DOI:
10.1039/D2RA04387D
(Paper)
RSC Adv., 2022,
12, 25587-25592
Aromatic fluorine atom-induced highly amine-sensitive trimethine cyanine dye showing colorimetric and ratiometric fluorescence change†
Received
16th July 2022
, Accepted 1st September 2022
First published on 7th September 2022
Abstract
Herein, introducing multiple fluorine atoms into aromatic rings of trimethine cyanine dyes is proposed as a powerful method for dramatically increasing sensitivity to amines. The highly sensitive ratiometric fluorescence properties previously available only by intramolecular addition can be exploited in reactions with intermolecular amines or other nucleophiles.
Introduction
Ratiometric fluorescence, a method wherein the ratio of fluorescence intensities at two or more wavelengths are used, has attracted much attention in analytical sensing and optical imaging because it can reduce the effects of many factors such as dye concentration, surrounding pH, polarity, and changes in environmental temperature compared to when single fluorescence intensity is measured.1–4
Amines could cause serious damage in public health and the environment owing to their toxic nature, so, simple, fast, low-cost, and accurate amine monitoring systems are becoming highly urgent.5 The detection of amines using ratiometric fluorescence through utilized intramolecular charge transfer (ICT),6–17 twisted ICT,18 aggregation-induced emission,19–23 and excited state intramolecular proton transfer24 has been reported.
Polymethine cyanine dyes have several features such as good fluorescence quantum yield and the ability to easily prepare the fluorescence wavelength by changing the methine chain length.25–27 However, they lack reactivity with amines, and the reported examples are limited to reactions with intramolecular amines.28–34 Herein, we report that the prepared ring-perfluorinated trimethine cyanine dye 2a (Fig. 1) has a significantly higher response to n-hexylamine than the non-fluorinated dye 2b. The dye 2a exhibited a dual change in the solution and fluorescence color at widely shifted wavelengths, visible to the naked eye.
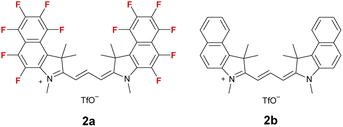 |
| Fig. 1 Structure of trimethine cyanine dyes 2a and 2b. | |
Furthermore, dye 2a adsorbed on the filter paper could be repeatedly used because the orange emission was instantly converted into blue by the amine vapor and returned to its original color after drying rapidly in the air. These results are the first example of the highly sensitive ratiometric fluorescence properties of polymethine cyanine dyes with intermolecular amines and advance the development of rapid and reversible vapochromic response materials to amines with two changes in papers and emission color.
Results and discussion
The responsivity of the ring-perfluorinated trimethine cyanine dyes 2a and 2b to n-hexylamine in tetrahydrofuran (THF) stored overnight in the dark at 25 °C were investigated; the results are summarized in Fig. 2 and Table 1. n-Hexylamine was used as a representative example of a primary aliphatic amine because it is not easily volatilized. As the amount of n-hexylamine gradually increased, the orange fluorescence at 596 nm from dye 2a decreased, and the blue emission at 404 nm increased at an excitation of 257 nm. This phenomenon led to the disappearance of the solution color and a shift in the fluorescence color from orange through pink to blue, according to the CIE color system. Thus, dye 2a was highly sensitive, completing the reaction with only 2 equiv. of the amine. For dye 2b, the red fluorescence at 612 nm diminished, and the blue fluorescence at 380 nm increased significantly at an excitation of 250 nm, although 6400 equiv. of the amine was required to complete the reaction with the non-fluorinated dye 2b. These results suggest that replacing all the hydrogen atoms with fluorine on the aromatic rings dramatically improved the sensitivity of trimethine cyanine dye to the amine.
