DOI:
10.1039/D2RA03562F
(Paper)
RSC Adv., 2022,
12, 28098-28103
Polyphosphoric acid-promoted one-pot synthesis and neuroprotective effects of flavanones against NMDA-induced injury in PC12 cells†
Received
9th June 2022
, Accepted 23rd September 2022
First published on 3rd October 2022
Abstract
We report herein an efficient polyphosphoric acid (PPA) promoted one-pot protocol for the synthesis of flavanone derivatives from 2-hydroxyacetophenones and benzaldehydes. A variety of flavanones were produced in moderate to excellent yields and evaluated for their neuroprotective effects against N-methyl-D-aspartate (NMDA)-induced excitotoxicity in PC12 cells. Derivatives bearing electron-donating groups exhibited better neuroprotective activity. Compound 3m demonstrated the best protective potency and reversed the intracellular calcium (Ca2+) influx caused by NMDA, suggesting that flavanones protected the PC12 cells against NMDA-induced neurotoxicity via inhibition of Ca2+ overload.
Introduction
Flavanone derivatives are found in numerous natural and synthetic products.1 They also are building blocks in the synthesis of biologically active compounds.2 Most flavanones display varied bioactivities such as anti-cancer,3 anti-inflammatory,4 anti-oxidant,5 and anti-microbial,6 to name a few. Therefore, the synthesis of flavanones has gained widespread attention. Several methods for construction of flavanones to make use of acid catalysts,7 bases,8 oxidants,9 transition-metal catalysts,10 and thermal, photochemical, and electrochemical transformations11 have been established. However, special starting materials such as phenyl alkenyl aryl ethers,10a 2-(benzo[d]thiazol-2-ylsulfonyl)-1-phenylethanones,8e and arynes12 are required for this approach. Recently, several one-pot procedures for the preparation of flavanones via aniline-mediated cyclization of 2-hydroxyacetophenones and benzaldehydes have been reported (Scheme 1a).13 Despite significant advances, the addition of aniline and longer reaction time are required. Therefore, it is still desirable to develop new efficient catalytic systems.
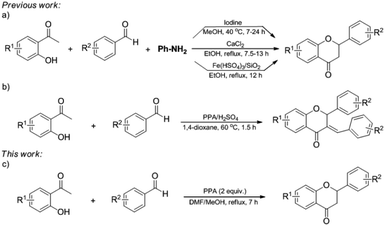 |
| Scheme 1 (a) One-pot approaches for the synthesis of flavanones. (b) One-pot synthesis of (E)-3-benzylideneflavanones. (c) This work. | |
The N-methyl-D-aspartate (NMDA) receptor belongs to ionotropic glutamate receptor that plays a role in brain development, brain function, learning and memory via Ca2+ influx.14 The excessive stimulation of the NMDA receptor leading to Ca2+ overload of neurons resulting neuronal death, which is responsible for a number of neurodegenerative diseases such as Alzheimer's disease, Parkinson's disease and depression.15 High concentration of NMDA can overactivation of NMDA receptor resulting the neuronal damage. PC12 cell line is one of the widely used neuronal cell lines in the evaluation of neuroprotective effects of NMDA receptor inhibitors against NMDA-induced injury.
Our group has developed an efficient and practical PPA/H2SO4 promoted one-pot synthesis of (E)-3-benzylideneflavanones (Scheme 1b),16 which inspired us to explore the one-pot synthesis of flavanones. Although the synthesis of flavanone via the cyclization of chalcone using PPA have been reported,7d we wish to describe another efficient one-pot procedure promoted by PPA for the synthesis of flavanone derivatives directly from readily available 2-hydroxyacetophenones and benzaldehydes (Scheme 1c). The neuroprotective activity of synthesized flavanones was evaluated using NMDA-induced PC12 cells injury model.
