DOI:
10.1039/D2RA03323B
(Paper)
RSC Adv., 2022,
12, 24232-24236
Cu(I)-catalyzed cross-coupling of primary amines with 2,2′-dibromo-1,1′-biphenyl for the synthesis of polysubstituted carbazole†
Received
27th May 2022
, Accepted 12th August 2022
First published on 25th August 2022
Abstract
A Cu(I)-catalyzed cross-coupling of primary amines with 2,2′-dibromo-1,1′-biphenyl for the synthesis of polysubstituted carbazole has been achieved. This protocol provides an efficient strategy for the synthesis of carbazole using cheap copper catalysts with diamine ligand, and it provides convenient access to a series of carbazole derivatives in moderate yields.
1. Introduction
Carbazole is an important organic nitrogen-containing heterocyclic skeleton, which is extensively applied as a building block in natural products,1 dyes2 and pharmaceuticals.3 As shown in Fig. 1, some representative bioactive molecules possessing carbazole moieties are listed. For example, murrayafoline A has strong antifungal and antitumor properties.4 Celiptium, as a potent DNA intercalator and topoisomerase II inhibitor, has been used as a medicine for the treatment of breast cancer.5 Clausenaquinone A showed an effective inhibitory activity on platelet aggregation as well as cytotoxicity in RPMI-7951, HCT-8 and TE671 tumor cells.6 Calothrixin A and calothrixin B, carbazole quinone alkaloids, are novel DNA topoisomerase inhibitors, which have excellent effects on anti-tumor cells.7 Curaxin 137 (CBLC137) is an effective inducer of apoptosis. It is toxic for proliferating various tumor cells and pancreatic cancer stem cells.8 Carbazole and its derivatives are also used in the synthesis of photoelectric functional materials.9 For these reasons, the synthesis of carbazole using simple substrates and methodologies is still an active research field. Recently, some novel organic synthesis strategies for the construction of carbazoles have been reported.10
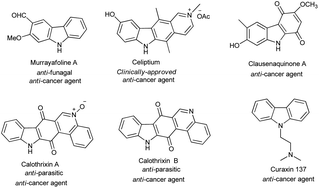 |
| Fig. 1 Biologically active carbazole derivatives. | |
The traditional approaches for the construction of the carbazole rings were to use the Buchwald–Hartwig C–N cross-coupling reaction.11 In 2003, the Nozaki group firstly presented the synthesis of carbazole with double N–H arylation of primary amides via the [Pa2(dba)3]/tBu3P/tBuONa system.12 Later, Chida et al. revealed the N-arylation protocol via the [Pa2(dba)3]/dialkylphosphinobiaryls/tBuONa system, and further applied it to the synthesis of murrayafoline A.13 In 2010, Zhou and co-workers developed the [Pa2(dba)3]/(tBu)2P
N–P(iBuNCH2CH2)3N/tBuONa system for the synthesis of carbazole under mild conditions.14 However, the need for expensive Pd catalysts and the frequent use of expensive phosphine ligands are the drawbacks of these methods. Recently, copper catalysts have become popular and have been widely used to synthesize carbazole.15 In 2010, Liao and coworkers developed a convenient Cu-catalyzed double C–N bond-forming reaction using 2,2-diiodobiphenyl and p-methylaniline for the synthesis of carbazole.15b However, only one example was reported, and the cheaper 2,2-dibromobiphenyl was not used in this reaction. Although Do and coworkers reported dibromide substrates using CuI/proline system for the synthesis of carbazole,15c the reaction requires higher temperatures and a strong base. Therefore, it is necessary to develop a new system for the synthesis of carbazole to use the cheaper 2,2-dibromobiphenyl as substrates in the copper-catalyzed C–N coupling reaction under mild conditions. Compared to palladium, copper catalyst has the advantages of low price and low toxicity, and it can be suitable for industrial-scale production. The Ullmann-type coupling reaction between aryl halides and nitrogen-based nucleophiles has also been used for the synthesis of carbazoles.16,17 However, these methods usually suffer from several disadvantages, such as the need to use stoichiometric copper catalysts and the high reaction temperatures (normally above 150 °C).17 In order to solve these problems, special ligands need to be designed to promote these coupling reactions. In this scenario, it is highly desirable to develop a simple, efficient and practical approach to the synthesis of carbazole derivatives using cheap Cu catalysts and ligands under mild conditions.