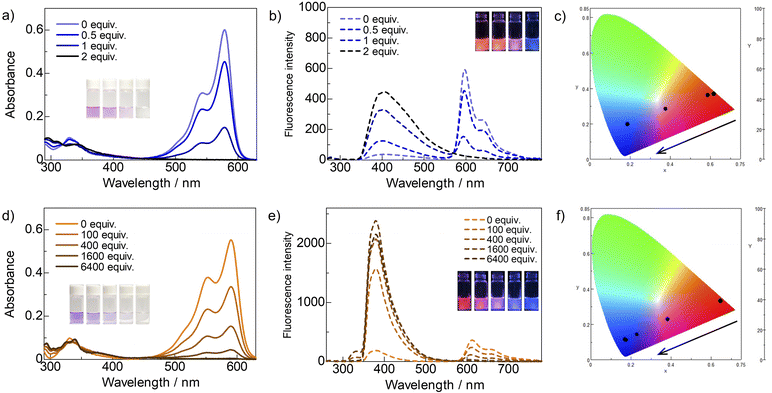 |
| Fig. 2 (a) Ultraviolet-visible (UV-vis), (b) fluorescence spectra, and (c) CIE color systems of dye 2a (5 × 10−6 M) in THF stored overnight in the dark at 25 °C after adding n-hexylamine. (d) UV-vis, (e) fluorescence spectra, and (f) CIE color systems of dye 2b under similar conditions. The excitation wavelengths were determined by the excitation spectra (Fig. S2†) and were as follows: 257 nm (dye 2a), 250 nm (dye 2b), and 365 nm (CIE color systems). Inset photos were obtained under daylight and UV light (λ = 365 nm), and the order of amine concentration is left to right. | |
Table 1 Optical properties of dyes 2a and 2b in THF with n-hexylamine
Dyea |
n-Hexylamine (equiv.) |
λmax (nm) (ε (M−1 cm−1)) |
λex (nm) |
Fmax (nm)b |
φfc |
τs (ns)e |
Measured dye 2 (5 × 10−6 M) in THF stored overnight in the dark at 25 °C after adding n-hexylamine. Measured using excitation wavelength (λex) at low sensitivity. Measured using an integrating sphere method excited at λex. Measured by excitation at 290 nm. Measured using a single-photon-counting method. |
2a |
0 |
579 (120 000) |
580 |
596 |
0.14 |
0.7 |
0.5 |
579 (91 000) |
— |
— |
— |
— |
1 |
579 (30 000) |
— |
— |
— |
— |
2 |
579 (200) |
257 |
404 |
0.04d |
6.1 |
2b |
0 |
591 (110 000) |
591 |
612 |
0.10 |
0.5 |
6400 |
254 |
250 |
380 |
0.24 |
12.5 |
The fluorescence quantum yields for dyes 2a and 2b were 14 and 10%, respectively. When the dyes reacted with the amine, the maximum excitation wavelength overlapped with the absorption band of THF; therefore, excluding this band, the dyes were excited at 290 nm, with fluorescence quantum yields of 4 and 24%, respectively. Furthermore, a calibration curve for the fluorescence intensity ratio of dye 2a (I404 nm/I596 nm) versus the concentration of n-hexylamine demonstrated good linearity (R2 = 0.988), while the estimated detection limit (3σ/slope) was 17 nM, as shown in Fig. 3. The obtained value shows sensitivity as high as that of an activated ester and aldehydes.8,10,11
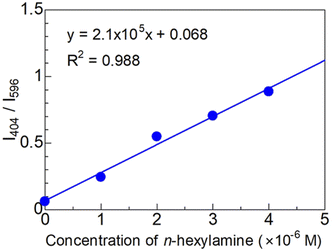 |
| Fig. 3 Calibration curve for the fluorescence intensity ratio (I404 nm/I596 nm) of dye 2a vs. the concentration of n-hexylamine (0–4 × 10−6 M). | |
Next, absorbance changes in various solvents were followed to examine the sensitivity of dye 2a to n-hexylamine (Fig. S1†). The results revealed that this reaction was primarily promoted by THF, followed by other solvents in the order dimethyl sulfoxide (DMSO), methyl alcohol (MeOH), acetone, chloroform, and dichloromethane (DCM). The large difference in the reactivity was related to the Gutmann donor number (DN) and acceptor number (AN) of solvent molecules, which are the empirical measures of the nucleophilic and electrophilic properties of the solvents.35,36
Solvents with high DN can stabilize cations,37 while an opposite phenomenon is observed for AN. Therefore, the reaction of the dye with the amine is more likely to proceed in THF and DMSO, which have larger DN and/or smaller AN than acetone, MeOH, chloroform, and DCM, because the cationic dye is most involved in this system. The DN and AN of these solvents are listed in Table S1.†
Other analytes, such as not only other amines (aniline, triethylamine, and diethylamine) but also anions (perchlorate, acetate, bromide, chloride, and fluoride) in THF were also examined. The results are summarized in Fig. 4. The reactions of dye 2a with 2 equiv. of amines such as triethylamine and diethylamine occurred smoothly, and the color of the fluorescent changed from orange to blue, as in the case of n-hexylamine, as illustrated in Fig. 4(c). Although, accurate measurement for aniline was not possible due to its fluorescence, the dye-derived maximum absorption was slightly reduced.