Results and discussion
Chemical synthesis
Our initial studies were carried out with the commercially available 2-hydroxyacetophenone 1a and benzaldehyde 2a as test substrates. A mixture of 1a (1 equiv.), 2a (4 equiv.) and PPA (4 equiv.) in N,N-dimethylformamide (DMF) and MeOH refluxed for 5 h under a nitrogen atmosphere to give the condensation product 3a in 50% yield (see the ESI, Table S1, entry 1†). Control experiment was performed to further improve the yield, the catalysts and additive such as P2O5, H3PO4 and H2SO4 were evaluated, PPA gave the best results (Table S1†). Then, we explored the effects of PPA amount, temperature and time. Increasing the amount of PPA to 6 equiv. had no obvious effect on the reaction (Table 1, entry 1). Delightly, we found that decreasing the amount of PPA further increased the yield (Table 1, entry 2). However, further decreasing the amount of PPA resulted in lower yield (Table 1, entry 3). Subsequently, we investigated the effect of reaction temperature and a decreased yield was observed upon decreasing or increasing the temperature to 100 or 200 °C (Table 1, entries 4 and 5). We then investigated the impact of the reaction time. Fortunately, the desirable compound 3a was obtained in 84% yield when the reaction time increased to 7 h (Table 1, entry 6). However, prolonging the time (9 h) had a slight reduction in yield (Table 1, entry 7). We then evaluated the reaction using microwave irradiation conditions, 25% of 3a was isolated (Table 1, entry 8). We further evaluated the effects of protonic, aprotic, dipolar aprotic and non-polar aprotic solvents. The results showed that DMF and MeOH were the best choice (Table 1, entry 6 vs. Table S2†). Finally, the optimized reaction conditions were determined as 1a (1 equiv.), 2a (4 equiv.) and PPA (2 equiv.) in DMF and MeOH refluxed for 7 h under a nitrogen atmosphere (Table 1, entry 6). With the optimized reaction conditions in hand, a gram scale reaction was carried out and provided the product 3a in 73% yield (Table 1, entry 9).
Table 1 PPA amount, temperature and time optimizationa
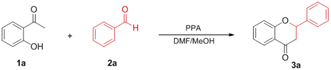
|
Entry |
PPA (equiv.) |
T (°C) |
Time (h) |
3ab (%) |
Reaction conditions: 1a (0.2 mmol), 2a (0.8 mmol), DMF (6.4 mL), MeOH (1.6 mL). Isolated yield. Reaction carried out in a pressure-resistant reaction bottle. Under microwave irradiation. Reaction conditions: 1a (8 mmol), 2a (32 mmol), DMF (16 mL), MeOH (4 mL). |
1 |
6 |
Reflux |
5 |
48 |
2 |
2 |
Reflux |
5 |
57 |
3 |
1 |
Reflux |
5 |
21 |
4 |
2 |
100 |
5 |
33 |
5c |
2 |
200 |
5 |
18 |
6 |
2 |
Reflux |
7 |
84 |
7 |
2 |
Reflux |
9 |
82 |
8d |
2 |
Reflux |
7 |
25 |
9e |
2 |
Reflux |
7 |
73 |
Under the optimized conditions, the substrate scope of this cyclization was examined by varying 2-hydroxyacetophenones 1 and benzaldehydes 2 (Table 2). As shown in Table 2, the reaction proceeded well with a variety of substrates to yield desired flavanone derivatives in moderate to excellent yields. The electronic properties of the substituents have an effect on the reaction yield. We found that electron-donating groups (Me, ethyl and OMe) on 2-hydroxyacetophenones 1 and/or benzaldehydes 2 provided higher yields (3g, 3h and 3k–3o). Hydroxyl-substituted benzaldehydes had limited influence on the reactivity (3i). However, electron-withdrawing groups, such as F, Cl, Br and CF3, gave lower yields (3b–3f and 3j). It is worthy to note that aliphatic aldehydes, such as cyclohexanecarboxaldehyde and propanal also underwent smooth transformation to afford the corresponding flavanones in moderate yields (3p and 3q).
