DOI:
10.1039/D2RA01842J
(Paper)
RSC Adv., 2022,
12, 19946-19952
Synthesis of two novel neutral polymeric bonding agents to enhance the mechanical properties of composite solid propellants†
Received
22nd March 2022
, Accepted 23rd June 2022
First published on 8th July 2022
Abstract
Currently, only a few bonding agents can be utilized efficiently in nitramine filler material-based solid rocket energetic binder systems. Herein, we demonstrate the synthesis and specific characterization of two kinds of neutral polymeric bonding agents (NPBA-1 and NPBA-2) and their application in composite propellants consisting of the nitramine octogen (HMX) and glycidyl azide polymer (GAP). The as-obtained NPBAs were well-coated on the surface of HMX and RDX due to their functionalized groups, and they significantly affected the viscosity of the uncured propellant mixtures and possessed obviously enhanced mechanical properties in the cured AP/HMX/GAP propellant mixtures, even at low concentrations (down to 0.001 wt% of the whole propellant). In addition, because of the existence of an epoxy group and no hydroxyl functionalities, NPBA-2 exhibited improved mechanical strength and glass transition temperature as compared to NPBA-1, which has plenty of reactive hydroxyl groups. The as-synthesized epoxy-modified NPBAs are essential for obtaining NEPE propellants with high bonding and mechanical properties.
Introduction
Composite solid propellants are a kind of energy-containing composite polymer polyurethane material with a polymer as the matrix and filler particles as the reinforcement material. The polymer matrix comprises a polymeric binder and bonding agents, and the solid particulates are oxidizer particles, such as HMX (cyclotetramethylene tetranitramine), RDX (cyclotrimethylene trinitramine), and ammonium perchlorate (AP), and metal fuels.1–3 The bonding agent is a functional additive specially used to improve the interface properties of composite propellants. The molecular structure of the bonding agent generally contains two active functional groups: one is a pro-enhancing material group, which improves the interaction force between the molecule and the solid particles through physical and chemical action, and the other is a matrix-friendly group, which generally enters into the polymer matrix cross-linked network through chemical reaction.4,5 Therefore, the introduction of a bonding agent greatly enhances the solid–liquid bonding interactions and further improves the mechanical properties of the propellant. Primitive application of the bonding agent in propellants was studied in the 1960s.6 The mechanical properties of solid propellants are obviously improved by adding a little dosage of a bonding agent. Nowadays, a number of bonding agents, including alkanolamine, aziridine, polyamine and their derivatives,7–12 and neutral polymeric bonding agents,13–15 are utilized in composite propellants. However, most of the bonding agents reported by various research groups are utilized in AP-containing composite propellants, and seldom for HMX- and RDX-based nitrate ester plasticized polyether (NEPE) propellants.9 In this study, two types of NPBAs based on acrylonitrile were synthesized and further functionalized by polar groups to interact with RDX and HMX. Glycidyl methacrylate (GMA) with plenty of unsaturated double bonds was added to the polymer structure to promote copolymerization. The structures and characterization of the as-prepared NPBAs were investigated in-depth, and their applications in propellant formulations based on HMX, GAP, and triethylene glycol dinitrate (TEGDN) were explored. Due to the interactions with HMX, the mechanical properties of the NEPE propellant are obviously improved.
Neutral polymeric bonding agents (NPBAs) were first synthesized by Kim in 1990 by dispersing nitramine crystals in a polar binder, which is a vinyl polymer with various functional groups (Scheme 1). Compared with the small-molecule bonding agents used in the past, NPBAs are polymeric bonding agents, which can solve the problem of the energetic polar plasticizer dissolving the small-molecule bonding agents, which hindered their interaction with the surface of the solid fillers.16–19 When 0.02% NPBA was added to the system with HMX as the filler and PEG (polyethylene glycol) as the binder, the strength of the propellant was 5 times that without NPBA.13 Although NPBAs solve the problem of the soft interface layer of the ammonium nitrate filler, the problems of “dewetting” and “interface bonding” of the oxidant still exist. By modifying the hydrophobic group to adjust the unit composition and solubility parameters of the NPBAs, the phase separation critical temperature can be adjusted. As a result, NPBAs are precipitated from the slurry, adsorbed on the surface of the ammonium nitrate solid particles, and bonded to the filler and the binder intensely.20 Alkynyl-modified NPBAs significantly enhance the tensile strength and interfacial tension of the propellant mixtures by reducing the problem of “dewetting”.21 Gholamian et al. added N-vinylpyrrolidone (NVP) into the NPBA polymer structure to change its phase separation temperature, which has good use in the bonding effect in propellants.22 Therefore, the development of a new type of NPBA with better bonding and stable performance is of great significance for the improvement of the performance of solid rockets.