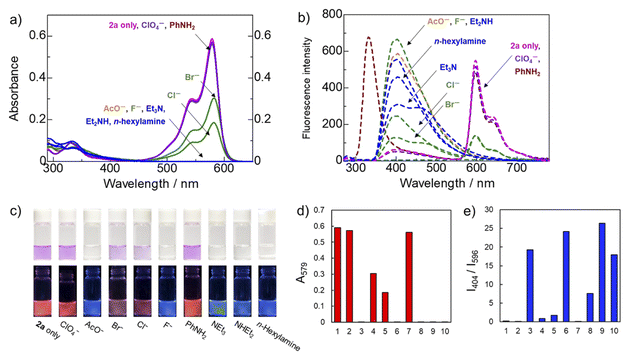 |
| Fig. 4 (a) UV-vis, (b) fluorescence spectra, and (c) the photos of dye 2a (5 × 10−6 M) in THF stored overnight in the dark at 25 °C after adding various analytes (2 equiv.). The excitation wavelength was 257 nm. (d) Absorbance at 579 nm and (e) fluorescence intensity ratio (I404 nm/I596 nm) were calculated from the UV-vis and fluorescence spectra, respectively. Analytes are as follows: (1) 2a only, (2) ClO4−, (3) AcO−, (4) Br−, (5) Cl−, (6) F−, (7) PhNH2, (8) NEt3, (9) NHEt2, and (10) n-hexylamine. Photos were captured under daylight and UV light (λ = 365 nm) with various analytes. The counter cations of these anionic species are all Bu4N+. | |
Using 2 equiv. of a couple of anions, such as acetate and fluoride,38 also resulted in observation to change the fluorescent color from orange to blue, as in the case of n-hexylamine, as illustrated in Fig. 4(c). The detection of other anionic species, such as bromide and chloride, demonstrated only moderate changes in the fluorescent color and reacted slightly with perchlorate.
To observe the difference in the detection of these neutral and anionic nucleophiles, UV-vis and fluorescence spectra of dye 2a were measured in detail in THF with different equiv. of not only diethylamine and triethylamine but also acetate and fluoride anions, were carried out. The results are summarized in Fig. 5. It was found that the highly nucleophilic anion, such as acetate and fluoride are more reactive than n-hexylamine. Detection of diethylamine appears to be similar to that of n-hexylamine, but somewhat lower for the tertiary triethylamine.
