DOI:
10.1039/D2RA02473J
(Paper)
RSC Adv., 2022,
12, 12983-12987
Palladium-catalysed difluoroolefination of benzyl tosylates toward the synthesis of gem-difluoro-2-trifluromethyl styrene derivatives†
Received
17th April 2022
, Accepted 21st April 2022
First published on 28th April 2022
Abstract
We have presented an efficient method to access gem-difluoro-2-trifluromethyl styrene derivatives via palladium catalysis. This method features mild reaction conditions, broad substrate scope and good product yields. Moreover, gram–scale reactions demonstrated the robustness and potential of this method. Control experiments revealed that the –CF3 group was essential to the success of this transformation. Finally, the practicality of this method was successfully proven by three synthetic applications.
Introduction
Fluorinated compounds have found wide applications in various fields due to their unique properties.1 Among them, gem-difluorostyrenes have been frequently used in the design of potential enzyme inhibitors.2 Introducing α –CF3 group into gem-difluoroolefins could not only retain its high electrophilicity towards many nucleophiles at the terminal carbon, but also increase the biological activity of the molecules (Fig. 1a).3
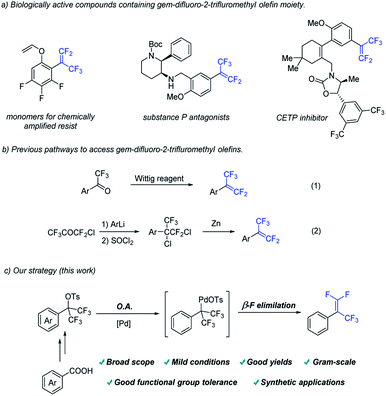 |
| Fig. 1 Importance of gem-difluoro-2-trifluoromethyl olefins and synthetic strategy. | |
Compared with considerable efforts devoted to the development of gem-difluorostyrenes,4,5 the synthesis of gem-difluoro-2-trifluromethyl styrenes is less investigated. The most common method is Wittig gem-difluoroolefination of trifluoroacetophenone (Fig. 1b(1)).6 Another pathway is a multistep strategy involving nucleophilic addition of an aryl metallic reagent to chloropentafluoroacetone, SN2 type substitution of chloride anions and dechlorination with Zn (Fig. 1b(2)).7 However, stochiometric phosphine oxide as a by-product, utilization of organometallic reagents and multistep operation have greatly restricted substrate scope and applications of the methods above. Therefore, it is of great significance to develop a complementary method for the synthesis of gem-difluoro-2-trifluromethyl styrenes.
On the other hand, transition-metal catalysis plays an irreplaceable role in modern organic synthesis.8 We hypothesize that a method including two elementary reactions to access gem-difluoro-2-trifluromethyl styrenes from trifluoromethyl-substituted benzyl tosylate by transition metal catalysis could be developed (Fig. 1c). From the perspective of elementary reactions, the oxidative addition of palladium catalyst into Csp3–O bond9 and β–F elimination of palladium complex10 have been realized in different transformations in the reported work respectively. Therefore, the key to success of this strategy is to find a suitable catalyst system which is compatible with the two elementary reactions above.
Results and discussion
To demonstrate our hypothesis, we began the study by evaluating gem-difluoroolefination of trifluoromethyl-substituted benzyl tosylate (1a) via palladium catalysis (Table 1). Compound 1a could be easily synthesized from corresponding aryl carboxylic acid.11 After evaluation of all reaction parameters, reaction conditions which could provide a high yield of 2a was identified. The optimum reaction conditions consisted of PdI2 (5 mol%) with bidentate ligand DPPP (5 mol%) as catalyst, zinc (2.0 equiv.) as reductant, and DMA as solvent at 80 °C (entry 1). Using other palladium sources as catalyst resulted in lower yields (entries 2–5). Variation of monodentate and other bidentate phosphine ligands from DPPP led to moderate yields of 2a (entries 6–11), while nitrogen ligands would inhibit the reaction with the majority of 1a unconverted (entries 12–13). Solvents screening revealed that DMA was the best choice for this transformation (entries 14–18). Lastly, reaction temperature investigation suggested that the desired product 2a could be formed in the highest yield at 80 °C, although the yield was acceptable at 40 °C (entries 19–21).