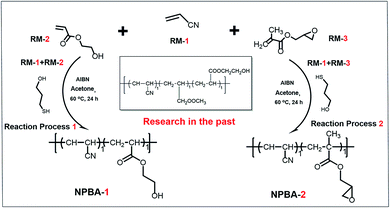 |
| Scheme 1 The molecular structures of a typical NPBA and the synthetic process of NPBA-1 and NPBA-2. | |
In this study, two different compositions of NPBAs based on bonding agents and modified with acrylonitrile were synthesized and further functionalized by polar groups to interact with RDX and HMX. Glycidyl methacrylate (GMA) with plenty of unsaturated double bonds was added to the polymer structure to promote copolymerization. The structures and characterization of the as-prepared NPBAs were investigated in-depth, and their applications in propellant formulations based on HMX, GAP, and triethylene glycol dinitrate (TEGDN) were explored. Due to the interactions with HMX, the mechanical properties of the NEPE propellant are obviously improved.
Experimental
Materials
Acrylonitrile (AN), CP, was obtained from Tianjin Fu Morning Chemical Reagent Factory. Glycidyl methacrylate (GMA), CP, was obtained from Jinan Pulaihua Chemical Co., Ltd. Azobisisobutyronitrile (AIBN), CP, was obtained from Hunan Huihong Reagent Co., Ltd. Mercaptoethanol (β-ME), CP, was obtained from Beijing Chemical Plant, and various analytical reagents, such as acetone and ethanol, were purchased from Sinopharm Chemical Reagent Co., Ltd. (China). GAP and triethylene glycol dinitrate (TEGDN) were both prepared by the Hubei Institute of Aerospace Chemical Technology (China). Cyclotrimethylene trinitramine was provided by Gan Su Ying Guang Chemical Industry Group Co., Ltd, and ammonium perchlorate was obtained from Dalian North Potassium Chlorate Plant (China).
Material characterization
A Tensor 27 FTIR spectrometer was employed to detect the FT-IR spectra of the samples with a KBr reference. The 1H nuclear magnetic resonance (1H NMR) and 13C nuclear magnetic resonance (13C NMR) spectra of the samples were recorded using a Bruker AV 500 (Bruker Co., Germany) to identify the structural information. A standard tensile testing machine (Instron 5567 Electronic Universal Testing Machine, Model 5567, USA) was used to test the mechanical performance of dumbbell specimens, and an ARES dynamic mechanical analysis (DMA) testing instrument (Advanced Rheometric Expansion System) from Rheometric Scientific (now belonging to Waters, Inc., BU TA Instruments) was used in the torsion mode. A scanning electron microscope (Quanta 650 FEG, FEI Company, Hillsboro, Oregon, USA) with an accelerating voltage of 15 kV GPC was used to investigate the morphology of the samples in the simulated propellants. The XPS spectra were recorded by a PHI 5300 X-ray photoelectron spectrometer (PerkinElmer Physical Electronics Co., USA) at an operating power of 250 W. The C, H, and N element analyses of the samples were carried out using a MikroKemi AB (Uppsala, Sweden). Gel-permeation chromatography (GPC) analysis was conducted by Polymer Standards Service (PSS) in Mainz, Germany using polystyrene (PS) calibration standards and DMF as a solvent with a speed of 1.0 mL min−1.