The efficient construction of functional nitrogen-containing heterocycles in a simple way is an important task in synthetic chemistry and some new synthetic strategies have been reported continuously.18 As a continuation of our long-term interest in the synthesis of heterocyclic compounds,19 in this study, we present a simple methodology for the synthesis of N-arylcarbazoles using substituted 2,2′-dihalobiphenyl and aromatic primary amine via the copper-catalyzed cross-coupling reaction.
2. Results and discussion
In our initial investigation, 2,2′-dibromo-1,1′-biphenyl 1a and aniline 2a were chosen as the model substrates in the presence of CuI (20 mol%), DMEDA (N,N′-dimethylethylenediamines) (20 mol%) and K2CO3 (3 equiv.) at 110 °C in 3 mL DMF for 72 h (Table 1, entry 1). To our disappointment, the required product 3a was not obtained. Other solvents such as THF, DMSO and toluene were screened and to our delight, the desired product 3a was obtained, in toluene with small polarity, in 64% yield (Table 1, entries 2–4). Changing base to Cs2CO3, tBuOK, DABCO, DBU and Et3N failed to improve the yield of the product 3a (Table 1, entries 5–9). Other Cu catalysts such as CuCl, CuBr, Cu(OTf)2 and Cu powder were also investigated for this cross-coupling reaction but no better results were observed (Table 1, entries 10–13). The effects of ligands in the cross-coupling reaction were then evaluated. The results showed that double N-chelated ligands played a significant role in the formation of carbazole; other ligands such as TMEDA (N,N,N′,N′-tetramethylethylenediamine), EDA (ethylenediamines) and 4,4′-dimethyl-2,2′-dipyridine only obtained lower yields, and PPh3 gave no desired product (Table 1, entries 4 and 14–17). On decreasing the temperature to 80 °C, only 26% yield was isolated (Table 1, entry 18). By raising the reaction temperature to 140 °C, the N-phenylcarbazole 3a was obtained in 60% yield (Table 1, entry 19). On decreasing 2a to 2 equiv., the lower yield was observed (Table 1, entry 20). When the catalyst loading was decreased to 10 mol%, the yield of 3a was decreased to 45% (Table 1, entry 21). Thus, the optimized reaction conditions chosen for all subsequent coupling reactions are as follows: 0.2 mmol of 2,2′-dibromo-1,1′-biphenyl, 0.6 mmol aniline, and 0.04 mmol CuI, 0.04 mmol DMEDA and 3 equiv. K2CO3 in 3 mL toluene, stirred at 110 °C, under the Ar atmosphere in a sealed tube for 72 h.