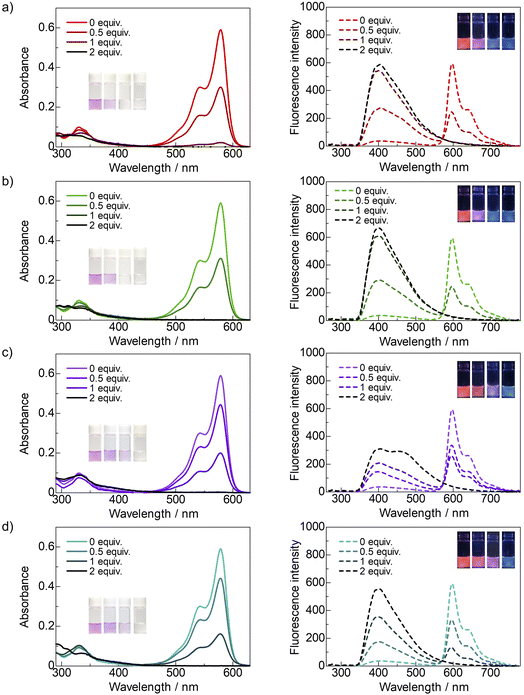 |
| Fig. 5 UV-vis and fluorescence spectra of dye 2a (5 × 10−6 M) in THF stored overnight in the dark at 25 °C after adding analytes which are as follows: (a) AcO−, (b) F−, (c) NEt3, and (d) NHEt2. The counter cations of these anionic species are all Bu4N+. | |
Importantly, the ring-perfluorinated trimethine cyanine dye 2a has a significantly higher response to very small amounts of neutral amines than the non-fluorinated dye 2b, and underwent a dual change in the solution and fluorescent. The reason can seem to be that the introduction of fluorine atoms into the aromatic ring significantly lowers the LUMO of trimethine cyanine dye 2a and promotes the addition reaction of even neutral amines, as shown in Fig. 6.39
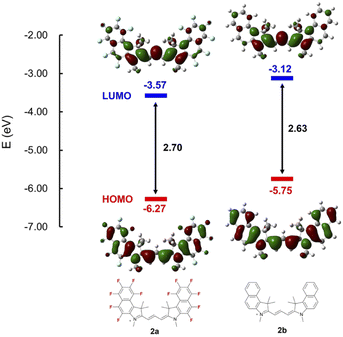 |
| Fig. 6 Energy diagrams and molecular orbitals of the cationic parts of dyes obtained using DFT calculations at the B3LYP/6-31+G(d,p) level. | |
For the reaction mechanism, the results of nuclear magnetic resonance (NMR) and high-resolution mass spectrometry illustrated in Fig. 7, S3, and S4† suggest the 1,2-addition of n-hexylamine to the iminium carbon of dye 2a. In particular, 1H NMR confirmed that the doublet signal split in two and the triplet signal changed to a double doublet, along with an up-field shift in all signals regarding the methine chain protons. Furthermore, the amine-added dyes 2a and 2b were characterized by MS analysis (Fig. S4†), where peaks at m/z 774.2719 (calculated = 774.2718, [C39H36F12N3]+) and m/z 558.3849 (calculated = 558.3848, [C39H48N3]+) were detected, respectively. Although it was difficult to measure the non-fluorinated dye 2b by NMR owing to its poor reactivity with amines, it is expected to react with amines in the manner similar to that as the fluorinated dye 2a because of its very similar structure and the MS result.
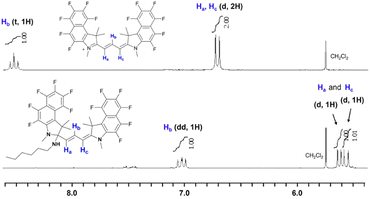 |
| Fig. 7 1H NMR analysis for the reaction of dye 2a (1 × 10−5 mol) with n-hexylamine (20 equiv.) in DMSO-d6. | |
In order to assess the versatility of application, the repeatability of dye 2a was investigated using filter paper, as shown in Fig. 8.
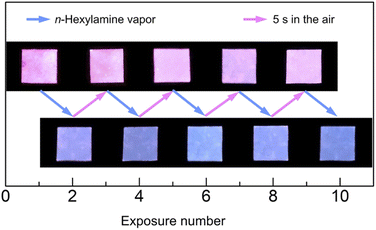 |
| Fig. 8 Repeatability of fluorescence using filter paper adsorbed with dye 2a upon alternative exposure to n-hexylamine vapor and drying in the air. The inset photos were captured under UV light (λ = 365 nm). | |
The filter paper for dye adsorption was fabricated by soaking a white filter paper overnight in the DCM solution of dye 2a (5 × 10−4 M) and then drying in the dark for 1 h at 25 °C. Exposure to n-hexylamine vapor resulted in rapid disappearance of the pink of the filter paper and change in the fluorescence from orange to blue. Subsequently, drying in the air caused the pink of the filter paper to return to pink and emit orange fluorescence. This reversible change in fluorescent color was particularly pronounced, with the blue fluorescence reverting to orange in only 5 s when kept in the air after exposure to the amine (see Video†).
