DOI:
10.1039/D2RA03036E
(Paper)
RSC Adv., 2022,
12, 23083-23090
Molecular evidence for improved tribological performances of MoDTC induced by methylene-bis(dithiocarbamates) in engine lubricants
Received
13th May 2022
, Accepted 31st July 2022
First published on 16th August 2022
Abstract
During engine tests, it has been observed that the combined use of molybdenum dithiocarbamates (MoDTC) and methylene-bis(dithiocarbamates) (MBDTC) in formulated engine oils resulted in better fuel efficiency, keeping the friction coefficient stable at low values for a longer period of time as compared to the same oil devoid of MBDTC. Therefore, the interactions between MBDTC and MoDTC have been investigated at the molecular level. The qualitative and quantitative evolution of MoDTC in two engine oils similarly formulated, but with and without MBDTC, were compared during engine tests using a specifically developed high performance liquid chromatography-mass spectrometry (HPLC-MS) analytical method. Parallel to the molecular study, the evolution of the friction coefficients of both lubricants as well as the evolution of the fuel consumption of the engine were determined. The combined use of MoDTC and MBDTC was shown to exhibit better fuel efficiency and to maintain a relatively low friction coefficient for longer periods of time as compared to the oil devoid of MBDTC. It could be determined that the enhanced performances observed were presumably related to an extension of the lifetime of MoDTC in the engine oil containing MBDTC. Since the MoDTC remaining at the end of the engine test in oil containing MBDTC exclusively bear ligands corresponding to the dithiocarbamate moieties of MBDTC, it can be concluded that the prolonged existence of MoDTC was due to the progressive replacement of the degraded dithiocarbamate ligands on MoDTC educts by those released from MBDTC during engine functioning. As a result, the concentrations of MoDTC could be maintained at a useful level for a longer period in the engine oil containing MBDTC, leading to better fuel consumption performances.
1. Introduction
The recent introduction of the new GF-6 lubricant specification by ILSAC (International Lubricant Standardization and Approval Committee) in 2020 has called for better fuel economy and reduction of smoke emissions. The addition of several new tests reflects higher standard and performance requirements for GF-6 oils as compared to GF-5 oils. To date, several technologies have been adopted to reduce fuel consumption and air pollutants, including hybridization of automotive powertrains,1–3 engine downsizing and turbocharging,4–6 gasoline direct injection,7,8 combustion optimization,9 improving aerodynamics,10,11 vehicle weight reduction12,13 etc. Another important approach to fulfill this new specification is to minimize the parasitic energy losses associated with friction in engines using appropriately designed formulated engine lubricants.14
Molybdenum-based complexes such as molybdenum dithiocarbamates (MoDTC, Fig. 1) are effective friction modifiers15–18 that have been shown to reduce friction coefficients to relatively low values under boundary lubrication conditions by generating lamellar structures of MoS2 layers at the tribocontact.19–23 Their effectiveness to reduce friction and to improve fuel efficiency has been investigated using either engine tests or bench friction tests in previous studies.21,24,25 However, it has been shown that MoDTC are unstable during oil ageing and undergo thermo-oxidative degradation during engine operation to form undesirable Mo-species such as molybdenum oxides (MoOx) and other highly oxidized compounds.19,26,27 Moreover, the accumulation of oxidized products can also impede the formation of a MoS2 tribofilm at the rubbing surfaces and cause a drop in friction reduction performance of engine oils.28
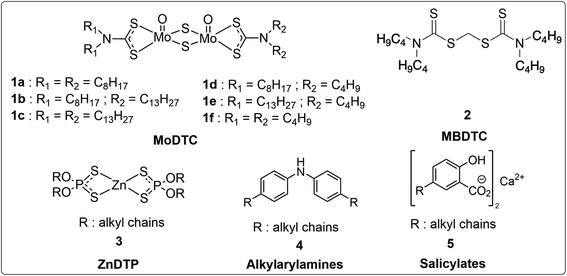 |
| Fig. 1 Chemical structures of the compounds mentioned in the text. | |
Nevertheless, it has been observed that the addition of antioxidants improves significantly the fuel efficiency of lubricant oils containing MoDTC.29,30 Other studies suggest that the combined use of MoDTC with phenolic and amine antioxidants exhibits excellent synergism that provides good retention of friction reducing capabilities of engine oils.31 Sulfur-containing peroxide decomposers and radical inhibitor-type antioxidants such as zinc dithiophosphates (ZnDTP; Fig. 1) are also believed to increase the durability of friction reducing properties of MoDTC and to reduce the decomposition rate of MoDTC under thermo-oxidative conditions.28,32 Thus, it clearly appears that the presence of effective antioxidants may contribute to delay the consumption rate of MoDTC caused by thermo-oxidative degradation.