Table 1 Optimization of the reaction conditionsa
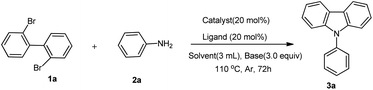
|
Entry |
Catalyst |
Solvent |
Base |
Ligand |
Temperature (°C) |
Yield 3ab (%) |
Reaction conditions: 1a (1 equiv., 0.2 mmol), 2a (3 equiv., 0.6 mmol), catalyst (20 mol% without other declaration), ligand (20 mol%), base (3 equiv.), solvent (3.0 mL), the reaction was carried out at 110 °C, under an Ar atmosphere in a sealed tube for 72 h. Isolated yield. 0.4 mmol 2a (2 equiv.) was used. |
1 |
CuI |
DMF |
K2CO3 |
DMEDA |
110 |
0 |
2 |
CuI |
DMSO |
K2CO3 |
DMEDA |
110 |
0 |
3 |
CuI |
THF |
K2CO3 |
DMEDA |
110 |
0 |
4 |
CuI |
Toluene |
K2CO3 |
DMEDA |
110 |
64 |
5 |
CuI |
Toluene |
CsCO3 |
DMEDA |
110 |
13 |
6 |
CuI |
Toluene |
tBuOK |
DMEDA |
110 |
22 |
7 |
CuI |
Toluene |
DABCO |
DMEDA |
110 |
0 |
8 |
CuI |
Toluene |
DBU |
DMEDA |
110 |
0 |
9 |
CuI |
Toluene |
Et3N |
DMEDA |
110 |
0 |
10 |
CuCI |
Toluene |
K2CO3 |
DMEDA |
110 |
45 |
11 |
CuBr |
Toluene |
K2CO3 |
DMEDA |
110 |
24 |
12 |
Cu(OTf)2 |
Toluene |
K2CO3 |
DMEDA |
110 |
47 |
13 |
Cu |
Toluene |
K2CO3 |
DMEDA |
110 |
48 |
14 |
CuI |
Toluene |
K2CO3 |
TMEDA |
110 |
19 |
15 |
CuI |
Toluene |
K2CO3 |
EDA |
110 |
34 |
16 |
CuI |
Toluene |
K2CO3 |
Ph3P |
110 |
0 |
17 |
CuI |
Toluene |
CsCO3 |
4,4′-Dimethyl-2,2′-dipyridine |
110 |
21 |
18 |
CuI |
Toluene |
K2CO3 |
DMEDA |
80 |
26 |
19 |
CuI |
Toluene |
K2CO3 |
DMEDA |
140 |
60 |
20 |
CuI |
Toluene |
K2CO3 |
DMEDA |
110 |
46c |
21 |
CuI (10%) |
Toluene |
K2CO3 |
DMEDA |
110 |
45 |
With the optimized reaction conditions, the scope of this coupling reaction was examined and the results are summarized in Scheme 1. Firstly, a series of functional groups were tolerated in different positions of arylamine in this reaction, such as –CH3, –OCH3, –Br and –Cl substituents on arylamine. When introducing the electron-donating groups, such as –CH3 and –OCH3 on the arylamine, the desired carbazoles were obtained in 50–70% yields (Scheme 1, 3b–3f). When bearing electron-withdrawing groups on arylamine, it slightly hindered the reaction (Scheme 1, 3g–3j). It should be noted that relatively lower yields were observed when ortho-substituted aromatic amines were used, and this might be due to steric hindrance (Scheme 1, 3b, 3e and 3j). Naphthylamine was also compatible with this coupling reaction, and the desired product 3k was obtained in 53% yield (Scheme 1, 3k). We continued to elucidate the scope of this reaction by replacing the hydrogen atom at the R1 position with –CH3 and –OCH3 groups (Scheme 1, 3l–3w). The results showed that strong electron-donating substituents on 2,2′-dibromo-1,1′-biphenyl gave lower yields (Scheme 1, 3s–3w). To further confirm the applicability of this reaction, a gram-scale reaction of 1a (1.56 g, 5.0 mmol) with 2a (1.40 g, 15 mmol) was carried out under the standard conditions, providing the corresponding product 3a in 54% yield (0.66 g).