Table 1 Optimization of the reaction conditionsa
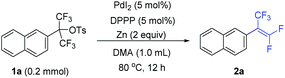
|
Entry |
Variation from std. conditions |
Yield of 2ab |
Standard reaction conditions: 1a (0.2 mmol), PdI2 (5 mol%), DPPP (5 mol%), Zn (2 equiv.), DMA (1.0 mL), 80 °C, 12 h. Yields were determined by GC analysis using dodecane as an internal standard. Isolated yield in the parenthesis. |
1 |
None |
93% (90%)c |
2 |
Pd(dba)2 instead of PdI2 |
60% |
3 |
PdCl2 instead of PdI2 |
61% |
4 |
Pd(acac)2 instead of PdI2 |
86% |
5 |
Pd(PPh3)2Cl2 instead of PdI2 |
82% |
6 |
PPh3 instead of DPPP |
64% |
7 |
PCy3 instead of DPPP |
68% |
8 |
(n-Bu)P(ad)2 instead of DPPP |
65% |
9 |
DPEPhos instead of DPPP |
27% |
10 |
DPPF instead of DPPP |
43% |
11 |
XantPhos instead of DPPP |
73% |
12 |
1,10-Phenanthroline instead of DPPP |
9% |
13 |
2,2-Bipyridine instead of DPPP |
20% |
14 |
DMF instead of DMA |
54% |
15 |
MeCN instead of DMA |
57% |
16 |
THF instead of DMA |
34% |
17 |
Toluene instead of DMA |
29% |
18 |
1,4-Dioxane instead of DMA |
31% |
19 |
100 °C instead of 80 °C |
85% |
20 |
60 °C instead of 80 °C |
86% |
21 |
40 °C instead of 80 °C |
75% |
With the optimized conditions in hand, the substrate scope of this transformation was investigated and the results are summarized in Scheme 1. Initially, substrates with electron-neutral aryl groups, such as naphthalene ring and benzene ring, were examined. The reactions proceeded smoothly and produced the corresponding gem-difluoroolefins in excellent yields (2b–2c). Benzene ring bearing a phenyl substituent at the para and ortho position also afforded the corresponding products in good yields (2d–2e). In addition, vinyl group were also well tolerated (2f). Next, compounds with electron-rich substituents were evaluated. The usage of isopropyl-, methyl-, methoxy-substituted substrates led the formation of corresponding products in moderated to good yields (2g–2k). Various electron-poor substrates were also investigated. Compounds containing halogen and trifluoromethyl groups in para position were adapted to the reactions and gave desired products in moderate yields (2l–2o). The configuration of compound 2o was confirmed by X-ray crystallography.12 Notably, aryl ring bearing bromide at the ortho position had positive hindrance effect on the reaction, resulting in good yields (2p–2q). In addition, both Boc- and sulfonamide-substituted tosylates were tolerated, giving the corresponding products 2r and 2s in 70% and 87% yields respectively. Aryl ring containing methoxy at the meta position (2t) obtained 85% yield, while substrate with an ester group (2u–2v) led to a lower yield. Pleasingly, the reaction was compatible with a range of heterocycles, as demonstrated by the excellent yields obtained for a series of substrates containing pyridine, furan and quinoline ring (2w–2z). Lastly, diaryltrifluoromethyl tosylate could also be converted to corresponding gem-difluorostyrene (2aa) in moderate yield. Unfortunately, nitro (2ab), amino (2ac) and cyano (2ad) groups were found to unsuitable for the reaction.