Preparation of the NPBAs
As shown in Scheme 1, NPBA-2 was synthesized using a previously reported procedure similar to that of NPBA-1 by adding glycidyl methacrylate in this work.13 Therefore, there are two units in the structure of NPBA-2: acrylonitrile and glycidyl methacrylate, which is different from NPBA-1 with its terminal hydroxyl groups. Typically, azobisisobutyronitrile (AIBN, approximately 3.00 g, 18.3 mmol) was dissolved in an acetone solution (125 mL) containing other materials: acrylonitrile (53.08 g, 1.00 mol), 2-hydroxyethyl acrylate (about 23.29 g, 0.20 mol), and 2-mercaptoethanol (3.92 g, 50.2 mmol). Then, the mixed solution was transferred to a round-bottomed flask (250 mL) and heated to 60 °C with magnetically stirring for 6 h. After cooling down to room temperature, the solution was poured into 1250 mL methanol with stirring and settled for 10 min to form a precipitation. The supernatant solvent was removed and methanol was added under continuous stirring for 10 min. The precipitation process was repeated several times. The mixture was left overnight. The final product was dried at room temperature under a high vacuum for 24 h. Both NPBA-1 and NPBA-2 are brittle solid polymers and are easily ground in a mortar and pestle into white powders for further use in propellant formulations. An analogous hydroxyl group-capped NPBA-1 based on acrylonitrile and glycidyl methacrylate was also prepared in a similar manner. GPC analysis of NPBA-1 and NPBA-2 (DMF, 1.0 mL min−1, PS calibration): Mn = 1920, Mw = 2530, D = 1.43 and Mn = 2060, Mw = 3210, D = 1.56, respectively.
Static thermal stability test
The static thermal stability of the samples was investigated using a Netzsch STA449F3 instrument. Generally, about 1 to 2 mg samples were placed in an alumina crucible with a heating rate of 10 K min−1 under N2 atmosphere with a flow rate of 60 mL min−1 from 50 to 800 °C. The TG curves were recorded from 20 to 110 °C by a DSC Waters Corp. 2920, and the time required to decompose 1% mass was recorded accordingly.
Preparation of the composite rocket propellants
To investigate the effects of the as-prepared NPBAs in the composite rocket propellants, the NPBAs were applied to the AP/HMX/GAP formulation energetic propellant system. The formulation of the mixture had 77 mass % solid content. The R value of the binder system was about 1.0. The formulation slurries were all kneaded and cast as a normalization process, which occurred at the temperature of 50 °C for 7 d.23 According to the ASTM1708-95 standard, the sample was sliced, cut into dumbbell-shaped pieces, and then tested for its mechanical properties using an Instron 5567 Electronic Universal Testing Machine (Model 5567, USA). The different mechanical properties of the as-prepared NPBA-1 and NPBA-2 were tested at −40 °C, 20 °C, and 70 °C with the speed of 100 mm min−1. The related parameters of the maximum tensile strength (σm), initial modulus (E0), elongation at maximum tensile strength (εm), and elongation at break (εb) were calculated by the stress–strain curves.24
Morphology and interfaces of the composite rocket propellants
Mixed samples of RDX with various bonding agents were synthesized as follows. NPBA-1 or NPBA-2 were dissolved in ethanol (0.5 g per 10 mL) and separately mixed with RDX (1 g). A similar composite ratio was used in the HMX system. The mixture was filtered after being stirred at 50 °C for 0.5 h and washed with fresh ethanol several times. The RDX/NPBA mixtures were dried at 50 °C for at least two days. The morphologies and interactions between the NPBAs and RDX or HMX were investigated by SEM and XPS.