Conclusions
We reported that the ring-perfluorinated trimethine cyanine dye 2a was highly sensitive to n-hexylamine and underwent a dual change in the solution and fluorescent color with a large shift. The methodology of introducing many fluorine atoms proved valuable for efficiently enhancing the sensitivity of the dye to amines. In addition, the fluorinated dye 2a adsorbed on the filter paper exhibited reversible ratiometric fluorescence properties, such that the orange emission was instantly changed to blue by the amine vapor and returned to its original color when dried in the air for 5 s.
Experimental
Materials
Acetone, methanol, and ethyl acetate were purchased from Kanto Chemical Industry Co., Inc. Tetrahydrofuran (THF; stabilizers not included), dimethyl sulfoxide, chloroform, triethylamine, aniline, and tetrabutylammonium chloride were purchased from FUJIFILM Wako Pure Chemical Co. n-Hexylamine, diethylamine, tetrabutylammonium fluoride, and tetrabutylammonium acetate were purchased from TCI Co., Ltd. Tetrabutylammonium bromide was purchased from Sigma-Aldrich. Tetrabutylammonium perchlorate and dichloromethane were purchased from Nacalai Tesque Inc. Dyes 2a and 2b were synthesized by our method.40
Measurement
1H nuclear magnetic resonance (NMR) spectra were measured at 392 or 400 MHz in hexadeuterodimethyl sulfoxide ((CD3)2SO) solutions with residual solvents as internal standards using a JEOL ECS-400 or ECX-400P FT-NMR spectrometer. 13C NMR spectra were obtained at 99 or 101 MHz in (CD3)2SO solution with residual solvents as internal standards using a JEOL ECS-400 or ECX-400P FT-NMR spectrometer. 19F NMR spectra were recorded at 369 or 376 MHz in (CD3)2SO solutions using a JEOL ECS-400 or ECX-400P FT-NMR spectrometer. The data were reported as (s = singlet, d = doublet, t = triplet, q = quartet, m = multiplet, br s = broad singlet, coupling constant(s), integration). Electrospray ionization mass spectroscopy (ESI-MS) analysis using MeOH was performed with a JEOL JMS-T100LP (AccuTOF LC-plus). Ultraviolet-visible absorption spectra were obtained using a Hitachi U-4100. The fluorescence spectra were acquired using a Jasco FP-8600 spectrofluorometer. The absolute fluorescence quantum yields were obtained on Hamamatsu Quantaurus-QY C11347-01. The fluorescence lifetimes were measured using Hamamatsu Quantaurus-Tau compact fluorescence lifetime spectrometer C11367-01.
Determination of limit of detection (LOD)
The fluorescence intensity ratio at 404 nm and 596 nm of the ring-perfluorinated dye 2a in THF was collected 10 times to obtain the background noise σ. The fluorescence intensity ratios were measured overnight after adding different concentrations of n-hexylamine. LOD (3σ/slope) was calculated as three times the background noise σ divided by the slope of the fluorescence intensity ratio fitted to a calibration curve as a function of the amine concentration.
Author contributions
KF and RK conceived and designed the project. RK performed all experiments, analysed the data, and wrote the manuscript. YK and TI contributed to useful discussions. KF reviewed and modified the manuscript.
Conflicts of interest
There are no conflicts to declare.
Acknowledgements
This work is supported by the “Interdisciplinary Frontier Next-Generation Researcher Program of the Tokai Higher Education and Research System” to RK from JST SPRING, grant number JPMJSP2125. This work was partially supported by JSPS KAKENHI grant numbers JP20K05647 to KF. We would like to thank Editage (http://www.editage.com) for English language editing.