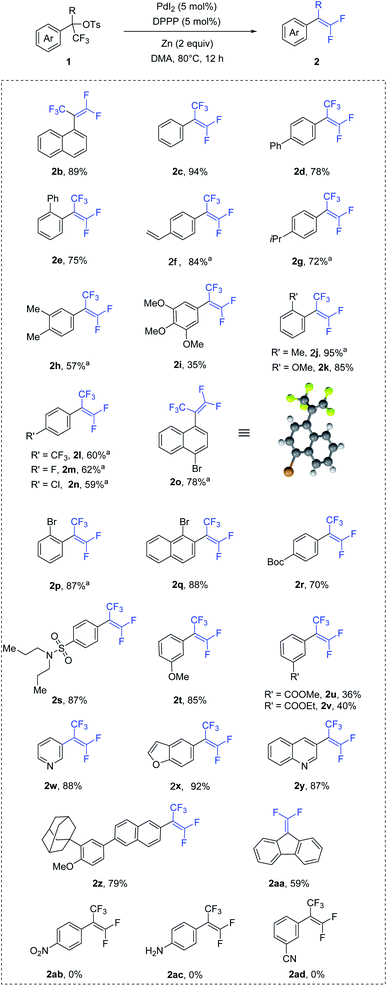 |
| Scheme 1 Substrate scope. aThe temperature is 100 °C and PdI2 (10 mol%), DPPP (10 mol%) were used. | |
The robustness and potential of this method have also been successfully demonstrated by 2a (88% yield) and 2c (72% yield) in gram–scale reactions (Scheme 2a). Next, the effect of –CF3 group was investigated (Scheme 2b). Mono–CF3–substituted benzyl tosylate 3 was subjected to the standard conditions, resulting in β-F elimination product 3a (10%), β-H elimination product 3b (36%) and protonated product 3c (28%). To gain more insight into the mechanism, a control experiment was carried out (Scheme 2c). The reaction was carried out in the presence of D2O (2.0 equiv.) or CD3OD (2.0 equiv.) under the standard conditions, leading to the formation of protonated product D-4 or D-4’. This result indicated that Pd(0) was oxidatively added into C–OTs bond rather than C–F bond.
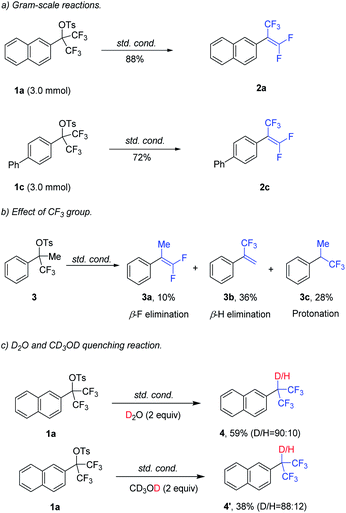 |
| Scheme 2 Gram-scale reactions and control experiments. | |
To illustrate synthetic utility of this methodology, previously synthesized 2a was subjected to subsequent transformations (Scheme 3). Firstly, the reaction of compound 2a with imidazole in the presence of K3PO4 could provide the N-(α-fluorovinyl)azole product 5.13,15 Likewise, treatment of 2a with sodium phenyl thiolate in THF at room temperature for 12 h resulted in the formation of vinyl sulfide 6 (Z
:
E = 7
:
1) in 75% yield.14,15 Lastly, in the presence of palladium catalyst, allylic alkylation between 2a and allyl tert-butyl carbonate 7 could take place, in which the nucleophilic addition of external fluoride onto gem-difluoroalkenes was the initial step.16
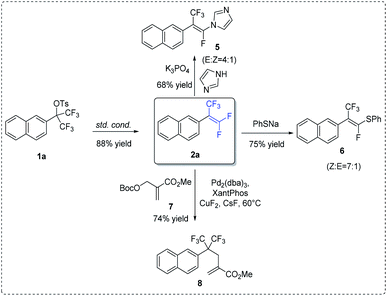 |
| Scheme 3 Synthetic applications. | |
Conclusions
In conclusion, we have developed an efficient pathway to access gem-difluoro-2-trifluromethyl styrene derivatives via palladium catalysis. This transformation features mild reaction conditions, broad functional group tolerance and good yields. Gram-scale reactions have demonstrated the robustness and potential of this method, and various synthetic applications have proved the practicality of this strategy.
Conflicts of interest
There are no conflicts to declare.
Acknowledgements
This work is supported by National Natural Science Foundation of China (21901168), “1000-Youth Talents Plan”, Sichuan Science and Technology Program (2021YJ0395) and “1.3.5 project for disciplines of excellence, West China Hospital, Sichuan University”. We also thank Jing Li from the Comprehensive Training Platform of Specialized Laboratory in College of Chemistry at Sichuan University for sample analysis.