Results and discussion
Structure analyses of the NPBAs
The 1H NMR and 13C NMR results of the as-prepared bonding agents NPBA-1 and NPBA-2 are shown in Fig. 1 and Tables S1 and S2.†
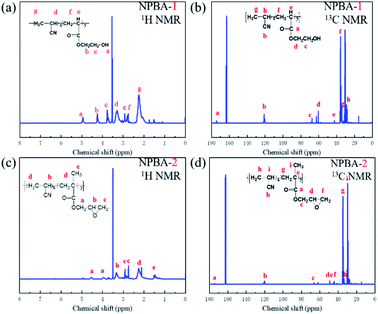 |
| Fig. 1 (a) The 1H NMR spectrum of NPBA-1 (b) 13C NMR spectrum of NPBA-1 (c) the 1H NMR spectrum of NPBA-2 (d) 13C NMR spectrum of NPBA-2. | |
As shown in Fig. 1a, there are seven different chemical shifts from a to g in the 1H NMR spectrum of NPBA-1, and the detailed groups are listed in Table S1.† Meanwhile, five proton signals are observed in the 1H NMR spectrum of NPBA-2, indicating different molecular structures. The 1H NMR spectra of both NPBA-1 and NPBA-2 show similar proton signals for –CN at 3.45 to 3.10 ppm and 3.45 to 3.20 ppm,25 respectively. In addition, the signals located at 3.82 to 3.74 ppm for NPBA-1 could be indexed to the
groups, while the signals at 3.45 to 3.20 ppm for NPBA-2 are assigned to the OCH2CH*CH2O groups. To further compare the differences between NPBA-1 and NPBA-2, 13C NMR tests were performed in DMSO-d6 at 125 MHz. As shown in Fig. 1b and d, the chemical shift of the –CN groups in NPBA-1 and NPBA-2 are located at 121.6 to 120.6 ppm and 121.6 to 120.3 ppm, respectively. The different terminal groups have an impact on the position of the chemical shift for –CN. In addition, the chemical shifts located at 67.8 to 67.0 ppm and 60.6 to 60.4 ppm are assigned to the OC*H2 and OC*H2CH2OH groups in NPBA-1, respectively. As shown in the 13C NMR spectrum of NPBA-2, the signals of C*HOCH2 and CHOC*H2 are attributed to the chemical shifts located at 50.0 to 49.8 and 49.8 to 45.2, respectively.
The functionalization groups of the as-prepared NPBA-1 and NPBA-2 are further detected using Fourier transform infrared spectroscopy. The FT-IR absorption vibration peaks of the hydroxyl, cyano and ester groups, located at ∼3489 cm−1, 2243 cm−1 and 1733 cm−1, respectively,26,27 were observed in the FT-IR spectrum of NPBA-1 (Fig. 2a). Meanwhile, the absorption vibration peaks of NPBA-2 located at 3440 cm−1 and 2244 cm−1, as well as 1456, 1170, and 1074 cm−1, were ascribed to the hydroxyl groups, –C
N groups and benzene rings,28 respectively (Fig. 2b). Therefore, it was confirmed that the as-prepared NPBA-1 possessed hydroxyl and cyano groups and NPBA-2 possessed cyano and epoxy groups, which are matches well with the structure design.
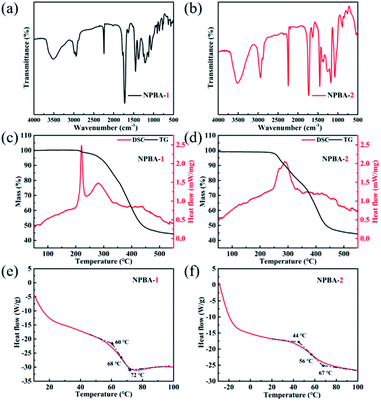 |
| Fig. 2 The FT-IR spectra of the as-prepared (a) NPBA-1 and (b) NPBA-2. The TGA-DTA curves of the as-prepared (c) NPBA-1 and (d) NPBA-2. The glass transition temperature of the as-prepared (e) NPBA-1 and (f) NPBA-2. | |
Thermodynamic behaviors are important determining factors for bonding agents in fabricating composite solid propellants. As shown in Fig. 2c, the thermogravimetric and differential scanning calorimetry (TG-DSC) results show that the thermal stability of NPBA-1 reached about 300 °C,29 which is higher than that of some reported bonding agents. In addition, the as-prepared NPBA-2 exhibits a decomposition temperature starting from about 250 °C, lower than that of NPBA-1. Furthermore, the Tg of NPBA-2 is 56 °C, which is lower than that of NPBA-1 (68 °C). The differences between NBPA-1 and NBPA-2 are attributed to the degree of polymerization and functional groups. The lower Tg makes NPBA-2 easily soften,30 which would be beneficial to the interfacial contact with explosives in composite solid propellants.