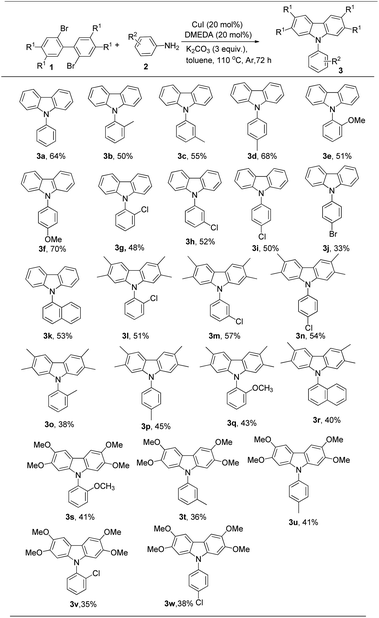 |
| Scheme 1 The scope of the coupling reaction. | |
To further expand the scope of this reaction, butylamine and benzylamine, which were also compatible with this reaction, were investigated and the corresponding carbazoles were obtained in 63% and 75% yields, respectively. In the presence of oxygen, N-benzylcarbazole reacted with tBuOK in DMSO to quickly remove benzyl groups to obtain carbazole in 90% yield (Scheme 2). This result is consistent with that reported in the literature.20
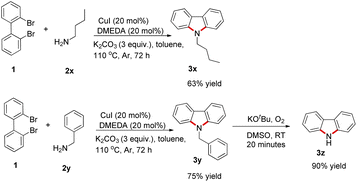 |
| Scheme 2 Cu(I)-catalyzed cross-coupling of aliphatic amines with 2,2′-dibromo-1,1′-biphenyl for the synthesis of substituted carbazole. | |
The possible mechanism of this copper-catalyzed cross-coupling reaction for the synthesis of carbazole is proposed in Scheme 3. Firstly, DMEDA combined with CuI to form A, which subsequently reacted with 2,2′-dibromobiphenyl via oxidative addition to form intermediate B. Then, the aniline, as a nucleophile, attacked B to produce C, in which HBr was also lost in the presence of base. The intermediate D was formed by reductive elimination. Subsequently, a similar oxidative addition occurred to give intermediate E, and then another HBr was lost with base. Finally, the desired product 3a was obtained with another reductive elimination, and Cu catalyst A was regenerated.
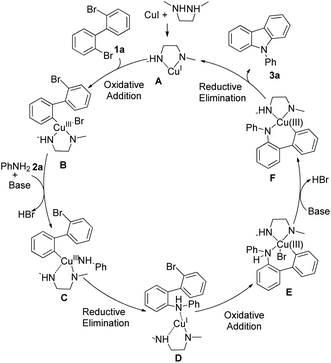 |
| Scheme 3 A plausible mechanism for the formation of 3a. | |
3. Conclusions
In summary, we have developed a one-pot method, via Cu-catalyzed coupling of primary amines with 2,2′-dibromo-1,1′-biphenyl, for the synthesis of polysubstituted carbazole. This reaction provides a simple and facile route to the straightforward synthesis of polysubstituted carbazole in moderate yields. Further synthetic applications are ongoing in our laboratory.
Author contributions
The design of this protocol was carried out by Y.-N. Niu and G.-Q. Li. The manuscript was written through contributions of Y.-N. Niu. The empirical data were acquired by Y.-N. Niu and Y. Qiao. K.-Y. Wang and B.-X. Sha participated in the modification of the manuscript. All authors have given approval to the final version of the manuscript.
Conflicts of interest
There are no conflicts to declare.
Acknowledgements
The authors gratefully acknowledge the financial support of this work by National Natural Science Foundation of China (22171108) and Natural Science Research Projects in Huai'an (HAB202065).
References
-
(a) A. W. Schmidt, K. R. Reddy and H. J. Knölker, Chem. Rev., 2012, 112, 3193 CrossRef CAS PubMed;
(b) C. M. Miller and F. O. McCarthy, RSC Adv., 2012, 2, 8883 RSC.
- J. Yin, Y. Ma, G. Li, M. Peng and W. Lin, Coord. Chem. Rev., 2020, 412, 213257 CrossRef CAS.
-
(a) S. Issa, A. Prandina, N. Bedel, P. Rongved, S. Yous, M. Le Borgne and Z. Bouaziz, J. Enzyme Inhib. Med. Chem., 2019, 34, 1321 CrossRef CAS;
(b) D. Zhu, M. Chen, M. Li, B. Luo, Y. Zhao, P. Huang, F. Xue, S. Rapposelli, R. Pi and S. Wen, Eur. J. Med. Chem., 2013, 68, 81 CrossRef CAS PubMed;
(c) A. D. Favia, D. Habrant, R. Scarpelli, M. Migliore, C. Albani, S. M. Bertozzi, M. Dionisi, G. Tarozzo, D. Piomelli, A. Cavalli and M. De Vivo, J. Med. Chem., 2012, 55, 8807 CrossRef CAS PubMed;
(d) C. Alayrac, D. Schollmeyer and B. Witulski, Chem. Commun., 2009, 1464 RSC.