Notes and references
-
(a) K. Müller, C. Faeh and F. Diederich, Fluorine in Pharmaceuticals: Looking Beyond Intuition, Science, 2007, 317, 1881–1886 CrossRef PubMed;
(b) E. P. Gillis, K. J. Eastman, M. D. Hill, D. J. Donnelly and N. A. Meanwell, Applications of Fluorine in Medicinal Chemistry, J. Med. Chem., 2015, 58, 8315–8359 CrossRef CAS PubMed;
(c) L. Xing, D. C. Blakemore, A. Narayanan, R. Unwalla, F. Lovering, R. A. Denny, H. Y. Zhou and M. E. Bunnage, Fluorine in Drug Design: A Case Study with Fluoroanisoles, ChemMedChem, 2015, 10, 715–726 CrossRef CAS PubMed.
-
(a) J.-M. Altenburger, G. Y. Lassalle, M. Matrougui, D. Galtier, J.-C. Jetha, Z. Bocskei, C. N. Berry, C. Lunven, J. Lorrain, J.-P. Herault, P. Schaeffer, S. E. O'Connor and J.-M. Herbertb, SSR182289A, a selective and potent orally active thrombin inhibitor, Bioorg. Med. Chem., 2004, 12, 1713–1730 CrossRef CAS PubMed;
(b) M. A. Rogawski, Diverse mechanisms of antiepileptic drugs in the development pipeline, Epilepsy Res., 2006, 69, 273–294 CrossRef CAS PubMed;
(c) W. R. Moore, G. L. Schatzman, E. T. Jarvi, R. S. Gross and J. R. McCarthy, Terminal difluoro olefin analogs of squalene are time-dependent inhibitors of squalene epoxidase, J. Am. Chem. Soc., 1992, 114, 360–361 CrossRef CAS.
-
(a) N. Mutsuo, H. Jun, W. Atsushi and H. Yuji, Styrenes as monomers for chemically amplified resist base polymers, JP 2001181226,A 2001 Search PubMed;
(b) L. Seohee, O. Jungtaek, L. Jaekwang, L. Jaewon, B. Suyeal, H. Nina and L. Sera, Preparation of cycloalkenyl aryl derivatives as therapeutic CETP inhibitor, WO 2012141487, A2 2012 Search PubMed;
(c) S. Kunio, S. Yuji and W. Hiroaki, Preparation of substituted benzylaminopiperidines as substance P antagonists, WO 9708144, A1, 1997 Search PubMed.
- For selected reviews on the synthesis of gem-difluorostyrenes, see:
(a) G. Chelucci, Synthesis and Metal-Catalyzed Reactions of gem-Dihalovinyl Systems, Chem. Rev., 2012, 112, 1344–1462 CrossRef CAS PubMed;
(b) J. Ichikawa, gem-Difluoroolefin synthesis: general methods via thermostable difluorovinylmetals starting from 2,2,2-trifluoroethanol derivatives, J. Fluorine Chem., 2000, 105, 257–263 CrossRef CAS;
(c) X. X. Zhang and S. Cao, Recent advances in the synthesis and C-F functionalization of gem-difluoroalkenes, Tetrahedron Lett., 2017, 58, 375–392 CrossRef CAS;
(d) F. T. Tian, G. B. Yan and J. Yu, Recent advances in the synthesis and applications of α-(trifluoromethyl)styrenes in organic synthesis, Chem. Commun., 2019, 55, 13486–13505 RSC.