Table 2 Evaluation of substrate scopea

|
Reaction conditions: 1 (0.2 mmol), 2 (0.8 mmol), PPA (0.4 mmol), DMF (6.4 mL), MeOH (1.6 mL). Reaction time (8 h). |
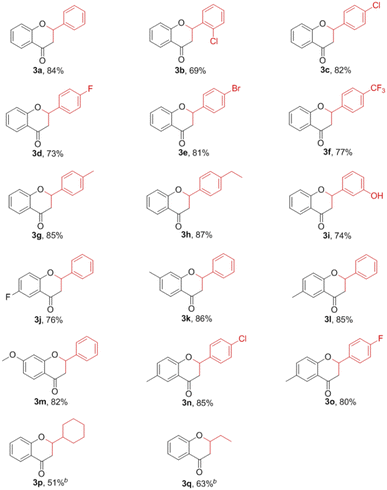 |
Thus, PPA is an effective catalyst for the one-pot synthesis of flavanones from 2-hydroxyacetophenones and benzaldehydes. PPA not only served as an acid catalyst but also widely used in dehydration condensation and cyclization reactions.17 As other acids, PPA promotes the enolization of ketone, and, perhaps more importantly, the dehydration and cyclization properties of it are responsible for the efficiency of this one-pot procedure.
The preliminary kinetic experiment of the formation of 3a was conducted under the standard condition. The yields were determined by 1H NMR with TTCE as internal standard. As depicted in Fig. 1, the conversion of 2-hydroxyacetophenone 1a and benzaldehyde 2a to flavanone 3a was occurred slowly in the course of 0.5 h heating and afterwards turned faster. In the late stage, a decreased conversion rate was observed.
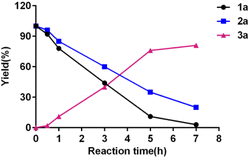 |
| Fig. 1 Kinetic of the formation of flavanone 3a. | |
A plausible mechanism for the one-pot synthesis of flavanone derivatives is depicted in Scheme 2. Firstly, intermediate 3 is formed from the enolization of 2-hydroxyacetophenone 1a. Then, the intermediate 3 coupled with benzaldehyde 2a followed by protonation to produce intermediate 4, which after subsequent dehydrative cyclisation promoted by PPA gives desired product 3a.
 |
| Scheme 2 Plausible mechanism. | |
Biochemistry
General information. Fetal bovine serum (FBS), Dulbecco's modified Eagle's medium (DMEM), penicillin and streptomycin were obtained from Gibco (Gibco, Paisley, UK). 3-(4,5-Dimethylthiazol-2-yl)-2,5-diphenyl-tetrazolium bromide (MTT), NMDA and Fura-2 acetoxymethyl ester (Fluo-2/AM) were purchased from Sigma-Aldrich (Saint Louis, MO, USA). MK-801 was from MCE (Shanghai, China).
Biological evaluation. Previous studies shown that flavonoid-rich plant or food extracts have exhibited improvements in cognition and learning,18 and that flavanone hesperetin has been observed to inhibit oxidative stress-induced neuronal apoptosis.19 Structurally, flavanones and most glycine antagonists are of a planar structure and flavanone 3a docked well to the glycine binding site of NMDA receptor (see ESI Fig. S1†). These inspired us to explore the neuroprotective effects of flavanones against NMDA-induced injury in PC12 cells (purchased from Institute of Cell Biology, Chinese Academy of Sciences, Shanghai, China) (Fig. 2).