In this context, the present study is dedicated to the investigation of the impact of MBDTC 2 (Fig. 1) as a lubricant additive on the service life of MoDTC in formulated lubricants. For this purpose, comparative engine tests were performed using the same formulated engine oils containing MoDTC without and with the addition of MBDTC (referred to as “oil A” and “oil AMBDTC”, respectively). Oil performances were compared in terms of tribological properties, duration of the friction reducing capacity of the lubricants, evolution of the fuel consumption of engines and evolution of the concentration of MoDTC with time. The qualitative and quantitative evolution of the MoDTC was monitored using a specifically developed high performance liquid chromatography-mass spectrometry (HPLC-MS) analytical method.33
2. Experimental section
2.1. Materials (formulated lubricants and additives)
The chemical structures of the compounds employed and discussed in this study are presented in Fig. 1. The formulated engine oils SAE 30 (oils A and AMBDTC) used in this work were provided by TotalEnergies Marketing Services. Oils A and AMBDTC have essentially the same composition and the additive package comprises, notably, MoDTC (Sakuralube 525; 1 wt%) 1a–1c as friction modifiers, secondary ZnDTP 3 (1 wt%) as anti-wear additive, alkylarylamines 4 as antioxidants and salicylates 5 as detergents (Fig. 1). These two oils only differ by the presence of MBDTC 2 (Vanlube 7723, 2 wt%) in the case of oil AMBDTC. The MoDTC complexes 1a–1c (Sakuralube 525) in the formulated engine oils were purchased from Adeka while MBDTC 2 (Vanlube 7723) was provided by Vanderbilt Chemicals.
2.2. Engine tests
Engine tests have been carried out according to a modified ASTM D8114 procedure for fuel economy retention measurement as specified in the ILSAC-GF-6A – test sequence VIE. Oils A and AMBDTC were separately introduced into the engine and aged at temperatures close to 115 °C for 143 h. Meanwhile, tribological friction coefficients and fuel economy were measured along the lubricant ageing periods. Tribological friction coefficients were determined using a ball on three flats setup (see section 2.3). On the other hand, fuel consumption was measured under the engine test conditions and compared to those measured using the reference oil SAE 20W-30 (specification reference). In fact, there are six engine test conditions (stage 1 to stage 6) with six different speed/torque/temperature conditions according to the classical ASTM D8114 standard test method.34 However, in this article, we will only discuss the results obtained from stage 1 and 3 engine test conditions corresponding to high temperature and high torque operating conditions. Indeed, under such conditions, a significant part of the friction losses is related to the “boundary lubrication regime”35,36 where MoDTC are known to be effective.
During the engine tests, oil samples were regularly collected over a period of 143 h for tribological tests and for HPLC-MS analyses.
2.3. Ball-on-flat tribotests
Tribological tests were carried out using an Anton Paar MCR302 rheometer with a T-PTD-200 tribology assembly (ball-on-three-flats). Schematics of the geometry are illustrated in Fig. 2. Using a pipette, approximately 1 mL of the test oil is introduced in the test cell in order to immerse the three lubricated contacts between the ball and the three flats. A Peltier module precisely controls the temperature of the lubricant in the test cell.
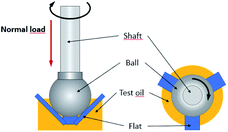 |
| Fig. 2 Schematics of the Anton Paar MCR302 with the ball-on-three-flats geometry. Side (left) and top (right) view. | |
The following parameters were chosen in our experiments: test temperature was 110 °C, normal load 5 N and sliding speed was kept constant throughout 15 min test at 0.01 m s−1. Upper ball was made of AISI 52100 hardened steel (roughness) and flats were made of 100Cr6 hardened steel (60–66 HRC). The surface roughness of the ball was 0.032 μm (Ra1) and 0.35 μm (Ra2) for the flats. The lubrication regime in the three lubricated contact is determined using the lambda ratio λ described in eqn (1) where h corresponds to the average film thickness in the contact. The lambda value calculated for our test conditions was 0.98. Values below the unity are generally representative of a “boundary lubrication regime”35,36 where significant asperities to asperities contacts occur, favouring the reaction of additives and tribofilm formation.