Interaction between NPBA-2 and solid fillers in propellants
The excellent thermal stability and abundance of hydroxyl groups and –C
N groups encouraged us to explore the applications of the as-prepared NBPA-2 in the field of composite solid propellants. NPBA-2 was separately mixed with HMX and RDX in a low molar ratio. As shown in Fig. 3, the morphologies of both HMX and RDX exhibit a blocky structure with a pristine surface. The particle size of HMX is about 100–200 μm. After coating with NPBA-2, the particle sizes of the explosives are not obviously changed, while small particles with a size of several micrometers can be observed on the surface of HMX. Due to the small amount of the bonding agents, the mass ratio of the bonding agents and HMX (RDX) being 50
:
1, and the fact that they are solid materials, the surface coating amount of the bonding agents is limited. Similarly, the mixture of RDX and NPBA-2 shows good compatibility. Therefore, the as-prepared NPBA-2 was coated on the surface of HMX and RDX very well due to the functionalized groups. The numerous active sites of cyano groups in NPBA-2 could bind polar fillers strongly, which is matched well with Kim's phase separation theory.31 Furthermore, the molecular structure makes NPBA-2 easily separate from ethanol at a lower temperature, which then can be deposited and eventually coated on the surface of nitramine solid particles.
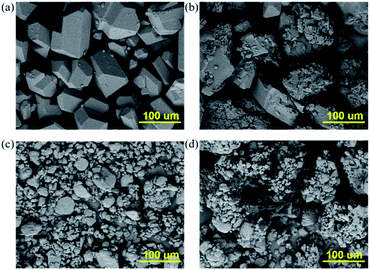 |
| Fig. 3 The morphologies of HMX (a) before and (b) after coating with NPBA-2. The morphologies of RDX (c) before and (d) after coating with NPBA-2. | |
Additionally, the bonding effects and interactions between NPBA-2 and various explosives were investigated using X-ray photoelectron spectroscopy (XPS). During XPS measurements, the charge problem was solved by a flood gun. The XPS instrument manufacturer was Thermo Fisher (USA), the model was an ESCALAB 250 Xi; excitation source: Al Kα (1486.7 eV), power: 150 W (15 kV, 10 mA), vacuum degree: 10−7 Pa (10−9 Torr), charged calibration was based on the adsorption of C 1s (284.8 eV), pass energy: 30 eV, and the data processing software was Avantage. As shown in Fig. 4a, the signals of N, O and C appear in the XPS spectra of HMX before and after NPBA-2 mixing. The signals of N, O and C appear in the XPS spectra of NPBA-2 (Fig. S2†). In addition, the N 1s spectrum was located at 397.02 eV, which is attributed to the groups of –C
N. The N 1s XPS spectra of HMX and the mixture of HMX and NPBA-2 are presented in Fig. 4b. The N 1s peaks of the pure HMX crystal were located at 405.04 and 399.54 eV,32 which are attributed to the –NO2 group and N–NO2 group, respectively. After coating with NPBA-2, a new peak appears at 397.34 eV, which is attributed to the cyano groups (–CN). Moreover, the original N 1s peaks shift to a lower binding energy by around 0.50 eV, indicating the interactions between the bonding agent and HMX. As shown in Fig. 4c and d, the electron density of –NO2 on the outer shell increased and the XPS peaks for N 1s of –NO2 and N–NO2 in the mixture of RDX and NPBA-2 moved towards lower energies by about 0.15 eV after mixing with the bonding agent NPBA-2. This phenomenon also suggests the strong interaction between explosives and NPBA-2. The excellent compatibility and strong interaction make NPBAs promising candidates in composite solid propellants.
