DOI:
10.1039/D2RA03614B
(Paper)
RSC Adv., 2022,
12, 20122-20137
Investigation of the anticorrosion and adsorption properties of two polymer compounds on the corrosion of SABIC iron in 1 M HCl solution by practical and computational approaches
Received
11th June 2022
, Accepted 27th June 2022
First published on 12th July 2022
Abstract
The anticorrosion efficiency of two polymer compounds, namely polystyrene (PS), polybutylene terephthalate (PBT), against the corrosion of SABIC iron (S-Fe) in 1.0 M HCl solution was investigated. The anticorrosion efficiency was estimated by chemical and electrochemical measurements. The anticorrosion efficiency increased with the increase in the concentration of the polymer compounds and reduction in temperature. All the obtained corrosion data confirmed the anticorrosion strength in the presence of PS and PBT compounds, such as the decreasing values of the corrosion current density, capacity of the double layer, and weight reduction, while the values of the charge-transfer resistance increased. Also, the pitting potential values moved in the noble (+) direction. The anticorrosion efficiency of the PBT compound was higher than that of the PS compound, which was 95.98% at 500 ppm concentration for PBT while for PS it was 93.34% according to polarization measurements. The anticorrosion activity occurred by the adsorption of PS and PBT compounds on the surface of S-Fe according to the Langmuir isotherm. The polarization curves indicated that the PS and PBT compounds were mixed-type inhibitors. Density functional theory (DFT) and Monte Carlo simulation (MC) were performed for the two polymer compounds. The computational quantum functions were found to be in agreement with the experimental results.
1. Introduction
SABIC iron (S-Fe) is considered one of the most important metals applied in industry, especially in the Kingdom of Saudi Arabia, where it is used in many vital industries, especially in construction.1,2 HCl solutions are applied for pickling, cleaning, and chemical and electrochemical etching of S-Fe. However, one of the disadvantages of using this acid is that it corrodes S-Fe. In order to diminish the risk of corrosion, scientists have turned to the use of corrosion inhibitors to diminish the rate of corrosion of S-Fe in acidic solution. Generally, organic compounds, especially those containing some hetero atoms in their structure are most commonly utilized as corrosion inhibitors for iron and steels in acidic media.3–20 The inhibition efficiency of these compounds relies on the concentration of the acidic solution, the nature of the metal or alloy used, the chemical composition of the additives and the capability to adsorb on the metal surface.
There are few studies on the inhibition of S-Fe corrosion in acidic solutions, because its use is concentrated in the Arabian Gulf region. Fawzy et al.21 used synthesized sodium N-dodecyl arginine compound as an efficient inhibitor for the corrosion of SABIC iron in acidic, alkaline, and neutral solutions. In previous studies expired antibacterial drugs,22 expired acyclovir and omeprazole drugs,23 and expired amoxicillin and cefuroxime drugs24 have been used as efficacious inhibitors for SABIC iron corrosion in 1.0 M hydrochloric acid solution. The high inhibition efficiency of these expired drugs was due to their strong adsorption on the S-Fe surface. A literature survey revealed that some polymer compounds have been applied to inhibit the corrosion of iron and steels in acidic solutions.25–32 For instance, polystyrene (PS) and polybutylene terephthalate (PBT) were previously used to inhibit the corrosion of steel in acidic solutions.33,34 The inhibition effect of these compounds depends on the chemical structure of the polymer and the presence of several active centers that enhance the adsorption process.
This study tested the use of two polymer compounds, namely polystyrene (PS) and polybutylene terephthalate (PBT), to retard the corrosion of S-Fe in 1 M HCl solution. The anticorrosive efficiency of PS and PBT compounds were measured by weight reduction (WR), galvanostatic polarization (GAP), potentiodynamic anodic polarization (PDAP), and electrochemical impedance spectroscopic (EIS) measurements. The thermodynamic functions for the activation and adsorption process were studied. Density functional theory (DFT) approaches were used to predict the corrosion inhibition performance. Theoretical quantum chemical calculations and Monte Carlo simulations were carried out on the studied polymers to correlate with the experimental findings.
2. Experimental
2.1. Materials
All the chemicals used, such as HCl, NaCl, and the tested two polymer compounds, namely PS and PBT, were supplied from Merck or Sigma-Aldrich. Double distilled water was used to prepare the solutions in all the investigations.
2.2. Technologies
SABIC iron (S-Fe) used in various technologies is produced by the Saudi Arabia Basic Industry Company (SABIC) with a purity of 99.99%. For weight reduction (WR), coupons with dimensions of 1 × 3.8 × 0.2 cm3 were used. The WR of S-Fe in mg was determined after an immersion time of 12 h in free 1 M HCl solutions and as well as in the presence of different concentrations of PS and PBT compounds. For the galvanostatic polarization (GAP), potentiodynamic anodic polarization (PDAP), and electrochemical impedance spectroscopic (EIS), cylindrical or galvanic polarization (GAP), potentiodynamic anodic polarization (PDAP), and electrochemical impedance spectroscopy (EIS) tests, a cylindrical S-Fe rod dipped in Araldites with an uncovered area of 0.38 cm2 was utilized. Prior to all experiments, the coupons or S-Fe rods were sanded with some grades of sand papers ranging from 300 to 1500 grades, after which they were cleaned with distilled water and acetone and finally dried by filter papers.
WR assays were done as formerly reported.34,35 GAP and PDAP technologies were performed utilizing a PGSTAT30 potentiostat/galvanostat in a conventional three-electrode cell with a platinum counter electrode (Pt), reference calomel electrode (RCE), and working electrode (S-Fe). In the electrochemical experiments, the electrode was immersed in the test solution until the steady state potential was attained after almost 40 min, and then the polarization started. The potential range for the PDP experiments was −1200 to +200 mV. For the GAP and PDAP tests, the scan rate was adjusted at 2.0 and 0.5 mV s−1, respectively. All the analyses were done at a constant temperature of 303 K in a temperature-controlled system. EIS was done in a frequency range of 100 kHz to 0.1 Hz with an amplitude of 4.0 mV from a peak-to-peak exploitation of AC signals in OCP.
2.3. Anticorrosive compounds
In this study, we used two polymer compounds, namely PS and PBT, as anticorrosive compounds, and these were purchased from Sigma Aldrich. The average molecular weights of PS and PBT were 35
000 and 90
000 g mol−1. The name, chemical structures, and IUPAC names are recorded in Table 1.
Table 1 Chemical structure and IUPAC names of the anticorrosion compounds
IUPAC names |
Chemical structure |
Name |
Poly(1-phenylethene) |
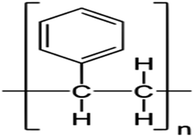 |
Polystyrene (PS) |
Poly(oxy-1,4-butanediyloxycarbonyl-1,4-phenylenecarbonyl) |
 |
Polybutylene terephthalate (PBT) |
2.4. Computational details
In this study, the two polymers were used as corrosion inhibitors for SABIC Fe. To conduct this computational study, the optimized structure geometries of both the dimer and the trimer of butylene terephthalate (D-BT and T-BT) and styrene (D-S and T-S) were found by density functional theory (DFT). The calculations were made using the B3LYP/6-31g(d,p) level of theory in aqueous media using a conductor-like polarizable continuum (CPCM) model.36 The energy of the highest occupied molecular orbital (EHOMO), energy of the lowest unoccupied molecular orbital (ELUMO), energy gap (ΔE), global hardness (η = ΔE/2), softness (σ = 1/η), back-donation (ΔEb-d), and dipole moment (μ) were all measured. The calculations were carried out using the Gaussian 09 code.37
2.5. Monte Carlo (MC) simulation
We carried out the MC simulation to study the interaction of the dimer polymer with the Fe (110) surface. The goal of this work was to determine the preferential adsorption of the dimer polymer on the iron surface. The simulation protocol was carried out on the Fe (110) plane with a five-layer thickness using the periodic boundary conditions. The Fe (110) plane was magnified to 15 × 15 supercells to provide a large surface area for interaction of the dimer inhibitor with the Fe (110) surface and a 25 Å vacuum was applied over the surface. The condensed-phase optimized molecular potentials for atomistic simulation studies (COMPASS) force field was utilized to search for the equilibrium configuration. The Ewald method was used to illustrate the electrostatic interactions with an accuracy of 1 × 10−5 kcal mol−1.38 The adsorption of the dimer inhibitor over Fe (110) was calculated by the following equation: |
Eads = EFe+dimer − Edimer − EFe(110)
| (1) |
where EFe+dimer, Edimer, and EFe(110) are the energies of the dimer on Fe, energy of the dimer, and energy of the Fe (110), surface respectively.