The observation of friction reduction with MoDTC containing oils during tribotests in this study is also indicative of tribofilm formation characteristic of the boundary lubrication regime.
|
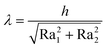 | (1) |
Two examples of friction curves measured with the selected tribological setup on an engine lubricant containing MoDTC and tested in fresh conditions (blue curve) and aged conditions (red curve) are shown in Fig. 3. The oil was aged using a glassware oxidation test at 170 °C for 72 h with 100 ppm iron catalyst. The blue curve is characteristic of the formation of a low friction tribofilm on the contact surface, whereas the red curve does not show any friction reduction performance, thereby indicating the lack of activation of the MoDTC in the lubricated contact. Similar curves are generated on engine test samples, and only final friction coefficients measured at the end of the 15 min tribotest are reported in this study.
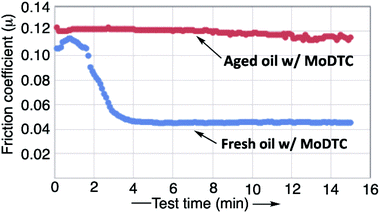 |
| Fig. 3 Examples of friction curves measured on the ball-on-three-flats tribotest on an engine lubricant containing MoDTC. Blue curve: fresh oil; red curve: aged oil (glassware oxidation test, 170 °C, 72 h, 100 ppm iron catalyst). | |
The precision values on the friction coefficient were calculated over a data set of 84 friction tests on the same reference oil. A standard deviation of 1.8 × 10−3 has been calculated on the friction coefficient leading to a ±0.002 confidence interval at 95% on the mean friction coefficient value.
2.4. HPLC-MS analyses
Oil samples collected during engine tests were analyzed by HPLC-MS using an Agilent HP 1100 series instrument equipped with an auto-injector and connected to a Bruker Esquire 3000Plus ion trap mass spectrometer. A Chemstation chromatography manager software (HPLC; Agilent) and a Bruker Data Analysis software (MS; Bruker) were used. Separations were achieved on a Zorbax SIL 5 μm column (Agilent, 4.6 × 250 mm) at 30 °C. The sample injection volume was set at 10 μL. Compounds were eluted with a flow rate of 0.4 mL min−1 in isocratic mode using n-heptane/isopropanol: 98.5
:
1.5 v/v as the mobile phase. The mass spectrometer was equipped with an atmospheric pressure photoionization source (APPI) used in the positive ionisation mode. Conditions for MS analyses were: nebulizer pressure 50 psi, APPI temperature 420 °C, drying temperature 350 °C, drying gas (N2) flow 5 L min−1, capillary voltage −2 kV, corona 4 microamperes, scan range m/z 400–2000. HPLC-MS analyses were performed in triplicate for each sample collected. Each sample was prepared as follows: 20 μL of internal standard (2,3-bis(n-octadecyloxy)propan-1-ol; 1.7 mg mL−1) were added to an aliquot (20 mg) of each sample collected from engine test diluted in 1 mL of solvent (n-heptane/isopropanol: 95
:
5 v/v). The samples were then diluted by 10-fold using the same solvent before analysis by HPLC-MS.
3. Results and discussion
3.1. Tribological performance of oils A and AMBDTC after engine tests – friction coefficients and fuel economy
Fig. 4 shows the evolution with time of the friction coefficient during the engine test performed following the ASTM D8114 standard test method using oils A and AMBDTC.
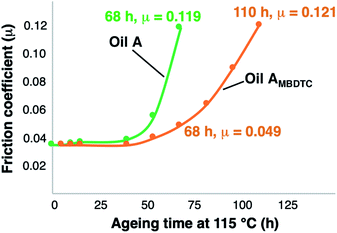 |
| Fig. 4 Evolution of friction coefficients for oil A and AMBDTC as a function of ageing time under engine test conditions. | |
Similar low values of friction coefficients were observed for both oils during the first 40 h of engine test. However, the friction coefficient of oil A increased sharply and reached a value of μ = 0.119 after 68 h of ageing time, implying a huge loss of the ability of oil A to reduce friction. Such a behaviour is likely related, at least partly, to a loss of performance of MoDTC complexes in this oil. On the other hand, oil AMBDTC showed a much lower value of the friction coefficient after 68 h of ageing time (μ = 0.049) and a value of the friction coefficient comparable to that of oil A after 68 h was attained for oil AMBDTC only after 110 h of ageing time (μ = 0.121). These results, showing that the friction reducing capability of oil AMBDTC after 110 h of ageing time is similar to that of oil A after 68 h of ageing time (μ = 0.121 vs. 0.119), indicate that oil AMBDTC possesses a better retention of friction reducing capabilities over longer periods of time as compared to oil A.