- Selected examples, see:
(a) Q. Pan, Y. Y. Ping, Y. F. Wang, Y. Guo and W. Q. Kong, Ni-Catalyzed Ligand-Controlled Regiodivergent Reductive Dicarbofunctionalization of Alkenes, J. Am. Chem. Soc., 2021, 143, 10282–10291 CrossRef CAS PubMed;
(b) J. Zheng, J. Cai, J. H. Lin, Y. Guo and J. C. Xiao, Synthesis and decarboxylative Wittig reaction of difluoromethylene phosphobetaine, Chem. Commun., 2013, 49, 7513–7515 RSC;
(c) T. B. Xiao, L. Y. Li and L. Zhou, Synthesis of Functionalized gem-Difluoroalkenes via a Photocatalytic Decarboxylative/Defluorinative Reaction, J. Org. Chem., 2016, 81, 7908–7916 CrossRef CAS PubMed;
(d) L. H. Wu, J. K. Cheng, L. Shen, Z. L. Shen and T. P. Loh, Visible Light-Mediated Trifluoromethylation of Fluorinated Alkenes via C–F Bond Cleavage, Adv. Synth. Catal., 2018, 360, 3894–3899 CrossRef CAS;
(e) Y. Ma, B. R. P. Reddy and X. H. Bi, Coupling of Trifluoroacetaldehyde N-Triftosylhydrazone with Organoboronic Acids for the Synthesis of gem-Difluoroalkenes, Org. Lett., 2019, 21, 9860–9863 CrossRef CAS PubMed;
(f) P. Gao, G. Q. Wang, L. L. Xi, M. Y. Wang, S. H. Li and Z. Z. Shi, Transition-Metal-Free Defluorosilylation of Fluoroalkenes with Silylboronates, Chin. J. Chem., 2019, 37, 1009–1014 CrossRef CAS;
(g) J. L. Zeng, Y. Zhang, M. M. Zheng, Z. Q. Zhang, X. S. Xue, F. G. Zhang and J.-A. Ma, Chemodivergent and Stereoselective Construction of gem-Difluoroallylic Amines from Masked Difluorodiazo Reagents, Org. Lett., 2019, 21, 8244–8249 CrossRef CAS PubMed;
(h) X. Lu, X. X. Wang, T. J. Gong, J. J. Pi, S. J. He and Y. Fu, Nickel-catalyzed allylic defluorinative alkylation of trifluoromethyl alkenes with reductive decarboxylation of redox-active esters, Chem. Sci., 2019, 10, 809–814 RSC;
(i) Y. Y. Cai, H. Zeng, C. L. Zhu, C. Liu, G. Y. Liu and H. F. Jiang, Double allylic defluorinative alkylation of 1,1-bisnucleophiles with (trifluoromethyl)alkenes: construction of all-carbon quaternary centers, Org. Chem. Front., 2020, 7, 1260–1265 RSC;
(j) B. J. Xiong, T. Wang, H. T. Sun, Y. Li, S. Kramer, G.-J. Cheng and Z. Lian, Nickel-Catalyzed Cross-Electrophile Coupling Reactions for the Synthesis of gem-Difluorovinyl Arenes, ACS Catal., 2020, 10, 13616–13623 CrossRef CAS;
(k) Y. W. Wang, L. F. Deng, X. Zhang, Z. D. Mou and D. W. Niu, A Radical Approach to Making Unnatural Amino Acids: Conversion of C–S Bonds in Cysteine Derivatives into C–C Bonds, Angew. Chem., Int. Ed., 2021, 60, 2155–2159 CrossRef CAS PubMed.
-
(a) F. Wang, L. C. Li, C. F. Ni and J. B. Hu, Deoxygenative gem-difluoroolefination of carbonyl compounds with (chlorodifluoromethyl)trimethylsilane and triphenylphosphine, Beilstein J. Org. Chem., 2014, 10, 344–351 CrossRef PubMed;
(b) K. Aikawa, W. Toya, Y. Nakamura and K. Mikami, Development of (Trifluoromethyl)zinc Reagent as Trifluoromethyl Anion and Difluorocarbene Sources, Org. Lett., 2015, 17, 4996–4999 CrossRef CAS PubMed.
-
(a) W. J. Middleton, D. Metzger and J. A. Snyder, 1-Trifluoromethyl-1,2,2-triphenylethylenes. Synthesis and postcoital antifertility activity, J. Med. Chem., 1971, 14, 1193–1197 CrossRef CAS PubMed;
(b) W. J. Middleton and E. M. Bingham, The synthesis of antiinflammatory α-(trifluoromethyl) arylacetic acids, J. Fluorine Chem., 1983, 22, 561–574 CrossRef CAS.
