DOI:
10.1039/D2RA03969A
(Paper)
RSC Adv., 2022,
12, 25025-25034
High-voltage pulse-assisted extraction of flavonoids from kapok using deep eutectic solvent aqueous solutions†
Received
28th June 2022
, Accepted 24th August 2022
First published on 2nd September 2022
Abstract
In this study, deep eutectic solvents coupled with a pulsed electric field (PEF-DES) were first applied to the extraction of traditional Chinese medicine plants. This study uses the PEF-DES extraction technique to extract TG-KF (Kapok flavonoid solution extracted with DES-TG). PEF-DES is a simple, effective and environmentally-friendly technology and can be used in industrial-scale production. For the optimal extraction conditions of TG-KF, DES-TG was used as a solvent, the DES-TG concentration was 50%, the solid–liquid ratio was 1
:
30, the electric field intensity was 0.55 kV cm−1, the number of pulses was 100, and the yield of flavonoids was 14.36 ± 0.35%. TG-KF has very good stability and there is no precipitation or discoloration within 6 months. The results of chicken embryo experiments and human patch tests show that 10% TG-KF aqueous solution has no irritation. DPPH experiments show that TG-KF has excellent efficacy as an antioxidant. Overall, TG-KF is expected to become a potential antioxidant raw material.
1. Introduction
Kapok is a unique plant in Southern China. As a medicinal and edible plant, it has analgesic,1 anti-inflammatory,2 antioxidative,3 antibacterial,4,5 whitening6 and other physiological activities. The main chemical components in kapok are flavonoids, organic acids, sterols, triterpenes, coumarins, etc.7 Flavonoids are the major chemical components of kapok. Flavonoids are a family of natural substances with various phenolic structures that are widely distributed in the plant kingdom along with multiple biological activities.8 Most recent studies working on extraction mention that flavonoids in kapok have been processed using organic solvents or water-mixed organic solvents due to the low aqueous solubility of flavonoids.9
With the development of green chemistry, many researchers have focused more on the discovery and application of environmental-friendly solvent. DES, a eutectic mixture consist of hydrogen bond acceptors (HBA) and hydrogen bond donors (HBD) at room temperature,10 is a new type of green solvent.11 Since the emergence of DES, the green properties of DES have caught considerable attentions. Based on preliminary researches, DES, as eco-friendly solvents, have been applied widely to extraction or separation of target compounds from crude plant extracts. Most DES are easy to prepare, inexpensive, and biodegradable.12 Also, DES enhances the stability of hydrophobic compounds that are unstable in aqueous solutions.13 The high kinematic viscosity (typically 200–500 mm2 s−1 at 40 °C) of DES, however, lead to some practical problems including time-consuming solvent-transfer operations and slow mass transfer during dissolutions or extractions.14 Diluting DES with water has been proved to be an efficient way of decreasing their viscosities and adjusting the solubility of compounds.15
To enhance the efficiency of DES in the extraction of phenolic compounds, DES have been combined with other extraction methods,16–18 such as ultrasound-assisted extraction (UAE),13,19 heating extraction (HE), microwave-assisted extraction (MAE), and stirring.20 All these extraction methods may cause losses or changes in the chemical properties of bioactive molecules due to thermal degradation, ionization, hydrolysis, or oxidation during extraction. PEF extraction is a non-thermal extraction technique that is currently used in the extraction of active ingredients from plants.21 The technique is based on electroporation, which is a technique to increase permeability of small molecules to cells. To be more specific is that putting the cell in a strong electric field causes accumulation of oppositely charged ions on both sides of the membrane, as transmembrane potential exceeds a critical value of approximately 1 V, the repulsion between charge-carrying molecules leads to membrane thickness reduction and permeabilization of small molecules. Moreover, the PEF equipment used in this study allows for large scale operation up to industrial level. PEF combined with DES yields more effective extraction of organic molecules from kapok. As far as we know, application of PEF-DES extraction technique on Chinese herbal medicine has not been reported in preliminary publications. This study found that PEF-DES extraction technique has the advantages of extremely high extraction efficiency, less time-consuming, increased stability and environmental protection, and is currently the most potential extraction technique for plant active components the extraction and separation techniques are not only used in the field of cosmetics, but also in the field of food and medicine and chemical industry.
2. Methods and materials
2.1 Materials and chemicals
During the period of May to September 2019, the newly dropped kapok was collected in Zhaoqing, Guangdong, China; the kapok was dried in a hot air oven at 45 °C for 4–5 days. Then, the dried plant material was grinded into fine powder and filtered by 80-mesh screen (180 μm). All alcohols with purities >99 wt%, namely propylene glycol, glycerin, were purchased from Adamas Reagent (Shanghai, China). Betaine (purity >98 wt%) was purchased from Maya Reagent (Jiaxing Zhejiang, China). Citric acid and malic acid were obtained from Adamas Reagent Co., Ltd (Shanghai, China). High-voltage pulsed electric field extractor (South China University of Technology Independent Research and Development) were utilized in the extraction step. Fertilized chicken embryo (White Leghorn chicken) was purchased from Xinxing Dahuanong Egg Co., Ltd (Guangdong, China). Rutin standard were provided by Aladdin Reagent Co., Ltd (Shanghai, China). High glucose DMEM medium, trypsin was provided by U.S. Gibico Corporation. Fetal bovine serum was provided by Hangzhou Sijiqing Bioengineering Materials Co., Ltd. All other chemicals and solvents were analytical grade or HPLC grade.