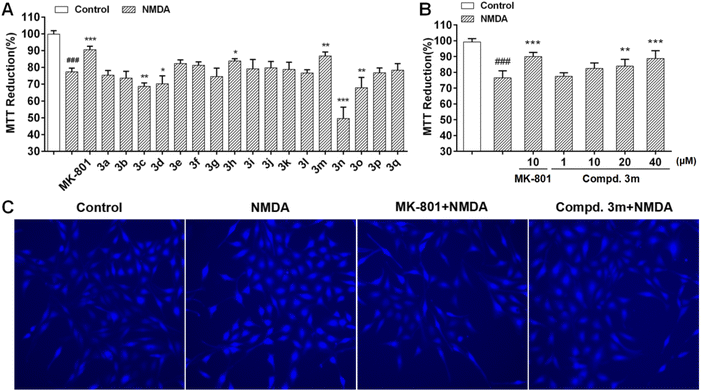 |
| Fig. 2 Protective and Ca2+ antagonistic effects of flavanones on NMDA-induced injury in PC12 cells. (A) Protective effects of MK-801 and flavanones at 20 μM. (B) Concentration-dependent protective effects of compound 3m. (C) Blue fluorescence shown the Ca2+ influx. Cytotoxicity was measured by MTT assay. Data are presented as mean ± SD (n = 3). ###p < 0.001 as compared to control group, *p < 0.05, **p < 0.01, ***p < 0.001 as compared to NMDA group. | |
NMDA injury was performed according to our previous reported protocol20 and reported in ESI.† The protective effects of the synthesized flavanones were evaluated via the MTT assay. Compounds with electron-donating group (ethyl or OMe) shown a significant increase in the cell viability as compared to NMDA treatment group, and which exhibited better protective activity than that bearing electron-withdrawing group (F, Cl or CF3) (Fig. 2A, 3h and 3m vs. 3b–3f and 3n, 3o).
Subsequently, concentration-dependent protective effects of the most active compound 3m on NMDA-induced damage was carried out (Fig. 2B). At 20 μM, there was a significant increase in the cell viability for compound 3m compared to NMDA treated group. It was found that pretreatment 40 μM compound 3m shown the similar neuroprotective potency to the NMDA receptor non-competitive antagonist MK-801 (10 μM). Then we evaluated the effect of compound 3m on NMDA-induced Ca2+ influx in PC12 cells (Fig. 2C). In the present study, the influx of Ca2+ was inhibited by MK-801 (10 μM) or compound 3m (40 μM), suggesting that the neuroprotective effects of compound 3m may via Ca2+ antagonism.
Molecular docking study
Molecular docking study between compound 3m and glycine binding site of NMDA receptor was conducted. Docking studies of (2R)-3m and (2S)-3m were conducted. The results indicated that (2R)-3m (green) and (2S)-3m (gray) fitted well in the active pocket of NMDA receptor and exhibited binding energy of −7.14 and −6.55 kcal mol−1 respectively (Fig. 3A). In the binding mode, (2R)-3m formed three hydrogen bonds with the amino acid residues ARG-131, THR-126 and SER-180 (Fig. 3B and D). It was observed that (2R)-3m being stabilized by Pi-stacking interaction with PHE-92 (Fig. 3B). The orientation and interactions network of (2S)-3m were little different from (2R)-3m, but the 2-phenyl part was overlapped (Fig. 3A). (2S)-3m was able to form the hydrogen bonding interaction with the residues SER-180 and ASP-224 (Fig. 3C and E). Additionally, the 2-phenyl moiety of (2R)-3m or (2S)-3m was embedded in the hydrophobic pocket formed by residues TRP-223, PHE-16 and PHE-250 (Fig. 3B and C).