-
(a) D. M. D'Souzaa and T. J. J. Müller, Multi-component syntheses of heterocycles by transition-metal catalysis, Chem. Soc. Rev., 2007, 36, 1095–1108 RSC;
(b) C. Zheng and S. L. You, Catalytic Asymmetric Dearomatization by Transition-Metal Catalysis: A Method for Transformations of Aromatic Compounds, Chem, 2016, 1, 830–857 CrossRef CAS;
(c) M. H. Feng and X. F. Jiang, Reactions of Arynes Involving Transition-Metal Catalysis, Synthesis, 2017, 49, 4414–4433 CAS;
(d) R. Kancherla, K. Muralirajan, A. Sagadevan and M. Rueping, Visible Light-Induced Excited-State Transition-Metal Catalysis, Trends Chem., 2019, 1, 510–523 CrossRef CAS;
(e) M. Belal, Z. Q. Li, X. Q. Lu and G. Y. Yin, Recent advances in the synthesis of 1,1-diarylalkanes by transition-metal catalysis, Sci. China: Chem., 2021, 64, 513–533 CrossRef CAS;
(f) H. Chen, Y. A. Liu and X. B. Liao, Recent Progress in Radical Decarboxylative Functionalizations Enabled by Transition-Metal (Ni, Cu, Fe, Co or Cr) Catalysis, Synthesis, 2021, 53, 1–29 CrossRef CAS.
- Selected examples, see:
(a) X. X. Wang, B. B. Xu, W. T. Song, K. X. Sun and J. M. Lu, N-heterocyclic carbene–palladium(I)-1-methylimidazole complex-catalyzed Suzuki–Miyaura coupling of benzyl sulfonates with arylboronic acids, Org. Biomol. Chem., 2015, 13, 4925–4930 RSC;
(b) Z. C. Cao, D. G. Yu, R. Y. Zhu, J. B. Wei and Z. J. Shi, Direct cross-coupling of benzyl alcohols to construct diarylmethanes via palladium catalysis, Chem. Commun., 2015, 51, 2683–2686 RSC;
(c) X. Q. Cao and Y. G. Zhang, Palladium catalyzed direct benzylation/allylation of malonates with alcohols – in situ C–O bond activation, Green Chem., 2016, 18, 2638–2641 RSC;
(d) S. Tabuchi, K. Hirano and M. Miura, Palladium-Catalyzed Asymmetric Benzylic Alkylation of Active Methylene Compounds with α-Naphthylbenzyl Carbonates and Pivalates, Angew. Chem., Int. Ed., 2016, 55, 6973–6977 CrossRef CAS PubMed;
(e) M. Brambilla and M. Tredwell, Palladium-Catalyzed Suzuki–Miyaura Cross-Coupling of Secondary α-(Trifluoromethyl)benzyl Tosylates, Angew. Chem., Int. Ed., 2017, 56, 11981–11985 CrossRef CAS PubMed;
(f) K. J. Schwarz, C. Yang, J. W. B. Fyfe and T. N. Snaddon, Enantioselective α-Benzylation of Acyclic Esters Using π-Extended Electrophiles, Angew. Chem., Int. Ed., 2018, 57, 12102–12105 CrossRef CAS PubMed;
(g) M. Komatsuda, K. Muto and J. Yamaguchi, Pd-Catalyzed Dearomative Allylation of Benzyl Phosphates, Org. Lett., 2018, 20, 4354–4357 CrossRef CAS PubMed;
(h) J. J. Chen, J. C. Xu, Y. Zhou, S. G. Xie, F. Gao, X. F. Xu, X. H. Xu and Z. Jin, Sequential ortho-C–H and ipso-C–O Functionalization Using a Bifunctional Directing Group, Org. Lett., 2019, 21, 7928–7932 CrossRef CAS PubMed.