-
(a) C. Börger, O. Kataeva and H. J. Knölker, Org. Biomol. Chem., 2012, 10, 7269 RSC;
(b) V. Humne, Y. Dangat, K. Vanka and P. Lokhande, Org. Biomol. Chem., 2014, 12, 4832 RSC.
-
(a) N. C. Garbett and D. E. Graves, Curr. Med. Chem.: Anti-Infect. Agents, 2004, 4, 149 CrossRef CAS PubMed;
(b) C. M. Miller and F. O. McCarthy, RSC Adv., 2012, 2, 8883 RSC;
(c) C. M. Miller, E. C. O'Sullivan, K. J. Devine and F. O. McCarthy, Org. Biomol. Chem., 2012, 10, 7912 RSC.
- T. S. Wu, S. C. Huang, P. L. Wu and K. H. Lee, Bioorg. Med. Chem. Lett., 1994, 4, 23958 Search PubMed.
-
(a) S. Lee, K.-H. Kim and C.-H. Cheon, Org. Lett., 2017, 19, 2785 CrossRef CAS PubMed;
(b) J. Guo, I. N. C. Kiran, J. S. Gao, R. S. Reddy and Y. He, Tetrahedron Lett., 2016, 57, 3481 CrossRef CAS;
(c) S. A. Kaliyaperumal, S. Banerjee and U. K. S. Kumar, Org. Biomol. Chem., 2014, 12, 6105–6113 RSC;
(d) N. Ramkumar and R. Nagarajan, J. Org. Chem., 2013, 78, 2802 CrossRef CAS PubMed.
- S. M. Thomas, A. Purmal, M. Pollastri and K. Mensa-Wilmot, Sci. Rep., 2016, 6, 32083 CrossRef CAS PubMed.
-
(a) B. Xu, E. Sheibani, P. Liu, J. Zhang, H. Tian, N. Vlachopoulos, G. Boschloo, L. Kloo, A. Hagfeldt and L. Sun, Adv. Mater., 2014, 26, 6629 CrossRef CAS PubMed;
(b) S. Kumar and Y.-T. Tao, J. Org. Chem., 2015, 80, 5066 CrossRef CAS PubMed.
-
(a) K. Takamatsu, K. Hirano, T. Satoh and M. Miura, Org. Lett., 2014, 16, 2892 CrossRef CAS PubMed;
(b) B. J. Stokes, B. Jovanović, H. Dong, K. J. Richert, R. D. Riell and T. G. Driver, J. Org. Chem., 2009, 74, 3225 CrossRef CAS;
(c) T. L. Guo, Q. B. Jiang, F. Huang, J. P. Chen and Z. K. Yu, Org. Chem. Front., 2014, 1, 707 RSC;
(d) T. Gensch, M. Rönnefahrt, R. Czerwonka, A. Jäger, O. Kataeva, I. Bauer and H.-J. Knölker, Chem.–Eur. J., 2012, 18, 770 CrossRef CAS PubMed;
(e) S. Maiti, T. K. Achar and P. Mal, Org. Lett., 2017, 19, 2006 CrossRef CAS PubMed.
-
(a) D. S. Surry and S. L. Buchwald, Chem. Sci., 2011, 2, 27 RSC;
(b) J. F. Hartwig, Acc. Chem. Res., 2008, 41, 1534 CrossRef CAS PubMed;
(c) D. S. Surry and S. L. Buchwald, Angew. Chem., Int. Ed., 2008, 47, 6338 CrossRef CAS PubMed.
-
(a) A. Kuwahara, K. Nakano and K. Nozaki, J. Org. Chem., 2005, 70, 413 CrossRef CAS PubMed;
(b) K. Nozaki, K. Takahashi, K. Nakano, T. Hiyama, H. Tang, M. Fujiki, S. Yamaguchi and K. Tamao, Angew. Chem., Int. Ed., 2003, 42, 2051 CrossRef CAS PubMed.
- T. Kitawaki, Y. Hayashi, A. Ueno and N. Chida, Tetrahedron, 2006, 62, 6792 CrossRef CAS.
- Y. Zhou and J. Verkade, Adv. Synth. Catal., 2010, 352, 616 CrossRef CAS.