Parallelly to the measurement of friction coefficients, the percentages of fuel economy induced by oils A and AMBDTC relative to fuel consumption when a reference lubricant (SAE 20W-30) was used were measured under stage 1 and 3 engine test conditions as described in section 2.2. The percentages of fuel economy for oils A and AMBDTC relative to the reference oil were calculated for various ageing times according to eqn (2).
|
% of fuel economy = [(FCtested oil − FCreference oil)/FCreference oil] × 100
| (2) |
Eqn (2): formula used to calculate the percentage of fuel economy induced by the use of oil A or oil AMBDTC relative to the reference oil (SAE 20W-30) during engine test performed following the ASTM D8114 standard method. FC: fuel consumption (in kg).
Fig. 5 depicts the evolution of the percentage of fuel economy for oils A and AMBDTC as a function of ageing time. Fuel consumption using oil AMBDTC was always shown to be lower than that measured with oil A after 54 h of ageing time under both stage 1 and stage 3 engine test conditions (Fig. 5). These results clearly showed that the use of oil AMBDTC led to a better fuel efficiency of the engine.
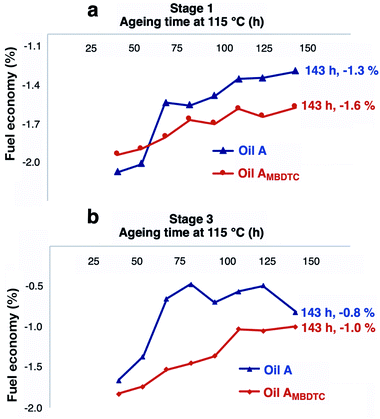 |
| Fig. 5 Evolution of the percentage of fuel economy related to the use of oils A and AMBDTC relative to the fuel consumption of engine using a reference oil (SAE 20W-30) under stage 1 (a) and 3 (b) of engine test performed following the ASTM D8114 standard. | |
3.2. HPLC-MS analysis of MoDTC in oils A and AMBDTC during engine tests
3.2.1 Qualitative analysis of MoDTC. HPLC-MS qualitative analysis was carried out using the samples collected from oils A and AMBDTC after 40 h, 82 h and 143 h of engine test at 115 °C. Our HPLC-MS analytical method was developed in order to separate the mixture of MoDTC substrates 1a–1c for identification purposes based on their mass spectra (Fig. 6 and 7).
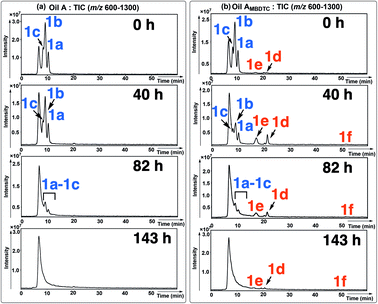 |
| Fig. 6 Chromatograms (HPLC-MS, APPI) showing the distribution of MoDTC in (a) oil A and (b) oil AMBDTC after 0 h, 40 h, 82 h, 143 h of engine test. | |
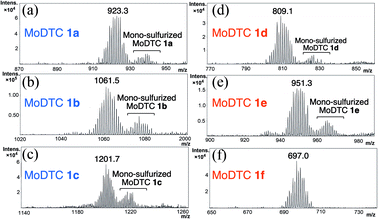 |
| Fig. 7 Mass spectra (APPI detection, positive ionisation mode) of (a–c) MoDTC substrates 1a–1c and (d–f) newly formed MoDTC complexes 1d–1f. | |
The HPLC chromatograms of oils A and AMBDTC at T = 0 h showed the presence of MoDTC 1a–1c which appear as three distinct peaks (partly) separated under the chromatographic conditions used (Fig. 6a and b). The mass spectra of the MoDTC substrates 1a–1c are characterized by a diagnostic complex isotopic pattern due to the presence of 2 molybdenum atoms (Fig. 7a–c). Compounds 1a and 1b (resp. 1c) are separated by 140 mass units (resp. 280 mass units) corresponding to 2 × C5H10 (resp. 4 × C5H10) alkyl chains from the DTC ligands (Fig. 6a–c). For both oils, a progressive decrease in concentration of MoDTC 1a–1c was observed with time, these compounds having completely disappeared between 82 and 143 h (Fig. 6a and b). However, in the case of the oil AMBDTC, the formation with time of new derivatives was noted (Fig. 6b). The specific complex isotopic patterns observed on the mass spectra of the newly formed compounds confirmed that 2 Mo atoms are present on their molecular structures (Fig. 7d–f), like in the case of the genuine MoDTC 1a–1c. In addition, the molecular masses of the newly formed compounds (i.e., [M + H]+ 693–700, 804–817 and 943–957; Fig. 7d–f) are separated by 140 mass units between the first and second eluted compounds, and by 112 mass units between the second and third eluted compounds, suggesting that these compounds correspond to a MoDTC series differing respectively by the presence of a “C10H20” and “C8H16” alkyl entity. Sulfurized MoDTC complexes could also be detected on the mass spectra (Fig. 7) as reported in our previous work.37
The formation of the new Mo species from the MoDTC substrates 1a–1c can be explained by the replacement of one or two of their original dithiocarbamate ligands bearing C8 or C13 alkyl chains by dithiocarbamate ligands bearing C4 alkyl chains, the latter obviously originating from MBDTC 2. The concentration of the new MoDTC complexes 1d–1f increased in oil AMBDTC between 0 and 40 h of engine tests, and progressively decreased between 40 and 143 h, but, in contrast to the genuine MoDTC 1a–1c, they were still present after 143 h of engine functioning (Fig. 6b).