3. Results and discussion
3.1. GAP tests
GAP curves of S-Fe in 1.0 M HCl solutions alone and in the presence of different concentrations of PS and PBT compounds are presented in Fig. 1A and B, respectively. Some corrosion functions, such as anodic (βa), cathodic (βc), and corrosion current density (Icorr.), which were determined from the intersection of the anodic and cathodic Tafel lines with the corrosion potential (Ecorr.) and anticorrosion efficiency (%AE) were computed and are recorded in Table 2.
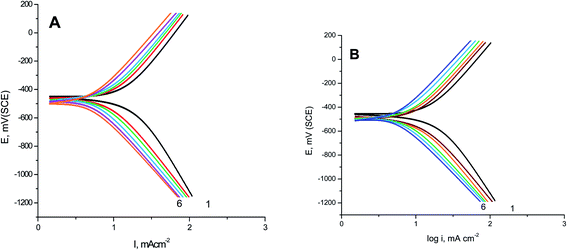 |
| Fig. 1 GAP curves for S-Fe corrosion in the free 1.0 M HCl solution and when containing different concentrations of (A) PS and (B) PBT at 300 K: (1) 0.0, (2) 100, (3) 200, (4) 300, (5) 400, (6) 500 mg l−1. | |
Table 2 Corrosion parameters obtained from the GAP measurements
Inh. |
Inh. conc. (mg l−1) |
−Ecorr. mV (SCE) |
βa (mV per decade) |
−βc (mV per decade) |
Icorr. (mA cm−2) |
%AEGAP |
— |
0 |
475 |
132 |
180 |
8.960 |
— |
PS |
100 |
480 |
135 |
185 |
4.122 |
53.99 |
200 |
482 |
140 |
189 |
2.951 |
67.06 |
300 |
486 |
144 |
192 |
1.704 |
80.98 |
400 |
488 |
148 |
196 |
0.851 |
90.50 |
500 |
490 |
152 |
202 |
0.597 |
93.34 |
PBT |
100 |
484 |
134 |
184 |
3.659 |
59.16 |
200 |
487 |
142 |
188 |
2.329 |
74.01 |
300 |
492 |
146 |
194 |
1.521 |
83.02 |
400 |
495 |
150 |
200 |
0.805 |
91.02 |
500 |
498 |
154 |
206 |
0.449 |
95.98 |
The percentage anticorrosion efficiency (%AEGAP) was calculated from the values of Icorr. using the following equation:
|
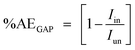 | (2) |
where
Iin and
Iun are the corrosion current densities in the blank 1.0 M Cl solutions and in the presence of different concentrations of the two polymer compounds (PS and PBT) ranging from 100 to 500 ppm.
It is evident that increasing the doses of polymer compounds in 1.0 M HCl solution hindered the anodic dissolution of iron and cathodic H2 evolution. In the beginning of the GAP curves, there were simultaneous anodic and cathodic curves. After this region, the potential increased with a positive trend (anodic polarization) and decreased with a negative trend (cathodic polarization). From Table 2, we note that the βa and βc were nearly constant, whereby the variation in βa was about 20 mV per decade and the variation in βc was about 22 mV per decade. These outcomes demonstrate the anticorrosive activity of the two tested polymer compounds occurred through adsorption on the surface of S-Fe according to a blocking adsorption mechanism. The adsorbed PS and PBT reduced the surface area available for anodic iron dissolution and cathodic H2 evolution reaction without altering the reaction mechanism. The constancy of the Tafel slopes demonstrated that the two polymer compounds (PS and PBT) could be classified as mixed inhibitors. Also, the Ecorr. values were nearly constant. The variations in the Ecorr. values were about 15 and 14 mV in the case of the PS and PBT compounds, respectively. These outcomes verified that the PS and PBT compounds were mixed inhibitors. Obviously, the Icorr. values decreased significantly, while %AEGAP was elevated at both PS and PBT concentrations. The maximum %AEGAP values were 93.34% and 95.98% at 500 ppm of PS and PBT compounds. It is clear from Table 2 that the %AEGAP for PBT in all the concentrations utilized was more than that of PS. This behavior is explained later in the mechanism of anticorrosive section.
3.2. EIS tests
The obtained results from the EIS tests can be stated in terms of the equivalent circuit (Fig. 2), which was applied in the previous embodiment of the SABIC Fe/acid interface.24 Fig. 3A and B present Nyquist plots for the investigated steel in 1 M HCl with and without different doses of PS and PBT compounds. It is clear from these plots that the semicircle in the presence of PS and PBT is higher than that in the free acid solution which actually providing a protective behavior of the investigated compounds.39 This behavior is illustrated by the increased values of Rct (Table 3), which could be due to the observed decrease in the Cdl values, especially at higher concentrations of additives used. This reveals the adsorption of the additive molecules on the surface of the corrosive CS, instead of the initially adsorbed water molecules.40 Also, the single semicircular capacitive ring observed in the free solution indicated a single charge-transfer process controlled the S-Fe corrosion process in this medium.31 The irregularity of the surface formed at the interface of the S-Fe solution was due to the heterogeneity and coarseness, which led to a decrease in the shape of the semicircle. The %AEEIS values were computed according to the following equation: |
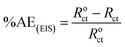 | (3) |
where Roct and Rct are the charge-transfer resistance in the blank (1 M HCl) solution and in the presence of PS and PBT compounds, respectively. Whereas the double layer capacitance Cdl is given by the following equation:where W is the angular frequency, Y0 and n are, respectively, the values of the CPE admittance and exponent. |
 | (5) |
where d is the thickness of the adsorbed film, εo is the permittivity of air of the medium, ε is a dielectric constant, and S is the surface area.
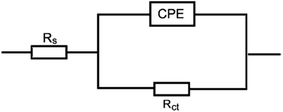 |
| Fig. 2 Electrochemical equivalent circuit applied to fit the EIS measurements. | |
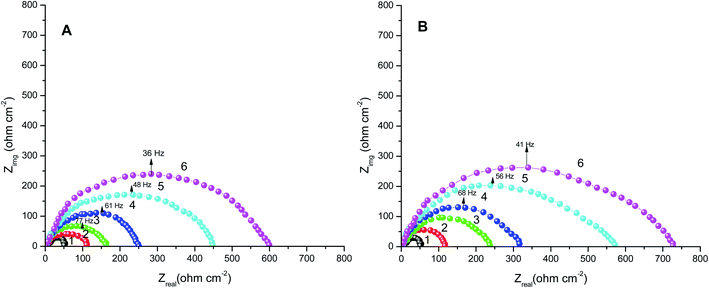 |
| Fig. 3 Nyquist plots for S-Fe corrosion in the blank 1.0 M HCl solution and when containing different concentrations of (A) PS and (B) PBT at 300 K: (1) 0.0, (2) 100, (3) 200, (4) 300, (5) 400, (6) 500 mg l−1. | |
Table 3 EIS data for S-Fe corrosion in free 1 M HCl solution and with different concentrations of PS and PBT compounds at 300 K
Inh. |
Inh. conc. (mg l−1) |
Rs, ohm cm−2 |
Rct, ohm cm−2 |
Cdl, (μF cm−2) |
%AE |
— |
0 |
1.2 |
48 |
98 |
— |
PS |
100 |
1.3 |
115 |
45.4 |
58.26 |
200 |
1.5 |
155 |
38.2 |
69.03 |
300 |
1.6 |
245 |
30.8 |
80.41 |
400 |
1.9 |
460 |
22.4 |
89.56 |
500 |
2.1 |
605 |
17.4 |
92.07 |
PBT |
100 |
1.4 |
120 |
42.6 |
60.00 |
200 |
1.6 |
228 |
34.4 |
78.94 |
300 |
1.8 |
312 |
28.6 |
84.61 |
400 |
2.0 |
570 |
18.4 |
91.57 |
500 |
2.2 |
725 |
12.6 |
93.37 |
The electrochemical parameters, such as Rct, Cdl, and AE(EIS)%, are recorded in Table 3. It is clear from this table that with the increase in the concentration of PS and PBT compounds, the Cdl values decreased due to the gradual replacement of water molecules by the adsorption of polymer compounds on the S-Fe surface, which formed a protective film on the S-Fe surface. For more evidence of the investigated inhibitors action against HCl corrosion, Bode and phase angle plots were made and are presented in Fig. 4A and B. As shown in the plots, the impedance modulus increased with the increase in the additive concentration at low frequencies, hence proving the adsorption of the PS and PBT compounds on the S-Fe surface, thus improving the inhibitory effect against HCl solution.41 Also, the appearance of a single peak in the phase angle diagrams illustrated the existence of a single time constant at the iron–solution interface.