- Selected examples, see:
(a) K. Sakoda, J. Mihara and J. Ichikawa, Heck-type 5-endo-trig cyclization promoted by vinylic fluorines: synthesis of 5-fluoro-3H-pyrroles, Chem. Commun., 2005, 37, 4684–4686 RSC;
(b) M. Yokota, D. S. Fujita and J. Ichikawa, Activation of 1,1-Difluoro-1-alkenes with a Transition-Metal Complex: Palladium(II)-Catalyzed Friedel–Crafts-Type Cyclization of 4,4-(Difluorohomoallyl)arenes, Org. Lett., 2007, 9, 4639–4642 CrossRef CAS PubMed;
(c) K. Fuchibe, T. Morikawa, K. Shigeno, T. Fujita and J. Ichikawa, Pinpoint-Fluorinated Phenacenes: New Synthesis and Solubility Enhancement Strategies, Org. Lett., 2015, 17, 1126–1129 CrossRef CAS PubMed;
(d) J. Xu, E. A. Ahmed, B. Xiao, Q. Q. Lu, Y. L. Wang, C. G. Yu and Y. Fu, Pd-Catalyzed Regioselective Activation of gem-Difluorinated Cyclopropanes: A Highly Efficient Approach to 2-Fluorinated Allylic Scaffolds, Angew. Chem., Int. Ed., 2015, 54, 8231–8235 CrossRef CAS PubMed;
(e) R. T. Thornbury and F. D. Toste, Palladium-Catalyzed Defluorinative Coupling of 1-Aryl-2,2-Difluoroalkenes and Boronic Acids: Stereoselective Synthesis of Monofluorostilbenes, Angew. Chem., Int. Ed., 2016, 55, 11629–11632 CrossRef CAS PubMed;
(f) S. Wada and R. F. Jordan, Olefin Insertion into a Pd–F Bond: Catalyst Reactivation Following β-F Elimination in Ethylene/Vinyl Fluoride Copolymerization, Angew. Chem., Int. Ed., 2017, 56, 1820–1824 CrossRef CAS PubMed;
(g) T. Fujita, K. Fuchibe and J. Ichikawa, Transition-Metal-Mediated and -Catalyzed C–F Bond Activation by Fluorine Elimination, Angew. Chem., Int. Ed., 2019, 58, 390–402 CrossRef CAS PubMed;
(h) S. Y. He and X. G. Zhang, Palladium-catalyzed selective defluorinative sulfenylation for the synthesis of fluorovinylthioethers, Org. Chem. Front., 2020, 7, 3174–3178 RSC;
(i) Z. Yang, M. Möller and R. M. Koenigs, Synthesis of gem-Difluoro Olefins through C–H Functionalization and β-fluoride Elimination Reactions, Angew. Chem., Int. Ed., 2020, 59, 5572–5576 CrossRef CAS PubMed;
(j) F. P. Wu, Y. Yuan, J. W. Liu and X. F. Wu, Pd/Cu-Catalyzed Defluorinative Carbonylative Coupling of Aryl Iodides and gem-Difluoroalkenes: Efficient Synthesis of α-Fluorochalcones, Angew. Chem., Int. Ed., 2021, 60, 8818–8822 CrossRef CAS PubMed.
- K. Takahashi, Y. Ano and N. Chatani, Fluoride anion-initiated bis-trifluoromethylation of phenyl aromatic carboxylates with (trifluoromethyl)trimethylsilane, Chem. Commun., 2020, 56, 11661–11664 RSC.
- CCDC-2074740 contains the supplementary crystallographic data for this paper.
- Y. Xiong, X. X. Zhang, T. Huang and S. Cao, Synthesis of N-(α-Fluorovinyl)azoles by the Reaction of Difluoroalkenes with Azoles, J. Org. Chem., 2014, 79, 6395–6402 CrossRef CAS PubMed.
- M. S. Kim and I. H. Jeong, A highly stereoselective preparation of CF3-substituted 1-aryl-1,2-diphenylethenes: application to the synthesis of panomifene, Tetrahedron Lett., 2005, 46, 3545–3548 CrossRef CAS.
- W. Dmowski, Nucleophilic reactions of fluoroolefins.IV. Regioselectivity in the reactions of 1-phenylpentafluoropropenes with alkyllithium reagents, J. Fluorine Chem., 1984, 26, 223–241 CrossRef CAS.
- P. P. Tian, C. Q. Wang, S. H. Cai, S. J. Song, L. Ye, C. Feng and T. P. Loh, F− Nucleophilic-Addition-Induced Allylic Alkylation, J. Am. Chem. Soc., 2016, 138, 15869–15872 CrossRef CAS PubMed.
|
This journal is © The Royal Society of Chemistry 2022 |
Click here to see how this site uses Cookies. View our privacy policy here.