3.2.2 Quantitative analysis of MoDTC. Aliquots of oils A and AMBDTC were collected after 40 h, 82 h and 143 h of engine test for HPLC-MS quantitative analysis. The relative abundances of MoDTC species were determined relative to an internal standard which was added prior to HPLC-MS analysis (see section 2.4).As mentioned above, for both oils A and AMBDTC, the relative concentrations of the MoDTC substrates 1a–1c decreased progressively over time during the first 82 h of engine test and MoDTC 1a–1c were completely consumed between 82 and 143 h (Fig. 8).
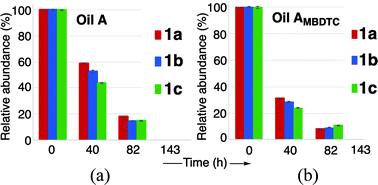 |
| Fig. 8 Evolution of the relative abundance of MoDTC 1a–1c in (a) oil A and (b) oil AMBDTC during engine test. Error bars correspond to triplicate HPLC-MS analyses of each sample. | |
At the first sight, it appeared that MoDTC 1a–1c were consumed faster in the case of the oil containing MBDTC (oil AMBDTC), with only ca. 9–14% of the initial MoDTC remaining after 82 h vs. ca. 15–18% in the case of oil A. However, in oil AMBDTC, the decrease of the concentrations of the MoDTC substrates 1a–1c was accompanied by the formation of new MoDTC complexes with dithiocarbamate ligands bearing C4 alkyl chains (see above).
Quantification showed a progressive increase of the concentrations of these newly formed MoDTC complexes 1d–1f during the first 40 h of engine test, followed by their degradation between 40 and 143 h (Fig. 9). It is worth noting that MoDTC 1d and 1e bearing one ligand with C4 alkyl chains were already present at the starting point (T = 0 h) of the engine test (Fig. 9a and b), which can be explained by the fact that this oil was homogenized by a pre-heating step at 60 °C for 60 min.
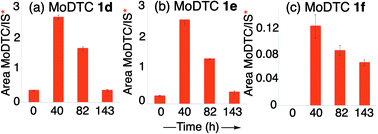 |
| Fig. 9 Evolution of the concentrations of the newly formed MoDTC complexes (a) MoDTC 1d, (b) MoDTC 1e and (c) MoDTC 1f in formulated oil AMBDTC during engine test. *Y-axis: arbitrary units. IS: internal standard. Error bars correspond to triplicate HPLC-MS analyses for each sample. | |
This clearly shows that the ligand exchange reaction between the genuine MoDTC and MBDTC is a rather fast-occurring process. Examination of the behaviour of the different newly formed MoDTC homologues 1d and 1e throughout the engine test experiment showed that MoDTC 1d and 1e bearing only one exchanged ligand presented the same concentration profile, with a sharp increase in concentration during the first 40 h, followed by a progressive decrease during the next 103 h of engine test. This decrease can be explained by two concomitant processes: first, part of the MoDTC 1d and 1e were progressively consumed upon engine functioning and participated to the formation of the MoS2 tribofilm, and second, since these two species of MoDTC 1d and 1e represent intermediates in the formation of MoDTC 1f bearing two exchanged ligands, they were progressively converted into MoDTC 1f with time. The concentration profile of MoDTC 1f, the end product of the ligand exchange reactions, was different in the sense that formation of this compound was only observed after 40 h of reaction, and then its concentration progressively decreased. Contrary to the MoDTC substrates 1a–1c in both oils A and AMBDTC, these three newly-formed MoDTC 1d and 1e complexes could still be detected in oil AMBDTC after 143 h of engine test and were likely still active to form a MoS2 tribofilm (Fig. 10).