Notes and references
- R. Gui, H. Jin, X. Bu, Y. Fu, Z. Wang and Q. Liu, Coord. Chem. Rev., 2019, 383, 82–103 CrossRef CAS.
- M. H. Lee, J. S. Kim and J. L. Sessler, Chem. Soc. Rev., 2015, 44, 4185–4191 RSC.
- J. Fan, M. Hu, P. Zhan and X. Peng, Chem. Soc. Rev., 2013, 42, 29–43 RSC.
- T. Doussineau, A. Schulz, A. Lapresta-Fernandez, A. Moro, S. Korsten, S. Trupp and G. J. Mohr, Chem.–Eur. J., 2010, 16, 10290–10299 CrossRef CAS PubMed.
- L. Wang, X. Ran, H. Tang and D. Cao, Dyes Pigm., 2021, 194, 109634 CrossRef CAS.
- S. Zhang, Z. Li, B. Zhang, F. Dong, B. Han, J. Lv, Y. Sun, H. Lu, Y. Yang and H. Ma, New J. Chem., 2021, 45, 20441–20446 RSC.
- Z. Quan, H. He, H. Zhou, Y. Liang, L. Wang, S. Tian, H. Zhu and S. Wang, Sens. Actuators, B, 2021, 333, 129535 CrossRef CAS.
- S. Sawminathan, S. Munusamy, D. Jothi and S. K. Iyer, ChemistrySelect, 2021, 6, 858–864 CrossRef CAS.
- H. Nawaz, J. Zhang, W. Tian, K. Jin, R. Jia, T. Yang and J. Zhang, J. Hazard. Mater., 2020, 387, 121719 CrossRef CAS PubMed.
- M. Saravanakumar, B. Umamahesh, R. Selvakumar, J. Dhanapal, S. K. A. Kumar and K. I. Sathiyanarayanan, Dyes Pigm., 2020, 178, 108347 CrossRef.
- S. Jeon, T.-I. Kim, H. Jin, U. Lee, J. Bae, J. Bouffard and Y. Kim, J. Am. Chem. Soc., 2020, 142, 9231–9239 CrossRef CAS PubMed.
- R. Jia, W. Tian, H. Bai, J. Zhang, S. Wang and J. Zhang, Nat. Commun., 2019, 10, 795 CrossRef CAS PubMed.
- D. Kim, S. W. Cho, Y. W. Jun and K. H. Ahn, Bull. Korean Chem. Soc., 2017, 38, 995–996 CrossRef CAS.
- S. Mallick, F. Chandra and A. L. Koner, Analyst, 2016, 141, 827–831 RSC.
- D. Meng, S. Yang, D. Sun, Y. Zeng, J. Sun, Y. Li, S. Yan, Y. Huang, C. W. Bielawski and J. Geng, Chem. Sci., 2014, 5, 3130–3134 RSC.
- J.-T. Hou, K. Li, B.-Y. Liu, Y.-X. Liao and X.-Q. Yu, Tetrahedron, 2013, 69, 2118–2123 CrossRef CAS.
- S.-i. Sasaki, Y. Kotegawa and H. Tamiaki, Tetrahedron Lett., 2006, 47, 4849–4852 CrossRef CAS.
- T. Han, W. Wei, J. Yuan, Y. Duan, Y. Li, L. Hu and Y. Dong, Talanta, 2016, 150, 104–112 CrossRef CAS.
- M. Kataria, J. Hwang, H. D. Chau, N. Y. Kwon, D. W. Lee, T. Kim, K. Lee, Y. Kim, S. Park and D. H. Choi, Sens. Actuators, B, 2021, 343, 130110 CrossRef CAS.
- R. Roy, N. R. Sajeev, V. Sharma and A. L. Koner, ACS Appl. Mater. Interfaces, 2019, 11, 47207–47217 CrossRef CAS PubMed.
- S. Pramanik, H. Deol, V. Bhalla and M. Kumar, ACS Appl. Mater. Interfaces, 2018, 10, 12112–12123 CrossRef CAS.