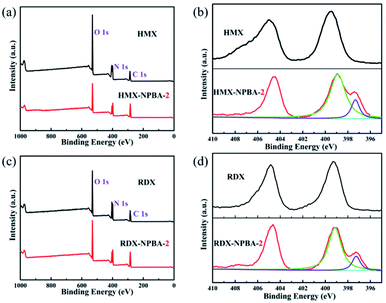 |
| Fig. 4 (a) The XPS spectra of pure HMX and mixture of HMX and NPBA-2. (b) The N 1s XPS spectra of pure HMX and mixture of HMX and NPBA-2. (c) The XPS spectra of pure RDX and mixture of RDX and NPBA-2. (d) The N 1s XPS spectra of pure RDX and mixture of RDX and NPBA-2. | |
Practical application of NPBAs in propellant systems
The prepared NPBA-1 and NPBA-2 were applied to the AP/HMX/GAP formulation to evaluate their potential for practical application. The mechanical properties of different propellant systems were examined at −40 °C, 20 °C, and 70 °C (Fig. 5). As shown in Table 1, the control sample without bonding agents presented weak mechanical properties at different temperatures, while the strength and elongation of the formulations were obviously improved after NPBA-1 or NPBA-2 addition. NPBA-2 exhibited better effects than NPBA-1, including in terms of strength and elongation, due to the different functional groups and decreased glass transition temperature. As demonstrated above, a lower glass transition temperature in NPBAs is conducive to the phase separation of the bonding agent from the propellant system. In addition, the bonding agents have little influence on the burning of propellants. The oxygen balance was calculated as below.
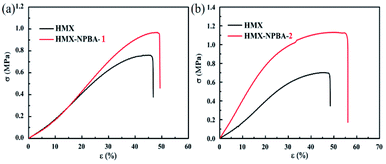 |
| Fig. 5 The mechanical properties of the control propellant system with the AP/HMX/GAP formulation and adding (a) NPBA-1 and (b) NPBA-2. | |
Table 1 Effects of the copolymerization ratio of NPBA on the mechanical properties of the AP/HMX/GAP propellant
Conditions |
−40 °C, σm/εm/εb, MPa/%/% |
20 °C, σm/εm/εb, MPa/%/% |
70 °C, σm/εm/εb, MPa/%/% |
Control |
2.0/20.8/32.2 |
0.56/25.8/30.5 |
0.25/24.8/30.5 |
NPBA-1 |
2.5/30.1/32.6 |
0.68/36.0/37.9 |
0.35/25.2/26.8 |
NPBA-2 |
3.0/34.7/36.8 |
0.77/46.3/48.0 |
0.40/33.2/35.8 |
The structural unit of the bonding agent of NPBA-1 is C8H11O3N and the molecular weight is 169.
The burning of 169 g NPBA-1 requires 296 g oxygen (corresponding to 37/4 oxygen atoms), and consequently the burning of 100 g NPBA-1 requires 296/169 × 100 = 175.15 g oxygen; thus, the oxygen balance of the bonding agent NPBA-1 is −175.15%.
The structural unit of NPBA-2 is C10H13O3N and the molecular weight is 195.
The burning of 195 g NPBA-2 requires 376 g oxygen (corresponding to 13/2 oxygen atoms), and consequently the burning of 100 g NPBA-2 requires 376/195 × 100 = 192.82 g oxygen; thus, the oxygen balance of the bonding agent NPBA-2 is −192.82%.
The bonding agents mainly affect the mechanical properties and aging properties of propellants due to them constituting only 0.05–0.5% of the formula components of the propellants. Depositing a large amount of NPBA on the surface of solid fillers would improve the interaction between the solid fillers and the binder.
Conclusions
In the present work, two new types of bonding agents based on acrylonitrile as the raw material were synthesized. The 1H NMR, 13C NMR, TGA-DSC and FT-IR spectra were recorded to confirm the molecular structures of NPBA-1 and NPBA-2. Their strong interactions with the two explosive materials RDX and HMX indicated the effects of functionalized polar groups on both bonding agents. The bonding agent NPBA-2, which is based on acrylonitrile and glycidyl methacrylate, exhibited a lower Tg than that of NPBA-1. Therefore, the NPBA-2-containing AP/HMX/GAP formulations presented enhanced mechanical properties, showing maximum elongation ratios from 20.8%, 25.8%, and 24.8% to 35.6%, 44.9%, and 32.5% at −40 °C, 20 °C, and 70 °C, respectively. This work shows an efficient route to improve the mechanical properties of composite solid propellants by designing and synthesizing functionalized NPBAs.
Conflicts of interest
There are no conflicts to declare.
Acknowledgements
This work was supported by the Project of Science Foundation of Aerospace (Grant No. 514010307-05) and Open Research Fund Program of Science and Technology on Aerospace Chemical Power Laboratory (Grant No. STACPL220181B04).