2.2 Preparation of the DES
Betaine-based DES samples listed in Table 1 are selected for preparation as betaine itself is a good moisturizing and hydrating raw material widely used in cosmetics. Preparation procedure is carried out according to previous publication22 with slight modifications as different raw materials were used. An ultrasonic-assisted preparation method was applied for sample preparation. Different components of hydrogen bond acceptor and hydrogen bond donor were mixed in 100 mL Erlenmeyer flasks according to the molar ratios shown in Table 1. Afterwards, the Erlenmeyer flasks were put into a SB25-12DTD ultrasonic bath (Ningbo Xinzhi Biotechnology Co., Ltd., Ningbo, China). The ultrasonic input power was changed from 300 W in the literature to 100 W, and the frequency was changed from 40 kHz in the literature to 37 kHz. After 5 hours of sonication, the mixture formed a clear and homogeneous liquid at room temperature. All DES samples were left at ambient temperature for an additional 24 hours after production processes to ensure the formation of a liquid mixture. The conductivity and pH value of DES were measured with a SEVEN EXCELLENCE professional multi-function measuring instrument (No. 589, Guiping Road, Shanghai, China).
Table 1 Chemical information of DESa
DES |
INCI |
Chemical structure |
Molar ratios |
Conductivity (μS cm−1) |
pH value |
INCI (International Nomenclature of Cosmetic Ingredients), indicates the composition of DES. |
DES-TBN |
Propylene glycol, citric acid, betaine |
 |
1 : 1 : 1 |
1553.90 ± 3.92 |
2.82 ± 0.22 |
DES-TPB |
Betaine, propylene glycol, malic acid |
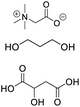 |
1 : 1 : 2 |
938.19 ± 3.49 |
2.88 ± 0.17 |
DES-TGN |
Betaine, propylene glycol, glycerin, citric acid |
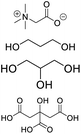 |
1 : 1:3 : 1 |
60.28 ± 2.48 |
2.95 ± 1.21 |
DES-TG |
Betaine, glycerin, propylene glycol |
 |
3 : 1 : 0.5 |
10.85 ± 1.24 |
6.28 ± 0.32 |
DES-TGP |
Betaine, propylene glycol, glycerin, malic acid, glycerin |
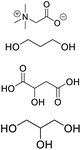 |
1 : 1:2 : 1 |
9.93 ± 0.18 |
2.94 ± 0.19 |
2.3 Determination of total flavonoid content
The determination method of total flavonoid content was referred to the method proposed of Jia et al.23 with modifications. After diluting the kapok flavonoid extract 10 times, 1.4 mL of ethanol (75%, v/v) and 0.4 mL of NaNO2 (5%, w/v) were added into 1 mL of sample diluent, the mixture was allowed to stand at room temperature for 6 min. Then, 0.4 mL of Al(NO3)3 (10%, w/v) was added into this mixture and incubated for 6 min at room temperature. Finally, after adding 4 mL of NaOH (4%, w/v) and 2.8 mL of ethanol (75%, v/v) into this mixture, the absorbance was determined at 510 nm. The standard curve was prepared using various rutin concentrations as the standard. The kapok flavonoid content was calculated with the following equation:
where the C was Concentration of flavonoids in kapok extract diluent, V was the total volume of extraction solution, F was the Dilution times and M was the quality of the kapok.
2.4 Screening of DES
Powders (1.0 g) of kapok and 10 mL aqueous solutions of the deep eutectic solvents (30%) were placed in Erlenmeyer flask, and then extracted with high-voltage pulsed electric field extractor. Then, centrifugation was performed at 4500 rpm for 20 min. The supernatant was separated and determination of the extraction rate of TG-KF with aluminum nitrate color method.
2.5 Optimization of PEF-DES by single factor tests and orthogonal experiment
The preparation process of TG-KF and the principle of PEF are shown in Fig. 3 and 4. The single factor experiments were performed in several combinations, such as using different DES concentration (10%, 20%, 30%, 40%, and 50%), solid–liquid ratios (1
:
10, 1
:
20, 1
:
30, 1
:
40, and 1
:
50), electric field strength (0.14, 0.27, 0.41, 0.55 and 0.68 kV cm−1) and pulse numbers (20, 30, 40, 50, and 60 times) in the high-voltage pulsed electric field extractor. A Box–Behnken design was applied for orthogonal experiment with Design-Expert 8.0.6 Trail software. Based on the above single factor experimental results, the chosen factor boundaries of DES concentration (A), liquid–solid ratio (B), electric field strength (C) and pulse numbers (D) were listed in Table 2. The experiments were performed in random order to avoid systematic error.24
Table 2 ANOVA of the fitted polynomial quadratic modela
Level |
Factors |
DES-TG concentration (%) |
Liquid–solid ratio |
Electric field strength (KV cm−1) |
Pulse numbers (times) |
Source: ANOVA using Design-Expert 8.0.6. |
1 |
30% |
1 : 30 |
0.41 |
60 |
2 |
40% |
1 : 40 |
0.55 |
80 |
3 |
50% |
1 : 50 |
0.68 |
100 |
2.6 Stability of TG-KF
2.6.1 Stability observations of TG-KF. TG-KF was observed at 2 °C, 25 °C, 45 °C, −18 °C, 18–45 °C and light conditions for 7 days. The kapok flavone extracts were tested for color change and odor development on days 7, 14, 30, 60, 90 and 180.