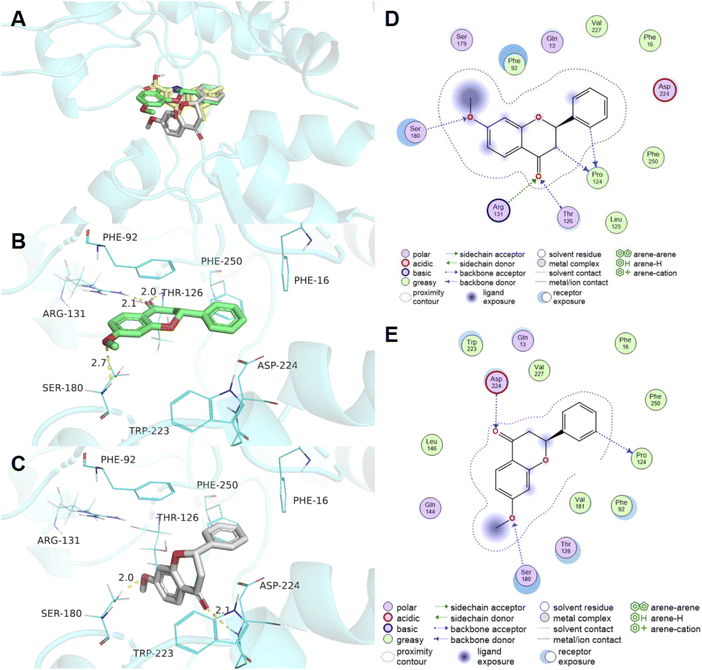 |
| Fig. 3 Docking of compound 3m with glycine binding site of NMDA receptor (PDB: 4NF4). (A) Alignment of (2R)-3m (green), (2S)-3m (gray) and co-crystallized ligand DCKA (yellow) in the active site. The interactions of (2R)-3m (B) and (2S)-3m (C) with the active site residues. Hydrogen bond interactions were shown in yellow dotted lines. 2D diagram of (2R)-3m (D) and (2S)-3m (E) interactions with the active site residues. | |
Conclusions
In conclusion, we have developed an efficient PPA-promoted one-pot approach for the preparation of flavanone derivatives from 2-hydroxyacetophenones and benzaldehydes. In addition, the neuroprotective effect of the synthesized flavanone derivatives against NMDA-induced injury in PC12 cells was evaluated. Derivatives with electron-donating groups shown a significant protective potency. Notably, 7-OMe substituted compound 3m exhibited good protective effects and could inhibit the excessive intracellular Ca2+ caused by NMDA.
Conflicts of interest
There are no conflicts to declare.
Acknowledgements
The authors are grateful for the help provided by the staff at Guizhou Vocational College of Agriculture and the Key Laboratory of Chemistry for Natural Products of Guizhou Province and the Chinese Academy of Sciences. The work here was supported by the National Natural Science Foundation of China (No. 81860609), the Project of Guizhou Science and Technology Platform and Talent Team (No. QKHZC[2021] General 424), the Project of Natural Science Research of Guizhou Vocational College of Agriculture (No. YJ202105) and the Project of Key Laboratory for Kharacteristics of Colleges and Universities of Guizhou Provincial Department of Education (No. QJHKYZ[2020]018).
Notes and references
- See for example:
(a) G. U. Siddikov, M. P. Yuldashev, S. F. Aripova, A. D. Vdovin, N. D. Abdullaev and E. K. Botirov, Chem. Nat. Compd., 2008, 44, 28 CrossRef CAS;
(b) Z. Zhou, P. Zhao, W. Huang and G. Yang, Adv. Synth. Catal., 2006, 348, 63 CrossRef CAS;
(c) A. Paredes, M. Alzuru, J. Mendez and M. Rodríguez-Ortega, Biol. Pharm. Bull., 2003, 26, 108 CrossRef CAS.
- W. D. Z. Li and B. C. Ma, Org. Lett., 2005, 7, 271 CrossRef CAS PubMed.
-
(a) A. Maiti, M. Cuendet, V. L. Croy, D. C. Endringer, J. M. Pezzuto and M. Cushman, J. Med. Chem., 2007, 50, 2799 CrossRef CAS PubMed;
(b) M. Cabrera, M. Simoens, G. Falchi, M. L. Lavaggi, O. E. Piro, E. E. Castellano and M. Gonzalez, Bioorg. Med. Chem., 2007, 15, 3356 CrossRef CAS PubMed.