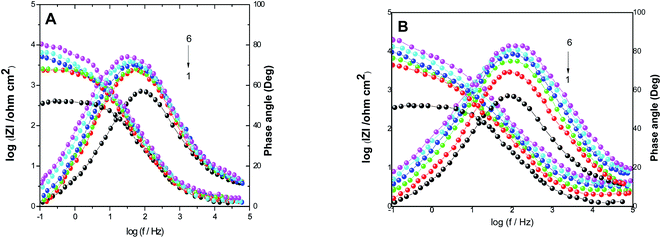 |
| Fig. 4 Bode plots for S-Fe corrosion in the blank 1.0 M HCl solution and when containing different concentrations of (A) PS and (B) PBT at 300 K: (1) 0.0, (2) 100, (3) 200, (4) 300, (5) 400 m (6) 500 mg l−1. | |
3.3. PDAP measurements
PS and PBT compounds were examined as pitting corrosion inhibitors by utilizing PDAP measurements. Fig. 5A and B present the PDAP curves for S-Fe in 1.0 M HCl + 0.5 M NaCl solution and in the presence of some concentrations of PS and PBT compounds, respectively, at a scan rate of 0.2 mV s−1. NaCl solution was applied as a pitting corrosion factor. From this figure, it can be seen that there are no peaks in the anodic scan, which clarified the constancy of the passive film created on the iron surface. The current remained constant until a certain potential, when it rose rapidly due to the demolition of the passive film and formation of the pitting attack. This potential is defined as the pitting potential (Epit.).42,43 With increasing the concentrations of PS and PBT, the values of Epit. moved to the noble (+) direction.
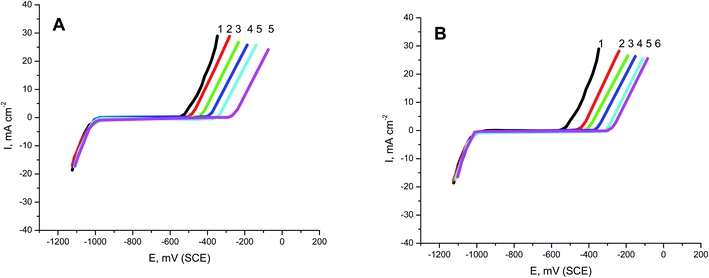 |
| Fig. 5 PDAP curves of S-Fe corrosion in the free 1.0 M HCl + 0.5 M NaCl solution and when containing different concentrations of (A) PS and (B) PBT at 300 K at a scan rate of 0.2 mV s−1: (1) 0.0, (2) 100, (3) 200, (4) 300, (5) 400, (6) 500 mg l−1. | |
Fig. 6 shows the relationship between the Epit. values and the logarithmic of the concentration of the two polymer compounds (PS and PBT). The same behavior was observed in the cases of the two polymers. Broken lines were obtained. At a lower concentricity (100 and 200 mg l−1) of PS and PBT, the shift in the Epit. values to the noble direction were low, but at a concentricity of more than 200 mg l−1, the Epit. values converted quickly to a noble trend according to the following equation:44,45
|
Epit. = γ + β log Cpolymer
| (6) |
where,
γ and
β are constants depending on the nature of the metal or alloy and the inhibitor used.
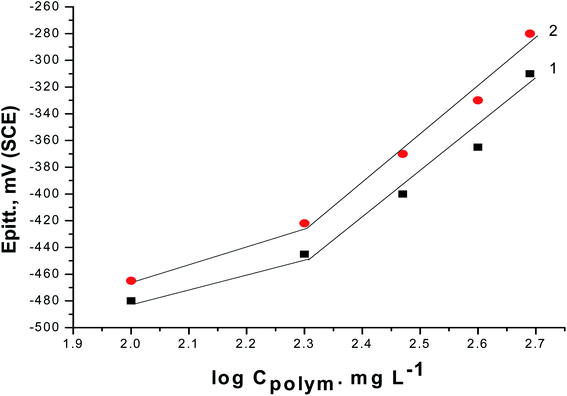 |
| Fig. 6 Relationship between Epitt and the logarithmic concentrations of the two polymer compounds: (1) PS and (2) PBT. | |
These outcomes confirmed that the presence of PS and PBT compounds increased the resistance to pitting corrosion. These compounds could thus be classified as pitting corrosion inhibitors. At all concentrations examined, the noble shift of Epit. (more resistance to pitting corrosion) for PBT compounds was more than for PS compounds. This completely agrees with the data acquired from the GAP and EIS measurements.
3.4. Weight reduction
3.4.1. Impact of the polymer concentrations. The impact of different concentrations of PS and PBT compounds ranging from 100 to 500 mg l−1 on the dissolution of S-Fe in 1.0 M HCl solution was assessed by applying WR methods. The dissolution of S-Fe depended on the area of the coupons utilized and the immersion time. The corrosion rate (Kcorr.) in mg cm−1 min−1 was computed from the subsequent equation:43,44 |
 | (7) |
where ΔW in mg equal to (W1 − W2) and W1, W2 are the weight of S-Fe before and after incubation in the examined solutions, respectively.The percentage anticorrosion efficiency (%AEWR) and surface coverage (θ) were determined from the values of Kcorr. using the following equations:46,47
|
 | (8) |
where
Kcorr.un and
Kcorr.inh are the corrosion rates in the blank 1.0 M HCl free solution and in the presence of two polymer compounds (PS and PBT).
The values of WR, Kcorr., θ, and %AEWR in the cases of the PS and PBT compounds are listed in Tables 4 and 5, respectively. Cleary from this table, with increasing the concentration of the PS and PBT compounds, the WR decreased, which led to an increase in the %AEWR. These data confirmed the inhibitory power of the two polymer compounds was owing to their strong adsorption on the S-Fe surface. The %AEWR reached 93.58% and 94.25% in the cases of 500 mg l−1 of the PS and PBT compounds, respectively. These data were roughly in agreement with the GAP and EIS results.
Table 4 Impact of increasing temperature on the corrosion functions acquired from WR measurements for the corrosion of S-Fe in blank 1.0 M HCl and in the presence of different concentrations of PS compounds
Temperature (K) |
Conc. of PS (mg l−1) |
WR (mg) |
Kcorr. × 10−4 mg cm−2 h−1 |
θ |
AE(WR)% |
303 |
0.00 |
0.296 |
10.067 |
— |
— |
100 |
0.133 |
4.523 |
0.551 |
55.07 |
200 |
0.095 |
3.231 |
0.679 |
67.90 |
300 |
0.059 |
2.001 |
0.801 |
80.12 |
400 |
0.030 |
1.020 |
0.899 |
89.86 |
500 |
0.019 |
0.646 |
0.936 |
93.58 |
313 |
0.00 |
0.389 |
13.229 |
— |
— |
100 |
0.191 |
6.494 |
0.491 |
49.09 |
200 |
0.147 |
4.999 |
0.622 |
62.21 |
300 |
0.097 |
3.298 |
0.751 |
75.07 |
400 |
0.058 |
1.972 |
0.851 |
85.09 |
500 |
0.043 |
1.462 |
0.889 |
88.95 |
323 |
0.00 |
0.478 |
16.256 |
— |
— |
100 |
0.267 |
9.081 |
0.441 |
44.14 |
200 |
0.205 |
6.972 |
0.561 |
56.11 |
300 |
0.138 |
4.693 |
0.711 |
71.13 |
400 |
0.091 |
3.095 |
0.809 |
80.96 |
500 |
0.076 |
2.585 |
0.841 |
84.09 |
333 |
0.00 |
0.562 |
19.113 |
— |
— |
100 |
0.337 |
11.461 |
0.400 |
40.03 |
200 |
0.275 |
9.355 |
0.511 |
51.05 |
300 |
0.185 |
6.292 |
0.671 |
67.08 |
400 |
0.135 |
4.591 |
0.760 |
75.98 |
500 |
0.112 |
3.809 |
0.801 |
80.07 |
Table 5 Impact of increasing temperature on the corrosion parameters acquired from WR measurements for the corrosion of S-Fe in blank 1.0 M HCl and in the presence of different concentrations of PBT compounds
Temperature (K) |
Conc. of PS (mg l−1) |
WR (mg) |
Kcorr. × 10−4 mg cm−2 h−1 |
θ |
AE(WR)% |
303 |
0.00 |
0.296 |
10.067 |
— |
— |
100 |
0.121 |
4.115 |
0.591 |
59.12 |
200 |
0.079 |
2.687 |
0.733 |
73.31 |
300 |
0.053 |
1.802 |
0.821 |
82.09 |
400 |
0.029 |
0.896 |
0.919 |
91.09 |
500 |
0.017 |
0.578 |
0.943 |
94.25 |
313 |
0.00 |
0.389 |
13.229 |
— |
— |
100 |
0.167 |
5.679 |
0.571 |
57.07 |
200 |
0.121 |
4.115 |
0.689 |
68.89 |
300 |
0.089 |
3.026 |
0.771 |
77.13 |
400 |
0.054 |
1.836 |
0.861 |
86.12 |
500 |
0.042 |
1.428 |
0.892 |
89.20 |
323 |
0.00 |
0.478 |
16.256 |
— |
— |
100 |
0.219 |
7.448 |
0.542 |
54.18 |
200 |
0.192 |
6.529 |
0.598 |
59.83 |
300 |
0.162 |
5.509 |
0.661 |
66.11 |
400 |
0.114 |
3.877 |
0.762 |
76.15 |
500 |
0.100 |
3.401 |
0.791 |
79.08 |
323 |
0.00 |
0.562 |
19.113 |
— |
— |
100 |
0.281 |
9.556 |
0.500 |
49.99 |