2.6.2 Multiple light scattering experiment. The TG-KF was loaded into the sample cell, the injection height was 30–40 mm, the scanning step of the backscattered light, the transmitted light detector and the near-infrared light source (wavelength of 880 nm) was 40 μm, and the measurement time was 40 μm. 1 time/1 h, a total of 24 h; the stability of TG-KF was analyzed by the change of the scanning pattern of the sample.25
2.7 Safety of TG-KF
2.7.1 Patch test. Thirty-three volunteers were selected, and their age composition and gender were randomly determined, and they met the inclusion and exclusion criteria of the 2015 cream hygiene code; the curved side skin of the forearm was selected as the test site, and the skin was divided into two parts, one was a blank control, and the other was a smear containing 10% kapok flavonoid cream; then the spot tester tape with spot test substance from the bottom was stuck up firmly; the patch test time is 48 h; after patching for 48 h, the patch tester was removed; after 30 minutes, then the test results were recorded. For detailed operation steps, refer to the patch test diagnostic kit instructions. Result judgments see Table 6.
Table 3 L9(34) orthogonal experiment results and range analysisa
Serial number |
A (%) |
B (w/v) |
C (KV cm−1) |
D (times) |
Flavonoid yield (%) |
DES concentration (A), liquid–solid ratio (B), electric field strength (C) and pulse numbers (D). |
1 |
30 |
1 : 30 |
0.41 |
60 |
6.94 ± 0.45 |
1 |
40 |
1 : 40 |
0.41 |
80 |
7.76 ± 0.12 |
1 |
50 |
1 : 50 |
0.41 |
100 |
10.73 ± 1.03 |
2 |
30 |
1 : 40 |
0.55 |
100 |
10.38 ± 0.72 |
2 |
40 |
1 : 50 |
0.55 |
60 |
12.72 ± 0.39 |
2 |
50 |
1 : 30 |
0.55 |
80 |
7.87 ± 1.09 |
3 |
30 |
1 : 50 |
0.68 |
80 |
14.38 ± 0.27 |
3 |
40 |
1 : 30 |
0.68 |
100 |
7.78 ± 0.68 |
3 |
50 |
1 : 40 |
0.68 |
60 |
11.77 ± 0.18 |
K1 |
8.477 |
10.567 |
7.530 |
10.477 |
|
K2 |
10.323 |
9.420 |
9.970 |
10.003 |
|
K3 |
11.310 |
10.123 |
12.610 |
9.630 |
|
R |
2.833 |
1.147 |
5.080 |
0.847 |
|
2.7.2 HET-CAM test for TG-KF. When the chick embryos were hatched to 9 days of age, the eggs were checked. Dental serrated curved forceps were used to peel off the eggshell part of the air chamber to expose the white egg membrane. Care should be taken not to damage the integrity of the egg membrane. 2 mL of 0.9% sodium chloride (NaCl) solution was added dropwise with a pipette to wet the egg membrane. 0.9% sodium chloride solution was used as the negative control, 0.1 mol L−1 sodium hydroxide was used as a positive control, and TG-KF was diluted to 20%, 15%, 10%, and 5% for experiments, and 0.3 mL was added to each sample. The observations were recorded accordingly.For the test using the reaction time method, the stimulus score (IS) was calculated using the formula (1), and the result was kept to two decimal places:
|
 | (1) |
where secH (hemorrhage time)—the average time to observe the onset of bleeding on the CAM membrane, in seconds (s). secL (vessel lysis time)—the average time to observe the onset of vascular ablation on the CAM membrane, in seconds (s). secC (coagulation time)—the average time to observe the onset of coagulation on the CAM membrane, in seconds (s).
According to the calculated IS value, the eye irritation of the test object is classified according to Table 8.
2.8 DPPH assay
Free radical scavenging experiment was performed using DPPH assay. The DPPH radical scavenging capacity of each extract was determined according to the method of Dudonn.26,27 DPPH radical has an absorption maximum at 515 nm, which disappears with reduction by an anti-oxidative compound. The DPPH˙ solution in methanol (6 × 10−5 M) was prepared daily, and 3 mL of this solution was mixed with 100 μL of methanolic solutions of plant extracts. The samples were incubated for 20 min at 37 °C in a water bath, and then the decrease in absorbance at 515 nm was measured (AE). A blank sample containing 100 μL of methanol in the DPPH˙ solution was prepared daily, and its absorbance was measured (AB). The experiment was carried out in triplicate. Radical scavenging activity was calculated using the following formula:
Inhibition(%) = [(AB – AE)/AB] × 100 |
where:
AB = absorbance of the blank sample, and AE= absorbance of the plant extract.