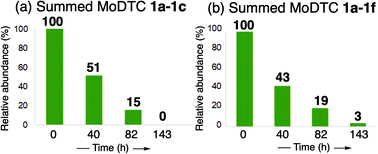 |
| Fig. 10 Evolution of relative abundance of the total pool of MoDTC in (a) oil A and (b) oil AMBDTC during engine test. | |
The evolution of the complete pool of MoDTC in the oils A and AMBDTC after engine tests are shown in Fig. 10. The global concentrations of MoDTC in oil A (sum of 1a–1c) decreased over time with half of the initial MoDTC being consumed after 40 h and fully consumed between 82 and 143 h of engine test (Fig. 10a). For the oil AMBDTC, although the global concentrations of MoDTC complexes (sum of 1a–1f) also decreased over time, small amounts of MoDTC were still present after 143 h, the persisting MoDTC species (ca. 3%) being however exclusively present as newly formed MoDTC 1d–1f (Fig. 10b).
Since the only MoDTC species persisting after 143 h of engine tests bear dithiocarbamate ligands with C4 alkyl chains (i.e., MoDTC 1d–1f) in oil AMBDTC (Fig. 11), their presence can be attributed to the continuous formation of new MoDTC complexes that result from ligand transfer reactions from the MBDTC compound 2 to the genuine MoDTC 1a–1c as well as to the partly altered MoDTC due to the thermo-oxidative conditions prevailing during the engine test (Fig. 11).
 |
| Fig. 11 Scheme showing the ligand transfer reactions between MBDTC 2 and MoDTC 1a–1c leading to the formation of new MoDTC complexes 1d–1f with dithiocarbamate ligands bearing C4 alkyl chains. | |
4. Conclusion
Based on engines tests performed using a formulated engine oil containing MoDTC and MBDTC and, for comparison, the same formulated engine oil devoid of MBDTC, our study has shown that the combined use of MoDTC and MBDTC results in an enhanced preservation of fuel efficiency with ageing time. It could be established unambiguously, and for the first time, at the molecular level using HPLC-MS, that this performance improvement was most likely related to the interactions between MoDTC and MBDTC which showed beneficial effect on the lifetime of MoDTC in lubricants, allowing the friction coefficient of engine lubricants to be maintained at lower level for longer periods of time and, as a consequence, fuel consumption to be reduced. Indeed, using a specifically developed HPLC-MS method, MoDTC could still be detected in the formulated lubricant containing MBDTC after 143 h of engine test, whereas MoDTC were completely consumed after only ca. 82 h under the same conditions in the same formulated lubricant but devoid of MBDTC. Since the remaining MoDTC after prolonged functioning of engine were shown to exclusively bear ligands corresponding to DTC moieties from MBDTC, it can be concluded that the prolonged existence of MoDTC was due to the progressive replacement of the degraded DTC ligands from MoDTC educts by DTC ligands released from MBDTC during engine functioning. These newly formed MoDTC complexes were progressively consumed during engine functioning as were the genuine MoDTC species, but were shown to be concomitantly regenerated and remained in the formulated engine oil at concentrations high enough to ensure the reduction of the friction coefficient to lower levels for an extended duration, as indicated by the tribological measurements. For the MoDTC progressively degraded upon engine functioning, MBDTC thus represents a “stock” of DTC ligands which are released by a yet unknown mechanism during engine functioning and regenerates active MoDTC species, leading to better fuel efficiency performances of the engine.
Conflicts of interest
There are no conflicts to declare.
Acknowledgements
The authors thank Total Marketing Services for funding this research work that was carried out at the University of Strasbourg and Total Solaize Research Center (CRES), and for supplying the base oils and the additives used in this study. The authors also thank E. Motsch for her help in the MS analyses. Y. M. K. thanks Total Marketing Services and the French “Ministère de l'Enseignement Supérieur, de la Recherche et de l'Innovation” for a doctoral fellowship (CIFRE).
Notes and references
- A. A. Malikopoulos, Impact of component sizing in plug-in hybrid electric vehicles for energy resource and greenhouse emissions reduction, J. Energy Resour. Technol., 2013, 135, 4 CrossRef.
- W. Sierzchula, S. Bakker, K. Maat and B. V. Wee, The competitive environment of electric vehicles: an analysis of prototype and production models, Environ. Innov. Soc. Transit., 2012, 2, 49–65 CrossRef.