200 |
0.253 |
8.604 |
0.550 |
54.98 |
300 |
0.213 |
7.244 |
0.621 |
62.10 |
400 |
0.162 |
5.510 |
0.712 |
71.17 |
500 |
0.141 |
4.795 |
0.749 |
74.91 |
3.4.2. Impact of elevated temperature. The effect of the elevated temperature from 300 K to 330 K on the corrosion data can be seen in the WR measurements of S-Fe in free 1.0 M HCl solution and when including different concentrations of PS and PBT compounds, and the results are tabulated in Tables 4 and 5. As the examined solution temperature increased from 303 K to 333 K, the values of WR and Kcorr. increased, while the values of θ and %AEWR declined. This demonstrated the physical adsorption of PS and PBT compounds on the S-Fe surface via van der Waals interaction. High temperature may destroy the physical bonds, making the polymer compound desorb from the surface S-Fe.48–50
3.4.3. Activation thermodynamic parameters. The values of the activation energy
for the dissolution of S-Fe in the blank 1.0 M HCl solutions and when adding specific concentrations ranging from 100 ppm to 500 ppm of PS and PBT compounds were evaluated by utilizing the Arrhenius equation as follows:50,51 |
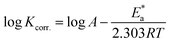 | (9) |
where A, R, and T represent the Arrhenius constant, gas constant, and absolute temperature, respectively.Fig. 7A and B exhibit the plots of log
Kcorr. and 1000/T for S-Fe in the 1 M HCl solution alone and in the presence of different concentrations of PS and PBT compounds. Straight lines were obtained with the linear regression coefficients nearly equal to one. The
values were assessed from the slope of the straight lines and are tabulated in Table 6. Obviously from the this table, it can be seen that the values of
in the presence of the two polymer compounds were greater than that observed in the blank 1.0 M HCl solution and the values of
increased with increasing the concentration of PS and PBT compounds. The increased activation energy of S-Fe corrosion indicated that the PS and PBT compounds acted as anticorrosion agents so as to delay the corrosion of S-Fe by the formation of a mass and charge-transfer barrier through them being adsorbed on the surface of S-Fe.
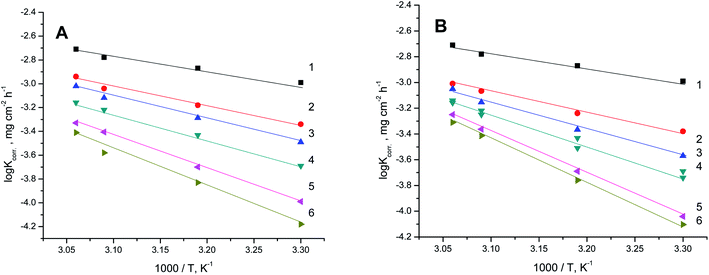 |
| Fig. 7 Plots of log Kcorr. versus 1/T for S-Fe in the free 1 M HCl solution and in the presence of different concentrations of (A) PS and (B) PBT compounds. (1) 0.0, (2) 100, (3) 200, (4) 300, (5) 400, (6) 500 mg l−1. | |
Table 6 Kinetics parameters for S-Fe in the blank 1 M HCl solution and in the presence of different concentrations of PS and PBT
Conc of inh. (ppm) |
Ea kJ mol−1 |
ΔH* kJ mol−1 |
ΔS* J mol−1 K−1 |
1 M HCl |
18.19 |
14.08 |
213.51 |
1 M HCl + 100 ppm PS |
22.98 |
21.16 |
265.62 |
1 M HCl + 200 ppm PS |
30.72 |
28.72 |
303.76 |
1 M HCl + 300 ppm PS |
36.63 |
32.67 |
335.42 |
1 M HCl + 400 ppm PS |
40.29 |
34.46 |
372.01 |
1 M HCl + 500 ppm PS |
48.86 |
39.43 |
398.28 |
1 M HCl + 100 ppm PBT |
26.81 |
25.68 |
295.92 |
1 M HCl + 200 ppm PBT |
36.53 |
30.86 |
313.86 |
1 M HCl + 300 ppm PBT |
45.95 |
44.33 |
356.80 |
1 M HCl + 400 ppm PBT |
58.25 |
51.44 |
394.84 |
1 M HCl + 500 ppm PBT |
68.92 |
60.29 |
436.26 |
The enthalpy (ΔH*) and entropy (ΔS*) of activation were determined by utilizing the following transition state equation:50,51
|
 | (10) |
where
h and
N are Planck's constant and Avogadro's number, respectively.
Fig. 8A and B present the relationship between
versus
for S-Fe in the blank 1 M HCl solution and in the presence of different concentrations of PS and PBT compounds. Straight lines were obtained. The ΔH* and ΔS* values were computed from the slopes and the intersections of the straight lines and are recorded in Table 6. It is evident from this table that the ΔH* values were positive in 1 M HCl solution alone and in the presence of different concentrations of the two polymer compounds (PS and PBT). This indicated the endothermic nature of the formation of the activated complexes during the corrosion process. Also, the values of ΔH* became more positive with increasing the concentrations of the PS and PBT, which made it more difficult for iron to corrode.52 The values of ΔSo were negative and became less positive when the concentration of the two polymer compounds increased. This suggested that the compound activated in the rate-limiting step was involved in binding not disengagement. This clarifies that the activated molecules were in a less chaotic state than those in the initial stage.53 The anticorrosion efficiency of PS and PBT compounds with respect to the rise in the values of
and ΔH*and the decline in the values of ΔS* followed the order: PBT > PS.
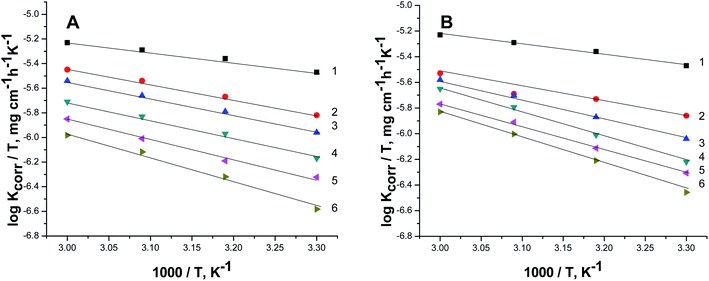 |
| Fig. 8 Plots of log Kcorr./T versus 1/T for S-Fe in the free 1 M HCl solution and in the presence of different concentrations of (A) PS and (B) PBT compounds: (1) 0.0, (2) 100, (3) 200, (4) 300, (5) 400, (6) 500 mg l−1. | |
3.5. Adsorption and mechanism of the anticorrosion process
The anticorrosiveness of the two investigated polymer compounds (PS and PBT) on the corrosion of S-Fe in 1 M HCl solution principally relied on its adsorption at the S-Fe surface interface. On the whole, the adsorption operation involves an exchange process between the polymer compounds in the aqueous phase [polymer(aq.)] and the number of water molecules adsorbed on the S-Fe surface due to this equation: |
Polymer(aq.) + αH2O(surf.) = polymer(surf.) + αH2O(aq.)
| (11) |
where α is the amount of adsorbed water molecules that are replaced by one polymer molecule. The adsorption of PS and PBT on the S-Fe surface depended on several factors, including the concentration of the mineral acid and the polymer compounds used, the molar mass of the polymer, the temperature, the presence of certain active centers in the chemical structure of the polymer. The adsorption of the two polymer compounds on the surface of S-Fe diminished the rate of corrosion and elevated the anticorrosion effectiveness. To choose a suitable isotherm for this adsorption, the surface coverage values (θ) were applied to several isotherms. The results obtained confirmed that the preferable isotherm was the Langmuir isotherm, which can be given by:54 |
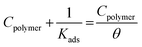 | (12) |
where Cpolymer is the concentricity of the polymer used and Kads is the equilibrium constant of adsorption.