2.9 Statistics analysis
All data are represented as mean ± standard deviation unless otherwise indicated. To compare differences between groups, one-way ANOVA was used to compare the three groups of physiological parameters.
3. Results and discussion
3.1 Single factor experiment
Using DES as the reagent, the flavonoids in kapok were extracted by the PEF. Furthermore, the potential parameters that affect the extraction efficiency, including DES type, DES concentration, solid–liquid ratio, electric field strength and PEF number of pulses, were evaluated in detail.
3.1.1 Effect of DES types. The structure of DES has significant influence on extraction of flavonoids from natural products,28 which might affect the extraction yields of flavonoids. In addition, it was reported that the factors influencing the extraction ability are very complicated, such as hydrogen bonding effect, electrostatic effect, and van der Waals force.29,30 Five DES solutions were chosen in current study to demonstrate how structures of DES affect extraction yields. To avoid the addition of organic solvents, water was used to dilute the DES. As shown in Fig. 1a, the extraction yield of flavonoids from DES-TG is significantly higher than other 4 DES water solutions (specific value). As shown in Table 1, there is higher content of phenolic hydroxyl groups, and no citric acid or malic acid, the pH value of DES-TG is 6.28 ± 0.32. Meanwhile, the pH values of other 4 DES are all around 2.9. Since kapok flavonoids are relatively stable and have the best solubility at pH = 6–8 (see Fig. 2), and lower under strong acid and strong alkali conditions, appropriate component and pH value of DES-TG may contribute to increase of flavonoids stability and solubility, which resulted in the higher extraction yield. Based on the above experimental results, DES-TG was selected as the further single factor study.
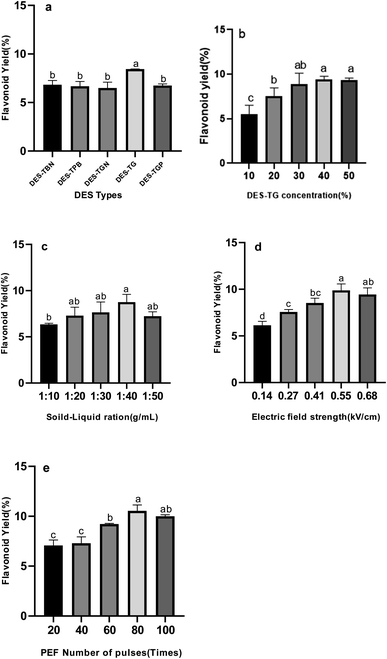 |
| Fig. 1 Effects of extraction parameters on kapok flavonoid yield. (a) DES types; (b) DES concentration; (c) solid–liquid ration; (d) microwave power; (e) extraction time. The bars show the mean values from three replicates with the respective standard deviations. Different letters on the tops of the bars denote significant differences (p < 0.05). | |
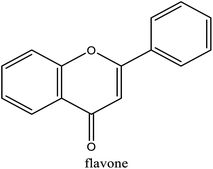 |
| Fig. 2 Structure of flavonoids. | |
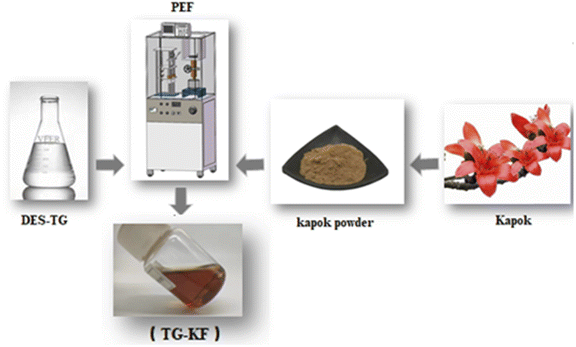 |
| Fig. 3 Extraction process of TG-KF. Dried kapok were pulverized and mixed with 50% DES-TG in a ratio of 1 : 30, the mixture was extracted by using PEF equipment according to the instrument manual, the extracts were centrifuged and the supernatant were collected as TG-KF. | |
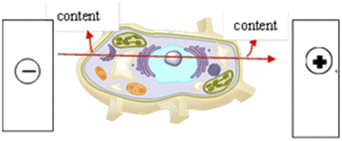 |
| Fig. 4 Principle of PEF breaking cells. The high-voltage pulsed electric field extraction technology generates a high-intensity electric field through the high potential difference between the electrodes. Under the alternating action of the electric field and the magnetic field, the cell membrane permeability of the treated material increases and the strength weakens, and pores are formed on the membrane. The membrane ruptures, causing the cytoplasm in the cell membrane to flow out to achieve the purpose of increasing the content of certain components in the material. Effective processing time is generally in the mini-seconds level. | |
3.1.2 Effect of DES-TG concentration. DES-TG concentration can be considered as the primary factor for extracting active ingredients of natural products.31 The effects of different DES-TG concentrations (10%, 20%, 30%, 40%, and 50%) on total flavonoids amount of the extracts were compared, and the results were shown in Fig. 1b. As DES-TG concentrations increased from 10% to 20%, the yield of flavonoids changed from 5.5% to 9.4%. However, when DES-TG concentration was at 40–50%, the product yield had no obvious change. As previous section mentioned, multiple intermolecular forces including dipole–dipole interactions, hydrogen bonding and pi–cation interactions are present between flavonoids and DES-TG. At lower concentrations, increasing the ratio of DES-TG can enhance intermolecular interactions between flavonoids and DES-TG so that the extraction efficacy can be improved. Whereas as the concentration of DES-TG reaches to a certain level, the intermolecular interactions between flavonoids and DES-TG will be saturated, which indicates that having more DES-TG in the solution will not improve the extraction rate.