- M. A. Tamor, C. Gearhart and C. Soto, A statistical approach to estimating acceptance of electric vehicles and electrification of personal transportation, Transport. Res. C Emerg. Technol., 2013, 26, 125–134 CrossRef.
- D. Petitjean, L. Bernardini, C. Middlemass and S. M. Shahed, Advanced gasoline engine turbocharging technology for fuel economy improvements, SAE Technical Paper No. 2004-01-0988, 2004, DOI:10.4271/2004-01-0988.
- D. Takaki, H. Tsuchida, T. Kobara, M. Akagi, T. Tsuyuki and M. Nagamine, Study of an EGR system for downsizing turbocharged gasoline engine to improve fuel economy, SAE Technical Paper No. 2014-01-1199, 2014, DOI:10.4271/2014-01-1199.
- Y. S. Jo, L. Bromberg and J. Heywood, Optimal use of ethanol in dual fuel applications: effects of engine downsizing, spark retard, and compression ratio on fuel economy, SAE Int. J. Engines, 2016, 9, 1087–1101 CrossRef.
- Y. Iwamoto, K. Noma, O. Nakayama, T. Yamauchi and H. Ando, Development of gasoline direct injection engine, SAE Technical Paper No. 970541, 1997, DOI:10.4271/970540.
- C. Park, S. Kim, H. Kim and Y. Moriyoshi, Stratified lean combustion characteristics of a spray-guided combustion system in a gasoline direct injection engine, Energy, 2012, 41, 401–407 CrossRef CAS.
- C. D. McAllister and T. W. Simpson, Multidisciplinary robust design optimization of an internal combustion engine, J. Mech. Des., 2003, 125, 124–130 CrossRef.
- K. R. Cooper, Commercial vehicle aerodynamic drag reduction: historical perspective as a guide, in The aerodynamics of heavy vehicles: trucks, buses, and trains, Lecture notes in applied and computational mechanics, ed. R. McCallen, F. Browand and J. Ross, Springer, Berlin, Heidelberg, 2004, pp. 9–28 Search PubMed.
- G. Sovran, Tractive-energy-based formulae for the impact of aerodynamics on fuel economy over the EPA driving schedules, SAE Technical Paper No. 830304, 1983, DOI:10.4271/830304.
- S. Cecchela, D. Chindamo, E. Turrini, C. Carnevale, G. Cornacchia, M. Gadola, A. Panvini, M. Volta, D. Ferrario and R. Golimbioschi, Impact of reduced mass of light commercial vehicles on fuel consumption, CO2 emissions, air quality, and socio-economic costs, Sci. Total Environ., 2018, 613–614, 409–417 CrossRef PubMed.
- S. Fukuoka, N. Hara, A. Mori and K. Ohtsubo, Friction loss reduction by new lighter valve train system, JSAE Rev., 1997, 18, 107–111 CrossRef.
- W. Waddey, H. Shaub, J. Pecoraro and R. Carley, Improved fuel economy via engine oils, SAE Technical Paper No. 780599, 1978, DOI:10.4271/780599.
- H. A. Spikes, Friction modifier additives, Tribol. Lett., 2015, 60, 5 CrossRef.
- M. Skjoedt, R. Butts, D. N. Assanis and S. V. Bohac, Effects of oil properties on spark-ignition gasoline engine friction, Tribol. Int., 2008, 41, 556–563 CrossRef CAS.
- A. B. Greene and T. J. Risdon, The effects of molybdenum-containing, oil-soluble friction modifiers on engine fuel economy and gear oil efficiency, SAE Technical Paper No. 811187, 1981, DOI:10.4271/811187.
- A. Morina, A. Neville, J. H. Green and M. Priest, Additive/additive interactions in boundary lubrication – a study of film formation and tenacity, Tribol. Interface Eng. Ser., 2005, 48, 757–767 CAS.
- M. I. De Barros Bouchet, J. M. Martina, Th. Le Mogne, P. Bilas, B. Vacher and Y. Yamada, Mechanisms of MoS2 formation by MoDTC in presence of ZnDTP: effect of oxidative degradation, Wear, 2005, 258, 1643–1650 CrossRef CAS.
- J. Graham, H. Spikes and R. Jensen, The friction reducing properties of molybdenum dialkyldithiocarbamate additives: Part II – durability of friction reducing capability, Tribol. Trans., 2001, 44, 637–647 CrossRef CAS.
- K. Inoue, E. Tominaga, K. Akiyama and T. Ashida, Effects of lubricant composition on fuel efficiency in modern engines, SAE Technical Paper No. 951037, 1995, DOI:10.4271/951037.