Fig. 9A and B present the Langmuir plots (Cpolymer and Cpolymer/θ) for the adsorption of the two polymer compounds (PS and PBT) on the surface of S-Fe at altered temperatures ranging from 303 K to 333 K. A straight line with a slope of about one was obtained, which confirmed that the Langmuir isotherm was a suitable isotherm. This isotherm assumed there was zero interaction between the adsorbed species on the S-Fe surface. From the intercept of the Langmuir plots, we computed the values of Kads. Specifically, the values of Kads for the PS compounds were computed from Fig. 9A and were (6.45, 5.71, 5.12, and 4.54) × 10−3 mol l−1 at temperatures of 303, 313, 323, and 333 K, respectively; while the values of Kads for the PBT compounds were computed from Fig. 9B and were (6.25, 5.40, 4.82, and 4.04) × 10−3 mol l−1 at the temperatures of 303, 313, 323, and 333 K, respectively. The values of Kads indicated that both PS and PBT on the surface of S-Fe were readily and cohesively adsorbed on the surface of S-Fe.
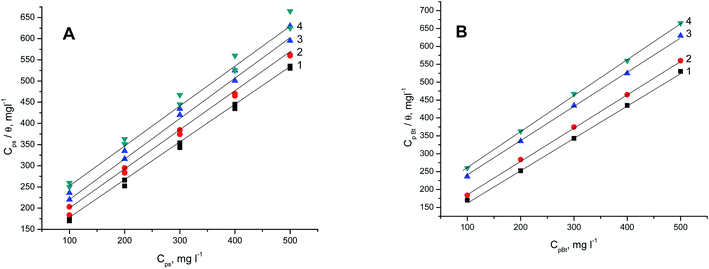 |
| Fig. 9 Langmuir adsorption isotherms for S-Fe in the blank 1 M HCl solution and in the presence of different concentrations of (A) PS and (B) PBT compounds at: (1) 303 K, (2) 313 K, (3) 323 K, (4) 333 K. | |
The values of free energy of adsorption (ΔGoads) were determined from the values of Kads according to the following equation:
|
55.5Kads = exp(−ΔGoads/RT)
| (13) |
The determined values of ΔGoads in the presence of PS compounds were −28.32, −27.14, −26.97, and −26.02 kJ mol−1 at temperatures of 303, 313, 323, and 333 K, respectively; while the values of ΔGoads in the presence of PBT compounds were −25.08, −24.42, −24.06, and −23.83 kJ mol−1 at temperatures of 303, 313, 323, and 333 K, respectively. It is evident that all the values of ΔGoads were negative, demonstrating that the adsorption of PS and PBT compounds on S-Fe was spontaneous. Vant's Hoff equation was applied to determine the enthalpy of adsorption ΔHoads according to the following equation:55
|
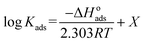 | (14) |
where
X is a constant.
Fig. 10 displays the relationship between log
Kads vs. 1/T (Van't Hoff plots) for PS and PBT adsorbed on the surface of S-Fe in 1.0 M HCl solution. Straight lines were obtained. From the slope of the straight lines, we could compute ΔHoads. The values of ΔHoads were −19.88 and −21.12 kJ mol−1 for PS and PBT compounds, respectively. The negative mark of ΔHoads indicated that the adsorption of PS and PBT compounds on the S-Fe surface was exothermic.
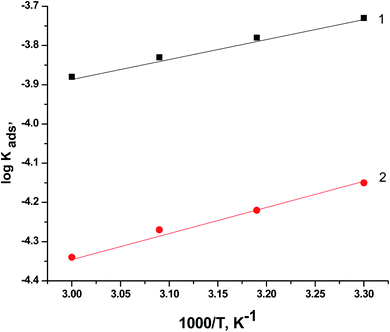 |
| Fig. 10 Plots of log Kads versus 1000/T for the corrosion of S-Fe in 1.0 M HCl solution in the presence of PS and PBT compounds at different temperatures: (1) PBT and (2) PS. | |
The entropy of adsorption (ΔSads) values were obtained from the following equation:
|
 | (15) |
The computed values of ΔSads for PS compounds were −0.159, −0.086, −0.022, and −0.018 kJ mol−1 at temperatures of 303, 313, 323, and 333 K, respectively; while the values of ΔSads for PBT compounds were −0.013, −0.011, −0.009, and −0.008 kJ mol−1 at temperatures of 303, 313, 323, and 333 K, respectively.
The negative markings of ΔSads indicated that the chaos diminished upon transition from the reactant to the adsorbent surface. This demonstrates the adsorption intensity of the PS and PBT compounds on the surface of S-Fe. The values of the adsorption thermodynamic functions were consistent with the low values of the anticorrosion efficiency at elevated temperatures.
The corrosion functions and the anticorrosion efficiency gained from the various technologies confirmed that the %AE of PS compounds was greater than that of PBT compounds. These outcomes can be explicated on the basis of the molar mass of the polymer compound tested, where the molar mass of PBT is greater than that of PS. Thus PBT compounds will create a larger protective-layer covering on the surface than PS, giving a higher anticorrosion efficiency. This layer isolates the surface of the iron from the corrosive acidic solution and prevents contact of the S-Fe surface with the acidic solution.
The results of the PDAP measurements showed that the PS and PBT compounds had a high ability to resist the pitting corrosion by shifting the value of the pitting potential to the noble (+) direction. The resistance of pitting attack can be explicated by the competitive adsorption that occurred on the S-Fe surface between Cl− ions and the PS and PBT compounds to reach the surface of S-Fe until the polymer compounds became dominant. This led to the formation of a protective layer on the surface of S-Fe, which impeded the pitting attack of chloride ions.
3.6. Computational study
The anticorrosion efficiency of the dimer and trimer of butylene terephthalate and styrene were investigated by finding the quantum parameters that correlated with the practical outcomes. The optimized geometries of the dimer and trimer of the two inhibitors are presented in Fig. 11 and 12. The optimizations were obtained by using the B3LYP level of theory. As shown in Table 7, most the quantum parameter values for the dimer and trimer of the butylene terephthalate inhibitor were the same, but there were slight changes between the dimer and trimer of the styrene inhibitor, so the frontier orbitals, molecular electrostatic potential, and adsorption of the polymer on the iron surface were investigated using the dimer molecules. The frontier molecular orbital (HOMO and LUMO) distributions could show the active sites that the inhibitors possess to interact with a metallic surface. As seen in Fig. 13, for the dimer of butylene terephthalate the HOMO was concentrated on the benzaldehyde moiety and LUMO in the terephthalate region, while the HOMO and LUMO distributions of the dimer of styrene were the same, indicating a high chance of electron transfer from the HOMO to the LUMO with bonding formation between the tested reagents.54 The high values of EHOMO are likely to indicate a molecule's tendency to donate electrons to suitable acceptor molecules with empty molecular orbitals. The LUMO energy reflects a molecule's ability to receive electrons. So, with increasing the HOMO and lowering the LUMO energy levels, the inhibitor's ability to attach to the metal surface is improved. As shown in Table 7, the EHOMO and ELUMO of the dimer of butylene terephthalate were lower than the dimer of styrene. The energy gap (ΔE) is another parameter that is correlated with the anticorrosion efficiency. A lower energy gap value indicates a higher inhibition efficiency, because the energy of removing an electron from the highest occupied orbital is low;55 therefore, the higher reactivity of an inhibitor toward adsorption on metallic surfaces. The results obtained, as seen in Table 7, indicate that the dimer of butylene terephthalate had better anticorrosion efficiency than the dimer of styrene.