3.1.3 Effect of solid–liquid ratio. The ratio of raw plant material to solvent can be a key determinant of extraction efficiency. Specifically, higher solvent volumes generally result in better extraction efficiency, but excessively large volumes can end up with complex extractions and substantial unnecessary waste. We observed significant increases in flavonoids extraction rates when the solid–liquid ratios were in the range from 1
:
10 to 1
:
30 (see Fig. 1c), potentially suggesting that at ratios below 1
:
40, the viscosity of the extract was too high, and thereby preventing efficient flavonoids extraction. Decreasing the solid–liquid ratio can improve flavonoids extraction such that peak extraction was achieved at a ratio of 1
:
40. As such, subsequent single factor experiments were conducted using this 1
:
40 solid–liquid ratio.
3.1.4 Effect of PEF electric field strength. The mechanism of the high-voltage pulse extraction technique is to use the principle of cell membrane electroporation to instantly break the cell wall under an external high-voltage pulse electric field, causing confusion in the cell membrane potential, and reversible or irreversible damage to the cell wall and cell membrane, thereby leading to outflow of intracellular components.32 With the increase of the applied electric field intensity, the yield of TG-KF increases (see Fig. 1d), but when the electric field intensity is greater than 0.55 kV cm−1, the yield of TG-KF begins to decrease, which may be that part of the flavonoids are destroyed due to strong electric field. Therefore, it is determined that the best pulsed electric field intensity is 0.55 kV cm−1.
3.1.5 Effect of number of pulses. The extraction of active ingredients from natural products requires a long time and prolonged extraction time produces a lot of impurities. Hence, it is necessary to determine the appropriate number of pulses, since the number of pulses determines the overall time of extraction. In this study, the effect of different number of pulses (20, 40, 60, 80 and 100 times) on the extraction rates of TG-KF is investigated. As illustrated in Fig. 1e, when the number of pulses is in the range of 20–80 times, the extraction rate of the flavonoids gradually increases as number of pulses rises, and the extraction rate of flavonoids reaches a maximum value at 80 times. However, the extraction rate shows a downward trend at 80–100 times. Our interpretation on such phenomena is that shorter extraction time will lead to incomplete extraction of the target product in the range of 20–80 times and, as the pulse number exceeds 80 times, the excess number of pulses will degrades the flavonoids and increase the energy consumption and cause wastage.33 Thus, 80 times of number of pulses are selected for further optimization.
3.2 Results of orthogonal test
The experimental data obtained from orthogonal design are shown in Table 3. K1, K2 and K3 was the average total flavonoid yield under the various investigated conditions, and the maximum value was the optimal value. In addition, according to the largest donating rule, the factor with the largest range value (Kmax–Kmin) has the greatest effect on the extraction of flavonoids. Table 4 shows that the rank order of the four influential factors is RC > RA > RB > RD.34
Table 4 Analysis of variance of the four studied microwave-assisted extraction parametersab
Source |
Sum of squares |
Degree of freedom |
F-ratio |
F0.05 |
Significance |
DES-TG concentration (A), liquid–solid ratio (B), electric field strength (C) and pulse numbers (D). Source: ANOVA using Design-Expert 8.0.6. |
A |
12.411 |
2 |
0.915 |
4.460 |
|
B |
2.006 |
2 |
0.148 |
4.460 |
|
C |
38.730 |
2 |
2.857 |
4.460 |
|
D |
1.080 |
2 |
0.080 |
4.460 |
|
Error |
54.23 |
8 |
|
|
|
To evaluate which factors had the greatest influence on flavonoid extraction yield, variance analyses (ANOVA) were applied to assess the results. The result (Table 4) indicates that DES concentration (factor C) has the greatest impact, and that solid–liquid ratio (factor D) has the least influence. The ANOVA result is in good agreement with data showed in Table 5. The maximal yields are obtained when the DES concentration is 50%; solid to liquid ratio is 1
:
30; the electric field strength is 0.55 kV cm; and the number of pulses is 100 times (A3B1C3D1, with the letter denoting the factor and number denoting the level). To redefine the optimum conditions, the extraction experiments were performed under this condition (A3B1C3D1), and the extraction yield of flavonoids reaches 14.38 ± 0.27. Overall, this deduced condition is rationally confirmed to be the best combination of different parameters.
