic studies, Org. Biomol. Chem., 2022, 20, 808–816 RSC.
- D. C. Dittmer, Sodium Hydrogen Sulfide, Encycl. Reagents Org. Synth., 2001, 11, 8861 Search PubMed.
-
(a) N. Watanabe, M. Okano and S. Uemura, The reaction of alkyl halides with mercuric thiocyanate, Bull. Chem. Soc. Jpn., 1974, 47, 2745–2748 CrossRef CAS;
(b) A. L. Schwan, Potassium Thiocyanate, Encycl. Reagents Org. Synth., 2001, 10, 8315 Search PubMed.
-
(a) I. Degani, R. Fochi and V. Regondi, The phase-transfer synthesis of unsymmetrical dialkyl sulfides via O,S dialkyl dithiocarbonates, Synthesis, 1979, 178–181 CrossRef CAS;
(b) R. N. Saičić, Potassium O-ethyl Xanthate, Encycl. Reagents Org. Synth., 2005, 10, 8169 Search PubMed.
- K. Mitamura, T. Yatabe, K. Yamamoto, T. Yabe, K. Suzuki and K. Yamaguchi, Heterogeneously Ni–Pd nanoparticle-catalyzed base-free formal C–S bond metathesis of thiols, Chem. Commun., 2021, 57, 3749–3752 RSC.
-
(a) G.-P. Lu and C. Cai, An odorless, one-pot synthesis of nitroaryl thioethers via SNAr reactions through the in situ generation of S-alkylisothiouronium salts, RSC Adv., 2014, 4, 59990–59996 RSC;
(b) P. Gogoi, S. Hazarika and P. Barman, Role of TBATB in nano indium oxide catalyzed CS bond formation, Sci. Rep., 2015, 5, 13873 CrossRef PubMed;
(c) Y.-M. Lin, G.-P. Lu, G.-X. Wang and W.-B. Yi, Odorless, regioselective synthesis of diaryl sulfides and α-triaryl carbonyls from sodium arylsulfinates via a metal-free radical strategy in water, Adv. Synth. Catal., 2016, 358, 4100–4105 CrossRef CAS;
(d) X. Ma, L. Yu, C. Su, Y. Yang, H. Li and Q. Xu, Efficient generation of C–S bonds via a by-product-promoted selective coupling of alcohols, organic halides, and thiourea, Adv. Synth. Catal., 2017, 359, 1649–1655 CrossRef CAS;
(e) Y.-M. Lin, G.-P. Lu, G.-X. Wang and W.-B. Yi, Acid/phosphide-induced radical route to alkyl and alkenyl, sulfides and phosphonothioates from sodium arylsulfinates in water, J. Org. Chem., 2017, 82, 382–389 CrossRef CAS PubMed;
(f) Z. Liang, K. Lv, S. Zhou, C. Zhu and X. Bao, Visible-light photocatalytic preparation of alkenyl thioethers from 1,2,3-thiadiazoles and Hantzsch esters: synthetic and mechanistic investigations, Org. Chem. Front., 2021, 8, 6499–6507 RSC;
(g) D. Wang, L. Zhang, F. Xiao, G. Mao and G.-J. Deng, The electrochemically selective C3-thiolation of quinolones, Org. Chem. Front., 2022, 9, 2986–2993 RSC.
-
(a) F. Shi, M. K. Tse, H. Martin Kaiser and M. Beller, Self-catalyzed oxidation of sulfides with hydrogen peroxide: a green and practical process for the synthesis of sulfoxides, Adv. Synth. Catal., 2007, 349, 2425–2430 CrossRef CAS;
(b) B. Li, A.-H. Liu, L.-N. He, Z.-Z. Yang, J. Gao and K.-H. Chen, Iron-catalyzed selective oxidation of sulfides to sulfoxides with the polyethylene glycol/O2 system, Green Chem., 2012, 14, 130–135 RSC;
(c) A. Rezaeifard, R. Haddad, M. Jafarpour and M. Hakimi, {Mo132} Nanoball as an efficient and cost-effective catalyst for sustainable oxidation of sulfides and olefins with hydrogen peroxide, ACS Sustainable Chem. Eng., 2014, 2, 942–950 CrossRef CAS;
(d) C. Li, N. Mizuno, K. Murata, K. Ishii, T. Suenobu, K. Yamaguchi and K. Suzuki, Selectivity switch in the aerobic oxygenation of sulfides photocatalysed by visible-light-responsive decavanadate, Green Chem., 2020, 22, 3896–3905 RSC;
(e) P.-W. Yang, X.-X. Liu, X.-Q. Li and M.-X. Wei, Transition metal-free and solvent-free calcium carbide promotes the formation of β-keto sulfoxide from acyl chloride and DMSO, Org. Chem. Front., 2021, 8, 2914–2918 RSC.