- S. Lohar, D. A. Safin, A. Sengupta, A. Chattopadhyay, J. S. Matalobos, M. G. Babashkina, K. Robeyns, M. P. Mitoraj, P. Kubisiak, Y. Garcia and D. Das, Chem. Commun., 2015, 51, 8536–8539 RSC.
- A. Ghosh, A. Sengupta, A. Chattopadhyay and D. Das, Chem. Commun., 2015, 51, 11455–11458 RSC.
- S. Zheng, Y. Fang, Y. Chen, Q. Kong, F. Wang and X. Chen, Spectrochim. Acta, Part A, 2022, 267, 120616 CrossRef CAS.
- K. Ilina and M. Henary, Chem. - Eur. J., 2021, 27, 4230–4248 CrossRef CAS.
- W. Sun, S. Guo, C. Hu, J. Fan and X. Peng, Chem. Rev., 2016, 116, 7768–7817 CrossRef CAS PubMed.
- A. Mishra, R. K. Behera, P. K. Behera, B. K. Mishra and G. B. Behera, Chem. Rev., 2000, 100, 1973–2012 CrossRef CAS PubMed.
- A. Mukherjee, P. C. Saha, R. S. Das, T. Bera and S. Guha, ACS Sens., 2021, 6, 2141–2146 CrossRef CAS PubMed.
- H. Mu, K. Miki, H. Harada, K. Tanaka, K. Nogita and K. Ohe, ACS Sens., 2021, 6, 123–129 CrossRef CAS PubMed.
- M. Oe, K. Miki and K. Ohe, Org. Biomol. Chem., 2020, 18, 8620–8624 RSC.
- M. Oe, K. Miki, H. Mu, H. Harada, A. Morinibu and K. Ohe, Tetrahedron Lett., 2018, 59, 3317–3321 CrossRef CAS.
- H. Mu, K. Miki, Y. Takahashi, N. Teshima, M. Oe, K. Kojima and K. Ohe, Chem. Lett., 2018, 47, 1147–1150 CrossRef CAS.
- K. Miki, K. Kojima, K. Oride, H. Harada, A. Morinibu and K. Ohe, Chem. Commun., 2017, 53, 7792–7795 RSC.
- L. He, W. Lin, Q. Xu, M. Ren, H. Wei and J.-Y. Wang, Chem. Sci., 2015, 6, 4530–4536 RSC.
- E. V. Vetrova, N. I. Makarova, N. I. Omelichkin, L. G. Minyaeva, V. V. Chernyavina, R. N. Borisenko and A. V. Metelitsa, J. Photochem. Photobiol., A, 2019, 370, 127–134 CrossRef CAS.
- V. Gutmann, The Donor-Acceptor Approach to Molecular Interactions, Springer, New York, 1978 Search PubMed.
- E. A. Ribeiro, T. Sidooski, L. G. Nandi and V. G. Machado, Spectrochim. Acta, Part A, 2011, 81, 745–753 CrossRef CAS.
- There is a report where an anionic fluoride ion can be detected via addition to non-fluorinated cyanine dye, see Y. Wang, S.-Y. Gwon, Y.-A. Son and S.-H. Kim, Mol. Cryst. Liq. Cryst., 2012, 566, 61–62 CrossRef CAS.
- Introduction CN groups also effective for detection of amines via addition to the fluorescent dyes, see Z. Li, R. Toivola, J. Yang, P.-N. Lai, T. Howie, S.-H. Jang, B. D. Flinn and A. K.-Y. Jen, Adv. Mater., 2016, 28, 6592–6597 CrossRef CAS.
- K. Funabiki, Y. Saito, T. Kikuchi, K. Yagi, Y. Kubota, T. Inuzuka, Y. Miwa, M. Yoshida, O. Sakurada and S. Kutsumizu, J. Org. Chem., 2019, 84, 4372–4380 CrossRef CAS PubMed.
|
This journal is © The Royal Society of Chemistry 2022 |
Click here to see how this site uses Cookies. View our privacy policy here.