Table 5 Stability of TG-KF under different conditionsa
Concentration |
Conditions |
Time (d) |
0 |
7 |
14 |
30 |
60 |
90 |
180 |
Note: “—” means that TG-KF has no precipitation, turbidity, discoloration, etc. |
Kapok flavonoid extract |
2 °C |
Brownish yellow, transparent and clear |
— |
— |
— |
— |
— |
— |
25 °C |
— |
— |
— |
— |
— |
— |
45 °C |
— |
— |
— |
— |
— |
— |
−18 °C |
— |
— |
— |
— |
— |
— |
Illumination |
— |
— |
— |
— |
— |
— |
−18 °C to 45 °C 7 days alternate |
— |
— |
— |
— |
— |
— |
Table 6 Skin adverse reaction grading standard
Grade |
Response level |
Skin reaction |
0 |
Negative reaction |
No erythema, edema, blisters, etc. |
1 |
Suspicious reaction |
Only weak erythema |
2 |
Weak positive reaction |
Erythema, infiltration, edema, and papules |
3 |
Strong positive reaction |
Erythema, infiltration, edema, papules, herpes; reaction may exceed the test area |
4 |
Very strong positive reaction |
Obvious erythema, severe infiltration, edema, fusion herpes; reaction beyond the test area |
3.3 Stability of TG-KF
3.3.1 Stability observation of TG-KF. The kapok flavonoid solution extracted by PEF-DES technique has high stability under different temperature and light conditions (2 °C, 25 °C, 45 °C, −18 °C, illumination and −18 °C to 45 °C 7 days alternate) (see Table 5). After 6 months of observation, no precipitation or discoloration is found, which may result from the presence of ions. The DES-TG and TG-KF have a similar structure. According to the principle of similar compatibility, the TG-KF and DES form a strong force.
3.3.2 Stability analysis of TG-KF. Referring to the national standard for multiple light scattering measurement methods: GB/T38431-2019 Tatic Multiple Light Scattering Method for Stability Evaluation of Particle Dispersion Systems and internal laboratory standards, as shown in Fig. 7, TG-KF meets the absolute stability standard of 45 °C. The stability of TG-KF can be indicated under the conditions, that is, the middle backscattered light/transmitted light ΔBS < 0.2%, and the bottom and top backscattered light/transmitted light ΔBS < 5% (Fig. 6).
3.4 Safety of TG-KF
3.4.1 Patch test. The test parts of the forearm of 33 subjects were observed for 48 hours, and the results are shown in Table 7. It can be seen from Table 7 that in the human patch test experiment, there were no adverse phenomena in the 33 subjects. Therefore, TG-KF is not irritating to human skin (see Table 7).35
Table 7 Summary of human skin patch test results
Group |
Number of participants |
Observation time |
Number of different skin reactions in patch test |
0 |
1 |
2 |
3 |
4 |
Sample |
33 |
0.5 h |
33 |
0 |
0 |
0 |
0 |
24 h |
33 |
0 |
0 |
0 |
0 |
48 h |
33 |
0 |
0 |
0 |
0 |
Control |
33 |
0.5 h |
33 |
0 |
0 |
0 |
0 |
24 h |
33 |
0 |
0 |
0 |
0 |
48 h |
33 |
0 |
0 |
0 |
0 |
Table 8 Stimulus scoring method result evaluationa
Stimulus score |
Irritant classification |
IS: irritation score. |
IS < 1 |
Non-irritating |
1 ≤ IS < 5 |
Mild irritation |
5 ≤ IS < 9 |
Moderately irritating |
IS ≥ 10 |
Strong irritant |
3.4.2 HET-CAM test for TG-KF. To simulate the skin irritation of TG-KF as closely as possible, we used the HET-CAM model. The HET-CAM model is a suitable alternative for skin compatibility assays, as an alternative to animal experiments, and as an additional necessary experiment to analyze the toxicity of our formulations. After injection of TG-KF solution the membrane, the irritation score of the formulation was calculated. IS includes three effects that can be observed after application of the test substance on the CAM: hyperemia, hemorrhage, and coagulation. For 10% TG-KF, there was no measurable stimulation potential during incubation on CAM. The observed parameters indicated that no disintegration of blood vessels, hemorrhage and clot formation were detected 5 minutes after administration. For 15% TG-KF, mild irritation was measurable during incubation on CAM with the IS of 2.01 (Fig. 7 and Table 9).
Table 9 Results of the HET-CAM assaya
Sample |
IS score |
IS: irritation score. |
0.1 mol L−1 NaOH |
18.97 |
0.9% NaCl |
0 |
15% TG-KF |
2.01 |
10% TG-KF |
0 |
3.5 DPPH radical scavenging capacity
Radical scavenging capacities of TG-KF were determined using DPPH assays. Results are shown in Fig. 5. The radical scavenging rate was impacted by the concentration of antioxidants that was directly correlated to the extraction effectivity of solvents. The scavenging rate of DPPH and half inhibitory concentration of DPPH free radicals (IC50) were used to evaluate the extraction effect of TG-KF. Overall, the clearance of DPPH was positively correlated to the concentration of TG-KF. TG-KF has very good antioxidant capacity and its IC50 is 1.24%.