-
(a) Z. Han, D. C. Reeves, D. Krishnamurthy and C. H. Senanayake, Comprehensive Chirality, Elsevier, 2012, vol. 3, pp. 560–600 Search PubMed;
(b) E. M. Skoda, J. R. Sacher, M. Z. Kazancioglu, J. Saha and P. Wipf, An uncharged oxetanyl sulfoxide as a covalent modifier for improving aqueous solubility, ACS Med. Chem. Lett., 2014, 5, 900–904 CrossRef CAS PubMed;
(c) K. V. Goncharenko, A. Vit, W. Blankenfeldt and F. P. Seebeck, Structure of the sulfoxide synthase EgtB from the ergothioneine biosynthetic pathway, Angew. Chem., Int. Ed., 2015, 54, 2821–2824 CrossRef CAS PubMed.
-
(a) Z. He, A. P. Pulis and D. J. Procter, The interrupted Pummerer reaction in a sulfoxide-catalyzed oxidative coupling of 2-naphthols, Angew. Chem., Int. Ed., 2019, 58, 7813–7817 CrossRef CAS PubMed;
(b) B. M. Trost and M. Rao, Development of chiral sulfoxide ligands for asymmetric catalysis, Angew. Chem., Int. Ed., 2015, 54, 5026–5043 CrossRef CAS PubMed;
(c) H. Pellissier, Use of chiral sulfoxides in asymmetric synthesis, Tetrahedron, 2006, 62, 5559–5601 CrossRef CAS;
(d) J. Han, V. A. Soloshonok, K. D. Klika, J. Drabowicz and A. Wzorek, Chiral sulfoxides: advances in asymmetric synthesis and problems with the accurate determination of the stereochemical outcome, Chem. Soc. Rev., 2018, 47, 1307–1350 RSC.
-
(a) V. H. Kassin, D. V. Silva-Brenes, T. Bernard, J. Legros and J. M. Monbaliu, A continuous flow generator of organic hypochlorites for the neutralization of chemical warfare agent simulants, Green Chem., 2022, 24, 3167–3179 RSC;
(b) P. Zhang, Y. Wang, H. Li and M. Antonietti, Metal-free oxidation of sulfides by carbon nitride with visible light illumination at room temperature, Green Chem., 2012, 14, 1904–1908 RSC;
(c) Y. Zhu, X. Qiu, S. Zhao, J. Guo, X. Zhang, W. Zhao, Y. Shi and Z. Tang, Structure regulated catalytic performance of gold nanocluster-MOF nanocomposites, Nano Res., 2020, 13, 1928–1932 CrossRef CAS.
-
(a) K.-J. Liu, Z. Wang, L.-H. Lu, J.-Y. Chen, F. Zeng, Y.-W. Lin, Z. Cao, X. Yud and W.-M. He, Synergistic cooperative effect of CF3SO2Na and bis(2-butoxyethyl)ether
towards selective oxygenation of sulfides with molecular oxygen under visible-light irradiation, Green Chem., 2021, 23, 496–500 RSC;
(b) K.-J. Liu, J.-H. Deng, J. Yang, S.-F. Gong, Y.-W. Lin, J.-Y. He, Z. Cao and W.-M. He, Selective oxidation of (hetero) sulfides with molecular oxygen under clean conditions, Green Chem., 2020, 22, 433–438 RSC;
(c) B. Yu, A.-H. Liu, L. N. He, B. Li, Z.-F. Diao and Y.-N. Li, Catalyst-free approach for solvent-dependent selective oxidation of organic sulfides with oxone, Green Chem., 2012, 14, 957–962 RSC.
- B. M. Trost and D. P. Curran, Chemoselective oxidation of sulfides to sulfones with potassium hydrogen persulfate, Tetrahedron Lett., 1981, 22, 1287–1290 CrossRef CAS.
- J. Clayden, N. Greeves and S. Warren, in Organic Chemistry, Oxford University Press, Oxford, 2nd edn, 2012, ch. 1, p. 4 Search PubMed.
- K. Mislow, H. M. Green, P. Laur, J. T. Melillo, T. Simmons and A. L. Ternay, Absolute configuration and optical rotatory power of sulfoxides and sulfinate esters1,2, J. Am. Chem. Soc., 1965, 87, 1958–1976 CrossRef CAS.
- H. Luo and S. Ma, CuI-catalyzed synthesis of functionalized terminal allenes from 1-alkynes, Eur. J. Org. Chem., 2013, 2013, 3041–3048 CrossRef CAS.
- A. B. Riddell, M. M. Michalski, M. R. Snowdon, M. J. Hirst and A. L. Schwan, N-Sulfanilamides as the sulfur source for alkyl allenyl sulfoxides via [2,3]-sigmatropic rearrangement, ChemistrySelect, 2021, 6, 11331–11336 CrossRef CAS.
- R. V. Kupwade, S. S. Khot, U. P. Lad, U. V. Desai and P. P. Wadgaonkar, Catalyst-free oxidation of sulfides to sulfoxides and diethylamine catalyzed oxidation of sulfides to sulfones using Oxone® as an oxidant, Res. Chem. Intermed., 2017, 43, 6875–6888 CrossRef CAS.
|
This journal is © The Royal Society of Chemistry 2022 |
Click here to see how this site uses Cookies. View our privacy policy here.