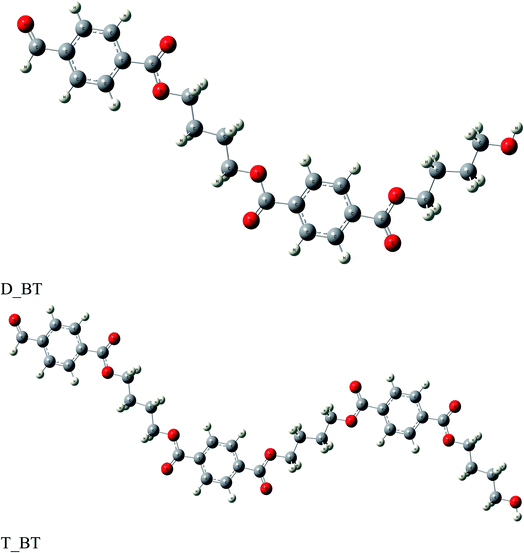 |
| Fig. 11 Optimized structure of di- and tri-butylene terephthalate. | |
 |
| Fig. 12 Optimized structure of di- and tri-styrene. | |
Table 7 Quantum parameters for di-, tri- PBT and PS calculated using B3LYP/6-31g(d,p) in the aqueous phase
|
EHOMO (eV) |
ELUMO (eV) |
ΔE (eV) |
μ (debye) |
σ (eV)−1 |
η (eV) |
ΔEd-b (eV) |
D_PS |
−6.39 |
−0.17 |
6.22 |
0.0001 |
0.32 |
3.11 |
−0.78 |
T_PS |
−6.39 |
−0.19 |
6.20 |
0.57 |
0.33 |
3.10 |
−0.77 |
D_PBT |
−7.27 |
−2.35 |
4.92 |
2.41 |
0.41 |
2.46 |
−0.62 |
T_PBT |
−7.27 |
−2.35 |
4.92 |
6.46 |
0.41 |
2.46 |
−0.62 |
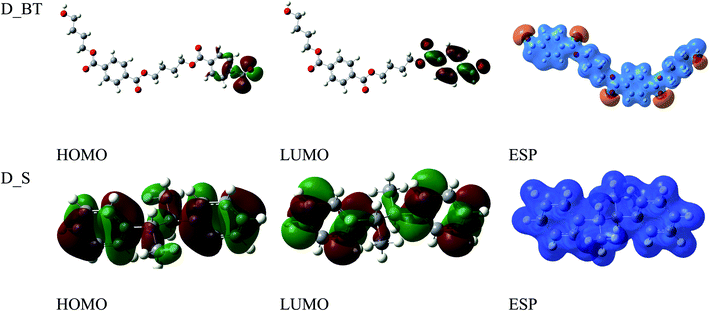 |
| Fig. 13 Frontier orbitals and ESP of di-butylene terephthalate and di-styrene. | |
The dipole moment (μ) is an index for predicting the direction of a corrosion inhibition process. The dipole moment is a measurement of polarity in a bond, which is linked to the electron distribution in a molecule. It is generally accepted that the adsorption of polar compounds with high dipole moments on the metal surface should result in improved anticorrosion efficiency. As shown in Table 7, the dipole moment of the dimer of butylene terephthalate was higher than that of the dimer of the styrene inhibitor, which showed a high symmetry of 0.0001 debye; therefore, the dimer of butylene terephthalate had a better anticorrosion efficiency than the dimer of styrene, which agreed with the experimental results.
The molecular electrostatic potential (ESP) is an important quantitative descriptor used to determine the reactive sites of electrophilic and nucleophilic attacks. As seen in Fig. 13, the negative electrostatic potential (red regions) for the dimer of butylene terephthalate was concentrated on the oxygen atom of C
O, indicating the acceptor sites. The positive electrostatic potential (blue regions) for the dimer of styrene was concentrated in all the molecules, which were thus associated with donor sites.
The softness (σ) and hardness (η) are called global reactivity descriptors. These parameters can be used to obtain the reactivity and stability of a molecule. The adsorption of polymer molecules on the S-Fe surface occur with a molecule that has a higher value of softness. Global hardness is a measure of the molecular resistance to electron cloud polarization or deformation due to minor chemical reaction disturbances. Soft molecules have a small energy gap but hard molecules have a higher energy gap.56 Generally, the inhibitor with the highest value of softness gives the highest anticorrosion efficiency.57,58 As presented in Table 7, the dimer of butylene terephthalate with a softness of 0.41 had the highest anticorrosion efficiency.
The back-donation of electrons (ΔEb-d) increases with the increase in anticorrosion efficiency. As shown in Table 7, this suggests the dimer of butylene terephthalate enhanced the back-donation when compared with the dimer of styrene.
3.7. MC simulation
The interaction assessment of the two dimers of butylene terephthalate and styrene on the Fe (110) surface was performed using Monte Carlo simulation.59 Fig. 14 shows the most stable adsorption configurations of the polymer molecule on the Fe (110) surface. The dimer of butylene terephthalate was adsorbed in parallel on the Fe (110), but for the dimer of styrene, we found that the molecule was adsorbed by the two benzene rings tilted on the Fe (110) surface. When comparing the adsorption energy of the two inhibitors, as presented in Table 8, the dimer of butylene terephthalate had a higher adsorption energy than the dimer of styrene. These results confirmed the dimer of butylene terephthalate had a higher anticorrosion efficiency than the dimer of styrene. These results coincided with the experimental anticorrosion efficiencies.
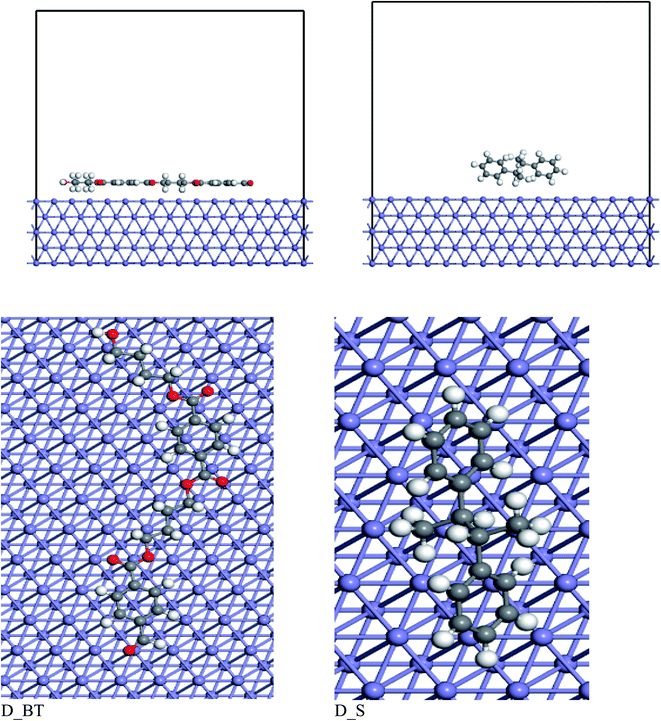 |
| Fig. 14 Top and side views for adsorption of the two dimers over Fe (110) surface. | |
Table 8 Adsorption energies of the two dimer inhibitors over the Fe (110) surface
System |
Adsorption energy (kcal mol−1) |
D_PS + Fe (110) |
−82.95 |
D_PBT + Fe (110) |
−231.06 |
4. Conclusions
The two polymer compounds (PS and PBT) acted as a good inhibitor for the corrosion of S-Fe in 1.0 M HCl solution. The anticorrosive efficiency rises with rising the concentrations of PS and PBT compounds. All the chemical, electrochemical and theoretical studies confirm that the anticorrosive efficiency of PBT is more than PS compound. The anticorrosive strength of these compounds was explicated by vigor spontaneous adsorption of these compounds on the S-Fe surface. The adsorption obeyed Langmuir isotherm. This theoretical study aims to compare the two polymer inhibitors based on quantum descriptors obtained by DFT. MC simulation was employed to determine the picture of the inhibitor molecule over the Fe (110) surface. The theoretical parameters are in agreement with experimental results.
Conflicts of interest
There are no conflicts to declare.
Acknowledgements
The authors would like to thank the Deanship of Scientific Research at Umm Al-Qura University for supporting this work with a grant, Grant Code: (22UQU4320006DSR01)
References
- M. Abdallah, A. S. Al-Gorair, A. Fawzy, H. Hawsawi and R. S. Abdel Hameed, Enhancement of adsorption and anticorrosion performance of two polymeric compounds for the corrosion of SABIC carbon steel in hydrochloric acid, J. Adhes. Sci. Technol., 2022, 36, 35–53 CrossRef CAS.
- M. Abdallah, A. Fawzy, H. Hawsawi, R. S. A. Hameed and S. S. Al-Juaid, Estimation of Water-Soluble Polymers (Poloxamer and Pectin) as Corrosion Inhibitors for Carbon Steel in Acidic Medium, Int. J. Electrochem. Sci., 2020, 15, 8129–8144 CrossRef.
- Z. Hajiahmadi and Z. Tavangar, Extensive theoretical study of corrosion inhibition efficiency of some pyrimidine derivatives on iron and the proposal of new inhibitor, J. Mol. Liq., 2019, 284, 225–231 CrossRef CAS.
- R. S. Abdel Hameed, A. H. Al-Bagawi, H. A. Shehata, A. H. Shamroukh and M. Abdallah, Corrosion Inhibition and Adsorption Properties of Some Heterocyclic Derivatives on C-Steel Surface in HCl, Journal of Bio- and Tribo-Corrosion, 2020, 51(6), 1–11 Search PubMed.
- R. S. Abdel Hameed and M. Abdallah, Corrosion inhibition of carbon steel in 1.0 M hydrochloric acid using some pyrazolo[3,4-d]Pyrimidnone derivatives, Prot. Met. Phys. Chem. Surf., 2018, 54, 113–121 CrossRef CAS.