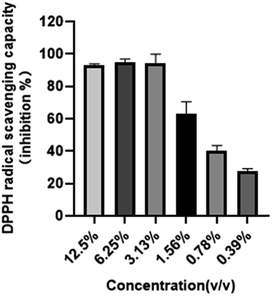 |
| Fig. 5 DPPH free radical scavenging rate of TG-KF. In vitro antioxidant capability of serial diluted TG-KF was tested by DPPH free radical scavenging assay. Data is expressed as mean SD, n = 3. | |
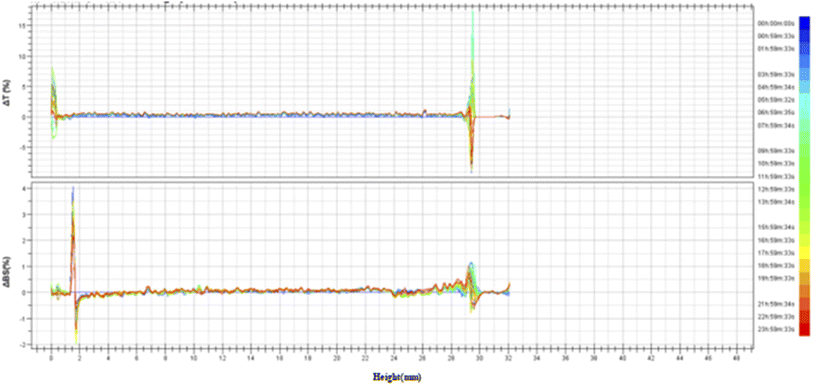 |
| Fig. 6 Transmitted light/backscattered light reference spectrum of TG-KF. The stability of TG-KF was analyzed by multiple light scattering instrument according to the national standard methods (GB/T38431-2019). The multiple light scattering instrument uses near-infrared light as the light source, and has a transmitted light detector and a backscattered light detector. ΔT: change value of transmitted light; ΔBS: change value of backscattered light. | |
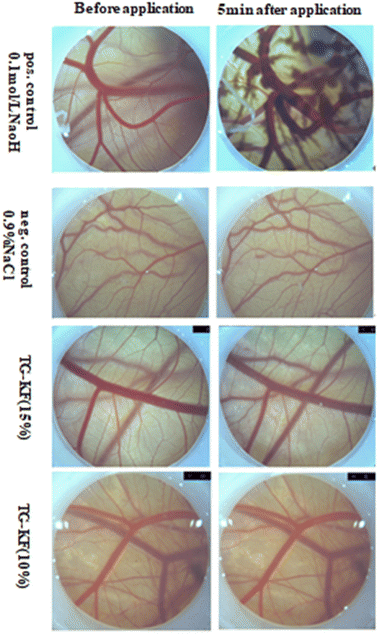 |
| Fig. 7 Results of the HET-CAM assay. The micrographs show the chorioallantoic membrane before and 5 minutes after treatment with 0.1 mol L−1 NaOH (positive control), 0.9% NaCl (negative control) and TG-KF (10% and 15%). | |
4. Conclusions
At present, the commonly used extraction methods of flavonoids include organic solvent extraction, ultrasonic extraction, ionic liquid extraction and so on. However, these extraction techniques have some disadvantages (Supplementary Table 1†), such as: the disadvantages of organic solvent extraction are high cost and not environmentally friendly, the disadvantage of ultrasonic extraction is that industrial production cannot be carried out, and the disadvantage of ionic liquid extraction is that it is difficult to purify. To improve the extraction efficiency, this paper uses the PEF-DES extraction technology to examine this factor and the factors that influence the PEF-DES extraction technique were examined and optimized for the extraction of flavonoids in kapok. Validation experiments confirm that the PEF-DES extraction method developed from this study is reliable and efficient for the extraction of flavonoid from kapok. Accordingly, the method based on DES with PEF provides a possible alternative for the green extraction of flavonoids in plant materials, particularly for those used in cosmetic applications. In addition, TG-KF does not require additional product separation steps, and the resulting aqueous solution containing DES extract can be safely applied in cosmetics and other products.
Funding
The authors are grateful for the financial support from the Marubi Joint Research Project Fund (YB202109131043), and Guangzhou Science and Technology Plan Project Fund (202103000055).
Author contributions
Rui-jing Wei: responsible for the design, experiment, and draft of the manuscript; Lu Hu: analysis summary and discussion; Li-hua Wang & Peng Yan: assisted testing; Ning Wang & Tao Lin: assist with manuscript revision; Huai-qing Sun & Bi-sheng Zheng: supervision; Chao-wan Guo: organized, supervised, and guided the whole work.
Conflicts of interest
The authors declare that they have no known competing financial interests or personal relationships that could appear to influence the work reported in this paper.
Abbreviations
PEF | High-voltage pulse |
DES | Deep eutectic solvents |
PEF-DES | High-voltage pulse-assisted deep eutectic solvent |
TG-KF | Kapok flavonoid solution extracted with DES-TG |
ANOVA | Analysis of variance |
HET-CAM | Chicken embryo allantoic chorionic test |
IS | Irritation score |
Acknowledgements
We thank Dr Nan JIA from for advisements and English editing of this manuscript. We are also very grateful for the technical support provided by Shenzhen Xuanjia Biotechnology Co., Ltd. in the preparation of DES.