- G. Spengler and A. Webber, On the lubricating performance of organic molybdenum compounds, Chem. Ber., 1939, 92, 2163–2171 CrossRef.
- C. Grossiord, K. Varlot, J. M. Martin, Th. Le Mogne, C. Esnouf and K. Inoue, MoS2 single sheet lubrication by molybdenum dithiocarbamate, Tribol. Int., 1998, 31, 737–743 CrossRef CAS.
- M. Johnson, R. Jensen and S. Korcek, Base oil effects on friction reducing capabilities of molybdenum dialkyldithiocarbamate containing engine oils, SAE Technical Paper No. 972860, 1997, DOI:10.4271/972860.
- A. Stipanovic and J. Schoonmaker, The impact of organomolybdenum compounds on the frictional characteristics of crankcase engine oils, SAE Technical Paper No. 932779, 1993, DOI:10.4271/932779.
- M. De Feo, PhD thesis, Université de Lyon, 2015 Search PubMed.
- M. De Feo, C. Minfray, M. I. De Barros Bouchet, B. Thiébaut and J. M. Martin, MoDTC friction modifier additive degradation: Correlation between tribological performance and chemical changes, RSC Adv., 2015, 5, 93786–93796 RSC.
- M. D. Johnson, R. K. Jensen, E. M. Clausing, K. Schriewer and S. Korcek, Effects of aging on frictional properties of fuel efficient engine oils, SAE Technical Paper No. 952532, 1995, DOI:10.4271/952532.
- A. Boffa and S. Hirano, Oil impacts on sequence VIB fuel economy, SAE Technical Paper No. 2001-01-1903, 2001, DOI:10.4271/2001-01-1903.
- Y. Yamada, M. Ishimaru, A. Yaguchi, K. Inoue, K. Akiyama and H. Kawai, Retention of friction reducing performance of MoDTC-containing fuel efficient gasoline engine oils during use, SAE Technical Paper No. 2000-01-2053, 2000, DOI:10.4271/2000-01-2053.
- L. Wang, L. Wang, G. Li and X. Xu, Effect of ash-less antioxidants on fuel efficiency retention of PCMO containing MoDTC, in Proceedings of the FISITA 2012 World Automotive Congress, Lecture Notes in Electrical Engineering, ed. SAE-China and FISITA, Springer, Berlin, Heidelberg, 2013, pp. 483–491 Search PubMed.
- K. Arai, M. Yamada, S. Asano, S. Yoshizawa, H. Ohira, K. Hoshino, F. Ueda and K. Akiyama, Lubricant technology to enhance the durability of low friction performance of gasoline engine oils, SAE Technical Paper No. 952533, 1995, DOI:10.4271/952533.
- Y. M. Kiw, P. Schaeffer, P. Adam, B. Thiébaut, C. Boyer and G. Papin, Ligand exchange processes between molybdenum and zinc additives in lubricants: evidence from NMR (1H,13C,31P) and HPLC-MS analysis, RSC Adv., 2020, 10, 37962–37973 RSC.
- ASTM D8114-21a, Standard Test Method for Measurement of Effects of Automotive Engine Oils on Fuel Economy of Passenger Cars and Light-Duty Trucks in Sequence VIE Spark Ignition, ASTM International, West Conshohocken, PA, 2021, https://www.astm.org Search PubMed.
- V. Sharma, R. B. Timmons, A. Erdemir and P. B. Aswath, Interaction of plasma functionalized TiO2 nanoparticles and ZDDP on friction and wear under boundary lubrication, Appl. Surf. Sci., 2019, 489, 372–383 CrossRef CAS.
- M. De Feo, M. I. De Barros Bouchet, C. Minfray, C. Esnouf, Th. Le Mogne, F. Meunier, L. Yang, B. Thiebaut, S. Pavan and J. M. Martin, Formation of interfacial molybdenum carbide for DLC lubricated by MoDTC: Origin of wear mechanism, Wear, 2017, 370–371, 17–28 CrossRef CAS.
- Y. M. Kiw, P. Adam, P. Schaeffer, B. Thiébaut and C. Boyer, Molecular evidence for sulfurization of molybdenum dithiocarbamates (MoDTC) by zinc dithiophosphates: a key process in their synergetic interactions and the enhanced preservation of MoDTC in formulated lubricants?, RSC Adv., 2022, 12, 3542–3553 RSC.
|
This journal is © The Royal Society of Chemistry 2022 |
Click here to see how this site uses Cookies. View our privacy policy here.