- M. Abdallah, H. M. Altass, B. A. AL Jahdaly and M. M. Salem, Some natural aqueous extracts of plants as green inhibitor for dissolution of carbon steel in 0.5 M sulfuric acid, Green Chem. Lett. Rev., 2018, 11, 189–196 CrossRef CAS.
- M. Abdallah, E. A. Helal and A. S. Fouda, Amino pyrimidine derivatives as inhibitors for corrosion of 1018 carbon steel in nitric acid solutions, Corros. Sci., 2006, 48, 1639–1654 CrossRef CAS.
- X. Li, S. Deng and H. Fu, Allyl thiourea as a corrosion inhibitor for cold rolled steel in H3PO4 solution, Corros. Sci., 2012, 55, 280–288 CrossRef CAS.
- J. H. Al-Fahemia, M. Abdallah, E. A. M. Gad and B. A. AL Jahdaly, Experimental and theoretical approach studies for melatonin drug as safely corrosion inhibitors for carbon steel using DFT, J. Mol. Liq., 2016, 222, 1157–1163 CrossRef.
- F. Benhiba, R. Hsissou, Z. Benzekri, M. E. Belghiti, A. Lamhamdi, A. Bellaouchou, A. Guenbour, S. Boukhris, H. Oudda, I. Warad and A. Zarrouk, Nitro substituent effect on the electronic behavior and inhibitory performance of two quinoxaline derivatives in relation to the corrosion of mild steel in 1 M HCl, J. Mol. Liq., 2020, 312, 113367 CrossRef CAS.
- M. Abdallah, H. M. Altass, A. S. Al-Gorair, J. H. Al-Fahemi and K. A. Soliman, Natural nutmeg oil as a green corrosion inhibitor for carbon steel in 1.0 M HCl solution: Chemical, electrochemical and computational methods, J. Mol. Liq., 2021, 323, 115036 CrossRef CAS.
- M. Abdallah, N. El Guesmi, A. S. Al-Gorair, R. El-Sayed, A. Meshabi and M. Sobhi, Enhancing the anticorrosion performance of mild steel in sulfuric acid using synthetic non-ionic surfactants: Practical and Theoretical Studies, Green Chem. Lett. Rev., 2021, 14(2), 382–394 CrossRef CAS.
- A. Berrissoul, E. Loukili, N. Mechbal, F. Benhiba, A. Guenbour, B. Dikici, A. Zarrouk and A. Dafali, Anticorrosion effect of a green sustainable inhibitor on mild steel in hydrochloric acid, J. Colloid Interface Sci., 2020, 580, 740–752 CrossRef CAS PubMed.
- S. Kaya, C. Kaya, L. Guo, F. Kandemirlic, B. Tüzün, İ. Uğurlu, L. H. Madkour and M. Saraçoğlu, Quantum chemical and molecular dynamics simulation studies on inhibition performances of some thiazole and thiadiazole derivatives against corrosion of iron, J. Mol. Liq., 2016, 219, 497–504 CrossRef CAS.
- K. Efil and I. B. Obot, Quantum Chemical Investigation of the Relationship Between Molecular Structure and Corrosion Inhibition Efficiency of Benzotriazole and its Alkyl-Derivatives on Iron, Prot. Met. Phys. Chem. Surf., 2017, 53, 1139–1149 CrossRef CAS.
- M. Abdallah, K. A. Soliman, B. A. Al Jahdaly, J. H. Al-Fahemi, H. Hawsawi, H. M. Altass, M. Sobhi and S. S. Al-Juaid, Natural parsley oil as a green and safe inhibitor for corrosion of X80 carbon steel in 0.5M H2SO4 solution: Chemical, electrochemical, DFT and MC simulation approach, RSC Adv., 2022, 12, 2959–2971 RSC.
- F. M. Al-Nowaiser, M. Abdallah and E. H. El-Mossalamy, N,N-di (Polyoxyethylene)-4-dodecylaniline as an inhibitors of iron in hydrochloric acid solutions, Chem. Technol. Fuels Oils, 2012, 47(6), 453–463 CrossRef CAS.
- M. Abdallah, K. A. Soliman, A. S. Al-Gorair, A. Al Bahir, J. H. Al-Fahemi, M. S. Motawea and S. S. Al-Juaid, Enhancing the inhibition and adsorption performance of SABIC iron corrosion in sulfuric acid by expired vitamins. Experimental and computational approach, RSC Adv., 2021, 11, 17092–17107 RSC.
- M. Abdallah, I. Zaafarany, J. H. Al-Fahemi, Y. Abdallah and A. S. Fouda, Antibacterial cephalosporin as inhibitors for the corrosion of iron in hydrochloric acid solutions, Int. J. Electrochem. Sci., 2012, 7(8), 6622–6637 CAS.
- N. I. Podobaev and Y. G. Avdeev, Prot. Met., 2004, 40, 7–13 CrossRef CAS.
- A. Fawzy, M. Abdallah, M. Alfakeer and H. M. Ali, Corrosion Inhibition of Sabic Iron in Different Media Using Synthesized Sodium N-dodecyl Arginine Surfactant, Int. J. Electrochem. Sci., 2019, 14, 2063–2084 CrossRef CAS.
- M. Abdallah, A. Al Bahir, H. M. Altass, A. Fawzy, N. El Guesmi, A. S. Al-Gorair, F. Benhiba, I. Warad and A. Zarrouk, Anticorrosion and adsorption performance of expired antibacterial drugs on Sabic iron corrosion in HCl solution: Chemical, electrochemical and Theoretical Approach, J. Mol. Liq., 2021, 330, 115702 CrossRef CAS.
- M. Abdallah, A. Fawzy and A. Al Bahir, The Effect of Expired Acyclovir and Omeprazole Drugs on the Inhibition of Sabic Iron Corrosion in HCl Solution, Int. J. Electrochem. Sci., 2020, 15, 4739–4753 CrossRef CAS.
- M. Abdallah, A. Fawzy and A. Al Bahir, Expired amoxicillin and cefuroxime drugs as efficient anticorrosive for Sabic iron in 1.0 M hydrochloric acid solution, Chem. Eng. Commun., 2022, 209(2), 158–170 CrossRef CAS.
- A. S. Al-Gorair, H. Hawsawi, A. Fawzy, M. Sobhi, A. M. Alharbi and R. S. Abdel Hameed, Evaluation of the Anticorrosion and Adsorption Properties of Polyethylene Glycol and Polyvinyl Alcohol for Corrosion of SABIC Iron in 1.0 M NaCl Solution, Int. J. Electrochem. Sci., 2021, 16, 211119, DOI:10.20964/2021.11.03.
- S. A. Umoren, Polymers as Corrosion Inhibitors for Metals in Different Media - A Review, Open Corros. J., 2009, 2, 175–188 CrossRef CAS.
- R. S. Abdel Hameed, A. El-Zomrawy, M. Abdallah, S. S. Abed El Rehim, H. I. AlShafey and N. I. Shaher, Polyoxyethylene stearate of molecular weight 6000 as corrosion inhibitor for mild steel in 2.0 M sulphuric acid, International Journal of Corrosion and Scale Inhibition, 2017, 6, 196–208 CAS.
- A. Ali, F. Sabirneeza, R. Geethanjali and S. Subhashini, Polymeric corrosion inhibitors for iron and its alloys: A Review, Chem. Eng. Commun., 2015, 202, 232–244 CrossRef.
- F. M. Al-Nowaiser, M. Abdallah and E. H. El-Mossalamy, N,N-di (Polyoxyethylene)-4-dodecylaniline as an inhibitors of iron in hydrochloric acid solutions, Chem. Technol. Fuels Oils, 2012, 47, 453–463 CrossRef CAS.
- D. E. Arthur, A. Jonathan, P. Ocheje Ameh and C. Anya, Int. J. Ind. Chem., 2013, 4, 2 CrossRef.
- M. Abdallah, A. Fawzy and H. Hawsawi, Maltodextrin and Chitosan Polymers as Inhibitors for the Corrosion of Carbon Steel in in 1.0 M Hydrochloric Acid, Int. J. Electrochem. Sci., 2020, 15, 5650–5663 CrossRef CAS.
- M. Beniken, R. Salim, E. Ech-chihbi, M. Sfaira, B. Hammouti, M. Ebn Touhami, M. A. Mohsin and M. Taleb, Adsorption behavior and corrosion inhibition mechanism of a polyacrylamide on C–steel in 0.5 M H2SO4: Electrochemical assessments and molecular dynamic simulation, J. Mol. Liq., 2022, 348, 118022 CrossRef CAS.
- M. D. Shittu, J. O. Olawale, M. O. Adeoye, K. M. Oluwasegun, K. M. Adebayo and O. O. Ige, Investigation of Corrosion Resistance of Polystyrene as an Inhibitor in Hydrochloric and Tetra-oxo Sulphate VI Acids, Int. J. Mater. Chem., 2014, 4(1), 9–13 Search PubMed.