References
- A. C. G. Miguel, F. C. Silva and G. da Silva, Short Review about Aerospace Materials Characterization- Bonding Agents and Thermal Insulation, Propellants, Explos., Pyrotech., 2020, 45, 1175 CrossRef.
- A. M. Abd elall and G. Lin, Synthesis of Long Chain Bonding Agent of CSRP and its Effect on Propellant Mechanical Properties, Propellants, Explos., Pyrotech., 2018, 43, 904 CrossRef CAS.
- T. F. Nihal and U. B. Zht, The Effect of Ammonium Nitrate, Coarse/Fine Ammonium Nitrate Ratio, Plasticizer, Bonding Agent, and Fe2O3 Content on Ballistic and Mechanical Properties of Hydroxyl Terminated Polybutadiene Based Composite Propellants Containing 20% AP, J. ASTM Int., 2005, 2, 233 Search PubMed.
- A. A. Ali, J. W. Zhang and G. B. Cai, Investigation of the Role of Aziridine Bonding Agents on the Aging of the Composite Solid Rocket Propellant (CSRP), J. Aerosp. Power, 2008, 23, 2101 Search PubMed.
- J. Sciamareli, M. F. K. Takahashi and J. M. Teixeira, Solid Polyurethane-Based Composite Propellant: I-Influence of the Bonding Agent, Quim. Nova, 2002, 25, 107 CrossRef.
- A. E. Oberth and R. S. Bruenner, Polyurethane-based propellants, in Advances in Chemistry Series 88-Propellants Manufacture, Hazards, and Testing, ed. R. F. Gould, ACS Publication, 1969, p. 84 Search PubMed.
- K. Hasegawa, M. Takizuka and T. Fukuda, Bonding Agents for AP and Nitramine/HTPB Composite Propellants, AIAA/SAE/ASME, 19th Joint Propulsion Conference, Washington, 1983, p. 1199 Search PubMed.
- D. C. Pires, A. M. Kawamoto and J. Sciamareli, Synthesis and Characterization by Infrared Spectroscopy of Hydantoin-Based Bonding Agents, Used in Composite Propellants, J. Aerosp. Technol. Manage., 2009, 1, 55 CrossRef CAS.
- A. Yada, C. S. Pant and S. Das, Research Advances in Bonding Agents for Composite Propellants, Propellants, Explos., Pyrotech., 2020, 45, 695 CrossRef.
- H. X. Tang, X. L. Liu and Q. Wu, Action Mechanism of Special Functional Agents in Composite Solid Propellant.(IV)- Processability/Mechanical Properties, J. Solid Rocket Technol., 2004, 27, 193 CAS.
- Y. Wang and G. Qiu, Thermal Behavior of Bonding Agent 1,1-(1,3-Phenylenedicarbonyl)Bis-2-methyl-aziridine, J. Propul. Technol., 2001, 22, 258 CAS.
- J. P. Consaga, Dimethyl Hydantoin Bonding Agents in Solid Propellants, US Pat., 4214928, 1980 Search PubMed.
- C. S. Kim, H. Youn and P. N. Noble, Development of neutral polymeric bonding agents for propellants with polar composites filled with organic nitramine crystals, Propellants, Explos., Pyrotech., 1992, 17, 38 CrossRef CAS.
- C. S. Kim, P. N. Noble and C. H. Youn, The Mechanism of Filler Reinforcement from Addition of Neutral Polymeric Bonding Agents to Energetic Polar Propellants, Propellants, Explos., Pyrotech., 1992, 17, 51 CrossRef CAS.
- C. S. Kim, Filler Reinforcement of Polyurethane Binder Using a Neutral Polymeric Bonding Agent, US Pat., 4915755, 1990 Search PubMed.
- Y. L. Wang, W. Zhang and K. C. Xiao, Experimental and simulation study of the phase separation of neutral polymeric bonding agent in nitrate ester plasticized polyether propellant and its application, Colloids Surf., A, 2020, 610 DOI:10.1016/j.colsurfa.2020.125665.
- R. Sanan and R. K. Ma ha jan, Polyethylene glycol assisted micellar, interfacial and phase separation studies of triblock copolymer-nonionic surfactant mixtures, Colloids Surf., A, 2013, 433, 145 CrossRef CAS.