References
- B. Nageen, I. Sarfraz and A. Rasul, et al., J. Asian Nat. Prod. Res., 2020, 22, 1–16 CrossRef CAS PubMed.
- J. H. Xu, Z. Q. Huang and L. C. Chun, et al., J. Fujian Med. Univ., 1993, 27, 110–112 Search PubMed.
- R. Chomchan, P. Puttarak and A. Brantner, et al., Antioxidants, 2018, 7, 57 CrossRef PubMed.
- C. Montenegro, G. Gonçalves and A. Oliveira Filho, et al., Molecules, 2017, 22, 869–887 CrossRef PubMed.
- G. K. Wang, B. B. Lin and R. Rao, et al., Nat. Prod. Res., 2013, 27, 1348–1352 CrossRef CAS PubMed.
- W. Poomanee, W. Chaiyana and M. Mueller, et al., Anaerobe, 2018, 52, 64–74 CrossRef CAS PubMed.
- L. Xusen, X. Guangkai and W. Guokai, et al., Chin. Wild Plant Resour., 2015, 34, 42–48 Search PubMed.
- W. Xu, C. Kedan and L. Huang, et al., Molecules, 2012, 17, 14323–14335 CrossRef CAS PubMed.
- Q. J. Lin, Study on the flavonoids extraction process and biological activity of Kapok Flower, Fujian Agriculture and Forestry University, 2015 Search PubMed.
- X. Li and K. H. Row, J. Sep. Sci., 2016, 06, 1–46 CAS.
- L. Wang, X. Fang and Y. Hu, et al., RSC Adv., 2021, 5, 17924–17935 RSC.
- B. K. Tang, H. Zhang and K. H. Row, J. Sep. Sci., 2015, 38, 1053–1064 CrossRef CAS PubMed.
- A. R. Mansura, N. Song and H. W. Jang, et al., Food Chem., 2019, 293, 438–445 CrossRef PubMed.
- Y. Dai, J. van spronsen and G. witkamp, et al., Anal. Chim. Acta, 2013, 766, 61–68 CrossRef CAS PubMed.
- Y. Dai, G. Witkamp and R. Verpoorte, et al., Food Chem., 2015, 123, 1–28 Search PubMed.
- M. I. Leong, M. R. Fuh and S. D. Huang, J. Chromatogr. A, 2014, 1335, 2–14 CrossRef CAS PubMed.
- A. Spietelun, A. Marcinkowski and M. de la Guardia, et al., Talanta, 2014, 119, 34–45 CrossRef CAS PubMed.
- Y. Chen, Y. Ao and W. Ding, RSC Adv., 2019, 9, 38669–38677 RSC.
- Y. Li, Z. Pan and B. Wang, et al., New J. Chem., 2020, 08, 1–8 Search PubMed.
- S. C. Cunha and A. J. Fernandes, Anal. Chem., 2018, 001, 1–39 Search PubMed.
- O. Parniakov, F. J. Barba and N. Grimi, et al., Food Chem., 2016, 192, 842–848 CrossRef CAS PubMed.
- Y. Hsieh, Y. Li and Z. Pan, et al., Ultrason. Sonochem., 2019, 10, 110–112 Search PubMed.
- F. J. Barba, C. M. Galanakis and M. J. Esteve, et al., J. Food Eng., 2015, 001, 1–7 Search PubMed.
- C. D. Jian, S. Qing and X. B. Quan, et al., Biomed. Environ. Sci., 2011, 24, 688–693 Search PubMed.
- M. Ramezani, G. Ferrentino and K. Morozova, et al., Foods, 2021, 10, 1–11 CrossRef PubMed.
- S. Dudonn, X. Vitrac and P. Couti, et al., J. Agric. Food Chem., 2009, 57, 1768–1774 CrossRef PubMed.
- Z. Liu, L. Kong and S. Lu, et al., J. Anal. Methods Chem., 2019, 48, 1–13 Search PubMed.
- A. P. Abbott, G. Capper and D. L. Davies, et al., Chem. Commun., 2001, b2, 2010–2011 RSC.
- M. A. F. Cláudio and L. Swift, et al., Phys. Chem. Chem. Phys., 2014, 16, 1–8 RSC.
- D. E. Yoo, K. M. Jeong and S. Y. Han, et al., Food Chem., 2018, 02, 1–43 Search PubMed.
- H. Ullah, C. D. Wilfred and M. S. Shaharun, J. King Saud. Univ., Sci., 2017, 014, 1–10 Search PubMed.
- L. Wang, M. S. Wang and X. Zeng, et al., J. Agric. Food Chem., 2016, 32, 1–38 Search PubMed.
- X. Liu, Y. Niu and J. Liu, et al., Molecules, 2019, 2942, 1–13 Search PubMed.
- C. Guo, W. Jin and H. Yong, et al., Pharmacogn. Mag., 2013, 09, 192–195 CrossRef CAS PubMed.
- C. I. Wootton, M. Soukavong and S. Kidoikhammouan, et al., PLoS One, 2020, 15, 1–6 Search PubMed.
|
This journal is © The Royal Society of Chemistry 2022 |
Click here to see how this site uses Cookies. View our privacy policy here.