-
(a) H. K. Hsieh, T. H. Lee, J. P. Wang, J. J. Wang and C. N. Lin, Pharm. Res., 1998, 15, 39 CrossRef CAS PubMed;
(b) D. Njamen, J. T. Mbafor, Z. T. Fomum, A. Kamanyi, J. C. Mbanya, M. C. Recio and J. L. Ríos, Planta Med., 2004, 70, 104 CrossRef CAS PubMed.
- H. A. Jung, D. M. Jeong, H. Y. Chung, H. A. Lim, J. Y. Kim, N. Y. Yoon and J. S. Choi, Biol. Pharm. Bull., 2008, 31, 908 CrossRef CAS.
- F. E. Ward, D. L. Garling, R. T. Buckler, D. M. Lawler and D. P. Cummings, J. Med. Chem., 1981, 24, 1073 CrossRef CAS.
-
(a) J. P. Monserrat, G. G. Chabot, L. Hamon, L. Quentin, D. Scherman, G. Jaouen and E. A. Hillard, Chem. Commun., 2010, 46, 145 RSC;
(b) D. Kumar, G. Patel, B. G. Mishra and R. S. Varma, Tetrahedron Lett., 2008, 49, 6974 CrossRef CAS;
(c) H. Kagawa, A. Shigematsu, S. Ohta and Y. Harigaya, Chem. Pharm. Bull., 2005, 53, 547 CrossRef CAS PubMed;
(d) L. Reichel and G. Proksch, Justus Liebigs Ann. Chem., 1971, 745, 59 CrossRef CAS;
(e) C. M. Brennan, I. Hunt, T. C. Jarvis, C. D. Johnson and P. D. McDonnell, Can. J. Chem., 1990, 68, 1780 CrossRef CAS;
(f) G. Wurm and D. Schnetzer, Arch. Pharm., 1992, 325, 717 CrossRef CAS;
(g) R. Chaturvedi, P. N. Patil and N. B. Mulchandani, Indian J. Chem., 1992, 31B, 340 CAS;
(h) G. Nabaei-Bidhendi and N. R. Bannerjee, J. Indian Chem. Soc., 1990, 67, 43 CAS.
-
(a) I. Akçok and A. Çağır, Bioorg. Chem., 2010, 38, 139 CrossRef;
(b) S. Chandrasekhar, K. Vijeender and K. V. Reddy, Tetrahedron Lett., 2005, 46, 6991 CrossRef CAS;
(c) L. Reichel and F. G. Weber, Die Pharmazie, 1975, 30, 195 CAS;
(d) D. D. Keane, K. G. Marathe, W. I. O'sullivan and S. Srivastava, J. Org. Chem., 1970, 35, 2286 CrossRef CAS;
(e) A. Kumar, S. Sharma, V. D. Tripathi and S. Srivastava, Tetrahedron, 2010, 66, 9445 CrossRef CAS.
- O. V. Sing, Org. Prep. Proced. Int., 1993, 25, 693 CrossRef.
-
(a) S. J. Joglekar and S. D. Samant, Tetrahedron Lett., 1988, 29, 241 CrossRef CAS;
(b) D. Kim, K. Ham and S. Hong, Org. Biomol. Chem., 2012, 10, 7305 RSC.
- See for example:
(a) Y. Maki, K. Shimada, M. Sako and K. Hirota, Tetrahedron, 1988, 44, 3187 CrossRef CAS;
(b) G. Pandey, G. Kumaraswamy and A. Krishna, Tetrahedron Lett., 1987, 28, 2649 CrossRef CAS;
(c) T. M. Harris and R. L. Carney, J. Am. Chem. Soc., 1967, 89, 6734 CrossRef CAS.
- A. V. Dubrovskiy and R. C. Larock, Org. Lett., 2010, 12, 3117 CrossRef CAS PubMed.