- S. Xiang, Z. H. Guo and Y. L. Wang, The microstructure regulation, strengthening, toughening and hydrophilicity of polyamide 6 in fabricating poly (vinylidene fluoride)-based flat membrane via the thermally induced phase separation technique, Eur. Polym. J., 2020, 126, 109568 CrossRef CAS.
- F. Wang, P. Altschuh and L. Ratke, Progress report on phase separation in polymer solutions, Adv. Mater., 2019, 31, 1806733 CrossRef PubMed.
- W. Hu, Y. Su, L. Zhou and A. Pang, Molecular Dynamics of Neutral Polymeric Bonding Agents (NPBA) as Revealed by Solid-State NMR Spectroscopy, Molecules, 2014, 19, 1353 CrossRef PubMed.
- S. Zhou, G. Tang, A. Pang, X. Guo, F. Wu, H. Song, X. Xu, X. Hu and Y. Wang, Synthesis of an alkynyl neutral polymer-bonding agent and its enhancing effect on tensile strength of glycidyl azide polymer-based propellants, Iran. Polym. J., 2019, 28, 943–955 CrossRef CAS.
- F. Gholamian, M. Ansari and M. Abdullah, et al. Intermolecular interaction of a neutral poly-meric bonding agent containing N-vinylpyrrol-idone units with ammonium perchlorate and Ke-to-RDX, Chin. J. Polym. Sci., 2013, 31, 1372–1381 CrossRef CAS.
- K. Tejasvi, V. Venkateshwara Rao, Y. PydiSetty and K. Jayaraman, Studies on Aluminum Agglomeration and Combustion in Catalyzed Composite Propellants, Combust., Explos. Shock Waves, 2021, 57, 203–214 CrossRef.
- D. M. Zhou, X. Y. Liu and X. Sui, et al., Effect of Pre-strain Aging on the Damage Properties of Composite Solid Propellants based on a Constitutive Equation, Propellants, Explos., Pyrotech., 2017, 42, 430–437 CrossRef CAS.
- X. L. Yang, Y. J. Lei and J. C. Zhong, et al., Preparation and thermal properties of novel phthalonitrile oligomer containing biphenyl ethernitrile/bisphthalonitrile blends, Appl. Polym. Sci., 2010, 119, 882–887 CrossRef.
- R. C. M. Santos, P. CristinaGurgel and N. SimenremisPereira, et al., Ethyl esters obtained from pequi and macaúba oils by transesterification with homogeneous acid catalysis, Fuel, 2020, 259 DOI:10.1016/j.fuel.2019.116206.
- M. Makarem, C. M. Lee and D. Sawada, Distinguishing Surface versus Bulk Hydroxyl Groups of Cellulose Nanocrystals Using Vibrational Sum Frequency Generation Spectroscopy, J. Phys. Chem. Lett., 2018, 9(1), 70–75 CrossRef CAS PubMed.
- Z. Hu, S. B. Ge and J. Yang, et al., Molecular characteristics and function of elliptical Kiwifruit, J. King Saud Univ., Sci., 2020, 32, 1884–1888 CrossRef.
- J. Singh, R. J. Staples and J. P. Hooper, et al., Pyrazole Bridges Ensure Highly Stable and Insensitive Bistetrazoles, Chem. Eng. J., 2022, 431 DOI:10.1016/j.cej.2021.133282.
- C. F. He, P. R. Christensen and T. J. Seguin, et al., Conformational Entropy as a Means to Control the Behavior of Poly(diketoenamine) Vitrimers In and Out of Equilibrium, Angew. Chem., Int. Ed., 2020, 59, 735–739 CrossRef CAS PubMed.
- C. S. Kim, Filler Reinforcement of Polyurethane Binder Using Neutral Polymeric Bonding Agent, US Pat., 4915755, 1990 Search PubMed.
- J. R. Zhang, Y. Ma and S. Y. Wang, et al., Accurate K-edge X-ray photoelectron and absorption spectra of g-C3N4 nanosheets by first-principles simulations and reinterpretations, Phys. Chem. Chem. Phys., 2019, 21, 22819–22830 RSC.
|
This journal is © The Royal Society of Chemistry 2022 |
Click here to see how this site uses Cookies. View our privacy policy here.