- M. M. Khowdiary, A. A. El-Henawy, A. M. Shawky and N. A. Negm, Synthesis and evaluation of novel corrosion inhibitors utilized from the recycling of polyethylene terephthalate polymer: Gravimetric, electrochemical, quantum chemical modeling, and molecular docking studies, J. Mol. Liq., 2020, 320(part B), 114504 CrossRef CAS.
- P. B. Mathur and T. Vasudevan, Reaction-rate studies for the corrosion of metals in acids: Iron in mineral acids, Corrosion, 1982, 38, 17–25 CrossRef.
- V. Barone and M. Cossi, Quantum calculation of Molecular Energies and Energy Gradient in Solution by a Conductor Solvent Model, J. Phys. Chem. A, 1988, 102, 1995–2001 CrossRef.
- M. J. Frisch, G. W. Trucks, H. B. Schlegel, G. E. Scuseria, M. A. Robb, J. R. Cheeseman, G. Scalmani, V. Barone, B. Mennucci, G. A. Petersson, H. Nakatsuji, M. Caricato, X. Li, H. P. Hratchian, A. F. Izmaylov, J. Bloino, G. Zheng, J. L. Sonnenberg, M. Hada, M. Ehara, K. Toyota, R. Fukuda, J. Hasegawa, M. Ishid, T. Nakajima, Y. Honda, O. Kitao, H. Nakai, T. Vreven, J. A. Montgomery, J. E. Peralta, F. Ogliaro, M. Bearpark, J. J. Heyd, E. Brothers, K. N. Kudin, V. N. Staroverov, T. Keith, R. Kobayashi, J. Normand, K. Raghavachari, A. Rendell, J. C. Burant, S. S. Iyengar, J. Tomasi, M. Cossi, N. Rega, J. M. Millam, M. Klene, J. E. Knox, J. B. Cross, V. Bakken, C. Adamo, J. Jaramillo, R. Gomperts, R. E. Stratmann, O. Yazyev, A. J. Austin, R. Cammi, C. Pomelli, J. W. Ochterski, R. L. Martin, K. Morokuma, V. G. Zakr-Zewski, G. A. Voth, P. Salvador, J. J. Dannenberg, S. Dapprich, A. D. Daniels, O. Farkas, J. B. Foresman, J. V. Ortiz, J. Cioslowski and D. J. Fox, Gaussian, Inc., Wallingford CT, 2010.
- I.-C. Yeh and M. L. Berkowitz, Ewald summation for systems with slab geometry, J. Chem. Phys., 1999, 111, 3155–3162 CrossRef CAS.
- G. Quartarone, M. Battilana, L. Bonaldo and T. Tartato, Investigation of the inhibition effect of indole-3-carboxylic acid on the copper corrosion in 0.5 M H2SO4, Corros. Sci., 2008, 50, 3467–3474 CrossRef CAS.
- H. Gerengi and H. I. Sahin, Schinopsis lorentzii Extract As a Green Corrosion Inhibitor for Low Carbon Steel in 1 M HCl Solution, Ind. Eng. Chem. Res., 2012, 51, 780–788 CrossRef.
- A. K. Singh and M. A. Quraishi, Inhibiting effects of 5-substituted isatin-based Mannich bases on the corrosion of mild steel in hydrochloric acid solution, J. Appl. Chem., 2010, 40, 1293–1306 CAS.
- M. Abdallah, B. H. Asghar, I. Zaafarany and M. Sobhi, Synthesis of some aromatic nitro compounds and its applications as inhibitors for corrosion of carbon steel in hydrochloric acid solution, Prot. Met. Phys. Chem. Surf., 2013, 49(4), 485–491 CrossRef CAS.
- M. Abdallah and A. I. Mead, Pitting corrosion of an iron electrode in HCO3−/Cl− solutions and its inhibition by some non ionic surfactants, Ann. Chim., 1993, 83, 424–430 Search PubMed.
- M. Abdallah and S. M. Abd El-Haleem, Initiation and inhibition of pitting corrosion of Incoloy 800 and 316 Stainless Steel, Bull. Electrochem., 1996, 12(7–8), 449–458 CAS.
- M. Abdallah, S. A. Al Karane and A. A. Abdel Fattah, Inhibition of the corrosion of nickel and its alloys by natural clove oil, Chem. Eng. Commun., 2009, 196, 1406–1416 CrossRef CAS.
- M. Sobhi, M. Abdallah and K. S. Khairou, Sildenafil citrate (Viagra) as a corrosion inhibitor for carbon steel in hydrochloric acid solutions, Monatsh. Chem., 2012, 143(10), 1379–1387 CrossRef CAS.
- M. Abdallah, H. M. Eltass, M. A. Hegazy and H. Ahmed, Adsorption and inhibition effect of novel cationic surfactant for pipelines carbon steel in acidic solutions, Prot. Met. Phys. Chem. Surf., 2016, 52(4), 721–730 CrossRef CAS.
- I. B. Obot, N. O. Obi-Egbedi and A. O. Eseola, Anticorrosion Potential of 2-Mesityl-1Himidazo[4,5-f][1,10]phenanthroline on Mild Steel in Sulfuric Acid Solution: Experimental and Theoretical Study, Ind. Eng. Chem. Res., 2011, 50, 2098–2110 CrossRef CAS.
- D. K. Yadav and M. A. Quraishi, Application of Some Condensed Uracils as Corrosion Inhibitors for Mild Steel: Gravimetric, Electrochemical, Surface Morphological, UV– Visible, and Theoretical Investigations, Ind. Eng. Chem. Res., 2012, 51, 14966–14979 CrossRef CAS.
- O. L. Riggs Jr and R. M. Hurd, Temperature coefficient of corrosion inhibition, Corrosion, 1967, 23, 252–258 CrossRef.
- K. J. Laider, Chemical Kinetics, Mc Graw Hill Publishing Company Ltd, 1965 Search PubMed.
- M. Abdallah and B. A. AL Jahdaly, Gentamicin, kanamycin and amikacin drugs as non -toxic inhibitors for corrosion of aluminum in 1.0M hydrochloric acid, Int. J. Electrochem. Sci., 2015, 10, 9808–9823 CAS.
- S. Abd El Wanees, M. Abdallah, A. S. Al-Gorair, F. A. A. Tirkistani, S. Nooh and R. Assi, Kinetics and Thermodynamic Studies on the Anodic Passivation of Ni in H2SO4 Solutions by the Galvanostatic Polarization Method, Int. J. Electrochem. Sci., 2021, 16, 150969 CrossRef.
- M. Abdallah, K. A. Soliman, R. Alfattani, A. S. Al-Gorair, A. Fawzy and M. A. A. Ibrahim, Insight of corrosion mitigation performance of SABIC iron in 0.5 M HCl Solution by tryptophan and histidine: Experimental and computational approaches, Int. J. Hydrogen Energy, 2022, 47(2), 12782–12797 CrossRef CAS.
- A. Fawzy, I. A. Zaafarany, H. M. Ali and M. Abdallah, New Synthesized Amino Acids-based Surfactants as efficient inhibitors for corrosion of mild Steel in hydrochloric acid medium: Kinetics and thermodynamic approach, Int. J. Electrochem. Sci., 2018, 13, 4575–4600 CrossRef CAS.
- J. S. M. Anderson, J. Melin and P. W. Ayers, Conceptual Density-Functional Theory for General Chemical Reactions, Including Those That Are Neither Charge- nor Frontier-Orbital-Controlled. 1. Theory and Derivation of a General-Purpose Reactivity Indicator, J. Chem. Theory Comput., 2007, 3, 358–374 CrossRef CAS PubMed.
- M. Finsgar, A. Lesar, A. Kokalj and I. Milosev, A comparative electrochemical and quantum chemical calculation study of BTAH and BTAOH as copper corrosion inhibitors in near neutral chloride solution, Electrochim. Acta, 2008, 53(28), 8287–8297 CrossRef CAS.
- F. Shojaie and N. Mirzai-Baghini, Molecular dynamics and density functional theory study on the corrosion inhibition of austenitic stainless steel in hydrochloric acid by two pyrimidine compounds, Int. J. Ind. Chem., 2015, 6, 297–310 CrossRef CAS.
- E. E. Ebenso, D. A. Isabirye and N. O. Eddy, Adsorption and Quantum Chemical Studies on the Inhibition Potentials of Some Thiosemicarbazides for the Corrosion of Mild Steel in Acidic Medium, Int. J. Mol. Sci., 2010, 11, 2473–2498 CrossRef CAS PubMed.
|
This journal is © The Royal Society of Chemistry 2022 |