-
(a) M. J. James, R. E. Clubley, K. Y. Palate, T. J. Procter, A. C. Wyton, P. O'Brien, R. J. K. Taylor and W. P. Unsworth, Org. Lett., 2015, 17, 4372 CrossRef CAS PubMed;
(b) Q. Liao, L. Zhang, F. Wang, S. Li and C. Xi, Eur. J. Org. Chem., 2010, 5426 CrossRef CAS;
(c) H. N. Do, N. M. Quan, B. V. Phuc, D. V. Tinh, N. Q. Tien, T. T. T Nga, V. T Nguyen, T. Q. Huang, T. T. Dang and P. Langer, Synlett, 2021, 32, 611 CrossRef CAS.
-
(a) S. V. Ley and A. W. Thomas, Angew. Chem., Int. Ed., 2003, 42, 5400 CrossRef CAS PubMed;
(b) F. Monnier and M. Taillefer, Angew. Chem., Int. Ed., 2009, 48, 6954 CrossRef CAS PubMed;
(c) G. Evano, N. Blanchard and M. Toumi, Chem. Rev., 2008, 108, 3054 CrossRef CAS;
(d) D. Zhu, Q. Liu, B. Luo, M. Chen, R. Pi, P. Huang and S. Wen, Adv. Synth. Catal., 2013, 355, 2172 CrossRef CAS.
-
(a) F. Bellina, C. Calandri, S. Cauteruccio and R. Ross, Eur. J. Org. Chem., 2007, 2147 CrossRef CAS;
(b) J. K. Kwon, J. H. Cho, Y.-S. Ryu, S. H. Oh and E. K. Yum, Tetrahedron, 2011, 67, 4820 CrossRef CAS.
- For selected papers:
(a) Z.-L. Wu, J.-Y. Chen, X.-Z. Tian, W.-T. Ouyang, Z.-T. Zhang and W.-M. He, Chin. Chem. Lett., 2022, 33, 1501 CrossRef CAS;
(b) Y. Wu, J.-Y. Chen, J. Ning, X. Jiang, J. Deng, Y. Deng, R. Xu and W.-M. He, Green Chem., 2021, 23, 3950 RSC;
(c) J.-Y. Chen, H.-Y. Wu, Q.-W. Gui, S.-S. Yan, J. Deng, Y.-W. Lin, Z. Cao and W.-M. He, Chin. J. Catal., 2021, 42, 1445 CrossRef CAS;
(d) Q.-W. Gui, F. Teng, Z.-C. Li, Z.-Y. Xiong, X.-F. Jin, Y.-W. Lin, Z. Cao and W.-M. He, Chin. Chem. Lett., 2021, 32, 1907 CrossRef CAS;
(e) Y. Wan, Q. Liu, H. Wu, Z. Zhang and G. Zhang, Org. Chem. Front., 2022, 9, 1634 RSC;
(f) Y. Shan, L. Su, Z. Zhao and D. Chen, Adv. Synth. Catal., 2021, 363, 906 CrossRef CAS;
(g) L. Tang, Y. Ouyang, K. Sun and B. Yu, RSC Adv., 2022, 12, 19736 RSC.
-
(a) X.-F. Xia and Y.-N. Niu, Org. Biomol. Chem., 2022, 20, 282 RSC;
(b) Q. Huang, M. Zhao, Y. Yang, Y.-N. Niu and X.-F. Xia, Org. Chem. Front., 2021, 8, 5988 RSC;
(c) Y.-N. Niu, X.-F. Xia and Y. Yuan, Synlett, 2018, 29, 617 CrossRef CAS;
(d) X.-F. Xia, L.-L. Zhang, X.-R. Song, Y.-N. Niu, X.-Y. Liu and Y.-M. Liang, Chem. Commun., 2013, 49, 1410 RSC.
- A. A. Haddach, A. Kelleman and M. V. Deaton-Rewolinski, Tetrahedron Lett., 2002, 43, 399 CrossRef CAS.
|
This journal is © The Royal Society of Chemistry 2022 |
Click here to see how this site uses Cookies. View our privacy policy here.