-
(a) V. Kavala, C. Lin, C. W. Kuo, H. Fang and C. F. Yao, Tetrahedron, 2012, 68, 1321 CrossRef CAS;
(b) H. Eshghi, M. Rahimizadeh and S. M. Mousavi, Nat. Prod. Res., 2014, 28, 438 CrossRef CAS PubMed;
(c) D. D. Kondhare, G. Gyananath, Y. Tamboli, S. S. Kumbhar, P. B. Choudhari, M. S. Bhatia and P. K. Zubaidha, Med. Chem. Res., 2017, 26, 987 CrossRef CAS.
-
(a) L. Zhang, Y. Zhao, J. Wang, D. Yang, C. Zhao, C. Wang, C. Ma and M. Cheng, Eur. J. Med. Chem., 2018, 151, 27 CrossRef CAS PubMed;
(b) P. Barai, N. Raval, S. Acharya and N. Acharya, Biomed. Pharmacother., 2018, 108, 374 CrossRef CAS PubMed.
-
(a) D. Bano and P. Nicotera, Stroke, 2007, 38, 674 CrossRef CAS PubMed;
(b) R. Guerrini, E. Marzola, C. Trapella, S. Molinari, M. C. Cerlesi, D. Malfacini and S. Salvadori, Bioorg. Med. Chem., 2014, 22, 3703 CrossRef CAS.
- L. Yang, E. Wang, Y. Fan, J. Yang, Z. Luo, Y. Wang, M. Peng, T. Deng and X. Yang, Tetrahedron Lett., 2020, 61, 151180 CrossRef CAS.
- See for example:
(a) H. R. Snyder and F. X. Werber, J. Am. Chem. Soc., 1950, 72, 2962 CrossRef CAS;
(b) C. D. Hurd and S. Hayao, J. Am. Chem. Soc., 1954, 76, 5065 CrossRef CAS;
(c) C. R. Hanser and J. G. Murray, J. Am. Chem. Soc., 1955, 77, 3858 CrossRef;
(d) A. V. Aksenov, D. S. Ovcharov, N. A. Aksenov, D. A. Aksenov, O. N. Nadein and M. Rubin, RSC Adv., 2017, 7, 29927 RSC;
(e) M. Chang, T. Lee and M. Wu, Org. Lett., 2012, 14, 2198 CrossRef CAS PubMed;
(f) A. V. Aksenov, V. Khamraev, N. A. Aksenov, N. K. Kirilov, D. A. Domenyuk, V. A. Zelensky and M. Rubin, RSC Adv., 2019, 9, 6636 RSC.
-
(a) R. L. Galli, B. Shukitt-Hale, K. A. Youdim and J. A. Joseph, Ann. N. Y. Acad. Sci., 2002, 959, 128 CrossRef CAS PubMed;
(b) K. A. Youdim and J. A. Joseph, Free Radical Biol. Med., 2001, 30, 583 CrossRef CAS;
(c) K. Unno, F. Takabayashi, T. Kishido and N. Oku, Exp. Gerontol., 2004, 39, 1027 CrossRef CAS PubMed;
(d) S. Kuriyama, A. Hozawa, K. Ohmori, T. Shimazu, T. Matsui, S. Ebihara and I. Tsuji, Am. J. Clin. Nutr., 2006, 83, 355 CrossRef CAS PubMed;
(e) A. M. Haque, M. Hashimoto, M. Katakura, Y. Tanabe, Y. Hara and O. Shido, J. Nutr., 2006, 136, 1043 CrossRef CAS PubMed.
- D. Vauzour, K. Vafeiadou, C. Rice-Evans, R. J. Williams and J. P. Spencer, J. Neurochem., 2007, 103, 1355 CrossRef CAS PubMed.
- L. Yang, Q. Yang, E. Wang, J. Yang, Q. Li, J. Cao, L. Wang, X. Liao, Y. Yang and X. Yang, Bioorg. Med. Chem., 2022, 59, 116675 CrossRef CAS.
|
This journal is © The Royal Society of Chemistry 2022 |
Click here to see how this site uses Cookies. View our privacy policy here.